Loading metrics
Open Access
Essays articulate a specific perspective on a topic of broad interest to scientists.
See all article types »
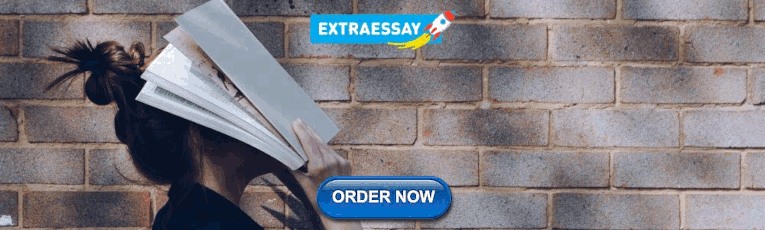
60 years ago, Francis Crick changed the logic of biology
* E-mail: [email protected]
Affiliation School of Biological Sciences, University of Manchester, Manchester, United Kingdom

- Matthew Cobb
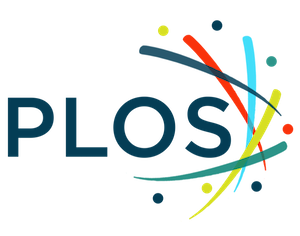
Published: September 18, 2017
- https://doi.org/10.1371/journal.pbio.2003243
- Reader Comments
In September 1957, Francis Crick gave a lecture in which he outlined key ideas about gene function, in particular what he called the central dogma. These ideas still frame how we understand life. This essay explores the concepts he developed in this influential lecture, including his prediction that we would study evolution by comparing sequences.
Citation: Cobb M (2017) 60 years ago, Francis Crick changed the logic of biology. PLoS Biol 15(9): e2003243. https://doi.org/10.1371/journal.pbio.2003243
Copyright: © 2017 Matthew Cobb. This is an open access article distributed under the terms of the Creative Commons Attribution License , which permits unrestricted use, distribution, and reproduction in any medium, provided the original author and source are credited.
Funding: Cold Spring Harbor Laboratory Sydney Brenner Research Scholarship. The funder had no role in study design, data collection and analysis, decision to publish, or preparation of the manuscript.
Competing interests: The authors have declared that no competing interests exist.
Provenance: Not commissioned; externally peer reviewed.
Introduction
This month marks the 60th anniversary of one of the most significant lectures in the history of biology. It was given on 19 September 1957 by Francis Crick as part of a Society for Experimental Biology symposium on the Biological Replication of Macromolecules, held at University College London. Originally entitled ‘Protein synthesis,’ the title acquired a magisterial introductory ‘On’ during writing up for publication the following year [ 1 ]. The lecture went far further than its title suggested: as Crick pointed out in the opening paragraph, he also addressed ‘the other central problems of molecular biology—those of gene action and nucleic acid synthesis.’
Crick’s talk is now often called the ‘central dogma’ lecture, for it was here that he first publicly presented this frequently misunderstood concept. While this was highly significant, the content of the lecture was even richer—it also saw Crick outline his view of the nature of life and of genetic information and the source of protein folding as well as making two bold and spectacularly accurate predictions: that there must exist a small ‘adaptor’ molecule (now known as tRNA) that could bring amino acids to the site of protein synthesis and that in the future, scientists would be able to explore rich evolutionary sources of information by comparing sequence data. In this one brief lecture, Crick profoundly influenced how we think. In The Eighth Day of Creation , journalist Horace Judson went so far as to claim that on that day 60 years ago, Crick “permanently altered the logic of biology [ 2 ].”
Crick’s presentation
Crick’s hour-long lecture was given on the third day of a leisurely 4-day meeting (at most four talks a day), with participants from France, the United States, Belgium, and Hungary as well as a solid contingent of Britons. One of the French speakers was molecular geneticist François Jacob, for whom this was his first encounter with Crick. The impression Crick made was lasting—30 years later, Jacob recalled the lecture:
“Tall, florid, with long sideburns, Crick looked like the Englishman seen in illustrations to 19th century books about Phileas Fogg or the English opium eater. He talked incessantly. With evident pleasure and volubly, as if he was afraid he would not have enough time to get everything out. Going over his demonstration again to be sure it was understood. Breaking up his sentences with loud laughter. Setting off again with renewed vigour at a speed I often had trouble keeping up with…Crick was dazzling.” [ 3 ]
There is no manuscript of Crick’s actual talk, only the 11,000-word article that was published in 1958, which Crick prepared for publication in October 1957. [ 4 ] This version would presumably have been too long for Crick to read out in his 60-minute slot, even if he did speak incredibly quickly and, as he recalled, ‘ran overtime’ [ 2 ]. According to the acknowledgement in the paper, the version with which we are all familiar was the product of many discussions with Sydney Brenner, who also played a role in “redrafting” the manuscript, presumably for publication.
Crick’s opening statement may seem unsettling to the modern reader:
“I shall…argue that the main function of the genetic material is to control (not necessarily directly) the synthesis of proteins. There is a little direct evidence to support this, but to my mind the psychological drive behind this hypothesis is at the moment independent of such evidence.”
This highlights how uncertain scientists were at the time about gene function—as Crick pointed out, at the time, not everyone accepted that nucleic acids were involved in protein synthesis [ 5 ]. In 1957, ribosomes were known only as microsomes, and their function and composition was uncertain; messenger RNA was still undreamt of—it would be properly identified only in the summer of 1960, and the discovery was not published until the following year [ 6 , 7 , 8 ].
Faced with the lack of experimental evidence as to how genes produced proteins, Crick fell back on what he excelled in: outlining general, bold concepts that drew together a wide variety of strands into a compelling whole. As he recalled: “In looking back I am struck…by the brashness which allowed us to venture powerful statements of a very general nature [ 9 ].”
Protein synthesis and the sequence hypothesis
Crick had been thinking at a very high level about the relation between DNA, RNA, and protein for several years, partly inspired by documents and letters that were exchanged between members of the 20-strong RNA Tie Club, a loose discussion group that included Brenner, Jim Watson, and a host of physicists and mathematicians, led by George Gamow [ 10 ]. In 1954, Watson wrote a series of letters to Crick as he tried to grapple with the role of RNA, which he jokingly called ‘the mysteries of life’ [ 11 ]. Watson initially thought that DNA might be chemically converted into RNA but gradually shifted his view and ended up arguing that DNA acted as a template for RNA, an answer he described as ‘not ugly’ [ 12 ].
Crick took these ideas and the experimental data that increasingly suggested that RNA was some kind of intermediate between DNA and protein (these data referred to ribosomes rather than mRNA) and developed a scheme to explain the relations between these three classes of biological molecules. In so doing, he had to get to grips with what exactly was in a gene and what took place if DNA was used as a template for RNA—not in biochemical terms, but in the most abstract way possible.
To do this, Crick had to resolve an issue that had been perplexing scientists since he and Watson introduced the concept of “genetic information” in their second, less often-read 1953 Nature article [ 13 ]. Although the idea had been rapidly and widely adopted, no one was clear what exactly genetic information might consist of. In his 1957 lecture, Crick gave a disarmingly straightforward definition—information in this context was simply ‘the determination of a sequence of units.’ This highlighted the existence of a link between the base sequences of nucleic acids and those of amino acids in a protein—they pointed to the reality of the genetic code. This in turn enabled Crick to conceptualize the link between gene and protein. He called this link “the flow of information” and added this concept to the factors that were generally accepted to describe protein synthesis and, indeed, life itself—the flow of matter and the flow of energy.
This definition of information raised a problem. Proteins are 3-dimensional (3D) structures whereas a DNA sequence is 1-dimensional (1D). Crick recognized that there might be some unknown source of information that enabled proteins to fold, but he argued that the ‘more likely hypothesis’ was that ‘folding is simply a function of the order of the amino acids.’ In other words, 3D protein structure is an emergent property of the 1D sequence. This simple ‘sequence hypothesis,’ as he termed it, remains essentially true today, despite the acknowledged role of molecular chaperones.
The central dogma
The most widely known of the powerful statements made by Crick in his lecture related to the flow of information between genes and proteins [ 14 ]. He had been musing about this for some time and in October 1956 wrote a set of notes entitled ‘Ideas on protein synthesis’ that took up 2 pages [ 15 ]. The second sentence of this document read, “The Central Dogma: ‘Once information has got into a protein it can’t get out again. Information here means the sequence of the amino acid residues, or other sequences related to it.’” This statement was repeated several times in the September 1957 lecture and also appeared in a Scientific American article on nucleic acids, which Crick published in October 1957 [ 16 ].
In Crick’s 1956 notes, this definition of the central dogma was followed by a diagram illustrating his idea, with arrows drawn in blue biro ( Fig 1 ). This figure was never published, although Crick did draw it on the blackboard when giving talks (see Fig 2 , from 1963—he may have done something similar in September 1957), and a slightly amended version was eventually published in 1970 [ 17 ].
- PPT PowerPoint slide
- PNG larger image
- TIFF original image
Credit: Wellcome Library, London.
https://doi.org/10.1371/journal.pbio.2003243.g001
Note the drawing of the central dogma on the blackboard. Credit : Cold Spring Harbor Laboratory .
https://doi.org/10.1371/journal.pbio.2003243.g002
For Crick, four kinds of information transfer clearly existed: DNA → DNA (DNA replication), DNA → RNA (the first step of protein synthesis), RNA → protein (the second step of protein synthesis) and RNA → RNA (RNA viruses copying themselves). There were two steps for which there was no evidence but that Crick thought were possible (hence the dotted lines in the figure): DNA → protein (this would mean RNA was not involved in protein synthesis) and RNA → DNA (structurally possible, but at the time, there no was no perceptible biological function).
Just as striking were the three flows of information that Crick considered to be impossible due to both lack of evidence and lack of biochemical mechanism. These were protein → protein, protein → RNA, and above all, protein → DNA. This was what Crick meant when he said that once information had gone from DNA into the protein, it could not get out of the protein and go back into the genetic code. This is the central dogma.
Crick admitted that the direct evidence for this hypothesis was ‘negligible’ and that it had a ‘speculative nature,’ but he defended his approach by pointing out that cosmologists had no qualms about constructing theories without adequate experimental data. That implicit comparison with grand theories of the universe is justified, for Crick was laying out the foundations of a new way of understanding how the cell works. The simplicity of the sequence hypothesis and the central dogma, together with the focus on information, brought a clear explanatory power to the synthesis of protein molecules that could take virtually any form and could ‘do almost anything,’ as Crick put it. Once the cell’s fundamental activity was conceived of in this way, everything fell into place. Crick advised his listeners to attempt to explain protein synthesis without these two basic principles—it was ‘an instructive exercise,’ he said. ‘One generally ends in the wilderness,’ he claimed.
Students are now often mistakenly taught that the central dogma is something like ‘DNA → RNA → protein’ (as popularised by Watson in his 1965 textbook Molecular Biology of the Gene [ 18 ]) or, even less precisely, ‘DNA makes RNA makes protein’ (as first suggested by Jean Brachet in 1960 [ 19 ]). This view, which went back to André Boivin in 1949 [ 20 ] and Alexander Dounce in 1953 [ 21 ], was very different to what Crick had in mind (it also confuses students, who often fail to grasp what the arrows mean or ‘makes’ implies [ 22 ]).
In 1970, following the discovery by Howard Temin and David Baltimore of reverse transcriptase, which enables information to flow in the direction RNA → DNA, Nature published an editorial entitled ‘Central dogma reversed’ [ 23 ]. Crick wrote a slightly tetchy response, repeating what he had actually stated in 1957, and rightly insisting that he had never argued that RNA → DNA was impossible [ 17 ]. In a distinctly undogmatic approach, he emphasised that our knowledge of cell biology was remarkably limited and that surprises might be in store, pointing to the example of the disease scrapie in which a protein seemed to act as an infectious agent (Stanley Prusiner later described this as a prion). However, even in the case of scrapie and other prion diseases, infection involves a change in conformation, not de novo synthesis.
Crick’s essential argument still holds: protein synthesis relies on nucleic acids, and once the genetic information has got into the protein, it cannot alter the DNA sequence. Despite recent excitement about transgenerational epigenetic inheritance due to histone modifications, DNA methylation, or other temporary modifications of material surrounding the genetic sequence, there is no evidence in any organism that the information in a DNA sequence can be rewritten from information in a protein.
In one aspect of the central dogma, Crick was mistaken. In reality, the ‘Central Dogma’ was anything but a dogma. Crick later claimed that he had not properly understood the meaning of ‘dogma’—Jacques Monod had to explain to him exactly what it meant. An indication of the truth of this assertion can be seen in the lecture when he states that the name that he has coined emphasizes the speculative nature of the idea—a dogma is not speculative. As Crick later acknowledged, a more accurate description would have been ‘basic assumption’ [ 17 ]. This does not sound quite so sexy, but it would have removed a lot of subsequent misunderstanding. Perhaps if Crick had not used such a dramatic turn of phrase, many subsequent critics would not have become so exercised about the question.
RNA and the adaptor
Crick used his lecture to publicly air another key idea about protein synthesis that he had been developing in private. In 1955, he circulated a note to the RNA Tie Club entitled ‘On degenerate templates and the adaptor hypothesis’ [ 24 ]. In this document, he argued that it was structurally impossible for any nucleic acid to act as a template for a particular amino acid; the duo of Crick and Brenner therefore came up with what Brenner called ‘the adaptor hypothesis’—an unknown class of molecule that would act like an electric plug adaptor, taking amino acids to the ribosome for protein assembly.
Crick was understandably unable to predict the nature of these adaptor molecules, but he felt that it was likely that they would contain nucleotides, which would be able to pair with both DNA and the RNA site of protein synthesis. Even allowing for the fact that he did not yet fully grasp the role of ribosomal RNA, Crick’s vision was astonishingly clear:
“The template could consist of perhaps a single chain of RNA…Each adaptor molecule containing, say, a di- or trinucleotide would each be joined to its own amino acid by a special enzyme. These molecules would then diffuse to the microsomal particles and attach to the proper place on the basis of the RNA by base-pairing.”
Crick and Brenner’s prediction would soon be proven correct—as Crick was giving his talk, Hoagland and Zamecnik were putting the finishing touches to their paper describing the isolation of the adaptor, which was eventually called tRNA [ 25 ].
Crick and evolutionary biology
There were two aspects of Crick’s lecture that related to evolutionary thinking. The first was that the central dogma supported the neo-Darwinian view that it was impossible for any character that was acquired during an organism’s life to affect its hereditary characters. This provided support for the widespread hostility to the view that had been held by Darwin, Lamarck, and others, according to which, patterns of use and disuse could lead to changes in the frequency of characters in subsequent generations.
Although in most organisms, including bacteria, plants, and even some animals, there is no separation between the copies of DNA used for protein synthesis and those used for transmitting genetic information to the next generation, Crick could see no conceivable mechanism whereby changes acquired during life could feed back into the DNA sequence. This was later considered to be an additional argument against Lamarckian inheritance and a reinforcement of Weismann’s separation of the germ and somatic cell lines (something that applies only to most animals) [ 2 ]. However, Crick did not mention either of these ideas.
The other evolutionary aspect to Crick’s lecture came in a brief and little-noticed aside, in which he essentially predicted the development of phylogenetics. In 1957, protein sequencing was extremely primitive, while sequencing DNA was two decades in the future. Complete amino acid sequences for insulin had been described for just five species, but nevertheless, Crick could see the way things would go. In an incredibly prescient prediction, he stated:
“Biologists should realise that before long we shall have a subject which might be called ‘protein taxonomy’—the study of the amino acid sequences of the proteins of an organism and the comparison of them between species. It can be argued that these sequences are the most delicate expression possible of the phenotype of an organism and that vast amounts of evolutionary information may be hidden away within them.”
This insight appears to have had little impact on thinking about the potential power of studying sequences—the history of bioinformatics [ 26 ] is generally traced back to the work of Dick Eck [ 27 ], Margaret Dayhoff [ 28 ], and Emile Zuckerkandl and Linus Pauling [ 29 ] in the early 1960s, none of whom cited Crick’s lecture. Further exploration of the work of the early bioinformaticians may reveal currently-unknown direct connections with Crick’s ideas, but whatever the case, the clarity of this vision underlines the power of Crick’s thinking.
It took some time for Crick’s lecture to exert its influence. Despite Jacob’s vivid description of how Crick presented his ideas, there is no indication that the content immediately changed the thinking of those in the audience. Only one of the other presentations at the symposium made any reference to Crick’s novel ideas in the revised printed version, and even here, the authors appear to have thought that Crick was indeed being dogmatic in his views because he speculated rather than strictly limiting himself to the experimental evidence [ 30 ].
Since then, the renown of the lecture has grown, and it has been cited over 800 times. The pattern of citations is U-shaped, with an early peak of 28 in 1962, followed by a trough of a handful of citations per year between 1971 and 1990, rising to 52 citations in 2014. Crick was later quite harsh on his lecture, describing it as ‘a mixture of good and bad ideas, of insight and nonsense’ [ 9 ]. This seems unfair—any nonsense is primarily due to lack of experimental evidence at the time. The reason why people still return to a 60-year-old lecture is because of the power of its ideas and the clarity with which they are presented. Crick’s style and intellectual verve continue to be both influential and inspirational; everyone should read or reread this brilliant lecture by one of the 20th century’s greatest scientists, a lecture that changed how we think.
- View Article
- PubMed/NCBI
- Google Scholar
- 2. Judson HF. The eighth day of creation: makers of the revolution in biology. Plainview: Cold Spring Harbor Laboratory Press; 1996.
- 3. Jacob F. The statue within. London: Unwin Hyman; 1988.
- 4. Crick FHC. On protein synthesis; 1957. Manuscript. Cold Spring Harbor Laboratory Archives, SB/11/5/4. http://libgallery.cshl.edu/items/show/52220 .
- 5. Cobb M. Life’s greatest secret: the race to crack the genetic code. London: Profile; 2015.
- 9. Crick F. What mad pursuit: a personal view of scientific discovery. Cambridge, Mass: Basic; 1998.
- 10. Watson JD. Genes, girls and Gamow. Oxford: Oxford University Press; 2001.
- 11. JD Watson to FHC Crick, 13 February 1954. Wellcome Library, Box 26 Folder PP/CRI/H/1/42/3. https://profiles.nlm.nih.gov/ps/access/SCBBWY.pdf
- 12. JD Watson to FHC Crick, 15 October 1954. Wellcome Library, Box 26 Folder PP/CRI/D/2/45. https://profiles.nlm.nih.gov/ps/access/SCBBJQ.pdf
- 15. Crick FHC. Ideas on protein synthesis (Oct. 1956). Unpublished note. Wellcome Library, PPCRI/H/2/6. https://wellcomelibrary.org/item/b18174139
- 18. Watson JD. Molecular biology of the gene. New York: W. A. Benjamin; 1965.
- 19. Brachet J. The biological role of nucleic acids. New York: Elsevier; 1965.
- 24. Crick FHC. On degenerate templates and the adaptor hypothesis. Unpublished note, RNA Tie Club; 1955. Wellcome Library, PPCRI/H/1/38. https://wellcomelibrary.org/item/b18186300
- 26. Stevens H. Life out of sequence: a data-driven history of bioinformatics. Chicago: University of Chicago Press; 2013.

An official website of the United States government
The .gov means it’s official. Federal government websites often end in .gov or .mil. Before sharing sensitive information, make sure you’re on a federal government site.
The site is secure. The https:// ensures that you are connecting to the official website and that any information you provide is encrypted and transmitted securely.
- Publications
- Account settings
- My Bibliography
- Collections
- Citation manager
Save citation to file
Email citation, add to collections.
- Create a new collection
- Add to an existing collection
Add to My Bibliography
Your saved search, create a file for external citation management software, your rss feed.
- Search in PubMed
- Search in NLM Catalog
- Add to Search
Revisiting the central dogma in the 21st century
Affiliation.
- 1 Department of Biochemistry and Molecular Biology, University of Chicago, Gordon Center for Integrative Science, Chicago, IL 60637, USA. [email protected]
- PMID: 19845625
- DOI: 10.1111/j.1749-6632.2009.04990.x
Since the elaboration of the central dogma of molecular biology, our understanding of cell function and genome action has benefited from many radical discoveries. The discoveries relate to interactive multimolecular execution of cell processes, the modular organization of macromolecules and genomes, the hierarchical operation of cellular control regimes, and the realization that genetic change fundamentally results from DNA biochemistry. These discoveries contradict atomistic pre-DNA ideas of genome organization and violate the central dogma at multiple points. In place of the earlier mechanistic understanding of genomics, molecular biology has led us to an informatic perspective on the role of the genome. The informatic viewpoint points towards the development of novel concepts about cellular cognition, molecular representations of physiological states, genome system architecture, and the algorithmic nature of genome expression and genome restructuring in evolution.
PubMed Disclaimer
Similar articles
- A 21st century view of evolution: genome system architecture, repetitive DNA, and natural genetic engineering. Shapiro JA. Shapiro JA. Gene. 2005 Jan 17;345(1):91-100. doi: 10.1016/j.gene.2004.11.020. Epub 2005 Jan 4. Gene. 2005. PMID: 15716117 Review.
- The evolution of plant genomes: scaling up from a population perspective. Flowers JM, Purugganan MD. Flowers JM, et al. Curr Opin Genet Dev. 2008 Dec;18(6):565-70. doi: 10.1016/j.gde.2008.11.005. Epub 2009 Jan 7. Curr Opin Genet Dev. 2008. PMID: 19131240 Review.
- Modularity: a new perspective in biology. Dani DN, Sainis JK. Dani DN, et al. Indian J Biochem Biophys. 2007 Jun;44(3):133-9. Indian J Biochem Biophys. 2007. PMID: 17650580 Review.
- All genomes great and small. Knight J. Knight J. Nature. 2002 May 23;417(6887):374-6. doi: 10.1038/417374a. Nature. 2002. PMID: 12024187 No abstract available.
- [The molecular logic of life--a half century of development in biochemistry and molecular biology]. Kovacević Z. Kovacević Z. Med Pregl. 1997 Nov-Dec;50(11-12):429-32. Med Pregl. 1997. PMID: 9471498 Croatian.
- An Innovative Multi-Omics Model Integrating Latent Alignment and Attention Mechanism for Drug Response Prediction. Chen HO, Cui YC, Lin PC, Chiang JH. Chen HO, et al. J Pers Med. 2024 Jun 27;14(7):694. doi: 10.3390/jpm14070694. J Pers Med. 2024. PMID: 39063948 Free PMC article.
- How advancements in molecular biology impact education and training. Walther RE, Hrabak M, Bernstein DA. Walther RE, et al. J Microbiol Biol Educ. 2024 Aug 29;25(2):e0006124. doi: 10.1128/jmbe.00061-24. Epub 2024 Jul 8. J Microbiol Biol Educ. 2024. PMID: 38975770 Free PMC article.
- "Molecular Biology"-Pleonasm or Denotation for a Discipline of Its Own? Reflections on the Origins of Molecular Biology and Its Situation Today. Greslehner GP. Greslehner GP. Biomolecules. 2023 Oct 12;13(10):1511. doi: 10.3390/biom13101511. Biomolecules. 2023. PMID: 37892193 Free PMC article. Review.
- Integrative proteomics and metabolomics profiling to understand the biochemical basis of beef muscle darkening at a slightly elevated pH. Kiyimba F, Cassens D, Hartson SD, Rogers J, Habiger J, Mafi GG, Ramanathan R. Kiyimba F, et al. J Anim Sci. 2023 Jan 3;101:skac376. doi: 10.1093/jas/skac376. J Anim Sci. 2023. PMID: 36638080 Free PMC article.
- Chromosome Changes in Soma and Germ Line: Heritability and Evolutionary Outcome. Bakloushinskaya I. Bakloushinskaya I. Genes (Basel). 2022 Mar 28;13(4):602. doi: 10.3390/genes13040602. Genes (Basel). 2022. PMID: 35456408 Free PMC article. Review.
- Search in MeSH
LinkOut - more resources
Full text sources.
- Ovid Technologies, Inc.
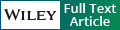
- Citation Manager
NCBI Literature Resources
MeSH PMC Bookshelf Disclaimer
The PubMed wordmark and PubMed logo are registered trademarks of the U.S. Department of Health and Human Services (HHS). Unauthorized use of these marks is strictly prohibited.
- Molecular Biology
The New Central Dogma of Molecular Biology
- This person is not on ResearchGate, or hasn't claimed this research yet.

- Missouri Baptist University

Abstract and Figures

Discover the world's research
- 25+ million members
- 160+ million publication pages
- 2.3+ billion citations

- Susan M Rosenberg

- Jonathan O Nelson
- George J Watase
- Yukiko M Yamashita
- NUCLEIC ACIDS RES

- Tsutomu Katayama
- Hiroko Tsujimoto

- Xiaoyang Dou
- Chuanyuan Chen

- TRENDS GENET
- Natalie Warsinger-Pepe

- Takehiko Kobayashi
- Jorge Ruiz-Orera
- Denis Noble
- Recruit researchers
- Join for free
- Login Email Tip: Most researchers use their institutional email address as their ResearchGate login Password Forgot password? Keep me logged in Log in or Continue with Google Welcome back! Please log in. Email · Hint Tip: Most researchers use their institutional email address as their ResearchGate login Password Forgot password? Keep me logged in Log in or Continue with Google No account? Sign up
Thank you for visiting nature.com. You are using a browser version with limited support for CSS. To obtain the best experience, we recommend you use a more up to date browser (or turn off compatibility mode in Internet Explorer). In the meantime, to ensure continued support, we are displaying the site without styles and JavaScript.
- View all journals
- Explore content
- About the journal
- Publish with us
- Sign up for alerts
- Published: 08 August 1970
Central Dogma of Molecular Biology
- FRANCIS CRICK 1
Nature volume 227 , pages 561–563 ( 1970 ) Cite this article
44k Accesses
1543 Citations
125 Altmetric
Metrics details
The central dogma of molecular biology deals with the detailed residue-by-residue transfer of sequential information. It states that such information cannot be transferred from protein to either protein or nucleic acid.
This is a preview of subscription content, access via your institution
Access options
Subscribe to this journal
Receive 51 print issues and online access
185,98 € per year
only 3,65 € per issue
Buy this article
- Purchase on SpringerLink
- Instant access to full article PDF
Prices may be subject to local taxes which are calculated during checkout
Similar content being viewed by others
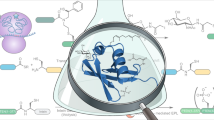
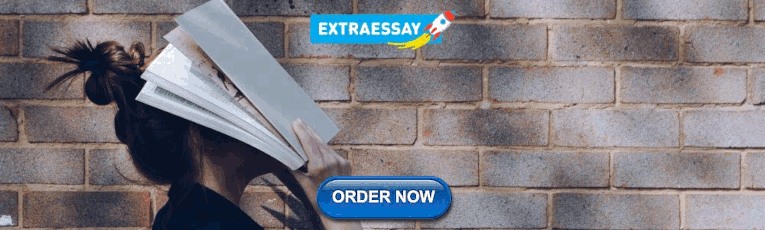
Deciphering protein post-translational modifications using chemical biology tools
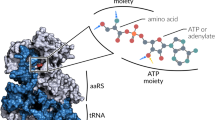
The structural basis of the genetic code: amino acid recognition by aminoacyl-tRNA synthetases
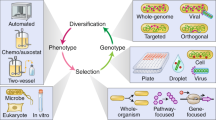
The developing toolkit of continuous directed evolution
Nature , 226 , 1198 (1970).
Temin, H. M., and Mizutani, S., Nature , 226 , 1211 (1970). This article contains the references to Dr Temin's earlier work dating back to 1963.
Article ADS CAS Google Scholar
Baltimore, D., Nature , 226 , 1209 (1970). See also the brief account of Spiegelman's recent work on page 1202.
Crick, F. H. C., in Symp. Soc. Exp. Biol., The Biological Replication of Macromolecules , XII, 138 (1958).
Google Scholar
Commoner, B., Nature , 220 , 334 (1968).
Fleischman, P., Nature , 225 , 30 (1970).
Hershey, A. D., Nature , 226 , 697 (1970).
McCarthy, B., and Holland, J. J., Proc. US Nat. Acad. Sci. , 54 , 880 (1965).
Gibbons, R. A., and Hunter, G. D., Nature , 215 , 1041 (1967).
Griffith, J. S., Nature , 215 , 1043 (1967).
Download references
Author information
Authors and affiliations.
MRC Laboratory of Molecular Biology, Hills Road, Cambridge, CB2 2QH
FRANCIS CRICK
You can also search for this author in PubMed Google Scholar
Rights and permissions
Reprints and permissions
About this article
Cite this article.
CRICK, F. Central Dogma of Molecular Biology. Nature 227 , 561–563 (1970). https://doi.org/10.1038/227561a0
Download citation
Received : 08 July 1970
Issue Date : 08 August 1970
DOI : https://doi.org/10.1038/227561a0
Share this article
Anyone you share the following link with will be able to read this content:
Sorry, a shareable link is not currently available for this article.
Provided by the Springer Nature SharedIt content-sharing initiative
This article is cited by
The hidden impact of in-source fragmentation in metabolic and chemical mass spectrometry data interpretation.
- Martin Giera
- Aries Aisporna
- Gary Siuzdak
Nature Metabolism (2024)
Advances in brain epitranscriptomics research and translational opportunities
- Valentina V. Ignatova
- Hongjun Song
Molecular Psychiatry (2024)
Cell-type specific and differential expression of LINC-RSAS long noncoding RNA declines in the testes during ageing of the rat
- Ajay Kumar Danga
- Sukhleen Kour
- Pramod C. Rath
Biogerontology (2024)
Whole Blood Transcriptome Analysis in Patients with Trigeminal Neuralgia: a Prospective Clinical Study
Journal of Molecular Neuroscience (2024)
Liver cancer-specific mutations in functional domains of ADAR2 lead to the elevation of coding and non-coding RNA editing in multiple tumor-related genes
Molecular Genetics and Genomics (2024)
By submitting a comment you agree to abide by our Terms and Community Guidelines . If you find something abusive or that does not comply with our terms or guidelines please flag it as inappropriate.
Quick links
- Explore articles by subject
- Guide to authors
- Editorial policies
Sign up for the Nature Briefing newsletter — what matters in science, free to your inbox daily.


An official website of the United States government
The .gov means it’s official. Federal government websites often end in .gov or .mil. Before sharing sensitive information, make sure you’re on a federal government site.
The site is secure. The https:// ensures that you are connecting to the official website and that any information you provide is encrypted and transmitted securely.
- Publications
- Account settings
Preview improvements coming to the PMC website in October 2024. Learn More or Try it out now .
- Advanced Search
- Journal List
- J Zhejiang Univ Sci B
- v.15(5); 2014 May
Transcription: the epicenter of gene expression
The complexity of a living organism is not driven by gene number but gene regulation. Controlling which genes to express and to what extent dictates the subsequent cell identity. Transcription, the critical initial stage in gene expression, is regulated delicately to maintain the cell status. Recent developments in the genomic approaches provided unparalleled coverage of the study of transcription. Still, basic molecular biology and biochemistry are providing mechanistic insights into how the regulation is achieved. In this feature “Regulation of transcription: mechanisms and biological functions”, the latest advances in epigenetics, mRNA processing, RNA quality control, and human immunodeficiency virus (HIV) transactivation are discussed.
Control transcription, control gene expression
A gene has been traditionally viewed as the basic molecular unit of heredity (Crick, 1958 ; 1970 ). In the form of DNA or RNA, it carries the raw genetic information that can be turned into functional products, usually proteins. However, the number of genes does not reflect the complexity of the organism. For example, a human has about 20 000 protein-coding genes, which is ∼6000 more than a fruit fly, ∼2000 more than Caenorhabditis elegans , and ∼14 000 more than budding yeast, but ∼10 000 less than a lab mouse, ∼5000 less than the model plant Arabidopsis , and ∼17 000 less than rice. Clearly, the level of complexity of the organism is achieved by regulating available genes, not simply by introducing more genes.
The central dogma of gene expression includes two sequential steps: transcription (DNA to RNA) and translation (RNA to protein). Transcription is the key step that controls the “on and off” of genes and subsequently underlines the identity and the status of the cell (Young, 2011 ; Lee and Young, 2013 ). For example, when I compare a string of my hair and my finger tip, they appear so different, as if they are made from different genetic materials. However, the fact is that all the different tissues and cells in my body contain the same DNA and it is the differential expression profiles that created the functional diversity. Understanding the mechanism of gene expression will help us to understand the formation and evolution of life and to find possible cures for diseases.
In this issue
We have invited scientists on the leading edge in their respective fields to share their expertise and perspectives. Ma et al. ( 2014 ) focused on the mechanism that opens and closes specific regions in the chromatin. The issue starts with the packaging of human DNA. If stretched out, the total length of DNA from a human cell is about 3 m. In a living cell, DNA is packaged into a highly compact form called chromatin. Genes are not active when they are in the compacted form. Factors involved in opening and closing specific regions control the accessibility of genes (Guertin and Lis, 2012 ). A set of factors called polycomb group (PcG) proteins is involved in modifying the chromatin structure and subsequently regulating a large number of genes. The dynamic nature of the multisubunit protein complex and its complex functional impact are described in detail.
Gene regulation does not stop at finding the gene. The transcription machinery finds an accessible promoter and assembles a multi-megadalton protein complex to initiate transcription (Kornberg, 2007 ). As soon as the nascent transcript emerges from the body of RNA polymerase II (Pol II), the enzyme that transcribes protein coding genes, the RNA is protected by the addition of a 5′ cap (Shatkin, 1976 ). A text-book would describe the capping to be a unanimous phenomenon that takes place on every molecule of the RNA transcripts. However, Zhai and Xiang ( 2014 ) summarize the recent discovery that improperly capped RNA is subjected to quality control and degradation. Previously, removal of the cap and subsequent RNA degradation are thought to only take place after the RNA is exported to the cytoplasm and translation has been initiated. This discovery changed the way that mRNA capping and de-capping are viewed in time and space.
RNA degradation or turnover has a significant place in gene expression (Houseley and Tollervey, 2009 ). Compared to DNA, RNA is a very short-lived species in cells. Its relatively rapid turnover provides the flexibility for organisms to react to the environment and adapt quickly to a change in gene expression profile. Historically, mRNA degradation is only thought to occur once at least a round of translation has taken place. Liu H. et al. ( 2014 ) provide an updated review of the many RNA degradation pathways taking place in the nucleus. Degrading nascent RNA transcripts in the nucleus has been viewed as a quality control mechanism to quickly get rid of undesired RNAs before they are exported to cytoplasm and waste the effort of ribosomes in translating wrong proteins.
The start of transcription relies on promoters. The termination signal typically relies on sequence information at the end of the genes (Richard and Manley, 2009 ; Guo et al., 2011 ). Davis and Shi ( 2014 ) review the current understanding of the sequence requirements and the protein factors involved in this process called mRNA 3′ end processing. It cuts the RNA off the moving Pol II and adds a stretch of adenines to the RNA. The sequence driving this process is called polyadenylation signal or polyA signal (Proudfoot, 1991 ). It has been known that the promiscuous nature of the polyA signal sequences leads to the selective use of multiple polyA signals (Tian and Manley, 2013 ). This feature, described as alternative polyadenylation, has been linked with many aspects of gene expression: pre-mature termination, RNA splicing, RNA stability and expression level (Mayr and Bartel, 2009 ). However, the exact mechanism that dictates alternative polyA signal usage is unclear. This article also provides a simple yet broadly applicable model to characterize how the choice among many polyA signals is made.
The process of making RNA nucleotide by nucleotide, transcription elongation, is tightly controlled and interlaced with all above events. Indeed, elongation control is a critical step in determining transcriptional output. Instead of looking for an open promoter to start transcription, an initiated and paused Pol II can be found on all expressed human genes (Core et al., 2012 ; Kwak et al., 2013 ). This paused Pol II population serves as a reserve for transcription to rapidly respond to activation signals (Guo and Price, 2013 ). Upon activation, positive transcription elongation factor P-TEFb triggers the fast-paced productive elongation that leads to mRNA production. P-TEFb is therefore seen as the key for rapid amplification of gene expression. Indeed, this is the exact factor virus hijack to promote the activation of themselves (Zhou et al., 2012 ). Liu R.D. et al. ( 2014 ) provide the latest understanding of factors involved in the transition into productive elongation and how HIV finds its niche to achieve replication effectively and in a deadly way.
On the journal
The Journal of Zhejiang University-SCIENCE B (Biomedicine & Biotechnology) has been at the frontier of peer-reviewed scientific journals based in China. Since its establishment in 2005, the editorial office has been dedicated to publishing high quality and high impact research and review articles.
In this special issue, we invited primary authors of recent high impact research articles to provide the most up-to-date view of their area of expertise. The studies they led were groundbreaking and provided significant milestones for their field. Justly, the invitees have all recently started their own labs with generous funding. Demonstrating their dedication to nurturing future scientists, they have all invited their trainees and colleagues to join in the adventure of composing the articles. We believe these cutting edge articles will promote discussions among established scientists and benefit the training of junior researchers.
Introducing the guest editor: Jiannan GUO received his Bachelor’s degree at Northeast Forestry University in China and his Master’s degree at the University of Bath in the United Kingdom. He then worked with Dr. Saverio BROGNA at the University of Birmingham in the United Kingdom for his Ph.D. Currently he is a postdoctoral fellow in the Price lab at the University of Iowa in the United States. Generally he is interested in the regulation of gene expression, and his expertise includes developing in vitro transcription assays to characterize factors involved in Pol II transcription elongation.

Jiannan GUO
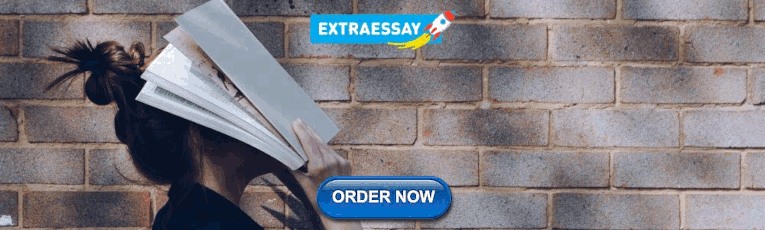
IMAGES
COMMENTS
Specifically, basic physiochemical research data does not support the assumption that peptides automatically fold into stable protein states containing the information encoded by their respective genes. Consequently, it is not just that the Central Dogma does not hold in the strict sense prohibiting a direct biomolecular flow of protein to DNA.
Sixty-three years ago, Francis Crick gave a lecture in which he presented the diagram called the "central dogma.". This dogma states that the transfer of information from DNA to DNA/RNA, or from nucleic acid to protein, may be possible, but the transfer from protein to protein or protein to nucleic acid is impossible (Cobb, 2017; CRICK, 1957).
The Sequence Hypothesis defines biological information transfer as the residue-by-residue transfer of sequence information between nucleic acids and to proteins. This is commonly summarized as DNA RNA protein and is colloquially referred to as the Central Dogma. More specifically, however, the Central Dogma expounded by Crick included a ...
The purpose of the present work is to emphasize the contribution of proteomics and systems biology to extending the central dogma (Figure 1). Whenever possible, studies from the cardiovascular literature are used to highlight conceptual and technical breakthroughs. Excellent reviews exist on novel means of quantifying the proteome, 8, 9 methods ...
The central dogma. The most widely known of the powerful statements made by Crick in his lecture related to the flow of information between genes and proteins [].He had been musing about this for some time and in October 1956 wrote a set of notes entitled 'Ideas on protein synthesis' that took up 2 pages [].The second sentence of this document read, "The Central Dogma: 'Once ...
When the central dogma was originally proposed, it was formulated in chemical terms as the irreversible flow of information from nucleic acids to proteins . Accordingly, the chemical properties of proteins have been considered integral to the central dogma . By contrast, the present study formulates the central dogma in functional terms, as the ...
Abstract. Since the elaboration of the central dogma of molecular biology, our understanding of cell function and genome action has benefited from many radical discoveries. The discoveries relate to interactive multimolecular execution of cell processes, the modular organization of macromolecules and genomes, the hierarchical operation of ...
Instead, this paper sought to evaluate the Dogma's practical significance (or usefulness) to biology, by considering the four major approaches for determining the usefulness of a scientific principle. We saw that the Central Dogma is not of practical significance to biology as a Galilean Idealization, as a tool for generating predictions at ...
A 'dogma' is defined as a principle or set of principles laid down by an authority and held to be incontrovertibly true. However, immunology is an experimental science and rarely if ever can ...
In principle, a violation of the Central Dogma could transpire through synthetic biology or by natural occurrence. To address these possibilities, we draw insights from existing modes of information transfer in protein synthesis and from synthetic C lustered R egularly- I nterspaced S hort P alindromic R epeats (CRISPR) gene-editing.
The 13th annual Chemical Biology Symposium at Yale University in May 2010 highlighted recent progress in the field, enriching our understanding of the central dogma and paving the way for future research at the interface between chemistry and biology. Two exciting talks at the symposium — one by Dr. Anna Pyle (Yale University) on self ...
The central dogma of molecular biology formulated by Francis Crick has greatly influenced our scientific research and perspective of life. However, it fails to adequately account for the following ...
The Elaboration of the Central Dogma. DNA to RNA to Protein: This is the Central Dogma, a term coined by Francis Crick in 1958. Since the discovery of the helical structure of DNA, scientists ...
The Function of Dogma in Scientific Research. THOMAS S. KUHN. e Function of Dogma in Scientific Research 1At some point in his or her career every mem that phrase may mean, the individual scientist ber of this Symposium. has, I feel sure, been ex is very often not. Whether rus work is predom posed to the image of the scientist as the inantly ...
The "Central Dogma" of molecular biology hypothesizes the impossibility of converting the amino acid sequence of a protein back into a nucleic acid sequence. (Nota bene: I cringe having to write "Dogma" in a science context, but the misnomer has stuck.) In his paper, Eugene Koonin convincingly argues that the exclusion of reverse ...
The limitation of the Central Dogma is not that it is wrong, but rather the dogma limits our minds. The entire focus of Crick's statements was on the transmission of information. However, the transmission of information explains nothing beyond those limited goals, albeit essential, but limits our horizons in appreciating not only the complex ...
Research Paper. The central dogma, "GMO" and defective epistemology. Giovanni Tagliabue Carugo, Como, Italy Correspondence [email protected]. Pages 209-215 | Received 24 Aug 2017, Accepted 10 Nov 2017, Published online: 03 Jan 2018. ... misunderstanding the Central Dogma. ...
The purpose of the present work is to emphasize the contribution of proteomics and systems biology to extending the central dogma ( Figure 1 ). Whenever possible, studies from the cardiovascular literature are used to highlight conceptual and technical breakthroughs. Excellent reviews exist on novel means of quantifying the proteome, 8, 9 ...
In this review, we explore aspects of this synthesis paradigm as applied to the chemistry and function of nucleic acids in biological systems and beyond, specifically, in genome resynthesis, synthetic genetics (i.e., the expansion of the genetic alphabet, of the genetic code, and of the chemical make-up of genetic systems), and the elaboration ...
1) represent the impossible transfers postulated by the central dogma. They are the three possible arrows starting from protein. little analysis showed that the transfer could be divided roughly ...
The expression "Genetically Modified Organisms" ("GMOs") was coined to identify a category of agricultural products; the term cov-ers microorganisms, animals and plants, food (e.g. maize, soybeans, rice, eggplants) and non-food (e.g. cotton, flax), which have had their genome engineered - i.e. their DNA "recombined" - by ...
This month marks the 60th anniversary of one of the most significant lectures in the history of biology. It was given on 19 September 1957 by Francis Crick as part of a Society for Experimental Biology symposium on the Biological Replication of Macromolecules, held at University College London. Originally entitled 'Protein synthesis,' the ...
The central dogma of gene expression includes two sequential steps: transcription (DNA to RNA) and translation (RNA to protein). Transcription is the key step that controls the "on and off" of genes and subsequently underlines the identity and the status of the cell (Young, 2011; Lee and Young, 2013). For example, when I compare a string of ...