Information
- Author Services
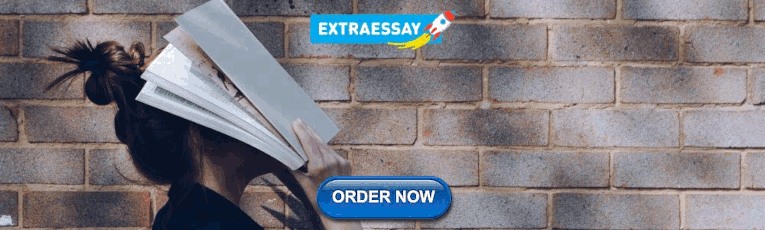
Initiatives
You are accessing a machine-readable page. In order to be human-readable, please install an RSS reader.
All articles published by MDPI are made immediately available worldwide under an open access license. No special permission is required to reuse all or part of the article published by MDPI, including figures and tables. For articles published under an open access Creative Common CC BY license, any part of the article may be reused without permission provided that the original article is clearly cited. For more information, please refer to https://www.mdpi.com/openaccess .
Feature papers represent the most advanced research with significant potential for high impact in the field. A Feature Paper should be a substantial original Article that involves several techniques or approaches, provides an outlook for future research directions and describes possible research applications.
Feature papers are submitted upon individual invitation or recommendation by the scientific editors and must receive positive feedback from the reviewers.
Editor’s Choice articles are based on recommendations by the scientific editors of MDPI journals from around the world. Editors select a small number of articles recently published in the journal that they believe will be particularly interesting to readers, or important in the respective research area. The aim is to provide a snapshot of some of the most exciting work published in the various research areas of the journal.
Original Submission Date Received: .
- Active Journals
- Find a Journal
- Proceedings Series
- For Authors
- For Reviewers
- For Editors
- For Librarians
- For Publishers
- For Societies
- For Conference Organizers
- Open Access Policy
- Institutional Open Access Program
- Special Issues Guidelines
- Editorial Process
- Research and Publication Ethics
- Article Processing Charges
- Testimonials
- Preprints.org
- SciProfiles
- Encyclopedia
Journal Description
Agriculture.
- Open Access — free for readers, with article processing charges (APC) paid by authors or their institutions.
- High Visibility: indexed within Scopus , SCIE (Web of Science) , PubAg , AGRIS , RePEc , and other databases .
- Journal Rank: JCR - Q1 ( Agronomy ) / CiteScore - Q1 ( Plant Science )
- Rapid Publication: manuscripts are peer-reviewed and a first decision is provided to authors approximately 20.2 days after submission; acceptance to publication is undertaken in 2.3 days (median values for papers published in this journal in the first half of 2024).
- Recognition of Reviewers: reviewers who provide timely, thorough peer-review reports receive vouchers entitling them to a discount on the APC of their next publication in any MDPI journal, in appreciation of the work done.
- Companion journals for Agriculture include : Poultry , Grasses and Crops .
Latest Articles
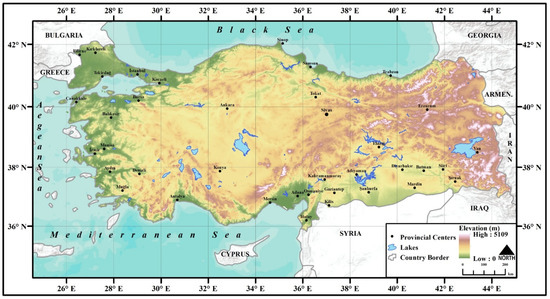
Journal Menu
- Agriculture Home
- Aims & Scope
- Editorial Board
- Reviewer Board
- Topical Advisory Panel
- Instructions for Authors
Special Issues
- Article Processing Charge
- Indexing & Archiving
- Editor’s Choice Articles
- Most Cited & Viewed
- Journal Statistics
- Journal History
- Journal Awards
Conferences
- Editorial Office
Journal Browser
- arrow_forward_ios Forthcoming issue arrow_forward_ios Current issue
- Vol. 14 (2024)
- Vol. 13 (2023)
- Vol. 12 (2022)
- Vol. 11 (2021)
- Vol. 10 (2020)
- Vol. 9 (2019)
- Vol. 8 (2018)
- Vol. 7 (2017)
- Vol. 6 (2016)
- Vol. 5 (2015)
- Vol. 4 (2014)
- Vol. 3 (2013)
- Vol. 2 (2012)
- Vol. 1 (2011)
Highly Accessed Articles
Latest books, e-mail alert.
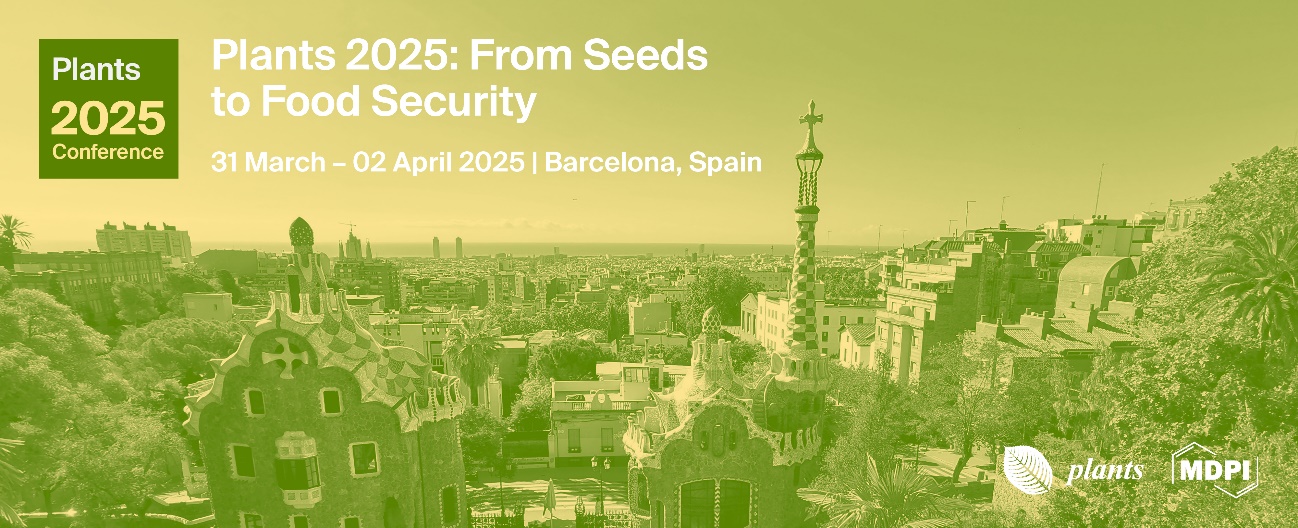
Further Information
Mdpi initiatives, follow mdpi.
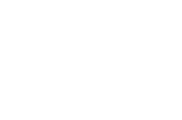
Subscribe to receive issue release notifications and newsletters from MDPI journals
Development of a Technology Valuation Model for Commercial Licensing: An Empirical Case of PB 1718 Basmati Rice Variety
- Stanishkar Thevaruparambil Sunil
- Akriti Sharma
- Girish Kumar Jha
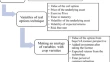
Morphological and Molecular Evidence of Fish Parasite Gyrodactylus kobayashi and Argulus japonicus Isolated from Ornamental Fish ( Carassius auratus )
- Basanta Kumar Das
- Souvik Dhar
- Bijay Kumar Behera
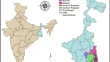
Application of Vegetation Indices to Determine the Reproductive Development of Açaí in the Eastern Amazon
- Jamile do Nascimento Santos
- Izadora de Cássia Mesquita da Cunha
- Fábio Júnior de Oliveira

Design, Development and Performance Evaluation of a Portable Battery-Operated Litter Agitator Installed with AI-Controlled Obstacle-Avoidance Robot with Sensors
- Rohit Bhojyareddy Gaddamwar
- Ajaya Kumar Dash
- Susant Kumar Dash
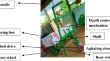
Specific Glycoprotein E ( gE ) Gene Based Nested Polymerase Chain Reaction Assay for Detection of Marek’s Disease Virus in Chickens
- Muskan Bhadok
- Adarsh Mishra
- Yashpal Singh Malik
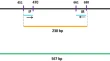
Influence of Histology and Histomorphometry of Thigh Muscles on Thermal Stress-Related Physiological and Plasma Hormonal Responses in Three Broiler Strains
- Haresh Kashinathrao Popalghat
- Nrusingha Charan Behura
- Soubhagya Muduli
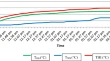
Characterization of Spreadable Goat Cheese from Animals Fed with Cactus ( Opuntia ficus-indica ) Cladodes as a Productive Alternative in Arid and Semiarid Areas
- Florencia Frau
- Jorge Nelson Leguizamón-Carate
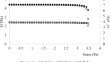
Development of a Power Operated Fenugreek Thresher
- C. Naveen Kumar
- R. K. Rathod
- S. M. Mathur
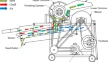
Molecular Characterisation and Dynamics of the Fusion Protein of an Emerging Genotype VIIi of Newcastle Disease Virus
- Rafia Maqbool
- Sabia Qureshi
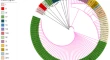
Comparative Metatranscriptomics of Rhizosphere Microbiomes in Survived and Dead Cocoa Plants Under Drought Condition
- Norasekin Tamchek
- Ping-Chin Lee

Problems and Its Related Factors Affecting the Hatchery Owners in Producing Fish Seeds in Rural Bangladesh
- Mohammed Nasir Uddin
- Arup Kumer Das
- M. Salim Uddin
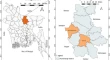
Identification and Differentiation of Mustard Crop with Associated Other Land Cover Features Using Multi-temporal Synthetic Aperture Radar (SAR) and Multispectral Instrument (MSI) Data with Machine Learning Approach Over Haryana, India
- Sultan Singh
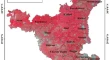
Soil Water Balance and Productivity of Different Maize-Based Crop Sequences Compared to Rice–Wheat System under Contrasting Irrigation Regimes in North–West India
- Navneet Kaur
- Krishan Kumar Vashist
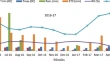
Soil Erosion Assessment Using the RUSLE Model, Remote Sensing, and GIS in the Woybo Watershed, Ethiopia
- Mudesir Nesru
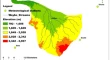
Soil Attributes Modulate the Fungal Population and Diversity of Phytopathogens and Biocontrol Agents
- Sumit K. Soni
- Poonam C. Singh
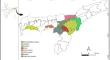
Exploring Oxyfluorfen's Environmental Fate: Soil Affinity, Persistence, Degradation Dynamics and Ecological Implications
- Khuram Shahzad Ahmad
- Abdulnasser M. Karami
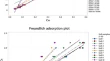
Examining the Prevalence and Predictors of Stunting in Indian Children: A Spatial and Multilevel Analysis Approach
- Venkata Naga Sindhuja Padigapati
- Selvaprakash Ramalingam
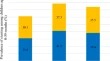
Buzzing for Broccoli ( Brassica oleracea var. italica ): Exploring Insect Pollinators, Their Behaviour, Single-Visit Efficiency and the Significance of Honey Bees in Yield Enhancement
- Sunaullah Bhat
- Johnson Stanley
- Sandeep Kumar
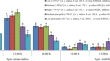
Predatory Behavior of Wasp Species, Antagonistic Defense Mechanism of Apis mellifera Honey Bees and Effective Wasp Management in Apiaries
- Mohammad Ilyas Motmayen
- Surender Kumar Sharma

An Investigation on the Present Status of Wetlands in Majuli River Island; The World Largest River Island and Its Fishery Resources
- Moirangthem Kameshwor Singh
- Chandopal Saikia
- Prabin Payeng
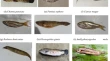
Quantitative Analysis on Expression of Insecticidal Crystal Proteins in Different Plant Parts of BG-II Cotton Hybrids at Various Phenological Stages
- Debashis Paul
- Rishi Kumar
- Y. G. Prasad

Level of Awareness and Willingness to Pay for Safe Milk: A Study of Urban Consumers in North India
- Indrajit Mondal
- Gunjan Bhandari
- Udita Chaudhary
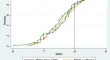
Variability in Seed Morphology, Germination, and Seedling Growth of Madhuca indica : Implications for Seed Source Selection
- Swati Shedage
- Dipika Aayate
- M. J. Dobriyal
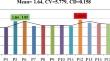
Economic and Technical Assessment of the Chinese Plum Varieties Using Multi-Criteria Analysis Methods
- Miroslav Nedeljković
- Jonel Subić

Assessment of Production and Soil Conservation Potential of Aromatic Grasses Grown Under Shifting Cultivated Degraded Hill Slopes of Eastern Ghats, India
- H. C. Hombegowda
- P. P. Adhikary
- Md. Basit Raza

Genetic Analysis of a Recombinant Inbred Line Population Derived from Salt-Tolerant Rice Landrace Korgut under Coastal Ecology
- K. K. Manohara
- Yogini Shanbhag
- Nagendra Kumar Singh
Impact of Plant Density and Irrigation Regimes on Physiological and Biochemical Responses of Cumin ( Cuminum cyminum )
- Abolfazl Sardashti-Nahi
- Hamidreza Ganjali
- Ahmad Mehraban
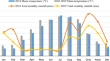
Development of an IOT-Based Semi-Autonomous Vehicle Sprayer
- Mrutyunjay Padhiary
- Sunny V. Tikute
- Laxmi Narayan Sethi

Engineering Properties of Indian Browntop Millet ( Brachiaria ramosa) as Influenced by Varietal and Moisture Differences
- Amisha Kaushik
- Dharmesh Chandra Saxena
- Sukhcharn Singh
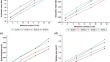
Quantifying Climate Influence on Net Ecosystem Exchange in Lowland Tropical Rice: A Five-Year Eddy Covariance Study
- Chinmaya Kumar Swain
- Amaresh Kumar Nayak
- Nihar Ranjan Singh
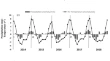
Effect of Various Nitrogen and Sulfur Sources on Maize-Wheat Yield and N:S Uptakes Under Two Different Climatic Conditions
- Dost Muhammad
- Maria Musarat
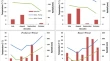
Analysing Land Use and Cover Transformations in Berhampore, West Bengal, India: A CA-Markov and ANN Simulation Approach for Future Predictions
- Md. Mustaquim
- Woheeul Islam
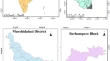
Soil Fertility, Physiological Traits, and Fruit Quality of Morinda citrifolia as Influenced by Agroecological Management Practices in a Tropical Ferralsol
- Aline Cavalcanti Dantas
- Tancredo Augusto Feitosa de Souza
- Larissa Nicássio Pessoa
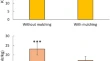
Enhancing Wheat Yield and Zinc Biofortification through Synergistic Action of Potent Zinc-Solubilizing Bacteria and Zinc Sulfate in Calcareous Soil
- Iftikhar Ahmed
- Muhammad Sharif
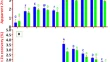
Field Assessment of a Plant Growth-Promoting Pseudomonas on Phytometric, Nutrient, and Yield Components of Maize in a Milpa Agrosystem
- Blanca Rojas-Sánchez
- Ma. del Carmen Orozco-Mosqueda
- Gustavo Santoyo
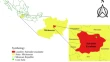
Fruiting and Physicochemical–Biochemical Characteristics of Apricot ( Prunus armeniaca ) Cultivar ‘New Castle’ as Influenced by Foliar Application of Antioxidants and Phytoregulators
- Neha Thakur
- Gopal Singh
- Uday Sharma

Correction: Image-Based Appraisal of Woody Starch Reserves in Grapevine
- Daniel Grigorie Dinu
- Vitale Nuzzo
- Laura Rustioni
Assessment of Hardseededness and Its Seasonal Dynamics Through Scanning Electron Microscopy in Green Gram ( Vigna radiata ) Genotypes
- S. K. Chakrabarty
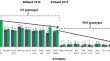
Chemical Characterization of Rare Unifloral Honeys of Ailanthus ( Ailanthus altissima ), Fennel ( Foenicum vulgare ), and Raspberry ( Rubus idaeus ) and their Antimicrobial and Antioxidant Activity
- Lara Saftić Martinović
- Nada Birkic
- Sandra Pedisić
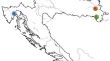
Impact of Bio-based and Synthetic Phosphorus Application on Growth, Yield, and Protein Profile of Two Chickpea Genotypes
- Shno Othman Sofi
- Shahen Kamil Talabani
- Hawar Sleman Halshoy

Optimizing Seed Physiological Maturity and Quality in Camelina Through Plant Density Variation: A Nonlinear Regression Approach
- Esmaeil Bakhshandeh
- Raoudha Abdellaoui
- Najmeh Mirzaaghpour
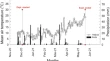
Frequency-Dependent Pre-Sowing Magneto-Priming of Anise Seeds Affecting Their Productivity
- Haitham S. Mohammed
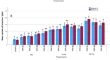
Amylase Activity and Soluble Sugars Content of Durum Wheat Seeds During Germination Under Water Stress
- Kamel Zemour
- Othmane Merah
Assessment of Lung Damage via Mitochondrial ROS Production Upon Chronic Exposure to Fipronil and Imidacloprid
- Gurvinder Kaur
- Sheza Farooq
- R. S. Sethi
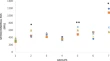
Biochar and AMF Improve Growth, Physiological Traits, Nutrients of Turmeric and Soil Biochemical Properties in Drought Stress
- Dilfuza Jabborova
- Pradyumna Kumar Singh
- Joginder Singh Duhan
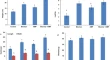
Nucleoredoxin Vis-à-Vis a Novel Thioredoxin in Regulating Oxidative Stress in Plants: A Review
- Soham Hazra
- Avishek Chatterjee
- Poulomi Sen
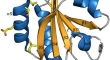
Co-application of Green Manure and Trichoderma spp. Induced Plant Growth Promotion by Nutrient Improvement and Increased Fungal Biomass in Soil
- Waleed Asghar
- Ryota Kataoka

Carbon Footprint and Emission Reduction Strategies During Potato Cultivation
- Jatish Chandra Biswas
- Md Mozammel Haque
- Pil Joo Kim
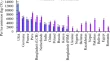
Impact of Conservation Agriculture on Soil Organic Carbon Sequestration and Enzyme Activity Under Rice–Wheat Cropping System in a Vertisol
- Ranjan Bhattacharyya
- Chaitanya Prasad Nath
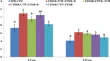
The Use of Indigenous Knowledge Systems Practices to Enhance Food Security in Vhembe District, South Africa
- Melanie D. Nicolau
- Shandukani C. Nenwiini
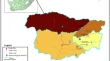
- Find a journal
- Publish with us
- Track your research
- Email Alert
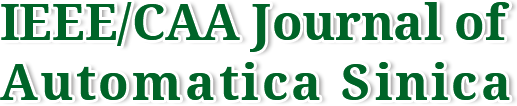
论文 全文 图 表 新闻
- Abstracting/Indexing
- Journal Metrics
- Current Editorial Board
- Early Career Advisory Board
- Previous Editor-in-Chief
- Past Issues
- Current Issue
- Special Issues
- Early Access
- Online Submission
- Information for Authors
- Share facebook twitter google linkedin
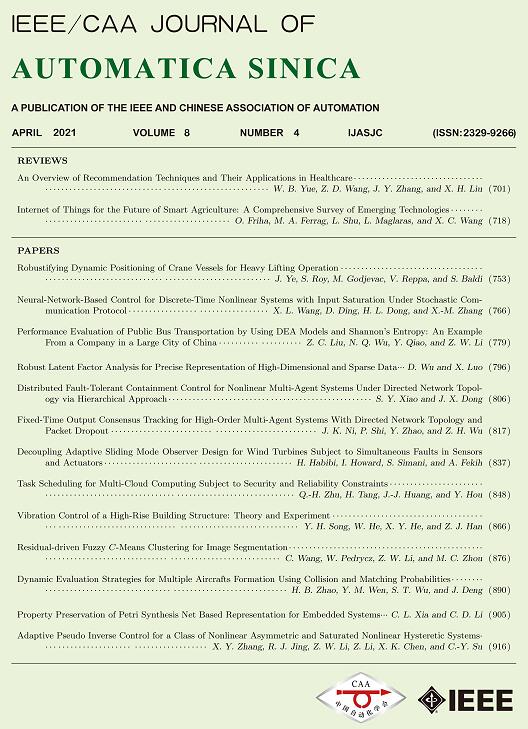
IEEE/CAA Journal of Automatica Sinica
- JCR Impact Factor: 15.3 , Top 1 (SCI Q1) CiteScore: 23.5 , Top 2% (Q1) Google Scholar h5-index: 77, TOP 5
Othmane Friha, Mohamed Amine Ferrag, Lei Shu, Leandros Maglaras, and Xiaochan Wang, "Internet of Things for the Future of Smart Agriculture: A Comprehensive Survey of Emerging Technologies," vol. 8, no. 4, pp. 718-752, Apr. 2021. doi: |
Othmane Friha, Mohamed Amine Ferrag, Lei Shu, Leandros Maglaras, and Xiaochan Wang, "Internet of Things for the Future of Smart Agriculture: A Comprehensive Survey of Emerging Technologies," vol. 8, no. 4, pp. 718-752, Apr. 2021. doi: |
Internet of Things for the Future of Smart Agriculture: A Comprehensive Survey of Emerging Technologies
Doi: 10.1109/jas.2021.1003925.
- Othmane Friha 1 , ,
- Mohamed Amine Ferrag 2 , ,
- Lei Shu 3, 4 , , ,
- Leandros Maglaras 5 , ,
- Xiaochan Wang 6 ,
Networks and Systems Laboratory, University of Badji Mokhtar-Annaba, Annaba 23000, Algeria
Department of Computer Science, Guelma University, Gulema 24000, Algeria
College of Engineering, Nanjing Agricultural University, Nanjing 210095, China
School of Engineering, University of Lincoln, Lincoln LN67TS, UK
School of Computer Science and Informatics, De Montfort University, Leicester LE1 9BH, UK
Department of Electrical Engineering, Nanjing Agricultural University, Nanjing 210095, China
Othmane Friha received the master degree in computer science from Badji Mokhtar-Annaba University, Algeria, in 2018. He is currently working toward the Ph.D. degree in the University of Badji Mokhtar-Annaba, Algeria. His current research interests include network and computer security, internet of things (IoT), and applied cryptography
Mohamed Amine Ferrag received the bachelor degree (June, 2008), master degree (June, 2010), Ph.D. degree (June, 2014), HDR degree (April, 2019) from Badji Mokhtar-Annaba University, Algeria, all in computer science. Since October 2014, he is a Senior Lecturer at the Department of Computer Science, Guelma University, Algeria. Since July 2019, he is a Visiting Senior Researcher, NAULincoln Joint Research Center of Intelligent Engineering, Nanjing Agricultural University. His research interests include wireless network security, network coding security, and applied cryptography. He is featured in Stanford University’s list of the world’s Top 2% Scientists for the year 2019. He has been conducting several research projects with international collaborations on these topics. He has published more than 60 papers in international journals and conferences in the above areas. Some of his research findings are published in top-cited journals, such as the IEEE Communications Surveys and Tutorials , IEEE Internet of Things Journal , IEEE Transactions on Engineering Management , IEEE Access , Journal of Information Security and Applications (Elsevier), Transactions on Emerging Telecommunications Technologies (Wiley), Telecommunication Systems (Springer), International Journal of Communication Systems (Wiley), Sustainable Cities and Society (Elsevier), Security and Communication Networks (Wiley), and Journal of Network and Computer Applications (Elsevier). He has participated in many international conferences worldwide, and has been granted short-term research visitor internships to many renowned universities including, De Montfort University, UK, and Istanbul Technical University, Turkey. He is currently serving on various editorial positions such as Editorial Board Member in Journals (Indexed SCI and Scopus) such as, IET Networks and International Journal of Internet Technology and Secured Transactions (Inderscience Publishers)
Lei Shu (M’07–SM’15) received the B.S. degree in computer science from South Central University for Nationalities in 2002, and the M.S. degree in computer engineering from Kyung Hee University, South Korea, in 2005, and the Ph.D. degree from the Digital Enterprise Research Institute, National University of Ireland, Ireland, in 2010. Until 2012, he was a Specially Assigned Researcher with the Department of Multimedia Engineering, Graduate School of Information Science and Technology, Osaka University, Japan. He is currently a Distinguished Professor with Nanjing Agricultural University and a Lincoln Professor with the University of Lincoln, U.K. He is also the Director of the NAU-Lincoln Joint Research Center of Intelligent Engineering. He has published over 400 papers in related conferences, journals, and books in the areas of sensor networks and internet of things (IoT). His current H-index is 54 and i10-index is 197 in Google Scholar Citation. His current research interests include wireless sensor networks and IoT. He has also served as a TPC Member for more than 150 conferences, such as ICDCS, DCOSS, MASS, ICC, GLOBECOM, ICCCN, WCNC, and ISCC. He was a Recipient of the 2014 Top Level Talents in Sailing Plan of Guangdong Province, China, the 2015 Outstanding Young Professor of Guangdong Province, and the GLOBECOM 2010, ICC 2013, ComManTel 2014, WICON 2016, SigTelCom 2017 Best Paper Awards, the 2017 and 2018 IEEE Systems Journal Best Paper Awards, the 2017 Journal of Network and Computer Applications Best Research Paper Award, and the Outstanding Associate Editor Award of 2017, and the 2018 IEEE ACCESS. He has also served over 50 various Co-Chair for international conferences/workshops, such as IWCMC, ICC, ISCC, ICNC, Chinacom, especially the Symposium Co-Chair for IWCMC 2012, ICC 2012, the General Co-Chair for Chinacom 2014, Qshine 2015, Collaboratecom 2017, DependSys 2018, and SCI 2019, the TPC Chair for InisCom 2015, NCCA 2015, WICON 2016, NCCA 2016, Chinacom 2017, InisCom 2017, WMNC 2017, and NCCA 2018
Leandros Maglaras (SM’15) received the B.Sc. degree from Aristotle University of Thessaloniki, Greece, in 1998, M.Sc. in industrial production and management from University of Thessaly in 2004, and M.Sc. and Ph.D. degrees in electrical & computer engineering from University of Volos in 2008 and 2014, respectively. He is the Head of the National Cyber Security Authority of Greece and a Visiting Lecturer in the School of Computer Science and Informatics at the De Montfort University, U.K. He serves on the Editorial Board of several International peer-reviewed journals such as IEEE Access , Wiley Journal on Security & Communication Networks , EAI Transactions on e-Learning and EAI Transactions on Industrial Networks and Intelligent Systems . He is an author of more than 80 papers in scientific magazines and conferences and is a Senior Member of IEEE. His research interests include wireless sensor networks and vehicular ad hoc networks
Xiaochan Wang is currently a Professor in the Department of Electrical Engineering at Nanjing Agricultural University. His main research fields include intelligent equipment for horticulture and intelligent measurement and control. He is an ASABE Member, and the Vice Director of CSAM (Chinese Society for Agricultural Machinery), and also the Senior Member of Chinese Society of Agricultural Engineering. He was awarded the Second Prize of Science and Technology Invention by the Ministry of Education (2016) and the Advanced Worker for Chinese Society of Agricultural Engineering (2012), and he also gotten the “Blue Project” in Jiangsu province young and middle-aged academic leaders (2010)
- Corresponding author: Lei Shu, e-mail: [email protected]
- Revised Date: 2020-11-25
- Accepted Date: 2020-12-30
- Agricultural internet of things (IoT) ,
- internet of things (IoT) ,
- smart agriculture ,
- smart farming ,
- sustainable agriculture
[1] | . Accessed on: Mar. 24, 2020. |
[2] | . Accessed on: Mar. 24, 2020. |
[3] | . Accessed on: Mar. 24, 2020. |
[4] | . Accessed on: Mar. 24, 2020. |
[5] | , vol. 142, pp. 283–297, Nov. 2017. doi: |
[6] | , vol. 9, no. 4, pp. 395–420, Jun. 2017. doi: |
[7] | , vol. 164, pp. 31–48, Dec. 2017. doi: |
[8] | , vol. 5, no. 5, pp. 3758–3773, Oct. 2018. doi: |
[9] | , vol. 157, pp. 218–231, Feb. 2019. doi: |
[10] | , vol. 19, no. 8, Article No. 1833, Apr. 2019. doi: |
[11] | , vol. 26, no. 6, pp. 56–63, Dec. 2019. doi: |
[12] | , vol. 108, no. 3, pp. 1785–1802, Oct. 2019. doi: |
[13] | , vol. 19, no. 17, Article No. 3976, Sept. 2019. |
[14] | , vol. 7, pp. 129551–129583, Aug. 2019. doi: |
[15] | , vol. 7, pp. 156237–156271, Oct. 2019. doi: |
[16] | , vol. 172, Article No. 107148, May 2020. doi: |
[17] | , vol. 8, pp. 32031–32053, Feb. 2020. doi: |
[18] | , 2020. DOI: |
[19] | , vol. 8, pp. 76300–76312, Apr. 2020. doi: |
[20] | : , vol. 48, no. 11, pp. 1939–1956, Nov. 2018. doi: |
[21] | , vol. 4, no. 1, pp. 6–18, Jan. 2017. doi: |
[22] | , vol. 6, no. 3, pp. 610–622, May 2019. doi: |
[23] | , vol. 7, no. 4, pp. 1026–1037, Jul. 2020. doi: |
[24] | , vol. 8, no. 2, pp. 273–302, Feb. 2021. doi: |
[25] | , vol. 153, pp. 69–80, May 2017. doi: |
[26] | , vol. 54, no. 15, pp. 2787–2805, Oct. 2010. doi: |
[27] | , vol. 29, no. 7, pp. 1645–1660, Sept. 2013. doi: |
[28] | , vol. 17, no. 4, pp. 2347–2376, Jun. 2015. doi: |
[29] | , vol. 4, no. 5, pp. 1125–1142, Oct. 2017. doi: |
[30] | , vol. 38, no. 4, pp. 393–422, Mar. 2002. doi: |
[31] | , vol. 5, no. 1, pp. 25–33, Feb. 2006. doi: |
[32] | , vol. 156, pp. 193–202, Jan. 2019. doi: |
[33] | , Kunming, China, 2016, pp. 1–6. |
[34] | , vol. 36, no. 2, pp. 263–270, Feb. 2014. doi: |
[35] | , vol. 105, pp. 20–33, Jul. 2014. doi: |
[36] | , vol. 156, pp. 467–474, Jan. 2019. doi: |
[37] | , vol. 4, no. 6, pp. 669–686, Nov. 2006. doi: |
[38] | , vol. 7, pp. 96879–96899, Jul. 2019. doi: |
[39] | , vol. 78, no. 20, pp. 29581–29605, 2019. doi: |
[40] | , Chengdu, China, 2019, pp. 1–2. |
[41] | . Accessed on: Mar. 24, 2020. |
[42] | . Accessed on: Mar. 24, 2020. |
[43] | . Accessed on: Mar. 24, 2020. |
[44] | AG,” [Online]. Available: . Accessed on: Mar. 24, 2020. |
[45] | . Accessed on: Mar. 24, 2020. |
[46] | . Accessed on: Mar. 24, 2020. |
[47] | . Accessed on: Mar. 24, 2020. |
[48] | . Accessed on: Mar. 24, 2020. |
[49] | , vol. 8, no. 3, Article No. 45, Aug. 2019. doi: |
[50] | , Quebec City, QC, Canada, 2018, pp. 1–5. |
[51] | . Singapore: Springer, 2019, pp. 521–528. |
[52] | . Accessed on: Mar. 24, 2020. |
[53] | , vol. 5, no. 2, pp. 234–8, Mar. 2017. doi: |
[54] | . Accessed on: Mar. 24, 2020. |
[55] | , vol. 155, pp. 473–486, Dec. 2018. doi: |
[56] | , Article No. 2017. |
[57] | . Accessed on: Mar. 24, 2020. |
[58] | |
[59] | , Tirunelveli, India, 2018, pp. 481–487. |
[60] | , vol. 114, no. 4, pp. 358–371, Apr. 2013. doi: |
[61] | , vol. 13, no. 6, pp. 693–712, Dec. 2012. doi: |
[62] | . Switzerland: Springer, 2020, pp. 39–50. |
[63] | . Switzerland: Springer, 2020, pp. 25–38. |
[64] | . Switzerland: Springer, 2020, pp. 51–69. |
[65] | , vol. 8, no. 10, Article No. e77151, Oct. 2013. doi: |
[66] | , Boston, MA, United States, 2017, pp. 515–529. |
[67] | , 2020. DOI: |
[68] | , vol. 7, no. 3, pp. 48–58, Sept. 2013. doi: |
[69] | , vol. 169, Article No. 105202, Feb. 2020. doi: |
[70] | . Cambridge, UK: Burleigh Dodds Science Publishing, 2019, pp. 1–25. |
[71] | , vol. 18, no. 4, pp. 574–614, Aug. 2017. doi: |
[72] | , vol. 169, Article No. 105216, Feb. 2020. doi: |
[73] | . Accessed on: Mar. 24, 2020. |
[74] | , vol. 68, pp. 112–129, Jul. 2017. doi: |
[75] | , Tampa, Florida, USA, 2014, pp. 201–210. |
[76] | . Accessed on: Mar. 24, 2020. |
[77] | , vol. 10, no. 3, pp. 4–7, Jul. 2011. doi: |
[78] | . Accessed on: Mar. 24, 2020. |
[79] | , vol. 35, no. 6, pp. 1201–1221, Apr. 2017. doi: |
[80] | , vol. 19, no. 3, Article No. 681, Feb. 2019. doi: |
[81] | , vol. 5, no. 1, pp. 1–7, Mar. 2019. doi: |
[82] | . Accessed on: Mar. 24, 2020. |
[83] | , vol. 3, no. 1, pp. 70–95, Feb. 2016. doi: |
[84] | , vol. 4, no. 1, pp. 1–20, Feb. 2017. |
[85] | , vol. 10, no. 3, Article No. 813, Jan. 2020. doi: |
[86] | , vol. 160, pp. 91–99, May 2019. doi: |
[87] | . Switzerland: Springer, 2016, pp. 343–353. |
[88] | , Sydney, Australia, 2013, pp. 41–44. |
[89] | , vol. 5, no. 2, pp. 141–145, Jun. 2019. doi: |
[90] | , vol. 20, no. 3, Article No. 596, Jan. 2020. doi: |
[91] | . Switzerland: Springer, 2015, pp. 137–152. |
[92] | . Accessed on: Mar. 24, 2020. |
[93] | . Accessed on: Mar. 24, 2020. |
[94] | . Accessed on: Mar. 24, 2020. |
[95] | , vol. 132, pp. 250–261, Oct. 2019. doi: |
[96] | . Accessed on: Mar. 24, 2020. |
[97] | , vol. 56, pp. 684–700, Mar. 2016. doi: |
[98] | , vol. 134, pp. 236–244, Feb. 2019. doi: |
[99] | , vol. 9, Article No. 100131, Mar. 2020. doi: |
[100] | , Helsinki, Finland, 2012, pp. 13–16. |
[101] | , vol. 177, pp. 4–17, Jan. 2019. doi: |
[102] | , vol. 71, pp. 124–136, Jan. 2017. doi: |
[103] | , vol. 24, pp. 12187–12196, Aug. 2020. doi: |
[104] | , vol. 7, pp. 116965–116974, Aug. 2019. |
[105] | , Fukuoka, Japan, 2019, pp. 889–892. |
[106] | , vol. 161, pp. 202–213, Jun. 2019. doi: |
[107] | , vol. 15, no. 12, pp. 6510–6521, Apr. 2019. doi: |
[108] | , vol. 5, no. 6, pp. 4589–4597, Dec. 2018. doi: |
[109] | , vol. 2, pp. 1–12, Jun. 2019. |
[110] | , vol. 33, no. 4, Article No. e4239, Mar. 2020. doi: |
[111] | , Chicago, Illinois, USA, 2014, pp. 1–6. |
[112] | . Accessed on: Mar. 24, 2020. |
[113] | , Hsinchu, Taiwan, China, 2014, pp. 671–676. |
[114] | . Accessed on: Mar. 24, 2020. |
[115] | . Accessed on: Mar. 24, 2020. |
[116] | , pp. 1–14, 2013. |
[117] | . Accessed on: Mar. 24, 2020. |
[118] | . Accessed on: Mar. 24, 2020. |
[119] | . Accessed on: Mar. 24, 2020. |
[120] | . Accessed on: Mar. 24, 2020. |
[121] | , vol. 147, pp. 70–90, Apr. 2018. doi: |
[122] | , vol. 7, pp. 45301–45312, Apr. 2019. doi: |
[123] | , vol. 99, pp. 500–507, Oct. 2019. doi: |
[124] | , vol. 19, no. 17, Article No. 3667, Aug. 2019. doi: |
[125] | , vol. 7, pp. 59069–59080, May 2019. doi: |
[126] | , vol. 3, no. 1, pp. 7–14, 2019. |
[127] | , vol. 103, no. 1, pp. 14–76, Jan. 2015. doi: |
[128] | , vol. 3, pp. 2542–2553, Dec. 2015. |
[129] | , vol. 16, no. 1, Article No. 108, Jan. 2016. doi: |
[130] | , vol. 16, no. 11, Article No. 1861, Nov. 2016. doi: |
[131] | , Alexandria, Egypt, 2018, pp. 1–5. |
[132] | , vol. 19, no. 5, Article No. 1044, Mar. 2019. doi: |
[133] | . Accessed on: Mar. 24, 2020. |
[134] | , Article No. 2011. |
[135] | . Accessed on: Mar. 24, 2020. |
[136] | . Trieste, Italy: Springer, 2017, pp. 137–147. |
[137] | . Accessed on: Mar. 24, 2020. |
[138] | , Article No. 2017. |
[139] | . Accessed on: Mar. 24, 2020. |
[140] | . Accessed on: Mar. 24, 2020. |
[141] | . Switzerland: Springer, 2020, pp. 341–387. |
[142] | , Halong Bay, Vietnam, 2015, pp. 487–490. |
[143] | , vol. 10, no. 11, Article No. 348, Nov. 2019. |
[144] | , vol. 162, pp. 882–894, Jul. 2019. |
[145] | , vol. 11, no. 9, Article No. 2658, May 2019. doi: |
[146] | , vol. 18, no. 19, pp. 7889–7898, Jul. 2018. doi: |
[147] | , vol. 20, no. 1, Article No. 21, Dec. 2019. doi: |
[148] | , vol. 19, no. 10, Article No. 2298, May 2019. doi: |
[149] | , vol. 99, pp. 278–294, Oct. 2019. doi: |
[150] | , vol. 98, Article No. 102047, Mar. 2020. doi: |
[151] | , vol. 164, Article No. 104836, Sept. 2019. doi: |
[152] | , vol. 3, no. 1, pp. 45–54, Mar. 2013. doi: |
[153] | , Graz, Austria, 2012, pp. 2640–2645. |
[154] | , Ancona, Italy, 2019, pp. 97–102. |
[155] | , vol. 9, no. 9, Article No. 1831, May 2019. doi: |
[156] | , vol. 5, no. 6, pp. 838–842, Jun. 2016. |
[157] | , vol. 20, no. 3, Article No. 817, Feb. 2020. doi: |
[158] | , vol. 9, no. 5, Article No. 216, Apr. 2019. doi: |
[159] | , Atlanta, GA, USA, 2019, pp. 937–944. |
[160] | , vol. 5, no. 6, pp. 4890–4899, Dec. 2018. |
[161] | , vol. 19, no. 7, Article No. 1598, Apr. 2019. doi: |
[162] | , vol. 19, no. 6, Article No. 1366, Mar. 2019. doi: |
[163] | , vol. 7, pp. 37050–37058, Mar. 2019. doi: |
[164] | , vol. 4, no. 1, pp. 10–15, Mar. 2017. doi: |
[165] | , vol. 19, no. 2, Article No. 276, Jan. 2019. doi: |
[166] | , vol. 162, pp. 979–990, Jul. 2019. doi: |
[167] | , vol. 19, no. 10, Article No. 2318, May 2019. doi: |
[168] | , vol. 15, no. 1, pp. 7–11, Jan. 2019. doi: |
[169] | , vol. 167, Article No. 107039, Feb. 2020. doi: |
[170] | , vol. 155, pp. 41–49, Dec. 2018. doi: |
[171] | , Coimbatore, India, 2018, pp. 478–483. |
[172] | , vol. 31, no. 1, pp. 277–292, Jan. 2019. |
[173] | , vol. 20, no. 1, Article No. 190, Dec. 2019. doi: |
[174] | , vol. 82, pp. 268–273, May 2018. doi: |
[175] | : , vol. 28, Article No. 100300, Dec. 2020. |
[176] | , vol. 150, pp. 26–32, Jul. 2018. doi: |
[177] | , Udupi, India, 2017, pp. 1201–1205. |
[178] | , Toronto, ON, Canada, 2020, pp. 1266–1267. |
[179] | , vol. 60, no. 4, pp. 393–404, Apr. 2014. doi: |
[180] | , vol. 3, no. 4, pp. 2870–2877, Jun. 2018. doi: |
[181] | , Milan, Italy, 2017, pp. 525–527. |
[182] | , 2015, pp. 66–70. |
[183] | , vol. 156, pp. 96–104, Jan. 2019. doi: |
[184] | , vol. 7, pp. 127098–127116, Aug. 2019. doi: |
[185] | , vol. 18, no. 11, Article No. 4051, Nov. 2018. doi: |
[186] | , vol. 15, no. 1, pp. 610–617, Jan. 2015. doi: |
[187] | , vol. 124, pp. 211–219, Jun. 2016. doi: |
[188] | , vol. 166, Article No. 105028, Nov. 2019. doi: |
[189] | , Samsun, Turkey, 2019, pp. 58–62. |
[190] | , vol. 89, Article No. 106128, Apr. 2020. doi: |
[191] | , vol. 11, no. 4, pp. 3640–3651, Mar. 2011. doi: |
[192] | , Tuscany, Italy, 2018, pp. 1–5. |
[193] | , vol. 161, pp. 225–232, Jun. 2019. doi: |
[194] | , vol. 16, no. 8, Article No. 1222, 2016. |
[195] | , vol. 21, no. 1, pp. 160–177, Feb. 2020. doi: |
[196] | , Coimbatore, India, 2017, pp. 1–10. |
[197] | , Coimbatore, India, 2016, pp. 1–5. |
[198] | , vol. 168, Article No. 105108, Jan. 2020. |
[199] | , vol. 168, Article No. 107036, Feb. 2020. doi: |
[200] | , vol. 21, no. 1, pp. 1–17, Feb. 2020. doi: |
[201] | , vol. 96, pp. 148–162, 2013. doi: |
[202] | , vol. 236, pp. 1–13, Apr. 2019. doi: |
[203] | , vol. 22, no. 4, pp. 8919–8927, Jul. 2019. |
[204] | , Tuscany, Italy, 2018, pp. 1–4. |
[205] | , vol. 134, pp. 393–398, Jan. 2018. doi: |
[206] | , Washington DC, USA, 2019, pp. 173–179. |
[207] | , vol. 170, Article No. 105251, Mar. 2020. doi: |
[208] | , Singapore, 2018, pp. 9–18. |
[209] | , vol. 118, no. 9, pp. 21–32, Jan. 2018. |
[210] | , vol. 119, no. 14, pp. 1193–1196, Jan. 2018. |
[211] | , vol. 73, pp. 223–229, Mar. 2017. doi: |
[212] | . Accessed on: Mar. 24, 2020. |
[213] | , Shanghai, China, 2019, pp. 1–6. |
[214] | , vol. 220, pp. 545–561, Jun. 2018. doi: |
[215] | , vol. 48, no. 12, pp. 3371–3380, Aug. 2018. |
[216] | , vol. 22, no. 5, pp. 65–67, Oct. 2007. doi: |
[217] | , vol. 18, no. 6, Article No. 1731, May 2018. doi: |
[218] | , vol. 6, pp. 67528–67535, Oct. 2018. doi: |
[219] | , 2019. DOI: |
[220] | , vol. 18, no. 5, Article No. 1333, Apr. 2018. doi: |
[221] | . Singapore: Springer, 2018, pp. 337–345. |
[222] | , vol. 13, no. 1, pp. 1–10, Jan. 2020. |
[223] | , vol. 246, no. 1–3, pp. 147–156, Sept. 2009. doi: |
[224] | , vol. 90, Article No. 102067, Aug. 2020. doi: |
[225] | , Ancona, Italy, 2019, pp. 47–52. |
[226] | ),” , vol. 15, no. 1, Article No. 131, Nov. 2019. doi: |
[227] | , Chuncheon-si Gangwon-do, Korea (South), 2018, pp. 749–752. |
[228] | , Paris, France, 2017, pp. 1–6. |
[229] | , vol. 5, no. 2, pp. 177–181, Feb. 2017. |
[230] | |
[231] | . Shanghai, China: Springer, 2016, pp. 105–121. |
[232] | , vol. 88, Article No. 101653, Jan. 2020. doi: |
[233] | , vol. 24, pp. 2671–2691, Feb. 2020. doi: |
[234] | , Amsterdam, Netherlands, 2018. |
[235] | , vol. 86, pp. 641–649, Sept. 2018. doi: |
[236] | , Kuala Lumpur, Malaysia, 2018, pp. 51–58. |
[237] | |
[238] | , Dalian, China, 2017, pp. 1–6. |
[239] | , vol. 9, no. 11, Article No. 382132, Sept. 2013. doi: |
[240] | , vol. 23, pp. 351–366, Dec. 2011. doi: |
[241] | , vol. 90, no. 2, pp. 115–125, Feb. 2005. doi: |
[242] | , vol. 6, no. 2, pp. 2188–2204, Apr. 2019. doi: |
[243] | . Switzerland: Springer, 2019, pp. 1029–1038. |
[244] | , vol. 67, no. 4, pp. 1285–1297, Nov. 2020. doi: |
[245] | , vol. 19, no. 14, Article No. 3119, Jul. 2019. doi: |
[246] | , vol. 8, no. 3, Article No. 58, Aug. 2019. doi: |
[247] | , vol. 36, no. 10, pp. 2725–2732, Oct. 2011. doi: |
[248] | , vol. 54, pp. 299–308, Feb. 2016. doi: |
[249] | , vol. 39, no. 4, Article No. 35, Jun. 2019. doi: |
[250] | , vol. 73, pp. 1–9, Jun. 2017. doi: |
[251] | , United States, 2019, pp. 236–237. |
[252] | , vol. 94, Article No. 101966, Nov. 2019. doi: |
[253] | , vol. 11, Article No. 14, Mar. 2015. doi: |
[254] | , vol. 169, Article No. 105156, Feb. 2020. doi: |
[255] | , vol. 27, no. 6, pp. 140–145, Dec. 2020. |
[256] | . Accessed on: Sept. 17, 2020. |
[257] | , vol. 7, no. 11, pp. 11223–11237, Nov. 2020. doi: |
[258] | , vol. 54, Article No. 102556, Oct. 2020. |
Proportional views
通讯作者: 陈斌, [email protected]
沈阳化工大学材料科学与工程学院 沈阳 110142
Figures( 12 ) / Tables( 9 )
Article Metrics
- PDF Downloads( 3002 )
- Abstract views( 15635 )
- HTML views( 1512 )
- We review the emerging technologies used by the Internet of Things for the future of smart agriculture.
- We provide a classification of IoT applications for smart agriculture into seven categories, including, smart monitoring, smart water management, agrochemicals applications, disease management, smart harvesting, supply chain management, and smart agricultural practices.
- We provide a taxonomy and a side-by-side comparison of the state-of-the-art methods toward supply chain management based on the blockchain technology for agricultural IoTs.
- We highlight open research challenges and discuss possible future research directions for agricultural IoTs.
- Copyright © 2022 IEEE/CAA Journal of Automatica Sinica
- 京ICP备14019135号-24
- E-mail: [email protected] Tel: +86-10-82544459, 10-82544746
- Address: 95 Zhongguancun East Road, Handian District, Beijing 100190, China
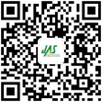
Export File

- Figure 1. The four agricultural revolutions
- Figure 2. Survey structure
- Figure 3. IoT-connected smart agriculture sensors enable the IoT
- Figure 4. The architecture of a typical IoT sensor node
- Figure 5. Fog computing-based agricultural IoT
- Figure 6. SDN/NFV architecture for smart agriculture
- Figure 7. Classification of IoT applications for smart agriculture
- Figure 8. Greenhouse system [ 101 ]
- Figure 9. Aerial-ground robotics system [ 67 ]
- Figure 10. Photovoltaic agri-IoT schematic diagram [ 251 ]
- Figure 11. Smart dairy farming system [ 254 ]
- Figure 12. IoT-based solar insecticidal lamp [ 256 ], [ 257 ]
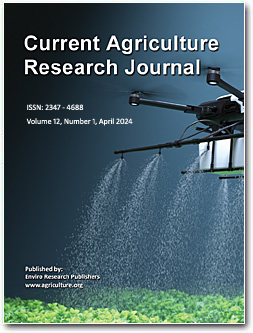
Print ISSN: 2347-4688 Online ISSN: 2321-9971
Frequency: Triannual (April, August, December)
Average Publish Time: 98 Days
Chief Editor: Dr. Surendra Singh Bargali
CARJ is a RoMEO green Journal
Journal Information
Current Agriculture Research Journal is an open access, international, scholarly peer-reviewed research journal which publishes original research after double-blind peer review. Published triannually in April, August and December with an aim to foster high-quality research in the field of agricultural sciences.
Recent Articles
Isolation and molecular characterization of plant growth promoting rhizobacteria from groundnut ( arachis hypogaea l.) rhizosphere, does agricultural credit mitigate the effect of climate change on sugarcane production evidence from uttar pradesh, india, classification of tomato leaf disease using a custom convolutional neural network, most read articles, direct seeded rice: prospects, problems/constraints….
Views: (50,368)
Jagmohan Kaur*, Avtar Singh
Impact of National Rural Employment Guarantee Scheme…
Views: (26,059)
K. Kareemulla 1* , P. Ramasundaram 2 , Shalander Kumar 3 , C. A. Rama Rao 4
Potential Use of Azotobacter Chroococcum in Crop…
Views: (20,860)
Sartaj A. Wani*, Subhash Chand, Tahir Ali
Effect of Air Pollution on Chlorophyll Content of Leaves
Views: (18,328)
Sumitra Giri 1* , Deepali Shrivastava 2 , Ketki Deshmukh 2 , Pallavi Dubey 2
Most Downloaded Articles
Downloads: (18280 )
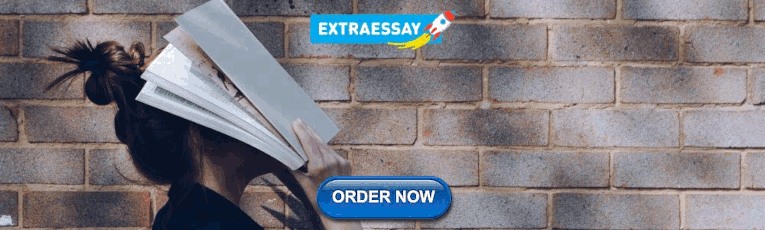
Potential Use of Azotobacter Chroococcum in Crop Production: An Overview
Downloads: (12507 )
Effect of Organic and Inorganic Fertilizers on the Quantitative and Qualitative Parameters of Rice (Oriza sativa L.)
Downloads: (12330 )
Direct Seeded Rice: Prospects, Problems/ Constraints and Researchable Issues in India
Downloads: (10795 )
Organic farming: as a Climate Change Adaptation and Mitigation Strategy
Downloads: (9389 )
Comparative effect of organics and biofertilizers on growth and yield of maize (Zea mays. L)
Downloads: (9138 )
Read Before Submitting
We strongly recommend all authors to follow the instructions while preparing and submitting the manuscript.
Please visit the Instructions page…..
Editorial and Review Process
Once the articles is submitted to Current Agriculture Research Journal. It goes through a initial review to check the scope, quality of content and adherence to journals format. Based on this report from Editorial Board Member its sent for further review.
Read more ……
Peer Review
Current Agriculture Research Journal uses double-blind review, in which the identity of both the reviewer and the author are unknown to each other. This ensures impartiality and helps every paper get an unbiased review.
Read more….
Publication ethics and malpractice statement
Current Agriculture Research Journal’s Publication Ethics and Publication Malpractice Statement is based, in large part, on the guidelines and standards developed by the Committee on Publication Ethics (COPE). The relevant duties and expectations of authors, reviewers, and editors of the journal are set out below.
Read more……
Plagiarism Policy
By submitting articles to Current Agriculture Research Journal, the author attests the following:
- None of the parts of the manuscript are plagiarized from other sources
- Proper reference is provided for all contents extracted from other sources
- Firm action will be taken against cases of plagiarism
All Policies
Academia.edu no longer supports Internet Explorer.
To browse Academia.edu and the wider internet faster and more securely, please take a few seconds to upgrade your browser .
- We're Hiring!
- Help Center
Agriculture
- Most Cited Papers
- Most Downloaded Papers
- Newest Papers
- Last »
- Agronomy Follow Following
- Sustainable agriculture Follow Following
- Agricultural Economics Follow Following
- Soil Science Follow Following
- Horticulture Follow Following
- Education Follow Following
- Plant breeding and genetics Follow Following
- Biology Follow Following
- Climate Change Follow Following
- Agricultural extension Follow Following
Enter the email address you signed up with and we'll email you a reset link.
- Academia.edu Journals
- We're Hiring!
- Help Center
- Find new research papers in:
- Health Sciences
- Earth Sciences
- Cognitive Science
- Mathematics
- Computer Science
- Academia ©2024
Thank you for visiting nature.com. You are using a browser version with limited support for CSS. To obtain the best experience, we recommend you use a more up to date browser (or turn off compatibility mode in Internet Explorer). In the meantime, to ensure continued support, we are displaying the site without styles and JavaScript.
- View all journals
- Explore content
- About the journal
- Publish with us
- Sign up for alerts
Waivers available for articles submitted in 2024, npj Sustainable Agriculture has waivers available to offer that can be allocated upon acceptance on an ad-hoc basis. For additional information contact the Managing Editor .
- Perspective
- Open access
- Published: 12 September 2024
Overview and recommendations for research on plants and microbes in regolith-based agriculture
- Laura E. Fackrell 1 ,
- Samson Humphrey 2 ,
- Rafael Loureiro 3 ,
- Andrew G. Palmer 4 , 5 &
- Jared Long-Fox 6
npj Sustainable Agriculture volume 2 , Article number: 15 ( 2024 ) Cite this article
Metrics details
- Microbial communities
- Element cycles
- Environmental microbiology
- Plant development
- Plant physiology
- Plant stress responses
The domestication of agriculture is widely recognized as one of the most crucial technological adaptations for the transition of humanity from hunter-and-gatherer groups into early city-states and ultimately, complex civilizations. As humankind sets forth to permanently establish itself on the Moon and use it as a testing ground to colonize other worlds, like Mars, agriculture will again play a pivotal role. In this case, the development of sustainable crop production systems capable of succeeding in these harsh environments becomes vital to the success of our star-faring journey. Over decades, studies varying in species and approaches have been conducted in microgravity, testing the limits of plants and various growth systems, to better understand how Earth-based agriculture could be translated into environmental conditions and therefore evolutionary pressures beyond what life on our planet has known. While we have passed several significant milestones, we are still far from the goal of a sustainable agricultural system beyond our planet Regolith-based agriculture (RBA) should be a component of sustainable agriculture solutions beyond Earth, one which can also provide insight into plant growth in poor soils across our own world. However, RBA studies are in their infancy and, like any other new field, need an established set of parameters to be followed by the RBA community so the generated data can be standardized and validated. Here, we provide an extensive multi-disciplinary review of the state of RBA, outline important knowledge gaps, and propose a set of standardized methods and benchmarks for regolith simulant development and selection as well as plant, microbe, and plant-microbe interaction studies conducted in lunar and Martian regolith. Our goal is to spur dialog within the RBA community on proper regolith simulant selection, experimental design, and reporting. Our methods are divided into complexity tiers, providing a clear path for even the simplest experiments to contribute to the bulk of the knowledge that will shape the future of RBA science and see it mature as an integrated part of sustainable off-world agriculture.
Similar content being viewed by others

Plants grown in Apollo lunar regolith present stress-associated transcriptomes that inform prospects for lunar exploration
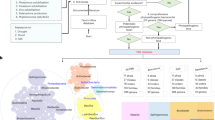
Fossil-fuel-dependent scenarios could lead to a significant decline of global plant-beneficial bacteria abundance in soils by 2100
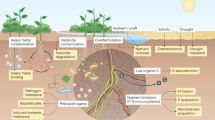
Soil microbiome engineering for sustainability in a changing environment
Introduction.
Crewed space missions depend upon the control and integration of a tremendous number of systems and features simultaneously, with the ultimate goal of establishing a sustainable artificial ecosystem despite being surrounded by an environment ill-suited for human life. Among the numerous mission-critical components which need to be considered in the development of this artificial ecosystem, consistent access to a safe, sustainable, and sufficiently nutritious food supply is one of the highest priorities 1 . Absolute dependence on resupply missions from Earth is expensive, risky, and would continue to deplete resources from our own planet, all factors which would ultimately jeopardize any established colony. With estimates that continuous off-world settlements will be a reality within 50 years, temporary habitats will be established far sooner, and a reliable supply of nutrition will be crucial to support these missions.
The development of bioregenerative food systems (BFS) have been proposed as the most cost-effective approach for reducing the frequency of resupply missions and are crucial to enabling sustainable planetary colonization 2 , 3 , 4 , 5 , 6 , 7 , 8 . Current food production systems can supplement present day missions within low Earth orbit (LEO) but are neither sustainable nor up to the task of supporting the future demands of deep space exploration 3 , 6 . Existing BFS such as Veggie growth chamber and the APH (Advanced Plant Habitat) employ hydroponic strategies that have proven effective in the microgravity environment of the International Space Station (ISS) 9 . However, different solutions will be required to support long-term surface settlements off-world.
Unlike the ISS, lunar and planetary settlement efforts can utilize additional in situ (i.e., on-site) resources (ISR) available including water, atmospheric components (e.g., O 2 and/or CO 2 ), and most notably, the regolith that comprises their surfaces. In situ resource utilization (ISRU) of regolith as a substrate for plant growth has been widely regarded as potentially valuable for food production as regolith is a physical substrate and a potential source of plant nutrients (Iron, Potassium, Magnesium, etc.). This substrate does not need to be shipped from Earth reducing total costs and labor. Moreover, regolith-based agriculture (RBA) leverages centuries of agricultural knowledge and the evolutionarily adapted relationship between plants, microorganisms, and soil to support plant growth and food production. RBA can incorporate sustainable agricultural approaches that we use on Earth to minimize fertilizer consumption, avoid the spread of pathogens, promote nutrient cycling, and support interactions with beneficial microorganisms 10 . Furthermore, RBA can operate in parallel with other agricultural approaches (e.g., hydroponics, soilless substrates, etc.), enabling us to design a more diverse, secure, and sustainable approach for off-world food production, instead of a one-size-fits-all system.
However, despite the potential of RBA to serve as a major component in lunar and planetary-based food systems, efforts to develop and evaluate the viability of this approach have faced a number of challenges. Chief among these is the fact that little or no supply of actual lunar or Martian regolith is available for this research, forcing a reliance upon terrestrially or anthropogenically sourced proxies (termed regolith simulant ’). This alone has created considerable variability in conclusions made about the viability of RBA. Additionally, studies vary widely in approach, growing conditions, plant selection, metrics for success, etc. This is not unexpected as emerging research areas, like all new frontiers, are fueled by innovation and marked by rapid, often unrestrained growth. Therefore, as the number of researchers and projects associated with any field continues to grow, it becomes necessary to establish specific conventions and best practices.
We stand at this point in the regolith community, where a critical mass of researchers has developed, and our capacity to successfully evaluate the true in situ resource potential of the lunar and Martian surface depends on establishing common protocols and terminology to effectively communicate and more objectively evaluate our work collectively. Herein we provide an extensive review of Martian and lunar regolith simulants applied to agricultural research in support of future off-world colonies. Drawing from this substantial, yet disparate, body of work we propose a ranked set of “best practices” for RBA research.
Our goals are to: (i) to inform researchers at multiple institutional levels and from various fields of expertise, (ii) to improve efforts to compare and communicate results of RBA research including increased collaboration and consultation, (iii) identify critical knowledge gaps in RBA research, (iv) and ultimately provide a more accurate assessment of the viability and technology readiness level (TRL) of RBA as a component of food-systems and bioregenerative-life support for off-world colonization. The writing of this document has been a collaborative exercise, one which has inspired new projects between the authors. In this spirit, we hope the readers will be similarly inspired to seek out collaborative approaches to best leverage expertise and produce the deliverables necessary to support sustainable food production on lunar or Martian colonies.
Properties and challenges of regolith as a growth substrate
The Moon and Mars have always been a subject of human fascination providing a wealth of data obtained through remote sensing and robot missions 11 , 12 and returned or soon to be returned samples for terrestrial-based analysis 13 , 14 . The regoliths of both surfaces could potentially provide multiple agriculturally relevant resources. For example, Mars provides access to carbonate and acidic sulfate materials potentially useful in the regulation of pH of nutrient fluids applied to agricultural systems. The Moon similarly provides potentially useful substrates and additionally serves as a testing ground for the first suite of off-world agricultural systems used to develop similar systems for Mars.
However, lunar and Martian regolith are also very different: The lunar regolith is created through space weathering (impact processes, radiation, no atmosphere) over billions of years in a reducing, vacuum environment 15 , 16 . while Martian regolith results from various impact, eolian, aqueous and other processes acting over billions of years on a globally basaltic crust 12 , 17 . This has produced two very different regolith substrates that present unique challenges for agriculture.
Analysis of the overall chemical makeup represented as elemental oxides alone is insufficient to fully describe or predict regolith viability (i.e., fertility). In evaluating the viability of soil, soilless media, or regolith as a growing medium, a variety of characteristics are considered including nutrient content and mobility, pH, salinity, contaminants/toxins, organic content, texture, density, porosity, strength (shear and compressive), etc. Thus, to understand the potential of Martian or lunar regolith as a growth medium (and appropriately mimic it) a variety of characteristics that more fully describe (or mimic) this should be applied. Here we provide an overall summary of lunar and Martian regolith-fertility as currently understood. This includes a summary of the overall chemistry, mineralogy, and physical characteristics (texture, soil strength, etc.), a comparison of overall elemental abundance to plant requirements (Table 1 ), and how well constrained/understood these aspects are currently and what relevance these hold to the application of regolith as a growing medium.
Defining regolith
To address the challenges facing RBA for successful implementation, it is essential to accurately simulate relevant characteristics of the target regolith. Indeed, even the term “regolith“ can introduce confusion into the discussion depending on the definition applied and the common practice of interchangeably using terms such as “regolith“ and “soil”, or even referring to non-regolith materials (e.g., bed-rock deposits) as regolith. Regolith is defined as the “unconsolidated material covering bedrock and can include dust, broken up rocks, soil and other related materials” 18 , 19 , 20 . Thus, regolith incorporates a variety of unconsolidated materials on the surface separating it from consolidated units that would be termed bedrock. However, weathering produces a gradation from un-weathered bedrock to fully developed soils and it can be difficult to pinpoint the exact point at which the material is unconsolidated enough to be considered regolith and no longer bedrock.
As regolith encompasses a broad range of materials, it is useful to distinguish different fractions of the regolith using terms such as soil and dust, depending on the particular context. For example, when examining the pedological history of the regolith, distinguishing among dust, soil, etc. is practical as different processes (i.e., eolian-wind and fluvial-river) affect these portions differently. Such variations provide important distinctions in interpreting the resulting history recorded in the materials. Similarly, from an engineering perspective “soil” and “dust” represent behaviorally and physically separable portions of the regolith 21 , 22 . For these and other examples, it is useful and appropriate to refer to a particular portion of the regolith as soil.
However, this “soil-like” portion of lunar and Martian regolith is distinctly “unsoil-like” from an agricultural perspective, in particular in its lack of well-developed soil horizons, absence of organic matter, and other characteristics more typical of common “agricultural soils”, making the term “soil” less useful in an agricultural context. Soil is a complex matrix, and details of its components can be found in Table S1 . Due to the distinct agriculturally relevant differences between agricultural earth soils and extra-terrestrial “soils”, it is useful to use the term regolith when speaking of extra-terrestrial “soils” as an agricultural material. Thus, this work will use the term regolith to refer to the unconsolidated “soil-like” materials or in other words material one could readily scoop off the surface, not necessarily including or excluding dust (SI Section Table S1 ). The term bedrock or other appropriate terms are used for non-regolith materials, and the term surface materials refers to any surface/near surface material: both regolith and non-regolith.
Lunar regolith characteristics
Lunar regolith composition and chemistry.
The lunar surface can be divided into two main, distinct geologic provinces: the felsic lunar highlands and the mafic lunar mare 23 . The lunar highlands are dominantly anorthositic 11 , 24 with up to 98% anorthite content 25 . The lunar highlands regolith (e.g., Apollo 16 and Luna 20) is dominantly felsic, composed of calcium-rich plagioclase with little pyroxene and other mafic, magnesium- and iron-rich mineral content 13 , 15 , 24 . It is assumed that the mixing of basaltic materials with the felsic highlands material is due to impact gardening and ejecta being distributed around the surface of the Moon due to the low gravity and high energy nature of impacts. The lunar mare is thought to have formed from basaltic volcanic materials rising from the lunar mantle after the initial formation of the Moon 26 . The mineralogy of the lunar mare regolith (e.g., Apollo 11, 12 and Luna 16, 24) is nominally basaltic, containing mostly ferromagnesian minerals with clinopyroxenes, olivine, ilmenite, and lesser amounts of calcium-rich plagioclase relative to the highlands 24 , 27 , 28 . Though the composition of regolith in the lunar mare is generally consistent from site to site, the youngest regions have unusually high concentrations of potassium (K), rare earth elements (REE), and phosphorus (P); these regions are called the Procellarum KREEP Terrane 29 . Apollo 15 and 17 landings were made in areas marginal to highlands and mare and have compositions intermediate to both with rock fragments derived from both provinces 13 , 15 . Phosphorus is found in relatively minor, but consistent, amounts in the lunar regolith 30 and particularly in the KREEP terranes in minerals such as schreibersite 31 . Potassium is an incompatible trace element in the lunar regolith 30 , and the potassium content of the lunar regolith is mostly found in the KREEP regolith. The lunar regolith has a negligible nitrogen content, and the nitrogen that is present was mostly deposited by solar winds 30 . This makes lunar KREEP materials potential sources of nutrients for plant growth (Table 1 ).
The lunar surface is significantly altered by space weathering, creating nanophase iron particles and glassy agglutinates in its regolith due to the Moon’s lack of atmosphere and vacuum conditions 15 , 32 ; Most lunar agglutinates are enriched in iron, magnesium, titanium, manganese, chromium, and scandium and other lithophile elements such as potassium, lanthanum, and cerium, but are depleted in elements compatible with plagioclase relative to bulk regolith regardless of formation in mare or highlands regions 33 , but the lunar mare is noted to have a higher agglutinate content than the highlands. The abundance of agglutinates and the ratio of nanophase iron concentration to the total iron content of lunar regolith is the maturity index of the regolith 32 , 34 in the lunar regolith and is a function of the duration of surface exposure 35 , 36 . The enrichment in ferromagnesian and lithophile elements is more pronounced in immature regolith and as regolith maturity increases, an increased proportion of plagioclase is observed in agglutinitic materials 33 , 34 . This maturity affects the regolith’s composition, especially in finer fractions, where the resemblance to agglutinate glasses increases and presence of nanophase iron increases, highlighting the dynamic nature of the lunar surface composition.
The composition described provides a medium rich in calcium, aluminum, magnesium, iron, and titanium but with much smaller amounts of nitrogen, phosphorus, potassium and sodium 30 , 37 . The high calcium, aluminum, magnesium, iron, and titanium content of the lunar regolith is potentially useful for plant growth, as these elements play roles in nutrient supply, growth, growth stimulation, and photosynthesis. However, these elements are not guaranteed to be bioavailable to plants and may require additional effort to mobilize these elements for use. The lack of nitrogen, phosphorus, and potassium also represents a significant challenge as these are major elements required for plant growth. This means that the lunar regolith will need to be processed and amended to increase mobility of certain nutrients and incorporate missing nutrients to enable optimal plant growth (see Table 1 ). What exactly these processing needs entail and what nutrients this will affect them is an area of needed research.
Lunar regolith physical properties and implications for plant growth
The particle size distribution and specific surface area of a medium for plant growth influence the permeability and fluid retention properties of the material 38 , and thus directly impact plant growth. Particle size analysis of lunar regolith shows that the mean grain size of returned samples ranges from 40 µm to 800 µm, with a lunar global average between 60 and 80 µm 15 , 39 . The wide range of particle sizes in lunar regolith allows for smaller particles to nest between larger ones, causing more complete filling of void space and decreasing permeability. Since the lunar regolith and appropriate simulants are composed of igneous mineral grains, water retention is controlled by the bulk porosity and the pore content of individual grains (measured by specific surface area), which is low compared to soils and other carbon-rich materials 38 . These properties of the lunar regolith imply that there are particle sizes and particle size distributions that give the required porosity and permeability for plant growth in lunar regolith that must be carefully balanced in regolith-soil mixes to allow for the most efficient delivery of water and nutrients.
In order to seek out local resources (e.g., water and other nutrients), plant roots grow and push their way through the growing medium. The compressive and shear strength of a plant growing medium determines the relative ease of penetration; a stronger material is harder for roots to push through. The shear and compressive strength of a material increases with increasing relative density and decreasing porosity. The density and porosity of lunar regolith have been shown to vary from site to site on the Moon and increase with depth within a single site, implying that mechanical strength varies in the same manner 40 . From a low relative density, the lunar regolith compresses significantly due to the high initial porosity and the crushing of weak, glassy grains during compression, but a minor change in initial relative density during compression leads to differing compressive strength estimates, especially in the relatively less dense upper 30 meters of regolith 40 . These results have strong implications for acceptable densities and amounts of compression of lunar regolith mixes for plant growth in terms of both root penetration and permeability for water delivery. Lunar regolith has high cohesive and frictional strength 40 , 41 making penetration (whether instrumentation or plant roots) difficult, and increasing the required amount of energy a plant must expend to reach nutrients as the root system expands.
Martian regolith characteristics
Martian regolith composition and chemistry.
The Martian regolith has a generally basaltic mineralogical profile that thus far has been globally homogenous (though there are locally altered soils that deviate from this) 12 , 42 , 43 , 44 , 45 , 46 , 47 The mineralogical profile of the regolith includes plagioclase, pyroxenes and olivine as major phases and magnetite, ilmenite, quartz, potassium feldspars and various salts as minor phases 48 , 49 , 50 , 51 . Two specific sites with samples taken by Curiosity from which this data is available include Rocknest and Gobabeb, both basaltic sands sampled within Gale Crater 49 .
In addition to identified mineral phases, all of Mars surface materials so far examined using XRD have included what has been termed an amorphous fraction consisting of crystallographically disordered materials that make-up ~20 to 50% of the sample 52 , 53 . The exact compositional make-up of this amorphous fraction is poorly constrained and variable among different surface materials, but a general chemical make-up of a particular sample can be understood with the application of multiple instruments and techniques 54 . It is thought to include materials like basaltic glass, nanophase iron oxides (e.g., ferrihydrite and maghemite), proto-phyllosilicates, carbonate and sulfate and other highly disordered materials (e.g., allophane or hesingerite) 48 , 54 , 55 , 56 , 57 , 58 , 59 . Such disordered phases are often the most reactive in Earth soils and can greatly influence overall soil fertility 60 . Thus, although poorly constrained, to the greatest extent possible it is important to appropriately represent these phases in regolith-based agricultural experiments 19 , 61 , 62 .
The compositional profile from both crystalline and non-crystalline phases provides an abundance of P, Mg, Ca, Fe, Na, Cl and Si moderate amounts of K and only trace amounts of N, relative to plant’s needs. Of course, this does not take into account the bioavailability of these nutrients but many of the minerals these are found in tend to be more bioavailable forms so at least some portion is expected to be plant extractable. Another important caveat is that some micronutrients (in particular Fe, Na, Cl, or Mg) may be present at phytotoxic levels. However, it must be noted that the amount of any micronutrient required to cause phytotoxicity varies depending on the plant species, substrate pH, and other synergistic or antagonistic nutrients present in the substrate. Additionally, though the overall chemistry and mineral profile (outside of the amorphous fraction) is fairly well understood for present day Mars soil 42 , 61 , 63 , other characteristics are less well-constrained or can only be inferred from indirect analysis based on available data. This includes features such as pH, salinity (including the implications of perchlorates/chlorates), nutrient content and mobility. All the major macronutrients and most required by plants have been detected in Mars regolith or meteorites 63 , 64 and the mobility and bioavailability of these can be inferred to some extent but is generally not well understood.
The Mars Phoenix Lander mission provides the only direct measurements of pH and salinity to present-day Mars soil providing one location and three samples of direct measurement 65 , 66 , 67 , though pH and other geochemical aspects can be somewhat inferred from other mission such as the Viking Landers. More recent analysis of soils at Gale crater provide enough information that we can indirectly infer that the overall characteristics are likely to carry over, though there is variation in the ratio of salts found 68 . The pH of Mars soil measured by Phoenix Lander averaged ~7.7 ± 0.3 indicating an alkaline soil 67 , 69 . It should be noted that this pH is within the acceptable range for most crop plants (~5 to ~8), and that at this pH most nutrients are bioavailable for plant uptake. Data from multiple missions (including Phoenix and Curiosity) provide an estimate of about 1–3 wt% salts in the regolith dominated by various Mg, Ca, and Na perchlorate, sulfate and carbonates 65 , 67 , 70 , 71 , 72 , though some regolith and bedrock salt concentrations can be much higher 73 . The ratio does vary (there is a higher proportion of perchlorates from Phoenix Lander samples than from Gale crater samples) though Mg and Ca tend to be much more dominant over Na salts 67 , 71 , 72 .
The presence of so much salt in the regolith makes salinity one of the major concerns for Martian regolith in growing plants (among other important potential applications). Preliminary regolith experiments that used high concentrations of perchlorates (2 wt%) resulted in no plant germination, where other experiments with lower concentrations resulted in plants with a significantly lower dry biomass and smaller leaf area overall 10 , 74 . Visscher et al. demonstrated severely limited growth of Arabidopsis thaliana in response to Mars-like levels of magnesium sulfate 73 . Thus, the overall salinity is high enough to be problematic for salt sensitive crops, but the most prominent concern is the perchlorate, as it can also induce molecular oxidative stress 75 . Perchlorates likely occur at high enough concentrations to create concerns for toxicity of food sources (however this depends on the extent that it is taken up into the edible organs, which depends on the crop), though a more precise understanding of the potential for bioaccumulation has not been established 76 , 77 .
Martian regolith physical properties and implications for plant growth
Much like the lunar regolith, Martian regolith has been subjected to a long history of impact processes which influence particle size frequency and other grain characteristics. Eolian and fluvial processes additionally sort and alter grain characteristics and the balance of these are key in the resulting grain characteristics and thus the resulting cohesive and shear strength of the regolith. For present day regolith eolian and impact processes are the dominant processes of concern 78 . Impact processes tend to have poorly sorted, angular materials which can increase cohesion while eolian processes tend to increase sorting and rounding of grains, decreasing cohesion. Particle size frequencies also differ: eolian ripples are often bimodal (occasionally multimodal) likely indicating the involvement of multiple processes 79 . Herkenhoff et al. measured bedforms in Gusev crater to have one mode dominated by grains between 1 and 2 mm and the other dominated by grains below 210 µm 79 . Outside the bedforms, a mixture of grain sizes and clasts was observed.
Thus, the cohesive and shear strength of Mars regolith resulting from grain characteristics can vary depending on which processes dominate. This is seen in recent challenges of “drilling” into the surface faced by the InSight mission “mole” probe designed to function in a cohesionless material (such as a basaltic sand) but failed to penetrate to adequate depth due in part to the higher than expected cohesion 80 . Like lunar regolith, these physical properties of the regolith limit the ability of root penetration and development making plant development difficult in the regolith without appropriate treatment.
In addition to the physical and mechanism contributions contributed by particle size, density, and angularity to the cohesion and shear strength of Martian regolith, the aggregational nature of certain materials in Mars regolith, namely salts, increase the regolith’s cohesive aspects 78 . The precipitation of salts in the regolith help to cement the soils together and the occurrence of cemented chunks or even duricrusts has been observed during the course of multiple missions including InSight and Curiosity 51 , 81 , 82 . Because this cement is at least partially soluble in water, any treatments or processing with water (including watering plants) can also affect the distribution or extent of cementation within a sample, perhaps decreasing it with rinsing. Thus, appropriate processing of Martian regolith must take these both the physical and chemical (cementing) aspects into account when considering effective approaches to addressing the regolith strength for agricultural applications.
Overview and current state of the challenges of lunar and Martian regolith as a growing medium
The above discussion presents both benefits and limitations for using regolith on the Moon and Mars for growing plants. The challenge for current and future research is to present ways in which limitations may be ameliorated, benefits maximized, and the overall result compared with other avenues to determine the most sustainable agricultural approaches to apply.
Since the Moon is Earth’s nearest cosmologic neighbor, human infrastructure that is developed on the lunar surface will benefit from the comparatively easy and quick access to terrestrial resources, even though established lunar colonies are intended to be mostly self-sustaining. This means that it is more feasible to supplement the lunar regolith with fertilizers from Earth or from waste and recycling if there are vital nutrient deficiencies that cannot be addressed using in situ lunar materials. This convenience is exclusive to the Moon because of the small distance to Earth, so other planetary settlements (e.g., Mars) will need to be able to supply the necessary nutrients with little to no material input from Earth.
In general, those nutrients required to supply fertilizer could be provided from in situ or waste materials efficiently recycled. Macronutrients such as nitrogen, phosphorus and potassium are of particular concern as they would be required at volumes that would be difficult to supply from Earth 64 . Phosphorus is relatively abundant on Mars and at least some fraction in plant extractable form 83 . Potassium is not particularly high in the Martian soil, but is present in bedrock units and could potentially be obtained from other surface materials in situ 84 . However, Potassium and Phosphorus are limited on the Moon and would likely need to be supplemented from Earth-based sources, or provided by plants and people pseudo-in situ 85 . Nitrogen has been detected on both the Moon and Mars, but only in trace amounts and no reserves large enough to support in situ supplies has been detected 68 , 86 . Micronutrients like iron, boron, and chloride are frequently detected on Mars and likely available in situ, but may also be problematic if present at too high of concentration of a bioavailable form to become toxic 87 . The Moon contains an abundance of metallic nanophase iron that depending on mobility may have concerns for iron toxicity as well, though studies are needed to establish the expectant mobility within closed systems presented by lunar colony habitats. Calcium, magnesium, and sulfur are very abundant on Mars and expected to be plant extractable through salts. Salts present a problem in and of themselves 66 , 67 , 88 , but even the issues of salinity on Mars have potential solutions 73 .
One potential solution is to genetically modify crop plants to tolerate higher concentrations of perchlorates, which has been somewhat effective in Arabidopsis 73 , however this has only been demonstrated in Arabidopsis, and will likely not be developed in larger crops within a reasonable timeframe, if at all. Another commonly cited solution is rinsing of the regolith as each of the problematic salts are readily soluble and could potentially be rinsed out of the soil. However, initial experiments from Oze et al. demonstrate limitations to this approach 74 . Perchlorate may also play a role as a resource as various biogenic reactions can degrade perchlorate to chloride producing oxygen as abyproduct 77 . However, even though potentially useful, there are many aspects of such an approach that need to be addressed: e.g., to what extent biogenic reactions would be effective, how much oxygen could be expected, and what to do with the chloride that results which is also problematic at those concentrations for the plants.
Lunar and Martian regolith simulants and agriculture research
In order to identify appropriate methods for processing regolith for agricultural applications, potential methods must be tested. However, samples of regolith are not generally available for use in agricultural research. Instead, regolith simulants are created that attempt to mimic the characteristics of actual regolith. In order for results to accurately reflect plant response to regolith-based growing mediums, they must accurately mimic agriculturally relevant characteristics of actual regolith 19 , 22 , 61 , 89 , 90 , 91 . Multiple regolith simulants have been produced for both the Moon and Mars and a summary of these is available from a recent review by ref. 22 .
The accuracy of a regolith simulant is limited by many factors including the availability of data for the target regolith, materials that resemble the target regolith, and feasibility of mimicking particular characteristics on a large scale 91 . For example, lunar regolith contains agglutinates and nanophase iron that are likely to have significant effects for agriculture, but very few simulants mimic these characteristics due to the difficulty of reproducing them on even a small scale. Martian regolith contains salts and other components relevant to agriculture identified in recent missions such as Curiosity. However, the most widely used Martian regolith simulants (JSC Mars 1a and Mars Mojave Simulant (MMS-1 and MMS-2)) do not contain these components as they were developed early in the exploration of Mars before such characteristics were well understood 19 , 61 , 89 , 92 . As data and methods improve, so does the need to create new simulants that more accurately mimic the target regolith. Indeed, the last few years have seen great efforts in increasing the accuracy of both lunar and Martian simulants including a few higher-quality commercially available options 19 , 61 , 93 , 94 . However, it is not feasible to mimic every aspect of regolith so even the more accurate simulants are often more accurate in features particular to a specific application. The review provided above and best practices recommended below do not intend to sponsor or promote any particular simulant. Rather we emphasize the need to ensure the accuracy of relevant characteristics for simulants aspects applied to RBA research to the extent possible and relevant for addressing the fundamental questions intended in the study.
A brief history of regolith-based agriculture research
Over decades, researchers have theorized about growing crops on the Moon and Mars.
As early as 1970, Walkinshaw and his collaborators analyzed plant physiology and productivity when exposed to lunar materials from the Apollo missions 95 , 96 , 97 .
Baur et al. and Milov and Rusakova, built on these preliminary studies, with the latter adapting a more applied view by suggesting a closed greenhouse system as a possible solution 98 , 99 .
The closed greenhouse idea would be later explored by Walkinshaw in 1986 while he tried to fully understand the interaction between plants, regolith, and microorganisms in an enclosed system 100 .
Mashinskiy and Nechitaylo’s “The Birth of Space Agriculture” was the first organized review on the subject 101 .
Ming and Henninger published a book that directly addressed this subject in a compilation of articles that discussed the feasibility of off-world Agriculture 102 . In this book, Fairchild and Roberts 103 contemplated options for human settlement on the Moon and Mars in a detailed way and divided their narrative into four scenarios entitled “The 1988 Case Studies” 102 . The first scenario was a human expedition to Phobos that did not involve ISRU utilization. The second was a human expedition to Mars with a crew of eight, where four crew members would reach the surface to collect data with heavy orbital support and some IRSU utilization. The third case study was a lunar observatory outpost that would switch categories and be classified as a science outpost in the resource allocation category. The outpost would house a crew of 4 for 20 days while all the resources would be provided in situ to the crew during this period but still with partial orbital support. The fourth and last case, the Lunar Outpost to Early Mars Evolution scenario, would be an ISRU-based, self-sustaining station housing a crew of eight over a period from 24 to 52 weeks between rotations 103 . The concerns and possible solutions to the technical, economic, political, and cultural issues that acted as barriers to permanent settlement beyond LEO in their time remain relevant to this day.
However, case studies like those listed above-inspired researchers to overcome these challenges and ultimately provided the foundational data to begin the discussion of ISRU for life support systems and led to the creation of NASA’s Controlled Ecological Life Support System program. Ripples of this movement were fast to follow, with publications focused on integrating ISRU with Regenerative Life Support Systems (RLSS) and the discussion of possible agricultural scenarios for lunar and Martian outposts 37 , 104 , 105 , 106 .
As knowledge progressed, researchers saw the necessity to develop more reliable tools to mitigate plant stress and thus improve crop productivity. One of these first approaches was by ref. 107 , who emulated ecological substrate colonization phases in regolith using pioneer species (e.g., Tagetes patula L.) associated with root-colonizing microorganisms. Kozyrovska observed that it took a long time until the substrate was ecologically stable enough to support full crop cycles, though this work did eventually grow plants 107 . These results further strengthen the idea that some regolith amendment was needed to make regolith-based agriculture possible, which steered the scientific community to explore several approaches to “hack” off-world agriculture.
In recent years, exploration of the topic has continued with a broad focus of applications from experiments that explore small-scale early exploration possibilities, challenges, and conditions 74 , 108 to long-term exploration that considers potential of much larger-scale agriculture (e.g., 61 ). Though, even after showing promising potential 64 , 109 , 110 , regolith amendment technologies still seem unable to create a sustainable crop rotation system. These challenges underscore the need for further investigations, especially when it comes to controlling severe pH shifts around the rooting zone stratification (either acidic or alkaline) that directly affect the plant’s physiology 111 , by the presence of elevated or trace levels of perchlorate in Martian regolith-based trials 10 , 74 , 112 or by the need of an active microbiome that is capable of surviving in a regolith substrate, utilizing the substrate and other in situ resources, and reverting them in a continuous way to create a closed nutrient cycle that has both plants and microbiome as the primary energy providers at each end of the spectrum. Most recently, small portions of lunar regolith collected during the Apollo Program were used to successfully germinate and grow Arabidopsis thaliana 113 . This study provided crucial confirmation that lunar regolith does indeed have the potential to function as a substrate for plant growth, albeit with significant stress to this model plant.
Applications and sources of microorganisms in regolith-based agriculture
Sterilization of nearly all surfaces, including biological ones like seeds, is a common strategy in space exploration for a variety of reasons. However, an axenic approach to sustainable agriculture i.e., one in which the plant(s) is/are the sole constituent step of a BFS, is not a reasonable, nor desirable approach in any environment. The origin and success of terrestrial plants are intimately coupled to the microbial cohorts that populate their surroundings and surfaces, affecting them physiologically, genetically, and biochemically throughout their life 114 , 115 , 116 , 117 . Indeed, the region of soil within 1 cm of the root surface, known as the rhizosphere, often contains a significantly more dense and diverse population of microorganisms than bulk soil. With estimates as high as ≈10 11 microbial cells/gram of host tissue with over 10,000 different species present roots are a “hotspot” of bio- and metabolic diversity 117 , 118 , 119 , 120 . These diverse microorganisms impact agricultural yields, plant disease resistance, nutrient utilization, nutrient uptake, ecological robustness, and secondary metabolite production 114 , 121 , 122 , 123 , 124 , 125 , 126 , 127 . So crucial is this relationship, that both plants and microbes have evolved a variety of biochemical pathways to drive the selection and maintenance of these interactions. This population adapts to and influences their host plants both spatially and temporally, making them crucial players which must be incorporated into any model for sustainable agriculture regardless of the ecosystem they will ultimately inhabit 114 , 122 , 124 , 128 , 129 , 130 .
Understanding and manipulating the holobiont, the total collection of the host plant and its microbial cohorts, is key to optimizing plant growth especially in challenging substrates like lunar or Martian regolith. While hormone manipulation, such as the reduction of ethylene production, is a generally useful feature conveyed by some plant growth-promoting (PGP) bacteria, activities that directly modify the growth substrate and/or nutrient availability are likely to be of greater utility in regolith-based agriculture 114 , 117 . PGP phenotypes that could directly impact nutrient availability or regolith composition include: (i) phosphate solubilization, (ii) nitrogen fixation, (iii) iron sequestration and redistribution, and (iv) the formation of soil organic matter (SOM). Phosphate solubilization is a common PGP phenotype observed in the rhizosphere performed by both fungi as well as bacteria. Similarly, bacteria-derived chelators known as siderophores can sequester and redistribute the rich iron deposits within regolith, improving availability of this important micronutrient to host plants. Nitrogen fixation by free-dwelling or nodulating species of bacteria is of particular interest given the limited amounts of this important macronutrient observed in regolith to date, as well as the costs (and risks) associated with shipping solid nitrogen-based fertilizers to a lunar or Martian colony 86 . Finally, rhizosphere bacteria can utilize root derived exudates as well as other exogenous sources of carbon to facilitate the conversion of regolith to SOM.
Though important differences exist between the microgravity environment of LEO and the hypogravity environments of the Moon and Mars, studies aboard the ISS can inform our understanding of some aspects of growth in regolith, such as the composition of available bacteria for formation of a robust microbiome at plant surfaces 131 , 132 . The present approach of seed surface and growth substrate sterilization means that the plant microbiome which does form in these isolated environments will be almost entirely derived from the microbiome of the colonists, or the endophytic bacteria safely protected within the seed coat 133 , 134 . However, even from within this population of primarily human-derived microorganisms, a number of potential PGP microbes have been identified 135 . This underscores the potential to select and develop beneficial microbiomes from the populations most likely to develop in these isolated environments and which have established spaceflight histories. While such studies are ongoing, an alternative or complementary approach will be the introduction of specific microbial cohorts to facilitate RBA, an approach which has been explored in a variety of regolith simulants. Here we review these initial efforts to exploit microorganisms to improve the ISRU viability of Martian and/or lunar regolith with a particular interest in their potential to support plant growth.
Establishing a baseline—microbial composition of regolith simulants
Both environmentally and anthropogenically sourced regolith simulants are likely to harbor microorganisms which can influence the results of RBA research. Sterilization of these simulants to remove these initial populations is problematic due to the limited ability to sterilize bulk quantities for larger research, as well as the potential for various sterilization methods to alter the composition of the regolith simulant. Yet little characterization of the initial microbial composition of these simulants has been conducted to date, despite their potential to impact the results of work conducted therein. Studies by Allen et al. examined the microbial profile of JSC Mars-1A, an early and heavily utilized MRS 136 and identified a number of bacterial and fungal species present in untreated simulant 137 , 138 . Among the eight species of Bacillus they identified were B. megaterium and B. licheniformis , both of which are potential PGP species via improved nutrient solubilization or gibberellic acid production, respectively. Several species from the order Actinomycetales were also identified, including at least one species of Streptomyces . Several fungi from the genera Aspergillus , Penicillium , and Fusarium were also isolated. Aspergilli and Penicillium species may have PGP potential via phosphate solubilization as well as the production of antimicrobial agents to control microbiome composition 139 , 140 . Meanwhile, the presence of Fusarium species is of significant interest, given they are well-associated with a variety of crop diseases 141 . Similar efforts to characterize the initial microbial load and composition of other regolith simulants have yet to be performed, but are important to adequately establish the starting microbiome for plant-microbial associations in RBA research. While standard enumeration assays could provide quick insight into the total number of microorganisms in these samples, species/genus resolution would help identify the potential for PGP phenotypes already present among these populations.
Microorganisms viability in and utilization of regolith simulants
Regolith simulants have been extensively used in research for astrobiology as well as planetary protection, as substrates for a variety of microorganisms exposed to the harsh environments of Mars. For example, several species of methanogenic Archaea introduced into a variety of regolith simulants can survive both desiccation and the hypobaric (low-pressure) environment associated with the surface of Mars 142 , 143 . In these species, metabolic activity, specifically methane production, was restored by rehydration suggesting a model for how microscopic life on Mars could persist via rounds of desiccation and rehydration via intermittent flows of water.
Similar studies were conducted with a variety of bacterial species and confirmed that strains of B. subtilis and Enterococcus faecalis suspended in a variety of regolith simulants were also resistant to extreme desiccation, perchlorate exposure, reduced atmospheric pressure, and Mars-equivalent UV exposures 144 . Regolith analogs have also been used for planetary protection studies, specifically to observe whether bacteria could be transferred from the wheels of a rover to the Martian surface 145 . Taken together, these studies further establish regolith as a viable substrate and reservoir for microorganisms, one that could protect some species over short durations in the case of life support system failures, a potential benefit to RBA efforts.
Based on existing data, both lunar and Martian regolith contain many macro- and micronutrients of benefit to the health of plants and humans, as well as potential industrial applications. In our discussion of existing microorganisms in regolith simulants above we identified several species with the potential to improve plant growth, based on their ability to improve the solubilization of important materials such as phosphate. However, the use of microorganisms to improve the extraction of other essential elements from regolith simulants has also been explored. Work by Cousins et al. explored the potential of JSC-Mars-1A as a biomining substrate for the extraction of iron by several species of bacteria including Acidithiobacillus ferrooxidans , Shewanella oneidensis , and Magnetospirillum gryphiswaldense 146 , 147 . In most cases, the cost-benefit analysis confirmed that the nutrient inputs required exceeded the value of the iron extracted in these studies. However, S. oneidensis did emerge as a promising candidate for the extraction of iron for a variety of applications in a cost-effective manner and could be utilized in an early pre-treatment step.
Further work with A. ferrooxidans utilizing both LMS-1 and MGS-1 as substrates confirmed their ability to improve the solubilization of Si 1+ , Mn 2+ , Mg 2+ , and iron (as Fe 2+ ) suggesting this microorganism may also be of benefit depending on the simulant and growth conditions employed 148 . In this latter study, cultures grown in a clinostat showed a significant increase in mineral solubilization relative to 1G controls. However, given that the microgravity conditions simulated by clinostats can vary significantly from the partial gravity environments on Mars and the Moon, the importance of these enhancements is unclear. Ultimately, the ability to mine essential minerals from regolith could be of considerable benefit to RBA.
Plant growth-promoting microorganisms in regolith
The studies above support the potential of microorganisms to transform regolith into substrates better suited for plant growth. While such bioweathering/bioleaching may occur with greater frequency within the rhizosphere and could provide inorganic nutrients in support of plant growth, they are not due directly to interactions with a host. Yet, the importance and potential benefits of plant-microbial associations are becoming increasingly clear in the development of next generation agricultural solutions here on Earth and will almost certainly be part of a strategy for successful RBA systems off-world. Here we will discuss preliminary regolith-based research which incorporated the two most well-established plant-microbial associations: nodulation and mycorrhizal fungi into regolith studies.
The legume-rhizobia symbiosis supports plant growth via nitrogen-fixation in ~20% of all land plant species and represents a crucial interaction which could minimize potentially damaging environmental inputs of fertilizers 149 . As a result, there is considerable interest in whether lunar or Martian regolith could support this mutualistic symbiosis. Seeds of Melilotus officinalis (sweet clover) have recently been shown to support the formation of active N-fixing nodules in conjunction with Sinorhizobium meliloti 1021 in MMS-1 150 . This study confirmed that nodulation was sufficient to increase total plant biomass accumulation by an order of magnitude relative to uninoculated controls. Species like clover are routinely used to introduce nitrogen and organic matter into soils and could be used to convert regolith into soil prior to farming attempts. Similarly, Rainwater and Mukherjee confirmed nodulation as well as increased growth in seedlings of Medicago truncatula , a model system for symbiosis research, in both MMS-1 and MMS-2 by three different species of rhizobia 151 . While both of these studies have been conducted in regolith simulants of lesser accuracy, they underscore the potential to capture this important symbiosis for sustainable agriculture. Notably, successful nodulation under hydroponic conditions is less established and their benefits on growth are poorly characterized in contrast to a wealth of information from soil research.
While nodulation represents an important and relatively common plant-microbial symbiosis it is far from being the most common of these associations. Indeed, an estimated 75% or more of terrestrial plants are capable of forming associations with mycorrhizal fungi to improve nutrient uptake, sensing, and disease resistance 152 . Such associations are presumed to be among the earliest plant-microbial symbioses to have evolved in terrestrial plants and harnessing this activity would be immensely useful for RBA systems. Preliminary efforts confirm that the lunar regolith simulant JSC-1A is capable of supporting the growth of the mycorrhizal fungi in association with prickly pear seeds (Opuntia ficus-indica ) 153 . Specifically, Trichoderma viride as well as one or more isolates of the genus Glomus, both of which can improve host plant nutrient uptake and/or limit pathogenic infections by deleterious fungi. The addition of mixed cultures of these fungi significantly improved the germination of seeds of O. ficus-indica underscoring the potential for such microbial amendments as a tool for improving cultivation at an additional point (germination) not just in support of vegetative or reproductive growth.
While specific plant-microbial interactions are beneficial, an approach that leverages species and functional diversity is more robust and sustainable over the life of a single host plant as well as for the substrate (regolith) that will sustain crop growth over multiple generations. Decomposers are an excellent example of such microbial systems, functioning similarly to the biomining species described above, but primarily liberating organic material from dead tissues. This activity is a crucial aspect of bioregenerative life support and will become increasingly important as researchers begin to incorporate crops with significant amounts of inedible biomass into our models for off-world food production. With this in mind, Gilrain et al. incorporated decomposers into their food production system by generating compost to supplement the growth of Beta vulgaris (Swiss chard) in JSC-Mars-1A 154 . Duri et al. utilized such decomposers to generate compost as a supplement for samples of butterhead lettuce ( Lactuca sativa ) grown in MMS-1 109 . Their findings suggested that compost may help to modulate cation release, limiting the phytotoxicity of elements like Al3+ while limiting runoff loss to others like Ca2+ and Mg2+. Complementary studies confirmed that the addition of composted material impacted hydrological parameters of the regolith, further limiting the risk of leaching of potential phytotoxins 111 .
Lytvynenko et al. made one of the first attempts to assemble an engineered microbiome for lunar regolith using anorthosite as a lunar regolith proxy 107 , 155 , 156 . These studies envisioned the use of this consortium to extract beneficial nutrients from regolith to support plant growth, in this case Tagetes patula (French marigolds). Among these were an isolate from the genus Paenibacillus (sp. IMBG156) which formed biofilms on the surface of sterilized anorthosite particles. In these biofilms, this strain liberated both Ca 2+ and Si 4+ as well as oxidizing Fe (II) to Fe (III), improving plant access to all three of these ions under conditions that would also support plant growth. Pioneering strains like these could be used to begin remodeling regolith in advance of planting facilitating the first round of growth. Other members of this consortium included known plant growth promoting microorganisms Klebsiella oxytoca IMBG26, Pseudomonas sp . IMBG163, P. aureofaciens IMBG164, and Pantoea agglomerans IMV56. This consortium supported T. patula growth in anorthosite improving germination, survival, and growth, most notably allowing plants to flower, which did not occur without the engineered microbiome in anorthosite.
While preliminary, these various lines of evidence support the potential of incorporating a variety of the plant-microbial associations that began with the earliest terrestrial plants. Ultimately, a systems or holobiont approach to RBA which incorporates select microbial cohorts will integrate more efficiently with any bioregenerative life support system at work in an off-world colony. This would replace the existing strategy of the indiscriminate elimination of all microorganisms which has proven remarkably unsuccessful though clearly, further work in this area is required.
“Best practices” for the future of research in regolith-based agriculture
The body of RBA research available to date consists mostly of preliminary plant growth experiments addressing fundamentally basic topics of agricultural science applied to the Moon or Mars. While this provides important groundwork and insights into the potential for RBA, many of these studies, including our own works, still fail to provide a clear picture of the precise procedures capable of providing successful approaches for regolith-based agriculture beyond Earth. In order to be successful, off-world we need to be able to accurately predict crop yield, even under potentially disastrous situations. Yet there is a significant gap between current research and the successful realization of an RBA system with such reliability. We can summarize these gaps into four broad areas for improvement: 1) Regolith simulant preparation, amelioration, and alteration, 2) evaluating plant growth and response, 3) microbial identification, community development, and response, and 4) environmental controls of the experiments themselves.
However, providing a tightly controlled environment, making accurate regolith simulants, testing plant physiology and genetics, and assessing microbial status all require specialized knowledge and training, can be very expensive, and the equipment needed is often difficult to access. Depending on access to funding and expertise, it may not be feasible to do all these aspects at the same time in every experiment. We highly recommend collaboration as a solution to this issue; however, this option is not necessarily available in every scenario. To help address the gaps both in knowledge and accessibility, we begin by identifying key knowledge gaps in the form of specific questions. We follow these questions with tiered recommendations for experimental design and reporting of results that will assist in filling these gaps. These tiers (Introductory, Intermediate, and Advanced levels) establish the experimental rigor dedicated to each of the 4 areas and are intended to be deployed in a “mix and match” fashion, permitting researchers to address specific RBA knowledge gaps with their research while meeting certain minimum requirements. The distinctions help guide researchers through a structured progression in their work, ensuring that basic concepts are mastered before more complex, nuanced, or technologically advanced investigations are undertaken.
Introductory
These are foundational research activities designed for new entrants into the field. They focus on basic principles and simple experiments that are critical for understanding the fundamentals of regolith-based agriculture. This level is meant to build a solid base of knowledge and skills.
Intermediate
At this stage, the research delves into more complex interactions within regolith agriculture, such as chemical properties and plant responses. It assumes a certain level of foundational knowledge and aims to expand on that by exploring more detailed aspects of plant growth and soil interaction.
This highest level involves sophisticated research methodologies and experiments. It’s aimed at those with a strong background in the field, looking to push the boundaries of current understanding. Advanced recommendations often involve integrating multiple complex factors or innovative technologies to address the challenges of growing plants in regolith.
These tiers are intended to be inclusive, not prohibitive, by providing clear standards and practices for experimental design and publication, that will make it easier for new researchers and their projects to join the RBA community. To better illustrate our intentions and provide some guidance, following this list of recommendations we have also provided a series of decision trees as well as an illustrative case study evaluating the four key areas of RBA questions and their specific tier. Through the act of asking “what is best?” we hope to help establish the current boundaries of our understanding, recommend research practices that bring coherence and an ability to communicate results more effectively in the community, and illustrate examples of some of the ways these recommendations can be applied. Together these key questions, recommendations, and illustrative examples can provide a set of “best practices” intended to help provide greater coherence and focus for RBA research that can begin to fill the gap and bring it to a TRL at which it becomes a reliable component of sustainable off-world agriculture.
Key questions and knowledge gaps
Here is a list of key questions or needs that make up the current knowledge gap of RBA research. This is not an exhaustive list, but a starting point that helps to inform the recommendations that follow. The authors have written this list with their expertise informed by literature and have refined into the questions they deem more pressing. Indeed, some of these questions represent noticeable knowledge gaps which must be addressed before all of our best practices can be implemented as well as questions for which the implementation of the recommended best practices can help provide more coherent answers. Additionally, microbes, plants, and regolith all interact within their environment, thus some overlap exists among questions approached from the perspective of each aspect. This merely underscores the utility of this effort. Even if it were possible to list all the current key questions, the very act of addressing these questions will bring forth more questions all of which will inform the recommendations that follow allowing them to evolve as the knowledge gap is filled. This exercise represents a starting point from which more questions must be asked, research approaches and practices can evolve and knowledge gaps can be filled. Here are specific questions that emerged from this exercise:
Regolith and regolith simulants
Q1. How accurately do regolith simulants currently developed represent actual Regolith?
Motivation: A few disparate studies that evaluate simulant accuracy are available, but most only examine a few simulants and use vastly different approaches for this evaluation. There are also a few reviews that list available simulants for the Moon and Mars covering many simulants (e.g., Duri et al.) but have limited exploration of simulant accuracy to actual regolith 109 . A more thorough evaluation of developed simulants that includes both a more extensive number of simulants and a thorough evaluation of simulant accuracy would be a valuable resource for understanding current simulant limitations, recommendations in simulant development, and a list from which researchers can select simulants most appropriate for the intended study. Though it is also recognized this represents a significantly challenging endeavor as published data or simulant samples are not always readily accessible.
Q2. What data is needed about actual lunar and Martian regolith to better evaluate regolith fertility and how these be measured by current and future robotic missions?
Motivation: Though we do understand much about lunar and Martian regolith mineralogy and chemistry, certain aspects relevant to agriculture are not well constrained. In particular the amount and bioavailability of plant relevant nutrients and phytotoxins, direct measurements of soil pH and salinity, the composition and geochemistry of poorly understood components of lunar and Mars regolith (for example the amorphous portion of Mars regolith and other surface materials), presence of minable in situ resources of fertilizer components not available in the regolith directly. Many of these aspects are difficult to apply to robotic exploration and thus have limited or no data available, but advances in engineering and technology have increased and will continue to increase the ability to measure such characteristics.
Q3. What non-regolith materials may also have agricultural applications and what data is needed to understand potential contribution to viability and fertility
Motivation: This is a continuation of question 2, but applied to other surface materials that could be processed or applied as well. For example, acidic-sulfates on Mars could help regulate the pH of soils or hydroponic fluid, however to adequately understand the viability of such techniques we need data from actual surface materials to better characterize them and simulants that accurately mimic their characteristics in order to test them.
Q4. What agriculturally relevant characteristics are lacking in available regolith simulants and are there viable techniques that can accurately replicate them at appropriate scales?
Motivation: If the comparisons suggested in question 1 can be more thoroughly established, that leads to consideration for simulants applied to RBA studies and what characteristics are not mimicked that may have important agricultural implications. To what degree is it possible to adapt current simulants or develop new simulants that do mimic these characteristics. It is also important to realize that the degree to which this question can be answered is limited by our current understanding of actual regolith and as that knowledge increases it is useful to reevaluate current simulants.
Q5. Which nutrient extraction methods are best correlated and calibrated for regolith materials, or are any of them?
Motivation: Nutrient extraction is used to evaluate not just the amount of nutrient in soil, but the amount of plant extractable nutrient 157 . These tests are not based on direct measures but rather known correlations among certain extracting materials and plant uptake that have been calibrated to fertilization recommendations. However, this correlation is not very consistent among different soil types as the extractants can behave very differently in differing soil conditions, thus different locations often select tests most applicable to major soils in their region. However, such correlations and calibrations have not been established for regolith simulants thus any nutrient or fertility evaluations may or may not provide viable results. A study that examines different extraction methods on different simulants and if they correlate with plant uptake or not is needed for such fertility tests to be accurately evaluated and for resulting recommendations for increasing fertility to be viable.
Q6. What effects do sterilization, and other pre-processing techniques, have on Regolith Simulant composition? How effective are these techniques at sterilizing regolith simulant materials?
Motivation: There are many situations in which it may be useful to sterilize regolith simulants (or at least attempt to) in order to provide a sterile medium for which to begin a study. However, many of these techniques can drastically alter mineral materials. The potential for alteration will differ among mineral type and sterilization method, but we are not aware of any studies that directly evaluate these alterations and the implications on simulant composition and its relevance to actual regolith. It is also difficult to truly sterilize soil-materials and it would be useful to examine the effectiveness of a method compared to the degree of alteration it induces. Though sterilization would be among the most commonly applied processing techniques expected, the same concerns hold true for any processing applied to a simulant to prepare it for a particular study.
Q7. How well do the many ameliorative techniques proposed improve the regolith fertility and how variable is this success among different regolith types?
Motivation: There are many approaches that have been proposed for developing viable materials from regolith. For example, rinsing Martian regolith to remove salts including perchlorates. However, testing such techniques on a Martian regolith simulant that does not contain salts would provide completely useless results. But even simulants that accurately represent Mars regolith salinity would need approaches and evaluations that are comparable among studies or studies that examine multiple simulants. This overall concept holds true for any ameliorative technique attempted: i.e., first and foremost such studies must use a simulant that accurately mimics the relevant characteristics seeking to be ameliorated, and even when that is the case comparison to other studies or among multiple simulants allows provides a more broadly applicable ameliorative solution.
Q8. How does exposure to the experimental conditions alter regolith simulants, how are the alterations evaluated and what implications does this have for actual regolith and how is that evaluated?
Motivation: Similar to the alterations that can be induced from processing techniques referred to in question 6, the alterations that occur as a result of the experiment are also important, though they depend on the intent of the study. However, as weathering or alteration of mineral materials can be slow these changes may be subtle (though not insignificant) and commonly applied techniques (like XRF or XRD) may not be able to measure some changes that are important for plant response. However, XRF and XRD are highly useful in that (partly because they are so common) can provide results more comparable among different studies while techniques more sensitive to small alterations may be more limited in their comparability. Simulants (and indeed most growing mediums) can be highly heterogeneous and thus insufficient replication or sample size can limit the ability to detect patterns. Thus a combination of techniques and adequate sample size are important to consider in determining how to measure changes in simulant characteristics.
Plant response to regolith-based substrates
Q9. In what ways are plant tissues chemically affected as a result of being grown in regolith or regolith simulant, and how might this affect edible and non-edible yield and quality. Which tissues are most and least affected by plant growth in regolith or regolith simulant?
Motivation: Photosynthesis is inhibited 158 and plant morphology and physiology are affected 159 by heavy metals, so both ultimate yield and plant quality varies depending on the elements (and elemental forms) taken up by plants. However, plant translocation of elements is complex, and depends on plant species, environmental content of elements, oxidation/reduction potential, pH, and other factors. Therefore we must explore these effects in regolith to understand how regolith may affect plant and human health in the long term.
Q10. When grown in regolith or regolith simulant based growing mediums, in what ways would plants alter the medium? How might root exudates alter regolith properties over the short-term and long-term? How might regolith simulants alter plants’ relationships with root-colonizing (especially root nodulating) microorganisms, and what key factors could cause these effects?
Motivation: We have established that soil quality has direct effects on plant physiology, growth, and quality, however plants also have reciprocal effects on the rhizosphere. Plant roots produce exudates that contain metabolites and a wide variety of compounds, which alter chemical and physical properties of soil, help maintain and support microbes, and have critical impacts on long-term plant health 160 , 161 . Therefore, to understand the long-term effects of using regolith for agriculture, we must elucidate how regolith may change over time as a result of agricultural use.
Q11. What avenues are most appropriate to increase organic carbon content in regolith or regolith simulant based growing mediums? What stable organic amendments are most effective at both supplying organic carbon for plants and improving the texture of the substrate for supporting plants? Are the avenues actually helpful at improving the viability of the mediums?
Motivation: Soil carbon is required for plant and microbial growth, however its availability in the soil is affected by soil amendments, root exudates, microbial activity, and other factors 162 . Therefore we must find methods to supply enough carbon to regolith to meet the needs of plants and the rhizosphere microbiome, and understand how organic amendments affect RBA.
Q12. Do current soil nutrient extraction tests applied to regolith or regolith simulants correlate sufficiently with plant uptake to determine reliable fertilization recommendations?
Motivation: fertilizer recommendations are made using soil/nutrient testing; however these tests may not provide accurate or adequate fertilizer recommendations when used on regolith. Therefore, we must examine whether these tests are accurate and work to optimize them for regolith applications, such as by using regolith simulants with known chemical properties to calculate the ideal dose of each nutrient.
Q13. As we consider RBA compared to hydroponic methods, what crop species may be particularly well-suited for RBA? Do these crops fulfill the needs of astronauts, or is further crop selection necessary? Are these crops that meet current crop selection criteria feasible to use in RBA, when other considerations (such as the need for food processing) are considered?
Motivation: Multiple studies have established a consensus of logical plant choices that fill nutrient requirements for future off-world agricultural efforts 131 . However, these are based on the assumption of fully hydroponic systems, and use of regolith may alter what considerations are most relevant. Therefore we must reconsider crop selection needs and reevaluate whether these crop lists are usable with RBA.
Microbial community identification and response to regolith-based substrates
Q14. What is the endogenous microbial composition of the common regolith simulants?
Motivation: A basic understanding of the existing microbiome of regolith simulants would help distinguish between substrate effects and microbial ones. As sterilization of large amounts of regolith simulants for scale-up studies is not possible, identifying these microbes becomes an important step moving forward.
Q15. Does this endogenous population significantly alter these simulants post-acquisition?
Motivation: Over time, the endogenous microbiome of the simulants may alter regolith composition. In other words, regolith simulants, stored under Earth conditions, will age differently than regolith on Mars or the Moon. Although such changes are likely to be slow it should still be part of our consideration as regolith becomes soil, in part, through the actions of microorganisms.
Q16. Do these simulants contain plant growth promoting microorganisms?
Motivation: The potential that plant growth promoting bacteria are already in regolith simulants may be impacting plant growth studies already and a baseline needs to be established.
Q17. How well can plant-associated microorganisms be cultivated in regolith?
Motivation: At present, there is no information on how the rhizosphere and phyllosphere of plants grown in regolith simulants may vary from controls grown in more traditional soil substrates, or how this affects plant-associated microorganisms. Low nitrogen levels in both Martian and lunar simulants, for example, may select for nitrogen-fixing microbes from the surroundings, even if they are not already present in the simulant. Understanding how these microbiomes vary as a function of substrate is crucial to implementing a cultivation program that utilizes microorganisms.
Q18. Does regolith alter the phenotypic behavior of plant-associated microorganisms?
Motivation: Closely related to Question 4, not only may there be differences in microbiome composition, but the phenotypes of specific microorganisms may vary based on regolith compositions. For example, the low nitrogen, high iron concentrations found in Martian regolith and its simulants may alter the behavior of non-nodulating nitrogen-fixing bacteria.
Q19. What challenges does regolith pose to the extraction and identification of organic compounds for evaluating the chemical composition of the rhizosphere?
Motivation: Regoliths (and simulants) are composed of materials which tend to complicate the extraction of organic compounds. This can complicate analysis of the rhizosphere chemicals both from plants as well as bacteria. Refined protocols are needed for this process for the different stimulants.
Q20. What challenges does regolith pose to the extraction and identification of bacteria or environmental DNA for evaluating the chemical composition of the rhizosphere?
Motivation: The challenges associated with the extraction of organics from the simulants will be similar for the extraction of DNA and possibly intact bacteria. This would represent an extra challenge for metagenomics and other microbial characterization approaches. Refined protocols are required for this process for the different simulants.
Q21. How does the microbiome of adjacent surfaces influence the endogenous microbiome of regolith (and simulants)?
Motivation: Off world-agriculture, regardless of its site, will occur in highly enclosed spaces for the foreseeable future. Such environments are prone to significant mixing of microbial populations between the inhabitants (human, plant, etc.) as well as the surfaces they encounter. Migration of microorganisms to regolith (and simulants) from biotic and abiotic sources in the environment could be an important source of both mutualistic as well as pathogenic cohorts. Indeed, prior studies have already identified migration from the human microbiome into the rhizo- and phyllosphere of plants aboard the ISS.
The growth environment
Q22. What is an acceptable balance of atmospheric conditions (composition, atmospheric pressure, humidity, etc.) for off-world closed food systems in a hypogravity environment such as the Moon or Mars? What considerations are needed for the interactions among plants, microbes, regolith, crew members, and the outside environment?
Motivation: The conditions conducive for agriculture in off-world systems must be investigated in order for researchers to, 1) create accurate cost projections, 2) to methods for maximizing yield, and 3) to enable engineers to design the systems that will support these crops. Researchers on Earth must also consider the air environment, especially the carbon dioxide concentration and volatile organic compounds, when undertaking analog experiments that include plants.
Q23. Similar to the aforementioned atmospheric conditions, which irrigation strategies are most effective for maintaining optimal hydration for growing plants in regolith simulant? What trade-offs exist for each irrigation strategy, and how can the effects of these trade-offs be minimized?
Motivation: Irrigation can have drastic effects on plant growth and development, and optimizing irrigation strategies for RBA will be vital. Water will be a limiting resource in space environments, making water conservation a high priority. Furthermore, the leaching proprieties of water should also be considered. Heavy or low-flow systems, systems that will only affect the rooting zone or the bulk area of the substrate, and strategies that are either bottom-up or surface sprays will have different impacts on nutrient leaching rates. Each approach will have different effects on the overall nutrient accessibility to the plants and will alter the substrate’s pH, thus influencing microbiome recruitment.
Q24. What sustainable management practices (e.g., no-till vs conventional till, or other cultural practices) applied to terrestrial agriculture may also be applicable to RBA? What are the direct and indirect effects of these management practices when applied to RBA?
Motivation: Thus far, no study has evaluated the potential benefits of conventional agriculture practices in RBA systems. Tilling is an excellent example as it may be a necessary strategy for lunar and Martian agriculture due to the low granulometry of each substrate. One downside of tilling is that nitrogen-fixing bacteria are exposed by revolving the lower tiers of the substrate, and this exposure could cause microorganism death, which would threaten the balance of the nitrogen cycling system. As tilling may be an early strategy for planting seeds in an RBA scenario before a microbiome is introduced, further studies are necessary to use conventional agriculture techniques and evaluate their transferability benefits to RBA systems. Other management practices must be explored to identify areas for improvement in RBA. Furthermore, research should prioritize practices that are realistic and feasible for use in real off-world settings.
Q25. What technology advancements would need to be developed or adapted in order to create these systems and how might multiple technologies be integrated while minimizing the risk of system failure?
Motivation: Much research has been done into hydroponics and other methods for growing crops in controlled environments, however this research has not thoroughly explored the possibility of using multiple methods (hydroponics, RBA) alongside each other, or in tandem for the same crop. Therefore further research must look at controlled environment technologies and other growing techniques, see what can (and cannot) be used with RBA, and consider the most efficient approach.
List of recommendations
Designing, selecting and monitoring changes to regolith simulants.
Access (including presumed future access) to Martian and lunar regolith is extremely limited and necessitates the use of simulant regolith materials used as proxies for regolith research. But for a simulant regolith to provide useful results, it must sufficiently mimic relevant characteristics of the actual regolith material. It is not possible to mimic every characteristic of a material, but characteristics important to the application can be the primary focus and the extent to which the simulant materials used actually reflects particular characteristics of actual regolith is used to organize the introductory, intermediate, and advance tiers of recommendations. Consideration for availability and accuracy of existing simulants is considered. Also important to consider is any treatments (e.g., sterilization) the regolith will be exposed to within the experimental design, how such treatments will affect the regolith, and what limitations that places on conclusions that can be made for some aspects of the experiment. Finally, using appropriate and consistent methods (or at least methods that can be readily compared among different studies) for tracking changes that occur to the regolith over the course of the experiment are each important to consider. Some examples of resources that provide lists of available simulants include the Planetary Simulant Database hosted by Colorado School of Mines ( https://simulantdb.com/ ), the Astromaterials Research and Exploration Science (ARES) lunar simulant project hosted by NASA ( https://ares.jsc.nasa.gov/projects/simulants/ ), and a recent review from ref. 22 (Table 2 ). Additionally, Figs. 1 – 3 provide examples for how these recommendations should be considered during experimental design.
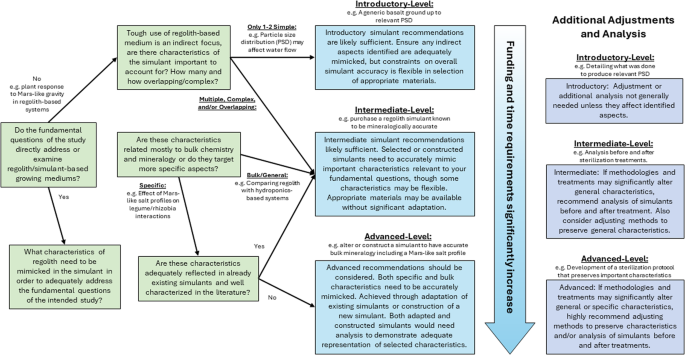
This is a decision tree describing questions and recommendations for designing RBA experiments, with an emphasis on regolith chemical and physical properties. The green boxes (left side) include questions researchers might consider when designing their experiments and choosing their methods. Arrows indicate which recommendations are appropriate, depending on researchers' answers to the questions in the green boxes. The blue boxes (middle of figure) indicate the recommendations that would be suitable for their research. The purple boxes (right side) indicate additional considerations and analyses that might improve the quality of research. The large downward-pointing arrow illustrates how the funding and time requirements of the recommendations listed significantly increase from the top to the bottom of the figure.
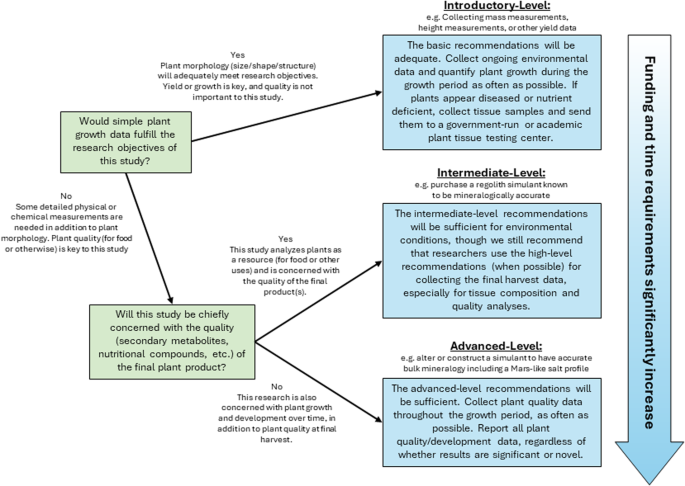
This is a decision tree describing questions and recommendations for designing RBA experiments, with an emphasis on plant growth and quality. The green boxes (left side) include questions researchers might consider when designing their experiments and choosing their methods. Arrows indicate which recommendations are appropriate, depending on researchers' answers to the questions in the green boxes. The blue boxes (right side) indicate the recommendations that would be suitable for their research. The large downward-pointing arrow illustrates how the funding and time requirements of the recommendations listed significantly increase from the top to the bottom of the figure.
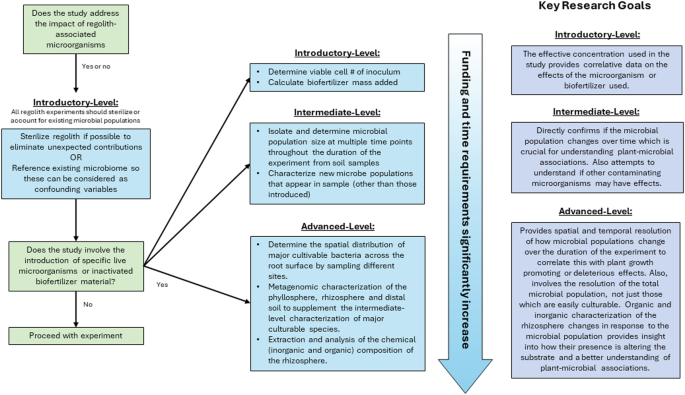
This is a decision tree describing questions and recommendations for designing RBA experiments, with an emphasis on microorganism evaluation. The green boxes (left side) include questions researchers might consider when designing their experiments and choosing their methods. Arrows indicate which recommendations are appropriate, depending on researchers' answers to the questions in the green boxes. The blue boxes (middle of figure) indicate the recommendations that would be suitable for their research. The purple boxes (right side) indicate additional considerations and analyses that might improve the quality of research. The large downward-pointing arrow illustrates how the funding and time requirements of the recommendations listed significantly increase from the top to the bottom of the figure.
Introductory recommendations for regolith
For experimental designs in which the composition and characteristics of regolith is secondary to other aspects (for example needing a sterile medium to examine specific aspects of plant-microbe interactions) it may be appropriate to select or design simulant regoliths that only roughly represent a Martian or lunar composition. For example, a study focusing on the capillary behavior of water at Mars-like gravity would need to adequately mimic porosity and permeability but depending on the particular aspects being examined may not need to exactly mimic mineralogy, thus a greater variety of materials can be feasibly used. A study that is testing the endogenous microbial composition of the common regolith simulants the selection of simulants is not based on the accuracy of the simulant as much as the commonality of its use in the literature.
For the Moon, a highland-like regolith can be roughly represented from crushed anorthositic materials (similar to that applied by Lytvynenko et al. 156 ) while the lunar mare can be reresented with a roughly basaltic composition. Mars, roughly basaltic materials crushed to represent Martian soil are adequate in work where highly constraining the composition is not required. There are commercially available materials that can serve this purpose as well (see resources above) and may be a viable solution where raw materials cannot be obtained or processed. Two existing Martian simulants that fulfill this requirement include the JSC-1a Mars and the Martian Mojave Simulant (MMS) as these may be more available or more affordable. For example, two variations of the MMS (MMS-1 and MMS-2) are available from The Martian Garden ( https://www.themartiangarden.com/ ) and can be a useful resource. However, it is important to have awareness of the limitations of these compared to Mars for example the high alteration that JSC-1a source rock has been exposed to and the lack of important components such as sulfate minerals and other salts in MMS (and its variations). Once selected or designed, it is important to provide detailed description of the design protocol and of any processing (e.g., sterilization) used to prepare the simulant for the experiment and to consider the effects this processing may have on the conclusions that can be drawn from the results. Depending on the questions being addressed, details of any visual or more readily measurable changes to the simulant (i.e., soil pH or EC) is also recommended though what is measured is expected to vary significantly depending on what is being tested and what methods are accessible with available equipment and funding.
Intermediate recommendations for regolith
The middle-level recommendations focus on bulk composition and general physical properties. For research questions where mimicking the bulk composition of the regolith requires greater accuracy, the selection or design of a regolith is an important consideration. For example, a study directly comparing hydroponic vs. regolith-based approaches for different crops would need to accurately reflect the bulk composition and general physical properties of actual regolith.
The specific characteristics that are recommended for a Mid-level approach include bulk chemistry and mineralogy, particle size distribution, and cohesive and compressive strength. These are characteristics for which there is an abundance of data for both the Moon and Mars and methods available to feasibly reproduce at scales relevant to agricultural studies. This can be achieved by designing a simulant from a mixture of appropriate components determined by examining available data 19 , 129 . However, obtaining appropriate materials can have high time and accessibility constraints which make this more difficult, however there are commercially available simulants that adequately represent bulk composition that can also be used 61 . Whether designed or purchased, the simulant needs to be analyzed to evaluate its accuracy and demonstrate that the accuracy is adequate for the particular research questions being addressed. The methods used to analyze the simulant accuracy need to be directly comparable to data available from actual regolith for example elemental oxide composition obtained by XRF or bulk mineralogy from XRD and comparable PSD (ASTM D6913, ASTM E2651, or commercial particle size analyzers) and penetration (ASTM D3441) tests to understand physical properties. Additionally, a standardized method of comparing these results in a way that scores or compares the simulants is recommended, though there is not a currently consistent standard. One approach that could become the standard (and has been applied to a variety of simulants) is the calculator of a figure of merit (FOM) (e.g., Schrader et al. 163 ; Metzger et al. 164 ) 19 , 62 , 129 .
Other mid-level aspects involve methodologies used to examine changes to regolith simulants due to pre-processing or as a result of the experiment itself. Again, the methods recommended here are those that focus on changes to bulk composition and characteristics. For example, a study examining the effects of various sterilization techniques on bulk regolith properties would need to apply appropriate methods for examining bulk changes. Though this would not capture more subtle changes that are discussed in the High-Level recommendations, those changes that can be examined in such bulk methods do provide useful information to address such questions. Similar to the Introduction-Level any pre-processing treatments of the regolith need to be described in detail, additionally the regolith should be analyzed before and after this treatment using methods appropriate for evaluating bulk composition and general physical properties (e.g., XRF, XRD, etc.) with similar recommendations for measuring bulk changes that occur from the experimental conditions.
Advanced recommendations for regolith
High-level recommendations focus on targeted compositions or characteristics. The reproduction and analysis of these characteristics requires greater access to materials, funding, time and expensive instrumentation limiting the accessibility of these methods. However, this level of accuracy is needed to address certain questions. For example, studies that seek to understand the effects of Mars-like salinity and the effectiveness of various ameliorative techniques require mediums that accurately mimic a Mars-like salt profile. However, this requires either designing or significant alteration of currently available simulants which is both time-consuming and expensive. It also represents a characteristic about which there is somewhat limited data making it difficult to truly determine the accuracy of a simulant compared to actual regolith.
For studies that examine a more targeted characteristic of a regolith (rather than bulk chemistry), it is recommended that an appropriate simulant be specifically designed. Depending on the characteristic, it may be possible to adapt commercially available simulants (either by the seller or the purchaser), but though this may be more feasible than developing a new simulant it can still be expensive. In either case, both the targeted characteristic and bulk characteristics need to be appropriately representative, so the mid-level recommendations are still under consideration in addition to these high-level recommendations.
Depending on the experimental approach, it may also be possible to isolate a particular characteristic which may alleviate the need to represent the bulk properties in addition to the targeted property. For example, using a generic potting soil with Mars-like salts introduced which may be more feasible than designing an entire simulant. Whatever the approach, the simulant or simulated conditions need to be analyzed for accuracy and demonstrated to represent that characteristic (i.e., specific analysis that compare simulation to target) as adequately as is feasible at the necessary scale.
The remaining high-level recommendations focus on methods used to analyze changes to the simulant weather from pre-processing treatments or the experiment itself. It is still important to track changes to the bulk composition and so any targeted analysis is recommended to be done in addition to those that evaluate bulk changes. There is a great diversity of techniques that are available to examine targeted characteristics such that it is not possible to determine a certain set of techniques that should be used every time or space to summarize all techniques available. The technique most appropriate will heavily depend on the particular characteristic and the goal of the study in mind. Some examples of these more specialized techniques include electron microscopy (EM) and computed tomography (CT) which allow for 3-D imaging of the changes to the rhizosphere soil characteristics compared to bulk soil (Gregory and Hinsinger 1999). This can range from relatively simple and accessible EM techniques such as Scanning EM (SEM) or Transmission EM (TEM) to much more expensive and difficult to access approaches such as Focused-ion-beam SEM (FIB-SEM). Structural changes, especially those in highly disordered phases common to soils, can also be examined through a variety of X-ray synchrotron techniques (which are extremely expensive and specialized). It is recommended that care be taken to select techniques appropriate to the research questions being addressed by a thorough examination of the literature and consultation with other researchers especially when such expertise is not directly available within the research group attempting the study and applied to samples before and after preprocessing or experimental treatments.
Measuring plant response to regolith-based substrates
Plants grown in regolith or regolith simulant have complex morphological, physiological, and biochemical responses. In order to evaluate the effects of regolith simulant on these responses, researchers must carefully manage the plant growth environment (air, water, nutrients, and other conditions) to make accurate causal inferences about RBA. More detailed recommendations specific to the environment are provided below, but it is also emphasized here due to the significant effects environment has on plant response and the importance of plant response as a measure of the viability of an off-world agriculture system.
In addition to the growth environment, many other factors such as plant genetics, associated microbiome, variation in simulant characteristics can influence plant response. Replications, especially over time, can also help to minimize the influence of these effects when analyzing plant response variables. In turn, as much as plants are affected by the variables, they also affect the variables including the regolith and microbiome. Additionally, techniques used to amend regolith simulants in the attempt to improve plant response or yield will change the nature of the simulant in ways that are important to quantify. Potential alterations must be considered when designing regolith simulant experiments. For example, as mentioned in the key questions above, research must be done to understand how plant roots and root exudates may cause “weathering” of regolith samples, to understand how fertilizer amendments impact the chemical and microbial properties of regolith, and to assess how other management practices affect the regolith simulant and microbiome (Table 3 ).
Introductory recommendations for plants
Many plant morphological and physical responses must be measured to get a holistic perspective on the effect of regolith, for any regolith simulant experiment. Plant species and cultivar should be reported, as well as the seed source (including company, location, and date). At the beginning of the experiment, germination success rates (expressed as a percentage) should be evaluated, and researchers should observe whether these seedlings are morphologically different from expected/normal. As they grow and develop, these plants should have measurements taken to quantify plant growth, such as plant height or average crown diameter (cm). At the end of the growth period, researchers should also measure the total mass of the resulting plants. Researchers should evaluate shoot fresh mass (SFM) and root fresh mass (RFM) (if it is possible to clean the roots of substrate), and after drying the samples, they should also measure shoot dry mass (SDM) and root dry mass (RDM).
If plant development includes reproductive stages (flowering, fruiting), this should be reported in detail. Important data include approximate growth period (days or weeks) before flower development was observed, average number of flowers and fruits per plant, size of fruit (length and diameter) and fruit mass (g). Observations should be recorded about whether fruits and flowers developed normally or abnormally compared to what is expected for the species or cultivar.
Intermediate recommendations for plants
Plant species, seed source, germination rate (%), and plant growth measurements (plant height, crown diameter, SFM, RFM, SDM, RDM) should be reported, as listed in the “basic-level” recommendations. Plant leaf area (cm 2 ) should be measured using a leaf area meter, leaf scanner, image processing software, or another tool. To evaluate plant physiology, stomatal conductance (mmol m −2 s −1 ) should be evaluated, especially if there is an a posteriori phytohormonal analysis of the samples (e.g., stomatal conductance with leaf abscisic acid concentrations). Stomatal conductance describes the rate of carbon dioxide entering or water vapor exiting through the stomata of a leaf, and depends greatly on carbon dioxide concentration and vapor pressure deficit. Leaf area (cm 2 ) and shoot dry mass can be used to calculate shoot leaf area, which quantifies the average leaf thickness per plant.
If reproductive stages are observed, detailed data should be collected (as described above) for growth period (days or weeks) before flower development, average numbers of flowers and fruits per plant, size of fruit (length and diameter), fruit mass (g), and flower/fruit morphology should be visually assessed.
Advanced recommendations for plants
Plant variables should be evaluated as described in the “mid-level” recommendations. Plant growth measurements (plant height, crown diameter) should be taken at regular intervals throughout the growth period, and measurements at harvest should include leaf area (cm 2 ), stomatal conductance (mmol m −2 s −1 ), SFM, RFM, SDM, and RDM. Additional measurements should evaluate chlorophyll content and relevant secondary plant metabolites, like anthocyanin content. This can be done with a SPAD leaf sensor or with tissue compositional analysis.
If reproductive development is observed, data should be collected as described in the “basic” and “mid-level” recommendations. Additional postharvest measurements should be collected for edible fruiting crops. Alongside fresh weight, fruit size (length and diameter), and average fruit number per plant, tissue should be tested for BRIX (sugar content) and anthocyanin content. Other species-specific fruit quality measurements should be taken, including nutritional content for key nutrients such as vitamin C (though key nutrients vary per species) 165 and capsaicin content (to quantify spiciness for crops like peppers). It is encouraged to test germination percent of any seeds that are produced.
Identifying the microbial community and monitoring response
Microbial populations shape and are shaped by soil across our planet. Their near ubiquitous distribution stands in stark contrast to the Martian or lunar surfaces which are likely devoid of life, or if it does exist, persists in only rarified locations. As all regolith simulants are terrestrially derived, they are all inaccurate from a microbiological perspective as they almost certainly contain an associated population of microscopic bacteria, eukaryotes, and archaea. The aseptic preparation and packaging of these simulants at production sites is unreasonable from a logistics perspective. Sterile procedures are also not reasonable from the perspective of most plant growth projects. Indeed sustainable agriculture in regolith systems will almost certainly depend on the incorporation of specific microbial cohorts to leverage the benefits described earlier in this text (e.g., nitrogen fixation, bioweathering, etc.). Therefore, a gnotobiotic, or knowing the identity of all microorganisms within the regolith, with an aim towards microbial management, is a more reasonable approach than a sterile one.
However, other than an early evaluation of JSC-1A, little information on the microbiomes of either lunar or Martian regolith simulants are available. This represents an obvious area of concern as the presence of specific microbial cohorts, either pathogens or mutualists, would significantly impact any plant growth studies performed in these simulants. Moreover, given the aforementioned potential for microorganisms to transform regolith, the endogenous microbial community of these simulants could continue to alter composition post-acquisition depending on the mechanism of storage. This lack of information regarding the starting microbiome composition represents a significant knowledge gap which needs to be addressed for research of plant growth in regolith . Addressing this concern at the very least should be part of the basic microbial considerations of any ongoing plant growth research. Here we will outline specific experimental considerations that may prove useful for both small as well as large scale studies. The former can often be performed in magenta boxes under sterile conditions, while the latter are often open air and therefore susceptible to contamination by the microbiome of the environment. In the case of a closed loop habitat like a lunar or Martian colony this will likely be endophytes from other plants as well as the endogenous microbiome of the colonists, or any other animals present.
Here we discuss several best practices for including microbes into in situ resource utilization studies with lunar and Martian regolith simulants. As above, we have attempted to categorize these practices with the goal of balancing encouragement of new regolith biology research while providing increased rigor to experimental design and data collection. In the process of developing these best practices, specific research questions which need to be addressed by the regolith research community for further progress in this area emerged and have also been included here (See above section “Key Questions and Knowledge Gaps”).
Introductory recommendations for microbiome
The vast majority of the current plant growth studies in regolith simulants pay little attention to the existing microbiome of the simulant, or consider the use of microbial partners for improving growth. However, leveraging the advantages provided by the incorporation of microbial partners is a research area we predict will experience significant growth over the next few years. Given this likelihood, we propose some simple parameters to eliminate or define the existing microbiome as well as minimum data that should be reported during inoculation studies. As the potential impact of the microbiome on plant growth and development is crucial, even studies not focused on the use of microbial partners should consider these parameters in their experimental design.
For small-scale studies, to be performed aseptically, such as in a Magenta box or other culture vessel, substrates should be sterilized by autoclave or other approved source that will limit changes to simulant mineralogy.
Studies incorporating fungi or bacteria need to include an accepted measure of the inoculum size, or the number of viable units being added. This can include colony-forming units in the case of non-filamentous microorganisms, or total chlorophyll as an approximation for filamentous cyanobacteria such as A. cylindrica 166 . Methods for such inoculations are readily available, and Heydarian et al. is a good example of this 167 .
As an alternative to the use of live microorganisms, microbe-derived biomass (algae, cyanobacteria, etc.) can be employed as a biofertilizing agent. Methods for calculating the dried biomass should be used to characterize how the simulant has been amended as described by ref. 168 .
For large-scale studies, sterilization of all regolith may not be feasible, therefore an accounting of the major species of the microbiome should be referenced if one is available. The lack of such a reference is a notable knowledge gap in the current research .
Intermediate recommendations for microbiome
In contrast to the introductory parameters, our mid-level protocols are intended to address studies focused on the direct investigation of plant-microbial associations. This can be through the use of the existing regolith simulant microbiome, or the intentional introduction of one or more defined species of microorganisms for gnotobiotic studies. This level builds on the introductory parameters, meaning that the microbiome of all simulants should be defined or eliminated by sterilization. At this level, there is no significant distinction between the best practices of small- and large-scale studies; only the sampling size between the two scales should be a consideration.
Observing relative densities of the major cultivable microbes along the rhizosphere between regolith grown samples and controls. A viable procedure for this has been described by ref. 169 . Such assays would need to be performed at LEAST in triplicate for each treatment type.
Characterizing the major cultivable microorganisms as potential plant growth promoting pathogens through plate-based methods, i.e., selective culture media. A summary of viable plate-based methods for the identification of plant growth promoting bacteria (PGPB) is available in ref. 135 . This should be followed with inoculations of plant seeds to confirm PGP activity.
Advanced recommendations for microbiome
Studies at this level move beyond simply identifying the most common inhabitants of the microbiome as well as their potential to improve plant growth, as defined by the mid-level parameters. Rather, high-level studies focus on understanding the temporal and spatial changes both microbiological as well as chemical within the rhizosphere or phyllosphere, the site of plant-microbial associations. Such studies are at the cutting edge of the plant research more broadly and their incorporation into regolith would be evidence that this area of research has indeed “arrived”. At this level, there is no significant distinction between the best practices of small- and large-scale studies; only the sampling size between the two scales should be a consideration.
Determining the spatial distribution of major cultivable bacteria across the root surface as well as distally in the regolith sample should be determined using procedures like those of ref. 169 .
Metagenomic characterization of the phyllosphere, rhizosphere and distal soil using established methods and primers. Common primers for metabarcoding include 16S (prokaryotes and archaea), 18S (protists), and ITS (fungi). Regolith chemical composition is likely to complicate isolation of DNA and an optimized approach needs to be developed. Electrophoretic approaches and isolation methods associated with iron rich substrates may be of particular assistance.
Extraction and analysis of the chemical composition of the rhizosphere. As with DNA extraction, such isolation is likely to be limited by regolith composition and will need to be isolated.
Performing KEGG metabolic network analysis based on identified rhizosphere metabolites to determine potential contributions to plant growth and long-term impacts on soil health.
Recommendations for designing and tracking variables in the growth environment
The growth environment has a significant effect on plant response, microbiome characteristics, and regolith alteration. In order to evaluate the effects of the experimental variables on the intended samples and separate them from the effects of non-experimental or unintentional variables, researchers must carefully manage the plant growth environment (air, water, nutrients, and other conditions) to make accurate causal inferences about RBA. If these tools, facilities, or methods are not within the scope of an experiment, we strongly recommend collaboration with other researchers. To minimize confounding variables, regolith-plant experiments should be done in a temperature-controlled greenhouse or controlled environment growth chamber. Controlled environment (CE) growth chambers are ideal because of their ability to maintain careful setpoints and their flexibility for a variety of environmental conditions. Additionally, replications over space and time can reduce variability in plant experiments, and should be used for regolith simulant experiments even when regolith simulant volume is limited. Ideally, one CE growth chamber would be treated as a replicate, and experiments would be replicated between several growth chambers. Less costly alternatives to this include repeating an experiment over time in one growth chamber, or transferring plants between CE growth chambers to minimize confounding environmental effects (Table 4 ).
Introductory recommendations for growth environment
These guidelines express the minimum frequency at which data should be taken. Dry bulb temperature (degrees Celsius) should be measured at least twice per day–once during the light period, once during the dark period–using a temperature sensor that is shielded from light and moisture and placed at canopy-level and control within limits, 10 degree C fluctuations. Atmospheric moisture should be measured as percent relative humidity (RH%) and controlled within limits, and data should be collected at the same frequency as dry bulb temperature. Carbon dioxide (CO 2 ) substantially affects plant growth and development, and although many plant growth chambers do not include CO 2 -controlling equipment, it is recommended that a CO 2 sensor be installed, and CO 2 concentration should be measured hourly throughout the growth period. Air velocity (m s −1 ) should be measured at the canopy level at least once during the experiment. Watering methods should be carefully detailed in the resulting publication, including the water delivery method (over top, subirrigation, etc.), watering frequency and amount, and pH/EC. If nutrients are applied, these should be measured in mg L −1 of liquid and total mg per plant if granular. Frequency of application should be described, and if liquid, pH of nutrient solution should be reported 165 .
Lighting also greatly affects plant growth and development, therefore at a minimum, light duration (photoperiod, hours per 24 h) and light intensity should be measured. Light intensity is expressed as Photosynthetic Photon Flux Density, PPFD (μmol m −2 s −1 ), where several measurements are taken at canopy height, to find the average light intensity of the growing area. Vertical light gradients depend on many factors, such as chamber size, lamp type, and lamp distribution, so light intensity data should be recorded at the beginning of the experiment and periodically as the plants grow taller. The frequency of this data collection depends on the rate of shoot elongation and the duration of the experiment, but at minimum this data should be collected from sensors placed at the lowest and tallest average plant height. When plants are grown at a greater distance from the lights, there is a less severe vertical light intensity gradient, which results in a more uniform growing environment (i.e., growing plants several meters’ distance from the light source is preferable compared to growing plants only a few dozen centimeters from the light source). Furthermore, when light fixtures are placed close to the plant canopy, this causes greater environmental variation between the place directly underneath the light fixture and the area surrounding this place. These factors should be taken into consideration during experimental design, and therefore accurate data must be reported in the resulting publication(s). It is recommended that authors include data for the distance (cm) between the tallest plants and the light source, and the standard deviation between PPFD readings collected from points across the growing area.
Environmental variables should also include any other pre-treatment of regolith simulant, including whether it was sterilized (and the sterilization method used), the amount of simulant used in each sample (volume and mass), and any nutrients or other amendments added to account for post-sterilization leaching.
Sensor calibration is key to ensuring accurate environmental measurements, and whenever possible, several sensors should be cross-checked to ensure they produce reasonably similar results (especially for light sensors). Sensors should be calibrated regularly, according to the manufacturer’s procedures and recommended frequency. Other useful reporting guidelines include those developed by the NCERA-101 Committee on Controlled Environment Technology and Use: minimum guidelines for greenhouses, and minimum guidelines for growth rooms and chambers, and the guidelines for greenhouses in ref. 170 . Other useful resources, such as Katagiri provides recommendations for building a more affordable, high-quality custom-built growth chamber 171 .
Intermediate recommendations for growth environment
The basis of these environmental variables is similar to those outlined above, in the “basic-level” recommendations, and greater frequency is encouraged. Dry bulb temperature (degrees Celsius) and relative humidity (%RH) should be measured several times during the light and dark period. We recommend that researchers use relative humidity and dry bulb temperature to calculate vapor pressure deficit (VPD, in kPa) within the publication. Carbon dioxide concentration (μmol mol −1 ) should be measured hourly, at a minimum. Air velocity (m s −1 ) should be measured at the canopy-level once daily. Watering methods should be reported as outlined in the “basic-level” recommendations, with some additions. Before any fertilizer is added, water type (feed water, deionized water, Ultrapure water) and its pH and EC should be reported, and water quality testing is recommended. Additionally, leachate should be collected and evaluated for pH and EC at the beginning and end of the growth period. Nutrients applied should be reported as described above, and any pre-treatment of regolith simulant should also be described in detail.
Lighting should be evaluated and reported including photoperiod (h), light intensity over time, and light quality. Light intensity should be expressed as PPFD (μmol m −2 s −1 ), and this data should be collected at canopy level as the plant grows, at least four times throughout the plant growth period, or more often if the plant height is rapidly increasing. Light quality is described as the spectrum of wavelengths emitted by the light source, for all wavelengths in the photosynthetically active radiation range (between 400 and 700 nm). It is expressed as a spectral graph, showing the PPFD of each wavelength. This data is often listed in the product details for the light bulb/diode array, and can also be measured by a spectroradiometer.
Advanced recommendations for growth environment
At this level, similar morphological and physiological observations should be made as are listed above, though with an increased frequency and with some additional variables. Dry bulb temperature (degrees Celsius) and relative humidity (RH%) should be measured at least every 10 min, and we recommend that researchers use these values to calculate vapor pressure deficit (VPD, in kPa) within the publication. Carbon dioxide concentration (μmol mol −1 ) should be measured hourly at a minimum, and air velocity (m s −1 ) should be measured once daily. Watering methods should be reported, and water quality tests should be conducted on the water before fertilizers are added. Researchers should report the nutrients applied (mg L −1 if liquid, total mg per macronutrient/micronutrient per plant if granular) and frequency of application. It is recommended that leachate be collected over time and repeatedly tested for pH and EC. To quantify how much nitrogen (a key macronutrient for plant growth) is retained in the substrate, we recommend that researchers measure the volume of leachate produced by each plant and use this to calculate the amount of nitrogen each plant receives. This also will indicate how much water is available to the plant and will aid in understanding the root environment.
Light reporting should include photoperiod (h), light intensity over time (μmol m −2 s −1 , at least four times over the growth period), and light quality (expressed as a spectral graph). Any other light treatments should be described in detail (such as end-of-day far-red radiation and night interruption), including the spectrum, duration, and time of application.
Applying the recommendations
The central focus of what has been discussed thus far has been the suggestion of primary models from which current or future investigators in the RBA field can use not only to be able to generate standardized datasets but also as a tool with which they will build their experimental designs based on their limitations, strengths and potential to collaborate with other researchers.
The three decision trees (regolith, bacteria, plant) represented in this section create straightforward paths for prospective authors to develop their experimental designs welcoming the standardized tiered system suggested in section 5.0. Each tree serves as a starting point for researchers that can rely on simple questions to shape their choices as they continue to develop an already functional design to have a more in-depth look into some of the other categories or start a new one.
Each tree functions on its own as part of what can be a fully integrated system study that would encompass all the main research foci that compose an RBA study.
Reiterations and conclusions
The success of future off-world colonies depends, at least in part, on safe and sustainable food production. Regolith-based agriculture draws on centuries of humanity’s experience in plant cultivation to make this future possible, while minimizing the costs as well as other challenges associated with the establishment of an off-world colony. Furthermore, RBA leverages the millennia of coevolution between plants and microorganisms to improve nutrient acquisition, reduce stress, and improve disease resistance. However, RBA faces numerous obstacles to implementation ranging from the geological to the biological. Questions and challenges such as which simulants to use and why or the viability of specific plant and/or microbial systems are crucial to the development of bioregenerative life support systems which will be vital to the success of these future missions. From our review of the rich history of regolith biology and geology here in these questions and challenges became clearer and we have summarized them here. These questions are akin to mile markers which must be passed on the road to sustainable plant growth in these challenging environments.
If we extend our analogy just a little, it will be exceedingly difficult to pass these collective mile-markers if we are all traveling on different roads. Indeed, our review also revealed the frequently disparate research and reporting practices within the community of regolith researchers. This is not at all surprising given the varied backgrounds of the community as well as the general lack of any authoritative agency or organization to fund and/or direct research in this area. Our proposed best practices are an attempt to rectify the current lack of oversight and provide a framework for consistent experimentation and reporting of results to accelerate all our research efforts. Finally, it is our sincerest hope that these recommendations will drive new collaborations, provide opportunities for new researchers to make substantiative contributions, and generally improve the connectivity of this community of researchers as we contribute to the development of crucial infrastructure for future off-world colonies.
Schwartzkopf, S. H. Design of a controlled ecological life support system. Bioscience 42 , 526–535 (1992).
Article CAS Google Scholar
Berkovich, Y. A. Evaluation of planting surfaces for crop production in microgravity. Adv. Space Res. 26 , 271–279 (2000).
Article Google Scholar
Cooper, M., Douglas, G. & Perchonok, M. Developing the NASA food system for long-duration missions. J. Food Sci. 76 , R40–R48 (2011).
Massa, G., Emmerich, J., Morrow, R., Bourget, C. & Mitchell, C. Plant-growth lighting for space life support: a review. Gravit. Space Biol. Bull. 19 , 19–30 (2006).
Google Scholar
Perchonok, M. & Bourland, C. NASA food systems: past, present, and future. Nutrition 18 , 913–920 (2002).
Perchonok, M. H., Cooper, M. R. & Catauro, P. M. Mission to Mars: food production and processing for the final frontier. Annu. Rev. Food Sci. Technol. 3 , 311–330 (2012).
Tibbitts, T. W., Wheeler, R. M., Mitchell, C. A. & Heidmann, J. Life sciences: space life support systems and the lunar farside crater Saha proposal. Adv. Space Res. 26 , 245–377 (2000).
Zwart, S. R., Kloeris, V. L., Perchonok, M., Braby, L. & Smith, S. M. Assessment of Nutrient Stability in Foods from the Space Food System After Long‐Duration Spaceflight on the ISS. J. Food Sci. 74 , H209–H217 (2009).
Zabel, P., Bamsey, M., Schubert, D. & Tajmar, M. Review and analysis of over 40 years of space plant growth systems. Life Sci. Space Res. 10 , 1–16 (2016).
Eichler, A. et al. Challenging the agricultural viability of Martian regolith simulants. Icarus 354 , 114022 (2021).
Crites, S. T. & Lucey, P. G. Revised mineral and Mg maps of the Moon from integrating results from the Lunar Prospector neutron and gamma-ray spectrometers with Clementine spectroscopy. Am. Mineral. 100 , 973–982 (2015).
Ehlmann, B. L. & Edwards, C. S. Mineralogy of the Martian surface. Annu. Rev. Earth Planet. Sci. 42 , 291–315 (2014).
Heiken G. H., McKay D. S., and Fruland R. M. Apollo 16 soils – grain size analysis and petrography. In Proc. 4th Lunar Science Conference 251–266 (1973).
Muirhead, B. K., Nicholas, A. K., Umland, J., Sutherland, O. & Vijendran, S. Mars sample return campaign concept status. Acta Astronaut. 176 , 131–138 (2020).
McKay, D. S., et al. Lunar Sourcebook: A User’s Guide to the Moon. The Lunar Regolith (Cambridge University Press, 1991).
McKay, C. P., Imre Friedman, E., Wharton, R. A. & Davies, W. L. History of water on Mars: a biological perspective. Adv. Space Res. 12 , 231–238 (1992).
McSween, H. Y., Jeffrey Taylor, G. & Wyatt, M. B. Elemental composition of the Martian crust. Science 324 , 736–739 (2009).
Chevrier, V. & Mathé, P. E. Mineralogy and evolution of the surface of Mars: a review. Planet. Space Sci. 55 , 289–314 (2007).
Fackrell, L. E., Schroeder, P. A., Thompson, A., Stockstill-Cahill, K. & Hibbitts, C. A. Development of Martian regolith and bedrock simulants: potential and limitations of Martian regolith as an in situ resource. Icarus 354 , 114055 (2021).
Richter, D. D. et al. Soil production and the soil geomorphology legacy of Grove Karl Gilbert. Soil Sci. Soc. Am. J. 84 , 1–20 (2020).
Certini, G. et al. Disambiguating the soils of Mars. Planet. Space Sci. 186 , 104922 (2020).
Duri, L. G. et al. The potential for Lunar and Martian regolith simulants to sustain plant growth: a multidisciplinary overview. Front. Astron. Space Sci. 8 (2022).
Spudis, P. & Pieters, P. Lunar Sourcebook: A User’s Guide to the Moon. Global and Regional Data about the Moon, p. 595–632 (Cambridge University Press, 1991).
Taylor, J. et al. Modal analyses of lunar soils by quantitative X-ray diffraction analysis. Geochim. Cosmochim. Acta 266 , 17–28 (2019).
Ohtake, M. et al. The global distribution of pure anorthosite on the Moon. Nature 461 , 236–240 (2009).
Taylor, G. J., et al. Lunar Sourcebook: A User’s Guide to the Moon. Lunar Rocks . (Cambridge University Press, 1991).
Labotka T. C., Kempa M. J., White C., Papike J. J. & Laul J. C. The lunar regolith: comparative petrology of the Apollo sites. In Proc. 11th Lunar and Planetary Science Conference, 11th, Houston, TX, March 17-21, 1980, Proceedings. Volume 2 (A82-22296 09-91) New York, Pergamon Press, p. 1285-1305. (Vol. 11, pp. 1285-1305).
Simon S. B., Papike J. J. & Laul J. C. The lunar regolith: Comparative studies of the Apollo and Luna sites. In Proc. 12th Lunar and Planetary Science Conference 12th, Houston, TX, March 16-20, 1981, Proceedings. Section 1. (A82-31677 15-91) Vol. 12, pp. 371–388 (New York and Oxford, Pergamon Press, 1982).
Jolliff, B. J., Gillis, J. J., Haskin, L. A., Korotev, R. L. & Wieczorek, M. A. Major lunar crustal terranes: Surface expressions and crust-mantle origins. J. Geophys. Res. Planets 105 , 4197–4216 (2000).
Haskin, L. & Warren, P. Lunar Chemistry. 357–474 (1991).
Pasek, M. A. Phosphorus as a lunar volatile. Icarus 255 , 18–23 (2015).
Taylor, L. A., Pieters, C. M., Keller, L. P., Morris, R. V. & McKay, D. S. Lunar Mare Soils: Space weathering and the major effects of surface-correlated nanophase Fe. J. Geophys. Res. 106 , 985–999 (2001).
Housley, R. M., Grant, R. W., and Paton, N. E. Origin and characteristics of excess Fe metal in lunar glass welded aggregates. Proc. 4th Lunar and Planetary Science Conference , 2737–2749 (1973).
Morris, R. V. The surface exposure (mature) of lunar soils: Some concepts and Is/FeO compilation. In Proc. 9th Lunar and Planetary Science Conference , 9th, Houston, Tex., March 13-17, 1978, Proceedings. Volume 2. (A79-39176 16-91) Vol. 9, pp. 2287–2297 (New York, Pergamon Press, Inc., 1978).
McKay D. S., Morrison D. A., Clanton U. S., Ladle G. H. & Lindsay J. Apollo 12 soil and breccias. In Proc. Second Lunar and Planetary Science Conference , vol. 2, p. 755–774 (1971).
Morris, R. V. Surface exposure indices of lunar soils: a comparative FRM study. In Proc. 7th Lunar and Planetary Science Conference , Houston, Tex., March 15-19, 1976, Proceedings. Volume 1. (A77-34651 15-91), Vol. 7, p. 315–335 (New York, Pergamon Press, Inc., 1976).
Ming, D. W. & Henninger, D. L. Use of lunar regolith as a substrate for plant growth. Adv. Space Res. 14 , 435–443 (1994).
Long-Fox, J., Perman, J., Landsman, Z., Metke, A., and Britt, D. T. Investigating the effects of composition on granular surface area: gas sorption analyses of high-fidelity lunar highlands and carbonaceous chondrite asteroid regolith simulants. In Proc. 53rd Lunar and Planetary Science Conference (2022).
Graf, J. C. Lunar soils grain size catalog. NASA Reference Publication 1265 (1993).
Carrier, D. W., Bromwell, L. G. & Martin, R. T. Strength and compressibility of returned lunar soil. Geochim. Cosmochim. Acta 3 , 3223–3234 (1972).
Mitchell, J. K., Houston, W. N., Carrier, W. D. III & Costes, N. C. Apollo soil mechanics experiment S-200 final report”, NASA Contract NAS 9–11266. Space Sci. Lab. Ser. 15 (University of California, Berkeley, 1974).
Ming, D. W. & Morris, R. V. Chemical, Mineralogical, and Physical Properties of Martian Dust and Soil (Lunar and Planetary Institute, 2017).
Ming, D. W. et al. Geochemical properties of rocks and soils in Gusev Crater, Mars: results of the alpha particle X-ray spectrometer from Cumberland ridge to home plate. J. Geophys. Res. E Planets 113 (2008).
Murchie, S. L. et al. A synthesis of Martian aqueous mineralogy after 1 Mars year of observations from the Mars Reconnaissance Orbiter. J. Geophys. Res. E Planets 114 , 1–30 (2009).
Viviano-Beck, C. E. et al. Revised CRISM spectral parameters and summary products based on the currently detected mineral diversity on Mars. J. Geophys. Res. Planets 119 , 1403–1431 (2014).
Yen, A. S., et al. Evidence for a global Martian soil composition extends to Gale Crater. In Proc. Lunar and Planetary Science Conference , XLIV2013, 44 (2013).
Yen, A. S. et al. An integrated view of the chemistry and mineralogy of martian soils. Nature 436 , 49–54 (2005).
Achilles, C. N. et al. Amorphous phase characterization through X-ray diffraction profile modeling: implications for amorphous phases in gale crater rocks and soils. In Proc. 49th Lunar and Planetary Science Conference p. 2661 (2018).
Achilles, C. N. et al. Mineralogy of an active eolian sediment from the Namib dune, Gale Crater, Mars. J. Geophys. Res. Planets 122 , 2344–2361 (2017).
Bish, D. L. X-ray diffraction results from Mars. Science. 341 , 1–6 (2013).
Blake, D. F. et al. Curiosity at Gale Crater, Mars: characterization and analysis of the rocknest sand shadow. Science 341 , 1239505 (2013).
Rampe, E. B. et al. Mineralogy of an ancient lacustrine mudstone succession from the Murray formation, Gale crater, Mars. Earth Planet. Sci. Lett. 471 , 172–185 (2017).
Rampe, E. B. et al. Mineral trends in early Hesperian lacustrine mudstone at Gale Crater, Mars. In Proc. Lunar and Planetary Science Conference (2017).
Smith, R. J., Rampe, E. B., Horgan, B. H. N. & Dehouck, E. Deriving amorphous component abundance and composition of rocks and sediments on Earth and Mars. J. Geophys. Res. Planets 123 , 2485–2505 (2018).
Dehouck, E., Mclennan, S. M., Sklute, E. C. & Dyar, M. D. Stability and fate of ferrihydrite during episodes of water/rock interactions in early Mars: an experimental approach. J. Geophys. Res. Planets 122 , 358–382 (2017).
Dehouck, E., Mclennan, S. M., Meslin, P.-Y. & Cousin, A. Constraints on abundance, composition, and nature of X-ray amorphous components of soils and rocks at Gale crater, Mars. J. Geophys. Res. Planets 119 , 2640–2657 (2014).
Jeute, T. J., Baker, L. L., Abidin, Z., Bishop, J. L. & Rampe, E. B. Characterizing nanophase materials on Mars: Spectroscopic studies of allophane and imogolite. in Lunar and Planetary Science XLVIII (2017).
Morris, R. V. et al. Chemical composition of crystalline, smectite, and amorphous components for Rocknest soil and John Klein and Cumberland mudstone drill fines using APXS, CheMin, and SAM datasets from Gale Crater Mars. In Proc. 45th Lunar and Planetary Science Conference 1319 (2014).
Squyres, S. W. et al. Detection of silica-rich deposits on Mars. Science 320 , 1063–1067 (2008).
Thompson, A., Rancourt, D. G., Chadwick, O. A. & Jon, C. D. Iron solid-phase differentiation along a redox gradient in basaltic soils. Geochim. Cosmochim. Acta 75 , 119–133 (2011).
Cannon, K. M., Britt, D. T., Smith, T. M., Fritsche, R. F. & Batcheldor, D. Mars global simulant MGS-1: a Rocknest-based open standard for basaltic martian regolith simulants. Icarus 317 , 470–478 (2019).
Fackrell, L. E. Applications of Critical Zone Science in the Exploration of Mars. (University of Georgia, 2021).
Schuerger, A. C., Ming, D. W., Newsom, H. E., Ferl, R. J. & McKay, C. P. Near-term lander experiments for growing plants on Mars: requirements for information on chemical and physical properties of Mars regolith. Life Support Biosphere Sci. 8 , 137–147 (2002).
Wamelink, G. W. W., Frissel, J. Y., Krijnen, W. H. J., Verwoert, M. R. & Goedhart, P. W. Can plants grow on Mars and the Moon: a growth experiment on mars and moon soil simulants. PLOS One. 9 , e103138 (2014).
Hecht, M. H. et al. Detection of perchlorate and the soluble chemistry of Martian soil at the Phoenix Lander Site. Science 325 , 64–67 (2009).
Kounaves, S. P. et al. Identification of the perchlorate parent salts at the Phoenix Mars landing site and possible implications. Icarus 232 , 226–231 (2014).
Toner, J. D., Catling, D. C. & Light, B. Soluble salts at the Phoenix lander site, mars: a reanalysis of the wet chemistry laboratory data. Geochim. Cosmochim. Acta 136 , 142–168 (2014).
Archer, P. D. Jr et al. Abundances and implications of volatile-bearing species from evolved gas analysis of the Rocknest aeolian deposit, Gale Crater, Mars. In Proc. 49th Lunar and Planetary Science Conference 2018 (LPI Contrib. No. 2083) 119 , 2806 (2014).
Kounaves, S. P. et al. Soluble sulfate in the Martian soil at the Phoenix landing site. Geophys. Res. Lett. 37 , 1–5 (2010).
Cull, S. C. et al. Concentrated perchlorate at the Mars Phoenix landing site: evidence for thin film liquid water on Mars. Geophys. Res. Lett . 37 (2010).
Galvin, D. P. Evidence for perchlorates and the origin of chlorinated hydrocarbons detected by SAM at the Rocknest aeolian deposit Gale Crater. J. Geophys. Res. Planets 118 , 1955–1973 (2013).
Hanley, J., Chevrier, V. F., Berget, D. J. & Adams, R. D. Chlorate salts and solutions on Mars. Geophys. Res. Lett . 39 , https://doi.org/10.1029/2012GL051239 (2012).
Visscher, A. M. et al. Growth performance and root transcriptome remodeling of arabidopsis in response to Mars-like levels of magnesium sulfate. PLOS One. 5 , e12348 (2010).
Oze, C. et al. Perchlorate and agriculture on Mars. Soil Syst. 5 , 37 (2021).
Hamissou, M. Selected physiological and molecular responses of Arabidopsis thaliana and Nicotiana tobacum plants irrigated with perhclorate-containing water. Asian J. Plant Sci. 10 , 255–262 (2011).
Calderón, R. et al. A review of perchlorate (ClO4−) occurrence in fruits and vegetables. Environ. Monit. Assess. 189 , 82 (2017).
Davila, A. F., Willson, D., Coates, J. D. & McKay, C. P. Perchlorate on Mars: a chemical hazard and a resource for humans. Int. J. Astrobiology 12 , 321–325 (2013).
Sullivan, R., Anderson, R., Biesiadecki, J., Bond, T. & Stewart, H. Cohesions, friction angles, and other physical properties of Martian regolith from Mars Exploration Rover wheel trenches and wheel scuffs. J. Geophys. Res. E: Planets 116 (2011).
Herkenhoff, K. E. et al. Textures of the soils and rocks at Gusev Crater from Spirit’s microscopic imager. Science 305 , 824–826 (2004).
Spohn, T. et al. The InSight-HP3 mole on Mars: lessons learned from attempts to penetrate to depth in the Martian soil. Adv. Space Res. 69 , 3140–3163 (2022).
Golombek, M. et al. Geology of the InSight landing site on Mars. Nat. Commun. 11 , 1–11 (2020).
Keane, J. T. InSight’s first look inside Mars. Nat. Geosci. 13 , 182 (2020).
McCollom, T. M., Donaldson, C., Moskowitz, B., Berquó, T. S. & Hynek, B. Phosphorous immobility during formation of the layered sulfate deposits of the burns formation at Meridiani Planum. J. Geophys. Res. Planets 123 , 1230–1254 (2018).
Treiman, A. H. et al. Mineralogy, provenance, and diagenesis of a potassic basaltic sandstone on Mars: CheMin X-ray diffraction of the Windjana sample (Kimberley area, Gale Crater). J. Geophys. Res. Planets 121 , 75–106 (2016).
Clauwaert, P. et al. Nitrogen cycling in Bioregenerative Life Support Systems: Challenges for waste refinery and food production processes. Prog. Aerosp. Sci. 91 , 87–98 (2017).
Stern, J. C. et al. Evidence for indigenous nitrogen in sedimentary and aeolian deposits from the Curiosity rover investigations at Gale Crater, Mars. Proc. Natl. Acad. Sci. USA 112 , 4245–4250 (2015).
Gasda, P. J. et al. In situ detection of boron by ChemCam on Mars. Geophys. Res. Lett. 44 , 8739–8748 (2017).
Smith, M. L., Claire, M. W., Catling, D. C. & Zahnle, K. J. The formation of sulfate, nitrate and perchlorate salts in the Martian atmosphere. Icarus 231 , 51–64 (2014).
Long-Fox, J. M. and Britt, D. T. Characterization of planetary regolith simulants for the research and development of space resource technologies. Front. Space Technol. 4 , https://doi.org/10.3389/frspt.2023.1255535 (2023).
Long-Fox, J. M., Landsman, Z. A., Easter, P. B., Millwater, C. A. & Britt, D. T. Geomechanical properties of lunar regolith simulants LHS-1 and LMS-1. Adv. Space Res. 71 , 5400–5412 (2023).
Sibille, L., Carpenter, P., Schlagheck, R., French, R. A. Lunar Regolith Simulant Materials: Recommendations for Standardization, Production, and Usage. Marshall Space Flight Center Technical Publication (2006).
Edmunson, J., McLemore, C. A. & Rickman, D. L. Appropriate simulants are a requirement for Mars surface technology. Concepts Approaches Mars Explor. 1679 , 2–3 (2012).
Clark, J. V. et al. JSC-Rocknest: A large-scale Mojave Mars Simulant (MMS) based soil simulant for in situ resource utilization water-extraction studies. Icarus 351 , (2020).
Scott, A. N., Oze, C., Tang, Y. & O’Loughlin, A. Development of a Martian regolith simulant for in situ resource utilization testing. Acta Astronaut. 131 , 45–49 (2017).
Walkinshaw, C. H. & Johnson, P. H. Analysis of vegetable seedlings grown in contact with Apollo 14 lunar surface fines. HortScience 6 , 532–535 (1971).
Walkinshaw, C. H., Sweet, H. C., Venketeswaran, S. & Home, W. H. Results of Apollo 11 and 12 quarantine studies on plants. BioScience 20 , 1297–1302 (1970).
Weete, J. D. & Walkinshaw, C. H. Apollo 12 lunar material: effects on plant pigments. Canad. J. Bot. 50 , 101–104 (1972).
Baur, P. S., Clark, R. S., Walkinshaw, C. H. & Scholes, V. E. Uptake and translocation of elements from Apollo 11 lunar material by lettuce seedlings. Phyton 32 , 133–142 (1974).
CAS Google Scholar
Milov, M. & Rusakova, G. Greenhouses in space-higher plants in closed ecological systems. Aviatsia Cosmonaut. 3 , 36–37 (1980).
Walkinshaw, C. H. Space greenhouses could operate efficiently at low pressures if fungi are controlled. Phytopathology 76 , 1141 (1986).
Mashinskiy, A. & Nechitaylo, G. Birth of space agriculture. Tek Molodezhi 4 , 2–7 (1983).
Ming, D. W. & Henninger, D. L. Lunar base agriculture: soils for plant growth. Am. Soc. Agron. 781 , 255 (1989).
Fairchild, K. O., Roberts, B. B. Options for the human settlement of the Moon and Mars. In Ming, D. W. and Henninger, D. L. (Ed.). Lunar Base Agriculture: Soils for Plant Growth. 255 (American Society on Agronomy, 1989).
Aglan, H., Mortley, D., Trotman, A., Loretan, P. & Hill, W. Sweetpotato growth using a microporous tube system with lunar simulant medium. SAE Trans. (JSTOR) 107 , 1012–1016 (1998).
Hossner, L. R., Ming, D. W., Henninger, D. L. & Allen, E. R. Lunar outpost agriculture. Endeavour. 15 , 79–85 (1991).
Walkinshaw, C. H. and Galliano, S. G. New crops for space bases. In: Janick J. and Simon J. E. (eds.) Advances in New Crops. 532–535 (Timber Press, Portland, OR, 1990).
Kozyrovska, N. O. et al. Growing pioneer plants for a lunar base. Adv. Space Res. 37 , 93–99 (2006).
Mortley, D. G., Aglan, H. A., Bonsi, C. K., and Hill, W. A. Growth of Sweetpotato in Lunar and Mars Simulants. SAE. Technical Paper, (No. 2000-01-2289). https://doi.org/10.4271/2000-01-2289 (2000).
Duri, L. G. et al. Mars regolith simulant ameliorated by compost as in situ cultivation substrate improves lettuce growth and nutritional aspects. Plants 9 , 628 (2020).
Wamelink, G. W. W., Frissel, J. Y., Krijnen, W. H. J. & Verwoert, M. R. Crop growth and viability of seeds on Mars and Moon soil simulants. Open Agric. 4 , 509–516 (2019).
Caporale, A. G. et al. Geo-mineralogical characterisation of Mars simulant MMS-1 and appraisal of substrate physico-chemical properties and crop performance obtained with variable green compost amendment rates. Sci. Total Environ. 720 , 137543 (2020).
Misra, G., Smith, W. Garner, M. and Loureiro, R. Potential biological remediation strategies for removing perchlorate from Martian regolith. New Space . 217–227. https://doi.org/10.1089/space.2020.0055 (2021).
Paul, A.-L., Elardo, S. M. & Ferl, R. J. Plants grown in Apollo lunar regolith present stress-associated transcriptomes that inform prospects for lunar exploration. Commun. Biol. 5 , 382 (2022).
Jacoby, R., Peukert, M., Succurro, A., Koprivova, A. & Kopriva, S. The role of soil microorganisms in plant mineral nutrition-current knowledge and future directions. Front. Plant Sci. 8 , 1617 (2017).
Lambers, H., Mougel, C., Jaillard, B. & Hinsinger, P. Plant-microbe-soil interactions in the rhizosphere: an evolutionary perspective. Plant Soil 321 , 83–115 (2009).
Lyu, D., et al. The coevolution of plants and microbes underpins sustainable agriculture. Microorganisms 9 . https://doi.org/10.3390/MICROORGANISMS9051036 (2021).
Morris, J. L. et al. Timescale of early land plant evolution. Proc. Natl Acad. Sci. 115 , E2274–E2283 (2018).
Bulgarelli, D., Schlaeppi, K., Spaepen, S., Van Themaat, E. V. L. & Schulze-Lefert, P. Structure and functions of the bacterial microbiota of plants. Annu. Rev. Plant Biol. 64 , 807–838 (2013).
Egamberdieva, D. et al. High incidence of plant growth-stimulating bacteria associated with the rhizosphere of wheat grown on salinated soil in Uzbekistan. Environ. Microbiol. 10 , 1–9 (2008).
Mendes, R. et al. Deciphering the rhizosphere microbiome for disease-suppressive bacteria. Science 332 , 1097–1100 (2011).
Barea, J. M., Azcón, R. & Azcón-Aguilar, C. Mycorrhizosphere interactions to improve plant fitness and soil quality. Antonie van Leeuwenhoek, Int. J. Gen. Mol. Microbiol. 81 , 343–351 (2002).
Donn, S., Kirkegaard, J. A., Perera, G., Richardson, A. E. & Watt, M. Evolution of bacterial communities in the wheat crop rhizosphere. Environ. Microbiol. 17 , 610–621 (2015).
Dutta, S. & Podile, A. R. Plant Growth Promoting Rhizobacteria (PGPR): the bugs to debug the root zone. Crit. Rev. Microbiol. 36 , 232–244 (2010).
Huang, X.-F. et al. Rhizosphere interactions: root exudates, microbes, and microbial communities. Botany 92 , 267–275 (2014).
Lynch, J. P. Turner review no. 14. Roots of the second green revolution. Aust. J. Bot. 55 , 493–512 (2007).
Mendes, R., Garbeva, P. & Raaijmakers, J. M. The rhizosphere microbiome: significance of plant beneficial, plant pathogenic, and human pathogenic microorganisms. FEMS Microbiol. Rev. 37 , 634–663 (2013).
Pingali, P. L. Green revolution: impacts, limits, and the path ahead. Proc. Natl. Acad. Sci. USA 109 , 12302–12308 (2012).
Busby, P. E. et al. Research priorities for harnessing plant microbiomes in sustainable agriculture. PLOS Biol. 15 , e2001793 (2017).
Emmett, B. D., Youngblut, N. D., Buckley, D. H., and Drinkwater, L. E. Plant phylogeny and life history shape rhizosphere bacterial microbiome of summer annuals in an agricultural field. Front. Microbiol . 8 . https://doi.org/10.3389/fmicb.2017.02414 (2017).
Nuccio, E. E. et al. Niche differentiation is spatially and temporally regulated in the rhizosphere. ISME J. 14 , 999–1014 (2020).
Massa, G. D. et al. VEG-01: veggie hardware validation testing on the international space station. Open Agric. 2 , 33–41 (2017).
Schuerger, A. C. et al. Fusarium oxysporum as an opportunistic fungal pathogen on Zinnia hybrida plants grown on Board the International Space Station. Astrobiology 21 , 1029–1048 (2021).
Avila-Herrera, A. et al. Crewmember microbiome may influence microbial composition of ISS habitable surfaces. PLoS One 15 , e0231838 (2020).
Khodadad, C. L. M. et al. Microbiological and nutritional analysis of lettuce crops grown on the International Space Station. Front. Plant Sci. 11 , 199 (2020).
Handy, D. et al. Identification of plant growth promoting bacteria within space crop production systems. Front. Astron. Sp. Sci. 8 , 183 (2021).
Allen, C. C., Griffin, C., Steele, A., Wainwright, N., & Stansbery, E. Microbial life in Martian regolith simulant JSC Mars-1. Lunar and Planetary Science XXXI (2000).
Gutiérrez-Mañero, F. J. et al. The plant-growth-promoting rhizobacteria Bacillus pumilus and Bacillus licheniformis produce high amounts of physiologically active gibberellins. Physiol. Plant. 111 , 206–211 (2001).
Nascimento, F. X., Hernández, A. G., Glick, B. R. & Rossi, M. J. Plant growth-promoting activities and genomic analysis of the stress-resistant Bacillus megaterium STB1, a bacterium of agricultural and biotechnological interest. Biotechnol. Rep. 25 , e00406 (2020).
Coutinho, F. P., Felix, W. P. & Yano-Melo, A. M. Solubilization of phosphates in vitro by Aspergillus spp. and Penicillium spp. Ecol. Eng. 42 , 85–89 (2012).
Yin, Z. et al. Phosphate solubilization and promotion of maize growth by Penicillium oxalicum P4 and Aspergillus niger P85 in a calcareous soil. Can. J. Microbiol. 61 , 913–923 (2015).
Perincherry, L., Lalak-Kánczugowska, J. & Stepién, L. Fusarium-produced mycotoxins in plant-pathogen interactions. Toxins 11 , 664 (2019).
Kral, T. A. & Aitheide, T. S. Methanogen survival following exposure to desiccation, low pressure and martian regolith analogs. Planet. Space Sci. 89 , 167–171 (2013).
Schirmack, J., Alawi, M. & Wagner, D. Influence of Martian regolith analogs on the activity and growth of methanogenic archaea, with special regard to long-term desiccation. Front. Microbiol. 6 , 210 (2015).
Schuerger, A. C., Golden, D. C. & Ming, D. W. Biotoxicity of Mars soils: 1. Dry deposition of analog soils on microbial colonies and survival under Martian conditions. Planet. Space Sci. 72 , 91–101 (2012).
Kerney, K. R. & Schuerger, A. C. Survival of Bacillus subtilis endospores on ultraviolet-irradiated rover wheels and Mars regolith under simulated Martian conditions. Astrobiology 11 , 477–485 (2011).
Cousins, C. R. et al. An ESA roadmap for geobiology in space exploration. Acta Astronaut. 118 , 286–295 (2016).
Volger, R. et al. Mining moon & mars with microbes: biological approaches to extract iron from Lunar and Martian regolith. Planet. Space Sci. 184 , 104850 (2020).
Kaksonen, A. H. et al. Potential of Acidithiobacillus ferrooxidans to grow on and bioleach metals from Mars and Lunar regolith simulants under simulated microgravity conditions. Microorg. 9 , 2416 (2021).
Desbrosses, G. J. & Stougaard, J. Root nodulation: a paradigm for how plant-microbe symbiosis influences host developmental pathways. Cell Host Microbe 10 , 348–358 (2011).
Harris, F., Dobbs, J., Atkins, D., Ippolito, J. A. & Stewart, J. E. Soil fertility interactions with Sinorhizobium-legume symbiosis in a simulated Martian regolith; effects on nitrogen content and plant health. PLoS One 16 , e0257053 (2021).
Rainwater, R. & Mukherjeeid, A. The legume-rhizobia symbiosis can be supported on Mars soil simulants. PLoS One 16 , e0259957 (2021).
Brundrett, M. C. Coevolution of roots and mycorrhizas of land plants. New Phytol. 154 , 275–304 (2002).
Konings-Dudin, G., Butcher, M. J., Castor-Macías, J. A., Kohanloo, B. & Garcia, M. Endomycorrhizal Fungi and Opuntia ficus-indica seed germination on a lunar regolith simulant. Adv. Microbiol. 2014 , 616–626, https://doi.org/10.4236/AIM.2014.410068 (2014).
Gilrain, M. R., Hogan, J. A., Cowan, R. M., Finstein, M. S., and Logendra, L. S. Preliminary study of greenhouse-grown Swiss chard in mixtures of compost and Mars regolith simulant. SAE Tech. Pap . https://doi.org/10.4271/1999-01-2021 (1999).
Zaets, I. et al. Bioaugmentation in growing plants for lunar bases. Advances in Space Research 47 , 1071–1078 (2011).
Lytvynenko, T. et al. A rationally assembled microbial community for growing Tagetes patula L. in a lunar greenhouse. Res. Microbiol. 157 , 87–92 (2006).
Alshaal, T. & El-Ramady, H. Foliar application: from plant nutrition to biofortification. Environ. Biodivers. Soil Secu. 1 , 71–83 (2017).
Clijsters, H. & Van Assche, F. Inhibition of photosynthesis by heavy metals. Photosynth. Res. 7 , 31–40 (1985).
Sharma, B. Responses of Some Vegetable Crops to Toxic Heavy Metals ( Doctoral dissertation) Tribhuvan University (2008).
Badri, D. V. & Vivanco, J. M. Regulation and function of root exudates. Plant Cell Environ. 32 , 666–681 (2009).
Bertin, C., Yang, X. & Weston, L. A. The role of root exudates and allelochemicals in the rhizosphere. Plant Soil 256 , 67–83 (2003).
Keiluweit, M. et al. Mineral protection of soil carbon counteracted by root exudates. Nat. Clim. Change 5 , 588–595 (2015).
Schrader, C. et al. Lunar regolith characterization for simulant design and evaluation using figure of merit algorithms. In 47th AIAA Aerospace Sciences Meeting Including the New Horizons Forum and Aerospace Exposition (p. 755) (2009).
Metzger, P., Britt, D., Covey, S., & Lewis, J. S. Figure of merit for asteroid regolith simulants. In European Planetary Science Congress (Vol. 11) (2017).
Moore, K. & Bradley, L. K. (eds.). North Carolina Extension Gardener Handbook (NC State Extension, College of Agriculture and Life Sciences, NC State University, 2018).
Gavilanes, F. Z. et al. Co-inoculation of Anabaena cylindrica with Azospirillum brasilense increases grain yield of maize hybrids. Rhizosphere 15 , 100224 (2020).
Heydarian, Z. et al. Inoculation of soil with plant growth promoting bacteria producing 1-aminocyclopropane-1-carboxylate deaminase or expression of the corresponding acdS gene in transgenic plants increases salinity tolerance in Camelina sativa. Front. Microbiol. 7 , 1966 (2016).
Kholssi, R., et al. Biofertilizing effects of Anabaena cylindrica biomass on the growth and nitrogen uptake of wheat. 53 , 1216–1225. https://doi.org/10.1080/00103624.2022.2043350 (2022).
Barillot, C. D. C., Sarde, C. O., Bert, V., Tarnaud, E. & Cochet, N. A standardized method for the sampling of rhizosphere and rhizoplan soil bacteria associated to a herbaceous root system. Ann. Microbiol. 632 , 471–476 (2012).
Both, A. J., et al. Guidelines for measuring and reporting environmental parameters for experiments in greenhouses. Plant Methods 11 . https://doi.org/10.1186/s13007-015-0083-5 (2015).
Katagiri, F. et al. Design and construction of an inexpensive homemade plant growth chamber. PLoS One 10 , e0126826 (2015).
Download references
Acknowledgements
The authors thank the NASA Solar System Exploration Research Virtual Institute (SSERVI) Center for Lunar and Asteroid Surface Science (CLASS) (NASA Cooperative Agreement 80NSSSC19M0124), the NASA Space Technology Graduate Research Opportunity (NSTGRO) Fellowship (NASA Cooperative Agreement 80NSSC23K1173), and the Planetary Society for their financial support, which made the publication of this manuscript possible. The authors also acknowledge the Evans Library Open Access Subvention Fund at Florida Tech for assistance with publication costs.
Author information
Authors and affiliations.
Department of Astronomy and Planetary Science, Northern Arizona University, Flagstaff, AZ, 86011, USA
Laura E. Fackrell
Department of Horticultural Science, North Carolina State University, Raleigh, NC, 27607, USA
Samson Humphrey
Department of Biological Sciences, Winston-Salem State University, Winston-Salem, NC, 27101, USA
Rafael Loureiro
Department of Ocean Engineering and Marine Sciences, Florida Institute of Technology, Melbourne, FL, 32904, USA
Andrew G. Palmer
Department of Biomedical Engineering and Science, Florida Institute of Technology, Melbourne, FL, 32904, USA
Department of Physics, University of Central Florida, Orlando, FL, 32816, USA
Jared Long-Fox
You can also search for this author in PubMed Google Scholar
Contributions
All authors contributed equally to the manuscript. S.H. and R.L. contributed most greatly to the plant-focused parts of this paper, L.F. contributed most greatly to the regolith properties parts of this paper, especially all the parts that refer to Martian regolith, J.L.F. contributed most greatly to the regolith properties parts of this paper, especially the parts that refer to Lunar regolith, and A.P. contributed most greatly to the parts of this paper that discuss the microbiome. All authors contributed to the graphs, and all authors were heavily involved with editing each others' work. Special thanks to Ralph Fritsche, who reviewed the manuscript and offered his insight before submission.
Corresponding author
Correspondence to Samson Humphrey .
Ethics declarations
Competing interests.
The authors declare no competing interests.
Additional information
Publisher’s note Springer Nature remains neutral with regard to jurisdictional claims in published maps and institutional affiliations.
Supplementary information
Supplementary table s1, rights and permissions.
Open Access This article is licensed under a Creative Commons Attribution 4.0 International License, which permits use, sharing, adaptation, distribution and reproduction in any medium or format, as long as you give appropriate credit to the original author(s) and the source, provide a link to the Creative Commons licence, and indicate if changes were made. The images or other third party material in this article are included in the article’s Creative Commons licence, unless indicated otherwise in a credit line to the material. If material is not included in the article’s Creative Commons licence and your intended use is not permitted by statutory regulation or exceeds the permitted use, you will need to obtain permission directly from the copyright holder. To view a copy of this licence, visit http://creativecommons.org/licenses/by/4.0/ .
Reprints and permissions
About this article
Cite this article.
Fackrell, L.E., Humphrey, S., Loureiro, R. et al. Overview and recommendations for research on plants and microbes in regolith-based agriculture. npj Sustain. Agric. 2 , 15 (2024). https://doi.org/10.1038/s44264-024-00013-5
Download citation
Received : 24 October 2023
Accepted : 12 March 2024
Published : 12 September 2024
DOI : https://doi.org/10.1038/s44264-024-00013-5
Share this article
Anyone you share the following link with will be able to read this content:
Sorry, a shareable link is not currently available for this article.
Provided by the Springer Nature SharedIt content-sharing initiative
Quick links
- Explore articles by subject
- Guide to authors
- Editorial policies
Sign up for the Nature Briefing: Microbiology newsletter — what matters in microbiology research, free to your inbox weekly.

Search within the TIB website or find specialist literature and information in the TIB Portal.
The TIB Portal allows you to search the library's own holdings and other data sources simultaneously. By restricting the search to the TIB catalogue, you can search exclusively for printed and digital publications in the entire stock of the TIB library.
Book Review Article (English)
- ISSN: 1035-8811 , 1757-6547
- Article (Journal) / Electronic Resource
How to get this title?
Show citation formats, export, share and cite, pricing information, please choose your delivery country and your customer group.
* Mandatory field
More details on this result
- Title: Book Review Article
- Published in: The Australian Journal of Anthropology ; 11, 1 ; 59-77
- Publisher: Blackwell Publishing Ltd
- New search for: Blackwell Publishing Ltd
- Publication date: 2000-04-01
- Size: 19 pages
- DOI: https://doi.org/10.1111/j.1835-9310.2000.tb00263.x
- Type of media: Article (Journal)
- Type of material: Electronic Resource
- Language: English
- Source: Wiley
Table of contents
Table of contents – volume 11, issue 1.
Show all volumes and issues
The tables of contents are generated automatically and are based on the data records of the individual contributions available in the index of the TIB portal. The display of the Tables of Contents may therefore be incomplete.
Similar titles
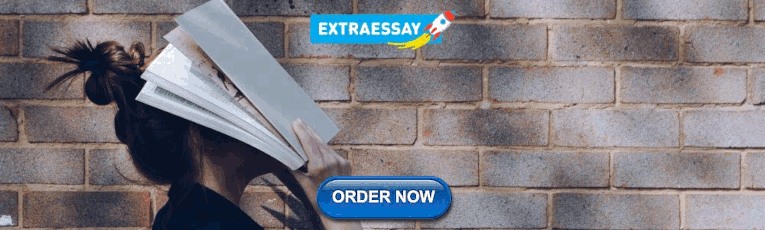
COMMENTS
Agricultural Research is a multi-disciplinary journal covering all disciplines of agricultural sciences to promote global research. The official publication of the National Academy of Agricultural Sciences (NAAS), India. Focuses on new and emerging fields and concepts in agricultural sciences. Provides a forum for Agricultural Scientists to ...
Agriculture is the cultivation of plants, animals, and some other organisms, such as fungi, for the production of food, fibre, fuel, and medicines used by society. ... Research, policy and ...
Outlook on Agriculture is a peer reviewed journal, published quarterly, which welcomes original research papers, reviews and perspectives on current developments in agricultural science and associated disciplines for an international and … | View full journal description. This journal is a member of the Committee on Publication Ethics (COPE).
Advances in Agriculture is an open access journal dedicated to publishing research on the cultivation of soil and crops, and the rearing of livestock. Its focus is on new methods and technologies for improving agricultural processes, increasing yield, conservation and breeding. As part of Wiley's Forward Series, this journal offers a ...
The Journal of Agriculture and Food Research is a peer-reviewed open access journal focusing on research in the agricultural and food sciences. The journal welcomes full length research articles, reviews, short communications, perspectives, and commentaries from researchers in academic …. View full aims & scope. $2120. Article publishing charge.
Agriculture is an international, scientific peer-reviewed open access journal published monthly online by MDPI.. Open Access — free for readers, with article processing charges (APC) paid by authors or their institutions.; High Visibility: indexed within Scopus, SCIE (Web of Science), PubAg, AGRIS, RePEc, and other databases. Journal Rank: JCR - Q1 (Agronomy) / CiteScore - Q1 (Plant Science)
FULL-LENGTH RESEARCH ARTICLE Open access 28 May 2024. 1. 2. …. 16. Next. Agricultural Research is a multi-disciplinary journal covering all disciplines of agricultural sciences to promote global research. The official publication ...
The journal welcomes research in areas including agricultural engineering, soil science and biotechnology, agroecology and agricultural management and economics, bioenergy crops, farming techniques, food security and much more. ... In this paper, we begin by summarising recent progress in designing or engineering in vitro CO 2 fixation pathways
In this systematic review paper, we aim to advance understandings of sustainable agriculture from a social science and governance perspective by identifying areas of complementarity and concern ...
The analysis (The State of Food Security and Nutrition in the World FAO; 2018) is the outcome of a close collaboration between five United Nations agencies — the Food and Agriculture ...
This paper presents a comprehensive review of emerging technologies for the internet of things (IoT)-based smart agriculture. We begin by summarizing the existing surveys and describing emergent technologies for the agricultural IoT, such as unmanned aerial vehicles, wireless technologies, open-source IoT platforms, software defined networking (SDN), network function virtualization (NFV ...
OFE research is demand driven, because the motivations of farmers to gain information relevant to their own farm drive the research process 14,16,17.OFE is a concrete, observable activity of clear ...
As agriculture meets digital technologies, a new frontier of innovation is emerging and creating multiple pathways to a smart farming future. This paper presents a case study of a smart farming innovation originating from a small-to-medium sized enterprise (SME) that designs and manufactures machinery used in broadacre, conservation tillage farming.
Leibniz Centre for Agriculture Landscape Research, Muencheberg, Germany. Search for more papers by this author. C. Ringler, ... In this paper, we analyze pathways of agricultural productivity, land use, and land-cover changes in India until 2030 and their impacts on terrestrial biodiversity and carbon storage. The results show that in order to ...
Current Agriculture Research Journal is an open access, international, scholarly peer-reviewed research journal which publishes original research after double-blind peer review. Published triannually in April, August and December with an aim to foster high-quality research in the field of agricultural sciences.
An interconnected combination of steps could help ensure that the most vulnerable countries and people get the nutrition they need. 1 The modest ambition of this paper is to review the economic literature on agriculture, focusing on the issues that are critical for agricultural productivity and poverty reduction.
The Oxford English Dictiona ry (1971) defines agriculture. very broadly as "The science and art of cultivating the soil, including the allied pursuits of. gathering in the crops and rearing live ...
New Agriculture Technology in Modern Farming. September 2021; 8(3):109-114; ... Discover the world's research. 25+ million members; 160+ million publication pages; 2.3+ billion citations;
While the use of the adjective regenerative is expanding among activists, civil society groups and corporations as they call for renewal, transformation and revitalization of the global food system (Duncan et al., 2021), in this paper we explore the calls for Regenerative Agriculture from an agronomic perspective.By this we mean a perspective steeped in the use of plant, soil, ecological and ...
The Journal of Agricultural Extension Management (JAEM) disseminates information relating to extension systems and practices, research on extension, efficient organization of technology transfer and other socio-economic issues concerning agriculture and allied areas. Papers on original research in the field of agricultural extension and allied ...
The main purpose of this paper is to introduce the modern technology adoption its importance, usage and role in agriculture improvement. In the last century, the basic agriculture technology like ...
23. Life Style, Agriculture, Time Use, Risk assessment. Review of Russian language studies on radionuclide behaviour in agricultural animals: part 1. Gut absorption. An extensive programme of experiments was conducted in the former USSR on transfer of radionuclides to a wide range of different agricultural animals.
Lunar and Martian regolith simulants and agriculture research. In order to identify appropriate methods for processing regolith for agricultural applications, potential methods must be tested ...
Research Paper Jun Du1, Xingyi Liu2, Oleksandr Shepotylo3, and Yujie Shi4 16 September 2024 Abstract ... agricultural goods showing an average imported varieties decline of around 4.75%, some goods decline drastically, such as HS03 (Fish, 51.2%) and HS10 (Cereals, 21.61%). Some products
Kekane M. A., (2013), Indian Agriculture-Status, Importance and Role in Indian Economy, International Journal of Agriculture and Food Science Technology., reviewed by Research India Publications ...
The TIB Portal allows you to search the library's own holdings and other data sources simultaneously. By restricting the search to the TIB catalogue, you can search exclusively for printed and digital publications in the entire stock of the TIB library.