- Primary Hub
- Art & Design
- Design & Technology
- Health & Wellbeing
- Secondary Hub
- Citizenship
- Primary CPD
- Secondary CPD
- Book Awards
- All Products
- Primary Products
- Secondary Products
- School Trips
- Trip Directory
- Trips by Subject
- Trips by Type
- Trips by Region
- Submit a Trip Venue
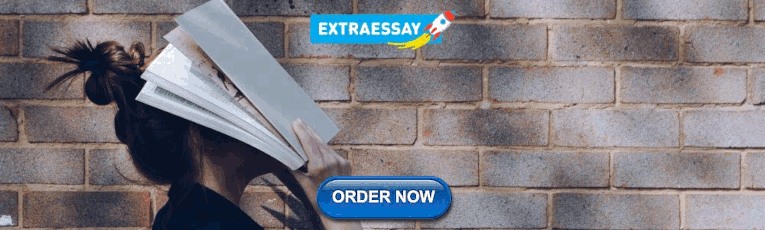
Trending stories
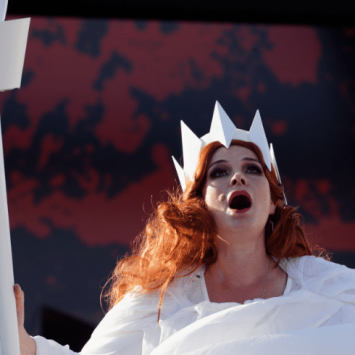
Top results
- Primary Maths How To Use Stem Sentences In Maths
Primary maths – How to use stem sentences in maths
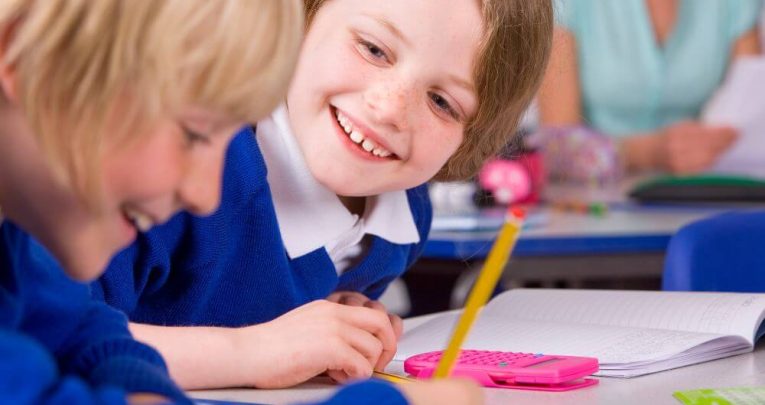
They’re not just for English lessons, says teacher John Bee…
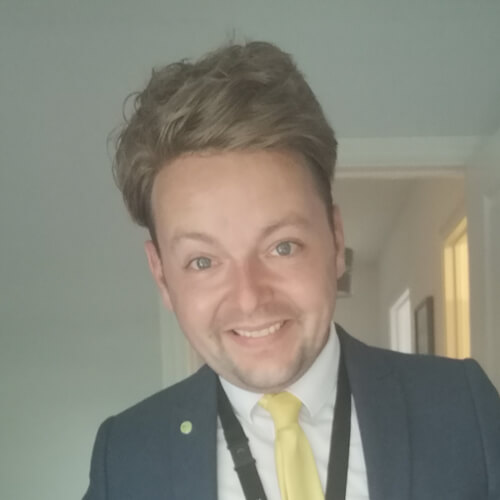
A stem sentence is nothing new in primary schools.
They support children (and teachers) to start sentences, rather than staring at a blank page. In English, stem sentences can be an effective strategy to structure writing. In maths they can be used in dynamic ways to support teaching and learning.
Maths lessons which include carefully chosen representations expose mathematical structures. A stem sentence can work alongside a mathematical representation by complementing it and revealing the structure. For example, the structure of fractions being part of a whole can be explored using part-whole or bar model representations.
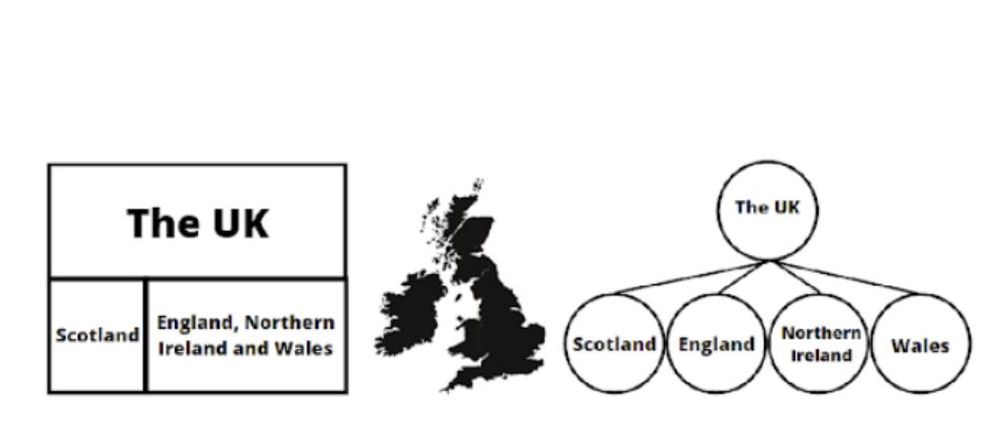
Stem sentence: If the UK is the whole, ______ is a part of the whole.
In the above example, the stem sentence supports the representations by deepening the structure of fractions being part of a whole. It exposes the relative size of fractions and can lead to rich discussions.
Maths has a highly specialised and technical language which needs to be explicitly taught. How many of us have asked children to explain their reasoning to be met with “Erm, well…” or other vague responses? Stem sentences can scaffold children to develop their reasoning and explanations by using the language of mathematics.
Put simply, mathematics requires children to talk like a mathematician. Just as we use the words ‘split digraphs’ and ‘phonemes’ in KS1, maths has its own semantics and culture. An effective stem sentence allows children to practise reasoning by changing variables and using precise and technical vocabulary.
Stem sentences can also be used to arrive at a generalisation or general rule. For example, when children in UKS2 build on their understanding of ‘factor x factor = product’, they explore how many factors a number has.
Children may notice particular features of some numbers (11, 13, 17, for example) and wonder why they only have two factors each. They can then be guided towards a generalisation: numbers that have exactly two factors are called prime numbers.
Test this key structure by using counters or factor wheels to show examples of prime numbers. Children may ask if all prime numbers are odd or always one more/less than a composite number (therefore, there aren’t any consecutive prime numbers, apart from two and three, which are prime factors).
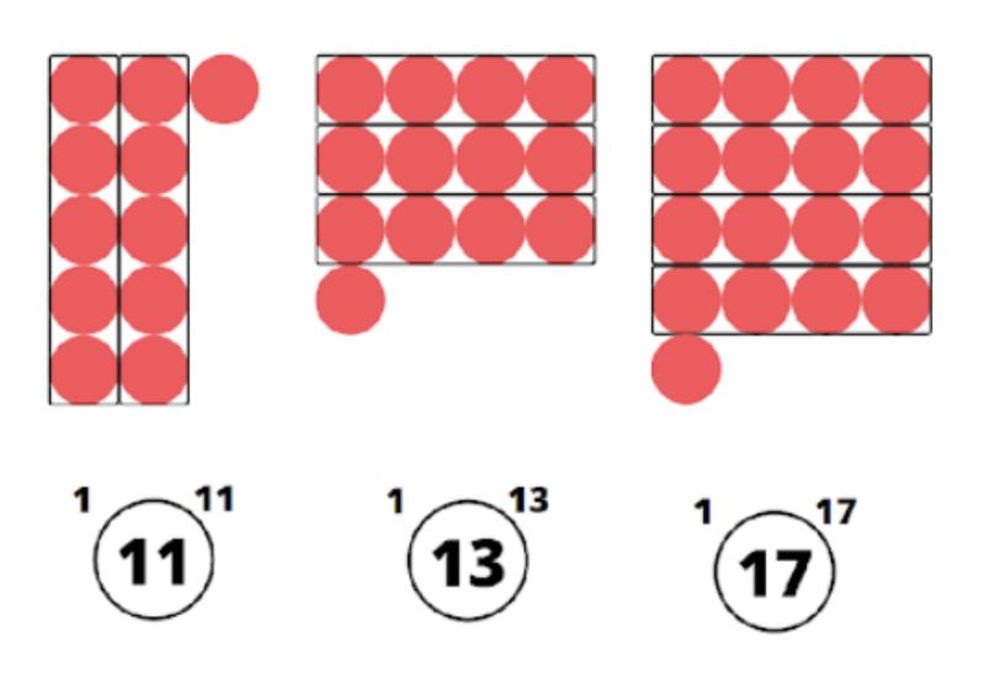
Strategies and pedagogical choices are important when developing stem sentences. An ‘I say, you say, we say’ approach can be effective: firstly, model the stem sentence so that pupils can hear mathematical vocabulary used accurately and correctly, then invite a selection of children to say the stem sentence, allowing them to rehearse reasoning.
Finally, invite the whole class to say the stem sentence together. In doing so, you’re explicitly teaching children to reason, deepening their understanding of mathematical structures and avoiding vague responses.
Of course, there’s still a place for children to construct their understanding, think out loud and offer unstructured responses to make sense and meaning of new concepts. Stem sentences aim to support fundamental mathematical structures which can be built on as children progress through their education.
To deepen children’s conceptual understanding of mathematical structures, change the variables in a stem sentence, or its context. Look at the examples below:
If the UK is the whole, ______ is a part of the whole. If ______ is the whole, England is part of the whole. If the bus is the whole, ______ is a part of the whole. If ______ is the whole, the windows are a part of the whole.
I’m often asked for a ‘bank’ of sentence stems which cover the curriculum. I feel this is the wrong approach for a few reasons: it limits teacher agency; it limits lesson design; it discourages teachers from engaging in discussions and planning around key concepts in maths.
Instead, coherent lesson design, representations through carefully selected models and images, mathematical thinking and vocabulary, fluency and variation can all be supported by the inclusion of stem sentences to draw attention to essential concepts in maths.
John Bee is head of KS2 and maths leader at a primary school. Follow him on Twitter at @mrbeeteach. Visit his website here .
Sign up to our newsletter
You'll also receive regular updates from Teachwire with free lesson plans, great new teaching ideas, offers and more. (You can unsubscribe at any time.)
Which sectors are you interested in?
Early Years
Thank you for signing up to our emails!
You might also be interested in...
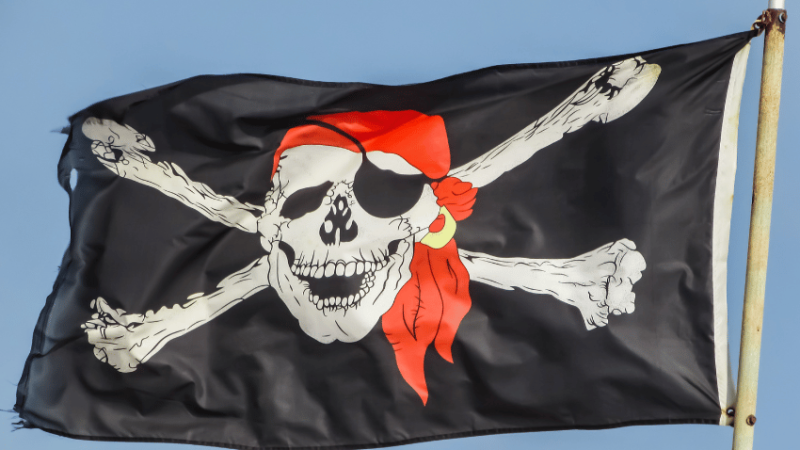
Why join Teachwire?
Get what you need to become a better teacher with unlimited access to exclusive free classroom resources and expert CPD downloads.
Exclusive classroom resource downloads
Free worksheets and lesson plans
CPD downloads, written by experts
Resource packs to supercharge your planning
Special web-only magazine editions
Educational podcasts & resources
Access to free literacy webinars
Newsletters and offers
Create free account
By signing up you agree to our terms and conditions and privacy policy .
Already have an account? Log in here
Thanks, you're almost there
To help us show you teaching resources, downloads and more you’ll love, complete your profile below.
Welcome to Teachwire!
Set up your account.
Lorem ipsum dolor sit amet consectetur adipisicing elit. Commodi nulla quos inventore beatae tenetur.
I would like to receive regular updates from Teachwire with free lesson plans, great new teaching ideas, offers and more. (You can unsubscribe at any time.)
Log in to Teachwire
Not registered with Teachwire? Sign up for free
Reset Password
Remembered your password? Login here

- WordPress.org
- Documentation
- Learn WordPress
- Members Newsfeed
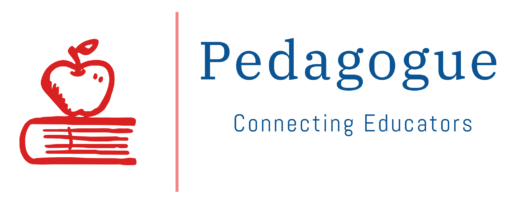
How to Use Sentence Stems (Plus 60+ Examples for Every Subject)
- EdTech & Innovation
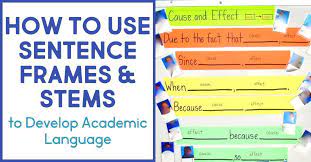
Introduction
Sentence stems are an effective tool that learners can use to develop their communication skills, improve comprehension, and enhance critical thinking. In this article, we will discuss how to use sentence stems and provide over 60 examples that can be personalized and adapted as per the specific learning context.
What are Sentence Stems?
Sentence stems are predetermined phrases designed to facilitate responses in discussions, writing assignments, or self-reflection. They allow learners to organize their thoughts and express themselves effectively while promoting quality engagement and deeper thinking across various subjects.
How to Use Sentence Stems
1. Choose relevant stems: Select stems that fit your subject area or learning objectives. For instance, if the goal is to encourage critical analysis in literature, choose sentence stems that require learners to analyze texts by comparing themes, characters, or plots.
2. Introduce them gradually: Introduce sentence stems progressively so that students have time to practice using them naturally and effectively. This can be done by incorporating one or two at a time into discussions or activities.
3. Model using sentence stems: Demonstrate how sentence stems can be used by providing examples during class sessions and incorporating them into your feedback on student work.
4. Encourage students’ use: Create opportunities for students to use sentence stems during group discussions or individual writing tasks. Utilize prompts that encourage reflection or debate requiring the use of these predetermined phrases.
5. Track progress: Monitor the students’ use of sentence stems over time and provide feedback and reinforcement on areas where they may need further assistance.
60+ Sentence Stem Examples for Every Subject
Mathematics:
1. “I noticed a pattern when I saw…”
2. “The formula changes if we…”
3. “My solution is different because…”
4. “Based on my observation, I predict…”
5. “This experiment demonstrated…”
6. “One way to classify this organism is…”
Social Studies:
7. “An important event in this time period was…”
8. “This primary source reveals…”
9. “A significant cultural difference is…”
Literature:
10. “A central theme in this story appears to be…”
11. “The author’s use of symbolism is evident when…”
12. “An interesting character trait of (character) is…”
13. “In my introduction, I will discuss…”
14. “To support my argument, I will provide evidence from…”
15. “My conclusion effectively summarizes my main points by…”
Reading Comprehension:
16. “The purpose of the author is to convey that…”
17. “The passage suggests that the character feels…”
18. “A significant turning point in the text occurs when…”
Critical Thinking:
19. “An alternative perspective on this issue might be…”
20. “This argument could be supported by considering…”
21. “One possible implication of this idea is…”
Self-Reflection:
22. “I feel more confident in my understanding of this subject because…”
23. “One aspect that challenged me during this exercise was…”
24. “To improve, I need to focus more on…”
Sentence stems provide a supporting structure that enables students to articulate their thoughts meaningfully and foster critical thinking skills across varied subjects and learning contexts. By incorporating sentence stems into your teaching methodology, you can help your learners become more confident communicators and reflective thinkers, and enhance their overall academic development.

Related Articles
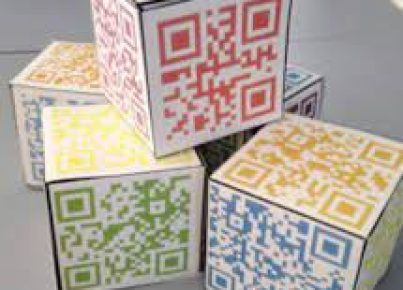
Incorporating technology in the classroom is an effective way to engage students…

In the educational arena, Bump It Up walls have become a popular…
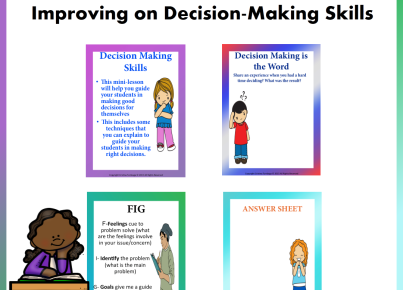
Decision making is a critical life skill that allows individuals to navigate…
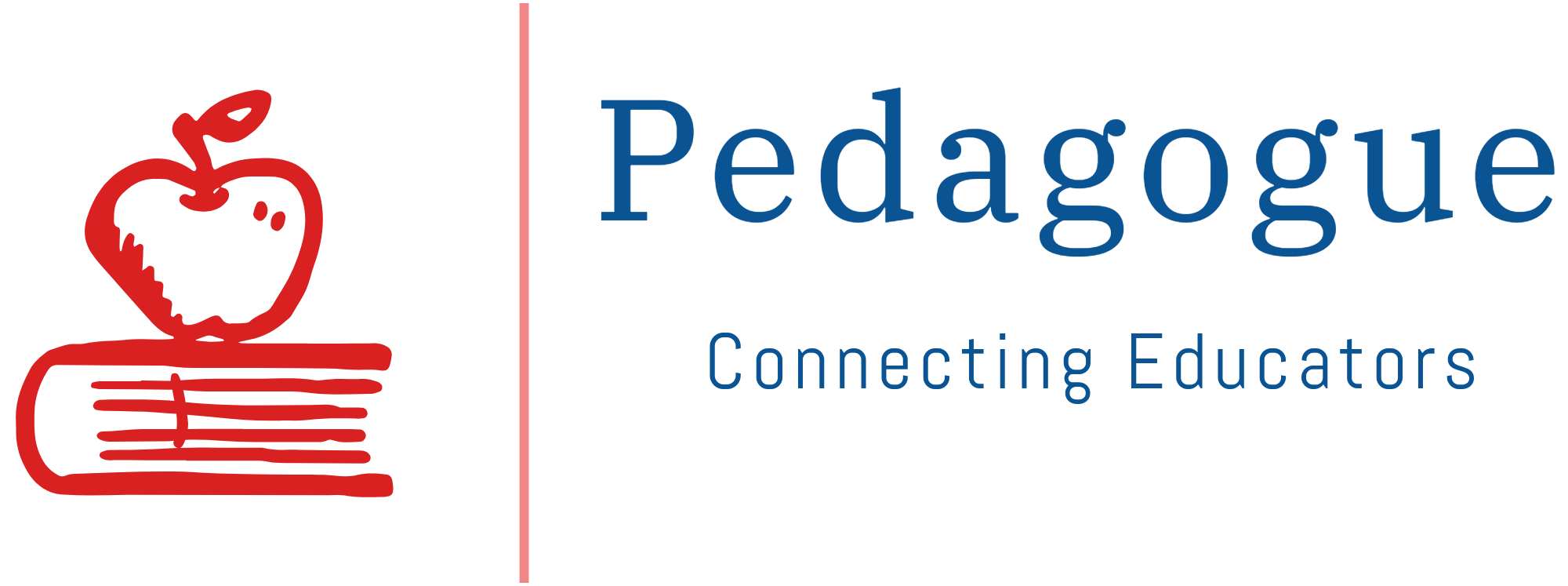
Pedagogue is a social media network where educators can learn and grow. It's a safe space where they can share advice, strategies, tools, hacks, resources, etc., and work together to improve their teaching skills and the academic performance of the students in their charge.
If you want to collaborate with educators from around the globe, facilitate remote learning, etc., sign up for a free account today and start making connections.
Pedagogue is Free Now, and Free Forever!
- New? Start Here
- Frequently Asked Questions
- Privacy Policy
- Terms of Service
- Registration
Don't you have an account? Register Now! it's really simple and you can start enjoying all the benefits!
We just sent you an Email. Please Open it up to activate your account.
I allow this website to collect and store submitted data.
- Skip to primary navigation
- Skip to main content
- Skip to primary sidebar
- Skip to footer
Don't Miss a Post! Subscribe
- Guest Posts

- Educational AI
- Edtech Tools
- Edtech Apps
- Teacher Resources
- Special Education
- Edtech for Kids
- Buying Guides for Teachers

Educators Technology
Innovative EdTech for teachers, educators, parents, and students
Sentence Stems Activities for Classroom
By Med Kharbach, PhD | Last Update: May 9, 2024

Sentence stems, those handy linguistic scaffolding tools, have the power to transform the classroom atmosphere from one of hesitation to one of vibrant, thoughtful discourse. By providing students with these structured starting points, we’re not just assisting them in constructing sentences; we’re inviting them to think deeply, express themselves clearly, and engage actively with the material in front of them.
The beauty of sentence stems lies in their versatility—they’re like Swiss Army knives for language skills, critical thinking, and engagement. Imagine a classroom where every student feels empowered to speak, where the blank page becomes less daunting, and where complex ideas are unpacked with ease. This is the power of integrating sentence stems into classroom activities.
Whether it’s through the reflective practice of journaling, the dynamic exchange of structured debates, or the careful consideration of peer feedback, sentence stems offer students a structured way to navigate their learning journey.
Related: Sentence Stems for Learning English
As I mentioned before, integrating sentence stems into classroom activities not only aids in developing students’ language skills but also enhances their critical thinking and engagement. Here are diverse activities where sentence stems can be effectively utilized, offering students structured ways to express their ideas and interact with content:
1. Journaling or Reflective Writing

Encourage students to use sentence stems for daily or weekly journal entries, reflecting on what they’ve learned, challenges they’ve faced, or goals they’ve set. This practice can deepen their understanding and help them articulate personal reflections more clearly.
- “Today, I felt ___ because…”
- “One challenge I faced was ___ and I overcame it by…”
- “I achieved my goal of ___ by…”
- “Something new I learned today was ___ and it matters because…”
- “My plan for improving ___ is to…”
2. Structured Debate

Organize debates on relevant topics where students use sentence stems to present arguments, rebuttals, and closing statements. This activity can help students learn to construct persuasive arguments and respond to opposing views thoughtfully.
- “My argument in favor of ___ is supported by ___ because…”
- “Contrary to ___, my viewpoint is ___ due to…”
- “An important consideration is ___ which suggests that…”
- “To rebut the opposing view, I argue that ___ because…”
- “In conclusion, ___ is preferable because…”
3. Peer Feedback Sessions

During peer review sessions, provide students with sentence stems to offer constructive feedback on each other’s work. Stems can guide students to comment on specific aspects of the work, ensuring feedback is focused and helpful.
- “A strength in your work is ___ because…”
- “Consider improving ___ by ___ because…”
- “Your explanation of ___ was very clear because…”
- “One suggestion for enhancing your argument is to…”
- “I really appreciated ___ in your presentation because…”
4. Book Talks or Literature Circles

In discussions about books or articles, use sentence stems to guide students’ thoughts on themes, characters, and plots. This can encourage deeper analysis and help students articulate their interpretations and reactions.
- “The character of ___ demonstrated ___ when…”
- “A theme that resonates with me is ___ because…”
- “This section of the text challenged my thinking by…”
- “I predict that ___ will ___ because…”
- “The author’s use of ___ symbolizes ___ because…”
5. Exit Tickets

At the end of a lesson, ask students to complete an exit ticket using a sentence stem that prompts them to summarize what they learned, pose questions they still have, or apply the day’s lesson to a new situation.
- “Today’s lesson on ___ taught me that…”
- “I’m still curious about ___ because…”
- “I can apply what I learned by…”
- “A question I have is ___ because…”
- “One thing I will remember is ___ because…”
6. Role-playing and Simulations

Incorporate sentence stems into role-playing activities or simulations, especially in social studies or language arts. Stems can help students articulate thoughts and dialogues from the perspectives of historical figures, characters, or various professions.
- “As [character/figure], I believe ___ because…”
- “Facing [situation], I would ___ because…”
- “The decision to ___ was influenced by ___ because…”
- “From [perspective], the issue of ___ appears as…”
- “If I were [character/figure], I would ___ to solve…”
7. Science Labs and Experiments

Use sentence stems to guide lab reports or reflections on experiments. Stems can help students articulate hypotheses, observations, and conclusions in a structured and scientific manner.
- “Our hypothesis was ___ because we thought…”
- “During the experiment, we observed ___ which suggests…”
- “The results indicate ___ because…”
- “If we were to repeat the experiment, I would change ___ because…”
- “Our conclusion is ___ based on ___.”
8. Math Problem-solving

Encourage students to use sentence stems to explain their problem-solving processes, the strategies they chose, and why. This can help demystify complex problems and foster a growth mindset toward tackling challenging questions.
- “I approached this problem by ___ because…”
- “A mistake I encountered was ___ and I fixed it by…”
- “To solve ___, I first ___ then ___ because…”
- “A pattern I noticed was ___ which helped me…”
- “The strategy of ___ was effective because…”
9. Art Critiques

In art classes, sentence stems can guide students in critiquing their own work or that of others, discussing elements of art, principles of design, and personal interpretations.
- “This piece evokes ___ because of the use of ___…”
- “The artist’s intention might have been ___ because…”
- “Comparing these two works, I prefer ___ because…”
- “The use of color/texture/space in this work ___ because…”
- “This artwork challenges ___ by…”
10. Group Projects and Collaborations

For group work, provide sentence stems that facilitate constructive discussions, decision-making processes, and reflections on group dynamics. This can help students communicate more effectively and reflect on their collaborative skills.
- “Our team decided to ___ because…”
- “A challenge we faced as a group was ___ and we overcame it by…”
- “Each member contributed by ___ which enhanced our project by…”
- “For better collaboration, we could have ___ because…”
- “The most successful aspect of our project was ___ because…”
11. Interactive Bulletin Boards

Create a bulletin board in the classroom where students can post responses to a question of the week using sentence stems. This can be a lively way to engage with current events, thematic studies, or class novels.
- “This week’s question made me think about ___ because…”
- “My opinion on ___ is ___ because…”
- “A related idea/question I have is ___ because…”
- “This topic is important because ___…”
- “After reading others’ responses, I think ___ because…”
12. Digital Storytelling

Incorporate sentence stems into digital storytelling projects, guiding students in structuring their narratives, descriptions, and reflections. This activity can merge creative expression with structured language practice.
- “The main message of my story is ___ because…”
- “In my narrative, the character faces ___ and overcomes it by…”
- “The setting of ___ plays a crucial role because…”
- “A turning point in my story is ___ because…”
- “I want the audience to feel ___ when they see/hear my story because…”
Related: Sentence Stems Vs Sentence Frames
Final thoughts
The activities outlined here, from reflective writing to digital storytelling, are just the tip of the iceberg when it comes to the versatility and power of sentence stems .I encourage my fellow educators to embrace sentence stems not just as a teaching strategy but as a philosophy of empowerment. By doing so, we’re not just teaching students to build sentences; we’re helping them build their voices, their confidence, and their capacity to contribute meaningfully to the conversations that shape our world.
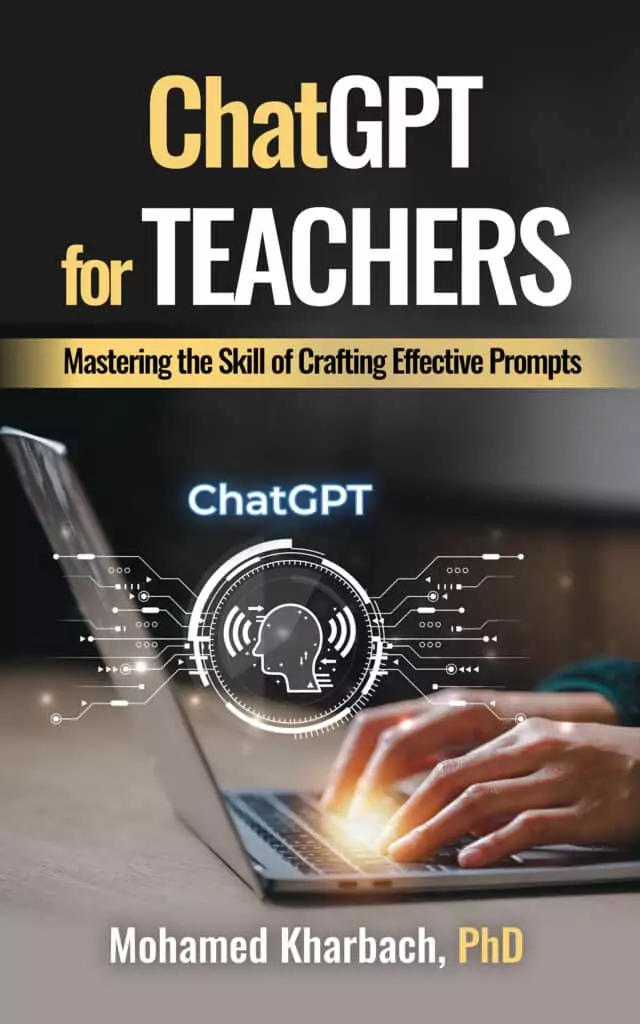
Join our mailing list
Never miss an EdTech beat! Subscribe now for exclusive insights and resources .
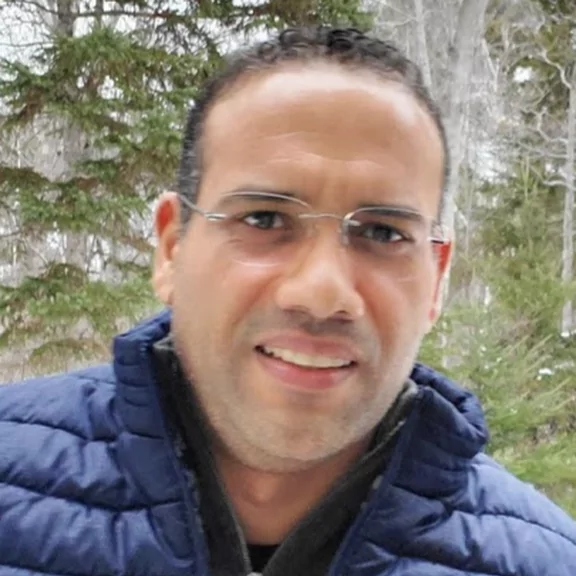
Meet Med Kharbach, PhD
Dr. Med Kharbach is an influential voice in the global educational technology landscape, with an extensive background in educational studies and a decade-long experience as a K-12 teacher. Holding a Ph.D. from Mount Saint Vincent University in Halifax, Canada, he brings a unique perspective to the educational world by integrating his profound academic knowledge with his hands-on teaching experience. Dr. Kharbach's academic pursuits encompass curriculum studies, discourse analysis, language learning/teaching, language and identity, emerging literacies, educational technology, and research methodologies. His work has been presented at numerous national and international conferences and published in various esteemed academic journals.
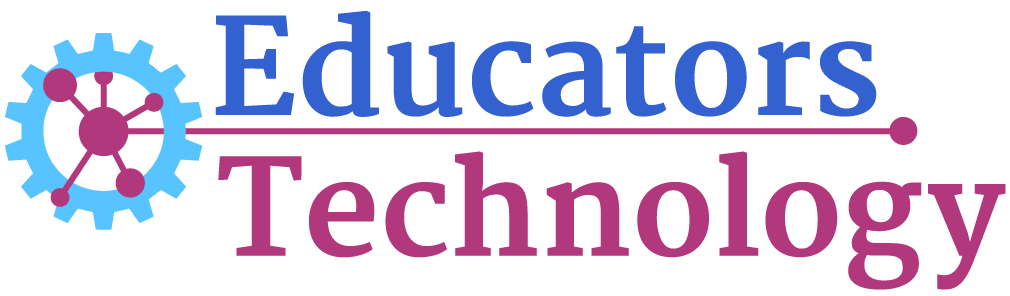
Join our email list for exclusive EdTech content.
Ways of thinking in STEM-based problem solving
- Original Paper
- Open access
- Published: 03 March 2023
- Volume 55 , pages 1219–1230, ( 2023 )
Cite this article
You have full access to this open access article
- Lyn D. English ORCID: orcid.org/0000-0001-9118-2812 1
This article proposes an interconnected framework, Ways of thinking in STEM-based Problem Solving , which addresses cognitive processes that facilitate learning, problem solving, and interdisciplinary concept development. The framework comprises critical thinking, incorporating critical mathematical modelling and philosophical inquiry, systems thinking, and design-based thinking, which collectively contribute to adaptive and innovative thinking. It is argued that the pinnacle of this framework is learning innovation, involving the generation of powerful disciplinary knowledge and thinking processes that can be applied to subsequent problem challenges. Consideration is first given to STEM-based problem solving with a focus on mathematics. Mathematical and STEM-based problems are viewed here as goal-directed, multifaceted experiences that (1) demand core, facilitative ways of thinking, (2) require the development of productive and adaptive ways to navigate complexity, (3) enable multiple approaches and practices, (4) recruit interdisciplinary solution processes, and (5) facilitate the growth of learning innovation. The nature, role, and contributions of each way of thinking in STEM-based problem solving and learning are then explored, with their interactions highlighted. Examples from classroom-based research are presented, together with teaching implications.
Similar content being viewed by others
Facilitating STEM Integration Through Design
Building a Competency-Based STEM Curriculum in Non-STEM Disciplines: A sySTEMic Approach
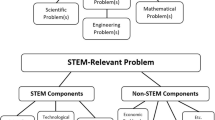
Inquiring into the Nature of STEM Problems
Avoid common mistakes on your manuscript.
1 Introduction
With the prevailing emphasis on integrated STEM education, the power of mathematical problem solving has been downplayed. Over two decades we have witnessed a decline in research on mathematical problem solving and thinking, with more questions than answers emerging (English & Gainsburg, 2016 ; Lester & Cai, 2016 ). This is of major concern, especially since work and non-work life increasingly call for resources beyond “textbook” problem solving (Chin et al., 2019 ; Krause et al., 2021 ). Such changing demands could not have been more starkly exposed than in the recent COVID 19 crisis, where mathematics played a crucial role in public and personal discourse, in describing and modelling current and potential scenarios, and in explaining and justifying societal regulations and restrictions. As Krause et al. ( 2021 ) highlighted, “No mathematical task we can create could be a richer application of mathematics than this real situation” (p. 88).
Modelling and statistical analyses that led the search for strategies to “flatten the curve” with minimal social or economic detriment were prominent in the media (Rhodes & Lancaster, 2020 ; Rhodes et al., 2020 ). As nations strive to rebuild their economies including grappling with crippling energy costs, advanced modelling again plays a key role (Aviso et al., 2022 ; Oxford Economics, 2022 ; Teng et al., 2022 ). Unfortunately, the gap between the mathematical modelling applied during the pandemic and how the public interpreted the models has been “palpable and evident” (Aguilar & Castaneda, 2021 ). Sadly, as Di Martino (Krause et al., 2021 ) pointed out regarding his nation:
People’s reactions in this pandemic underlined the spread of a widely negative attitude toward mathematics among the adult population in Italy. We have witnessed the proliferation of strange, unscientific, and dangerous theories but also the risk of refusing to approach facts that involve math and the resulting dependence to fully rely on others when mathematics is used to justify decisions. Also, on this occasion many people showed their own fear of math and their rejection of mathematical arguments as relevant factors in justification (p. 93).
This heightened visibility of mathematics in society coupled with a general lack of mathematical literacy sparked major questions about the repercussions for education and research (Bakker & Wagner, 2020 ; Kollosche & Meyerhöfer, 2021 ). One such repercussion is the need to reconsider perspectives on STEM-based problem solving and how different ways of thinking can enhance or hinder solutions. With reference to engineering education, Dalal et al. ( 2021 ) indicated how numerous calls for a focus on ways of thinking have largely been taken for granted or at least treated at a superficial level. As these authors pointed out, while perceived as a theoretical concept, ways of thinking “can and should be used in practice as a structure for solution-oriented outlooks and innovation” (p. 109).
Given the above points, I propose an interconnected framework, Ways of thinking in STEM-based Problem Solving (Fig. 1 ), which addresses cognitive processes that facilitate learning, problem solving, decision-making, and interdisciplinary concept development (cf. Slavit et al., 2021 ). The framework comprises critical thinking (including critical mathematical modelling and philosophical inquiry), systems thinking, and design-based thinking. Collectively, these thinking skills contribute to adaptive and innovative thinking (McKenna, 2014 ) and ultimately lead to the development of learning innovation (Sect. 3.4 ; English, 2018 ).

These thinking skills have been chosen because of their potential to enhance STEM-based problem solving and interdisciplinary concept development (English et al., 2020 ; Park et al., 2018 ; Slavit et al., 2021 ). Highlighting these ways of thinking, however, is not denying the importance of other thinking skills such as creativity, which is incorporated within the adaptive and innovative thinking component of the proposed framework (Fig. 1 ), and is considered multidimensional in nature (OECD, 2022 ). Other key skills such as communication and collaboration (Stehle & Peters-Burton, 2019 ) are acknowledged but not explored here.
In line with Dalal et al. ( 2021 ), I consider the proposed ways of thinking as providing an organisational structure both individually and interactively when enacted in practice—notwithstanding the contextual and instructional influences that can have an impact here (Slavit et al., 2022 ). Prior to exploring these ideas, I consider STEM-based problem solving with a focus on mathematics.
2 STEM-based problem solving: a focus on mathematics
Within our STEM-intensive society, we face significant challenges in promoting STEM education from the earliest grades while also maintaining the integrity of the individual disciplines (Tytler, 2016 ). With the increasing need for STEM skills across multiple workforce domains, contrasted with difficulties in STEM implementation in many schools (e.g., Dong et al., 2020 ), the urgency to advance STEM education has never been greater. With the massive disruption caused by COVID-19, coupled with problematic international relations, our school students’ futures have become even more uncertain—we cannot ignore the rapid changes that will continue to impact their lives. Unlike business and industry, where disruption creates a “force-to-innovate” approach (Crittenden, 2017 , p. 14), much of school education seems oblivious to preparing students for these disruptive forces or at least are restricted in doing so by set curricula.
Preparing our students for an increasingly uncertain and complex future requires rethinking the nature of their learning experiences, in particular, the need for more relevant and innovative problems that are challenging but manageable, and importantly, facilitate adaptive learning and problem solving (McKenna, 2014 ). A failure to provide such opportunities may have detrimental effects on young students’ learning and their future achievements (Engel et al., 2016 ). Despite mathematics being cited as the core of STEM education and foundational to the other disciplines (e.g., Larson, 2017 ; Roberts et al., 2022 ; Shaughnessy, 2013 ), it is frequently ignored in integrated STEM activities (English, 2016 ; Maass et al., 2019 ; Mayes, 2019 ; Shaughnessy, 2013 ). For example, quantitative reasoning, which is critical to integrated STEM problem solving, is frequently “misrepresented, underdeveloped, and ignored in STEM classrooms” (Mayes, 2019, p. 113). Likewise, Tytler ( 2016 ) warned that there needs to be an explicit focus on the mathematical concepts and thinking processes that arise in STEM activities. Without this focus, STEM programs run the risk of reducing the valuable contributions of mathematical thinking. If children fail to see meaningful links between their learning in mathematics and the other STEM domains, they can lose interest not only in mathematics but also in the other disciplines (Kelley & Knowles, 2016 ).
Traditional notions of mathematical problem solving (e.g., Charles, 1985 ) are now quite inadequate when applied to our current world. At a time of increasing creative disruption, it is essential for mathematical problem solvers to be adaptive in dealing with unforeseen local, national, and international problems. Increasingly, STEM-based problems in the real world encompass more than just disciplinary content and practices. While not denying the essential nature of these components, issues pertaining to cultural, social, political, and ethical dimensions (Kollosche & Meyerhöfer, 2021 ; Pheasants, 2020) can also impact the solution process, necessitating the application of appropriate thinking skills. As Pheasants stressed, “If STEM education is to prepare students to grapple with complex problems in the real world, then more attention ought to be given to approaches that are inclusive of the non-STEM dimensions that exist in those problems.”
In light of the above arguments, I view mathematical and STEM-based problems as goal-directed experiences that (1) demand STEM-relevant ways of thinking, (2) require the development of productive and adaptive ways to navigate complexity, (3) enable multiple approaches and practices (Roberts et al., 2022 ), (4) recruit interdisciplinary solution processes, and (5) facilitate growth of learning innovation for all students regardless of their background (English, 2018 ). In contrast to traditional expectations, such problems need to embody affordances that facilitate learning innovation, where all students can move beyond their existing competence in standard problem solving and be challenged to generate new knowledge in solving unanticipated problems. Even students who achieve average results on standardised tests display conceptual understanding and advanced mathematical thinking not normally seen in the classroom—especially when current common practices emphasise number skills at the expense of problem solving and reasoning with numbers (Kazemi, 2020 ).
Limited attention, however, has been paid to how problem experiences can be developed that press beyond basic content knowledge (Anderson, 2014; Li, Schoenfeld et al., 2019), encompass the STEM disciplines, and develop important ways of thinking. In their recent article, Slavit et al. ( 2021 ) argued that STEM education should be “grounded in our knowledge of how students think in STEM-focused learning environments” (p. 1), and that fostering twenty-first-century skills is essential. Yet, as these authors highlighted, there is not much research on STEM ways of thinking, with even fewer theoretical perspectives and frameworks on which to draw. In the next section, I consider these ways of thinking, defined in Table 1 , and provide examples of their applications to STEM-based problem solving.
3 Ways of thinking in STEM-based problem solving
3.1 critical thinking.
Although long recognised as a significant process in a range of fields, research on critical thinking in education, especially in primary education, has been limited (Aktoprak & Hursen, 2022 ). Critical thinking has long been associated with mathematical reasoning and problem solving, but their association remains under-theorized (Jablonka, 2020 ). Likewise, connections between critical thinking and design thinking have had limited attention largely due to their shared conceptual structures not being articulated (Ericson, 2022 ). As a twenty-first century skill, critical thinking is increasingly recognised as essential in STEM and mathematics education (Kollosche & Meyerhöfer, 2021 ) but is sadly lacking in many school curricula (Braund, 2021 ). As applied to STEM-based problem solving, critical thinking builds on inquiry skills (Nichols et al., 2019 ) and entails evaluating and judging problem situations including statements, claims, and propositions made, analysing arguments, inferring, and reflecting on solution approaches and conclusions drawn. Although critical thinking can contribute significantly to each of the other ways of thinking, its application is often neglected. For example, critical thinking is increasingly needed in design and design thinking, which play a key role in product development, environmental projects, and even in forms of social interaction (as discussed in Sect. 3.3 ; Ericson, 2022 ).
3.1.1 Critical mathematical modelling
One rich source of problem experiences that foster critical thinking is that of modelling. The diverse field of mathematical modelling has long been prominent in the secondary years (e.g., Ärlebäck & Doerr, 2018 ) but remains under-researched in the primary years, especially in relation to its everyday applications. Effective engagement with social, political, and environmental issues through modelling and statistics demands critical thinking, yet such aspects are not often considered in school curricula (Jablonka, 2020 ). This is of particular concern, given the pressing need to tackle such issues in today’s world.
As noted in several publications, the interdisciplinary nature of mathematical modelling makes it ideal for STEM-based problem solving (English, 2016 ; Maass et al., 2019 ; Zawojewski et al., 2008 ). Numerous definitions of models and modelling exist in the literature (e.g., Blum & Leiss, 2007 ; Brady et al., 2015 ). For this article, modelling involves developing conceptual innovations in response to real-world needs; effective modelling requires moving beyond the conventional ways of thinking applied in typical school problems (Lesh et al., 2013 ) to include contextual and critical analysis.
Much has been written about the role of modelling during COVID-19. Mathematical models played a major role in grappling with COVID 19, but their projections were a source of controversy (Rhodes & Lancaster, 2020 ). There seems little appreciation of the critical nature of mathematical models in society (Barbosa, 2006 ) and how assumptions in the modelling process can sway decisions. STEM-based problem solving needs to incorporate not just modelling itself, but also critical mathematical modelling. Critiquing what a model yields, and what is learned, is of increasing social importance (Aguilar & Castaneda, 2021 ; Barbosa, 2006 ). Indeed, as Braund ( 2021 ) illustrated, the Covid-19 pandemic has revealed the urgent need for “critical STEM literacy” (p. 339)—an awareness of the complex and problematic interactions of STEM and politics, and a knowledge and understanding of the underlying STEM concepts and representations is essential:
There are two imperatives that emerge: first, that there is sufficient STEM literacy to negotiate the complex COVID-19 information landscape to enable personal decision taking and second, that this is accompanied by a degree of criticality so that politicians and experts are called to account (Braund, 2021 , p. 339).
Kollosche ( 2021 ) shed further light on the lack of critical thinking in the media’s reporting on COVID, with his argument that “most newspaper reports were effective in creating the problems” because of their focus on ideal forms of mathematical concepts and modelling, without discussing the assumptions and methods behind the reported data. Of concern is that “mass media still fail to present scientific models and results in a way that allows for mathematical reflection and a critical evaluation of such information by citizens.”
Modelling experiences that draw on students’ cultural and community contexts (Anhalt et al., 2018 ; English, 2021a , 2021b ; Turner et al., 2009 ) provide rich opportunities for critical thinking from a citizen’s perspective. Such opportunities can also assist students in appreciating that mathematics is not merely a means of calculating answers but is also a vehicle for social justice, where critical thinking plays a key role (Cirillo et al., 2016 ; Greer et al., 2007 ). In their studies of critical thinking in cultural and community contexts, Turner and her colleagues (Turner et al., 2009 , 2021 , 2022 ) explored culturally responsive, community-based approaches to mathematical modelling with elementary teachers and students. Using a range of authentic modelling contexts, Turner and her colleagues illustrated how students’ modelling processes generated a number of issues that required them to think critically about their lives and their lives within their community. In one activity, students were applying design thinking and processes as they redesigned their local park. They generated, for example, mathematical models to estimate how long children would have to wait to use the swings—this informed their decision that the park did not meet the needs of the community. This community-based modelling highlighted “ongoing negotiation between students’ experiences and intentions related to the community park, the constraints of the actual context, and the mathematical issues that arose” (Turner et al., 2009 , p. 148).
Another example of modelling involving critical thinking in cultural and community contexts was implemented in a sixth-grade Cyprus classroom. Students were required to develop a model for their country to purchase water supplies from a choice of nearby nations (English & Mousoulides, 2011 ). Students were to consider travel distances, water price, available supply per week, oil tanker capacity, costs of water and oil, and quality of the port facilities in the neighbouring countries. The targeted model had to select the best option not only for the present but also for the future. Students’ models ranged from a basic form, where port facilities and water supply were ignored, to more sophisticated models, where all factors were integrated, with carbon emissions also considered. One of the student groups who took into account environmental factors commented, “It would be better for the country to spend a little more money and reduce oil consumption. And there are other environmental issues, like pollution of the Mediterranean Sea.” The more sophisticated models reflected systems thinking (Sect. 3.2 ), where the impact of partial factors such as oil consumption on the whole domain (ocean ecosystems and community) was also considered.
3.1.2 Philosophical Inquiry
One underrepresented means of fostering critical thinking in mathematical and STEM-based problem solving is through philosophical inquiry (Calvert et al., 2017 ; English, 2013 , 2022 ; Kennedy, 2012 ; Mukhopadhyay & Greer, 2007 ). Such inquiry encourages a range of thinking skills in identifying hidden assumptions, determining alternative courses of action, and reflecting on conclusions drawn and claims made. Several studies have shown how engaging children in communities of philosophical inquiry nurtures critical thinking dispositions, which become both a goal and a method (Bezençon, 2020 ; Daniel et al., 2017 ; Lipman, 2003 , 2008 ). At the same time, philosophical inquiry can lead to “conceptual deepening” (Bezençon, 2017), where analysis of mathematical and related STEM concepts as they apply beyond the classroom can be fostered. Given the increased societal awareness of mathematics and STEM in recent years, philosophical inquiry can be a powerful tool in enhancing students’ understanding and appreciation of how these disciplines shape societies. At the same time, philosophical inquiry can stimulate consideration of ethical issues in the applications of these disciplines (Bezençon, 2020 ). For example, Mukhopadhyay and Greer ( 2007 ) indicated how mathematics education should “convey the complexity of mathematical modeling social phenomena and a sense of what demarcates questions that can be answered by empirical evidence from those that depend on value systems and world-views” (p. 186).
A comprehensive review by O’Reilly et al. ( 2022 ) identified pedagogical approaches to scaffolding early critical thinking skills including inquiry-based teaching using classroom dialogue or questioning techniques. Such techniques include philosophical inquiry and encouraging children to construct, share, and justify their ideas regarding a task or investigation. Other opportunities for philosophical inquiry include group problem solving, peer sharing of created models, and facilitating critical and constructive peer feedback. For example, Gallagher and Jones ( 2021 ) reported on integrating mathematical modelling and economics, where beginning teachers were presented with a task involving a problematic community issue following a school shooting. In such cases, numerous courses of action are typically proposed for addressing the problem. Not surprisingly, various community opinions exist on such proposals, giving rise to valuable contexts for philosophical inquiry and critical modelling, where data and their sources are carefully analysed. With the escalation of statistical data from the mass media, it is imperative to commence the foundations of critical and philosophical thinking early. Students’ skills in asking critical questions as they work with data in constructing and improving a model, reflect on what their models convey, consider consequences of their models, and justify and communicate their conclusions require nurturing throughout school (Gibbs & Young Park, 2022 ).
3.2 Systems thinking
Systems thinking cuts across the STEM disciplines as well as many other fields outside education. It is considered a key component of “critical thinking and problem solving” in 21st Century Learning (P21, 2015 ) and is often cited as a “habit of mind” in engineering education (e.g., Lippard et al., 2018 ; Lucas et al., 2014 ). Numerous definitions exist for systems thinking (e.g., Bielik et al., 2022 ; Damelin et al., 2017 ; Jacobson & Wilenski, 2022 ), with Bielik et al. ( 2022 ) identifying such thinking as the ability to “consider the system boundaries, the components of the system, the interactions between system components and between different subsystems, and emergent properties and behaviour of the system” (p. 219). In more basic terms, Shin et al. ( 2022 ) refer to systems thinking as “the ability to understand a problem or phenomenon as a system of interacting elements that produces emergent behavior” (p. 936).
Systems thinking interacts with the other thinking forms including those displayed in Fig. 1 , as well as computational thinking (Shin et al., 2022), critical thinking (Curwin et al., 2018 ) and mathematical thinking more broadly (Baioa & Carreira, 2022 ). Systems thinking is considered especially important in conceptualizing a problematic situation within a larger context and in perceiving problems in new and different ways (Stroh, 2018 ). Of special relevance to today’s world is the realisation that perfect solutions do not exist and the choices one makes in applying systems thinking will impact on other parts of the system (Meadows, 2008 ). What is often not considered in today’s complex societies—at least not to the extent required—is that we live in a world of intrinsically linked systems, where disruption in one part will reverberate in others. We see so many instances where particular courses of action are advocated or mandated in societal systems, while the impact on sub-components is perilously ignored. Examples are evident in many nations’ responses to COVID-19, where escalating lockdowns impacted economies and communities, whose demands had to be balanced against purely epidemiological factors. The reverberations of such actions stretch far and wide over long periods. Likewise, the various impacts of current climate actions are frequently ignored, such as how the construction of vast areas of renewable resources (e.g., wind turbines) can have deleterious effects on the surrounding environments including wildlife.
Despite its centrality across the STEM domains, systems thinking is almost absent from mathematics education (Curwin et al., 2018 ). This is despite claims by many researchers that modelling, systems thinking, and associated thinking processes should be significant components of students’ education (Bielik et al., 2022 ; Jacobson & Wilenski, 2022 ). Indeed, systems thinking is featured prominently in the US A Framework for K-12 Science Education (NRC, 2012 ) and the Next Generation Science Standards (NGSS Lead States, 2013 ), and has received considerable attention in science education (e.g., Borge, 2016 ; Hmelo-Silver et al., 2017 ; York et al., 2019 ) and engineering education (e.g., Lippard & Riley, 2018 ; Litzinger, 2016 ).
Given the complexity of systems thinking in today’s world, and the diverse ways in which it is applied, students require opportunities to experience how systems thinking can interact with other forms such as design thinking and critical thinking (Curwin et al., 2018 ; Shin et al., 2022). Such interactions occur in many popular STEM investigations including one in which fifth-grade students designed, constructed, and experimented with a loaded paper plane (paper clips added) in determining how load impacts on the distance travelled (English, 2021b ). Students observed that changing one design feature (e.g., wingspan or load position) unsettles some other features (e.g., fuselage depth decreases wingspan). The investigation yielded an appreciation of the intricacy of interactions among a system’s components, and their scarcely predictable mutual effects.
Other studies have revealed how very young children can engage in basic systems thinking within STEM contexts. For example, Feriver et al. ( 2019 ) administered a story reading session to individual 4- to 6-year-old children in preschools in Turkey and Germany, and then followed this with individual semi-structured interviews about the story. The reading session was based on the story book, “The Water Hole” (Base, 2001 ), which draws on basic concepts of systems within an ecosystem context. Their study found the young children to have some complex understanding of systems thinking in terms of detecting obvious gradual changes and two-step domino and/or multiple one-way causalities, as well as describing the behaviour of a balancing loop (corrective actions that try to reduce the gap between a desired level or goal and the actual lever, such as temperature and plant growth). However, the children understandably experienced difficulties in several areas, in which even adults have problems. For example, detecting a reinforcing loop, identifying unintended consequences, detecting hidden components and processes, adopting a multi-dimensional perspective, and predicting how a system would behave in the future were problematic for them. In another study, Gillmeister ( 2017 ) showed how preschool children have a more complex understanding of systems thinking than previously claimed. Their ability to utilize simple systems thinking tools, such as stock-flow maps, feedback loops and behaviour over time graphs, was evident.
Although the research has not been extensive, current findings indicate how we might capitalise on the seeds of early systems thinking across the STEM fields. With the pervasive nature of systems thinking, it is argued that its connections to other forms of thinking across STEM should be nurtured (cf., Svensson, 2022 ). This includes links to design-based thinking where designed products, for example, operate “within broader systems and systems of systems” (Buchanan, 2019 ).
3.3 Design-based thinking and STEM-based problem solving
Design-based thinking plays a major role in complex problem solving, yet its contribution to mathematics learning has been largely ignored, especially at the primary levels. Design is central in technology and engineering practice (English et al., 2020 ; Guzey et al., 2019 ), from bridge construction through to the development of medical tools. Design contributes to all phases of problem solving and drives students toward innovative rather than predetermined outcomes (Goldman & Zielezinski, 2016 ). As applied to STEM education, design thinking is generally viewed as a set of iterative processes of understanding a problem and developing an appropriate solution. It includes problem scoping, idea generation, systems thinking, designing and creating, testing and reflecting, redesigning and recreating, and communicating. Both learning about design and learning through design can help students develop more informed analytical approaches to mathematical and STEM-based problem solving (English et al., 2020 ; Strimel et al., 2018 ).
Learning about design in solving complex problems emphasises design thinking processes per se, that is, students learn about design itself. Design thinking as a means for learning about design is not as prevalent in integrated STEM education. Although learning through and about design should not be divorced during problem solving (van Breukelen et al., 2017 ), one learning goal can take precedence over the other. Both require greater attention, especially in today’s design-focused world (Tornroth & Wikberg Nilsson, 2022 ).
A plurality of solutions of varying levels of sophistication is possible in applying design thinking processes, so students from different achievement levels can devise solutions (Goldman & Kabayadondo, 2017 )—and research suggests that lower-achieving students benefit most (Chin et al., 2019 ). The question of how best to develop design learning across grades K-12, however, has not received adequate attention. This is perhaps not surprising given that comparatively few studies have investigated the design thinking of grades 6–12 and even fewer across the elementary years (Kelley & Sung, 2017 ; Strimel et al., 2018 ).
Also overlooked is how design-based thinking can foster concept development, that is, how it can foster “learning while designing”, or generative learning (English et al., 2020 ). Design-based thinking provides natural opportunities to develop understanding of the required STEM concepts (Hjalmarson et al., 2020 ). As these authors pointed out, when students create designs, they represent models of their thinking. When students have to express their conceptual understanding in the form of design, such as an engineering designed product, their knowledge (e.g., of different material properties) is tested. Studies have revealed such learning occurs when elementary and middle school students design and construct various physical artifacts. For example, in a fifth-grade study involving designing and building an optical instrument that enabled one to see around corners, King and English ( 2017 ) observed how students applied and enriched their understanding of scientific concepts of light and how it travels through a system, together with their mathematical knowledge of geometric properties, angles and measurement.
In a study in the secondary grades (Langman et al. ( 2019 ), student groups completed modules that included a model-eliciting activity (Lesh & Zawojewski, 2007 ) involving iterative design thinking processes. Students were to interpret, assess, and compare images of blood vessel networks grown in scaffolds, develop a procedure or tool for measuring (or scoring) this vessel growth, and demonstrate how to apply their procedure to the images, as well as to any image of a blood vessel network. The results showed how students designed a range of mathematical models of varying levels of sophistication to evaluate the quality of blood vessel networks and developed a knowledge of angiogenesis in doing so.
In another study implemented in a 6th-grade classroom, students were involved in both learning about and learning through design in creating a new sustainable town with the goal of at least 50% renewable energy sources. The students were also engaged in systems thinking, as well as critical thinking, as they planned, developed, and critically analysed the layout and interactions of different components of their town system (e.g., where to place renewable energy sources to minimise impact on the residences and recreational areas; where to situate essential services to reach different town components). Students also had to consider the budgetary constraints in their town designs. Their learning about design was apparent in their iterative processes of problem scoping, idea generation and modification, balancing of benefits and trade-offs (dealing with system subcomponents), critical reflections on their designs, and improvements of the overall town design. Learning though design was evident as students displayed content knowledge pertaining to renewable energy and community needs, applied spatial reasoning in positioning town features, and considered budgetary constraints in reaching their “best” design.
Engineering design processes form a significant component of design thinking and learning, with foundational links across the STEM disciplines including mathematical modelling. Engineering design-based problems help students appreciate how they can apply different ideas and approaches from the STEM disciplines, with more than one outcome possible (Guzey et al., 2019 ). Such design thinking engages students in interpreting problems, developing initial design ideas, selecting and testing a promising design, analysing results from their prototype solution, and revising to improve outcomes.
It is of concern that limited attention has been devoted to engineering design-based thinking in mathematics and the other STEM disciplines in the primary grades. This is despite research indicating how very young children can engage in design-based thinking when provided with appropriate opportunities (e.g., Cunningham, 2018 ; Elkin et al., 2018 ). Elkin et al., for example, used robotics in early childhood classrooms to introduce foundational engineering design thinking processes. Their study illustrated how these young learners used engineering design journals and engaged in design thinking as they engineered creative solutions to challenging problems presented to them. It seems somewhat paradoxical that foundational engineering design thinking is a natural part of young children’s inquiry about their world, yet this important component has been largely ignored in these informative years.
In sum, design-based thinking is a powerful and integrative tool for mathematical and STEM-based problem solving and requires greater focus in school curricula. Both learning about and through design have the potential to improve learning and problem solving well beyond the school years (Chin et al., 2019 ). At the same time, design-based thinking, together with the other ways of thinking, can contribute significantly to learning innovation (English, 2018 ).
3.4 Adaptive and innovative thinking for learning innovation
Disruption is rapidly becoming the norm in almost all spheres of life. The recent national and international upheavals have further stimulated disruptive thinking (or disruptive innovation), where perspectives on commonly accepted (and often inefficient) solutions to problems are rejected for more innovative approaches and products. Given the pressing need for problem solvers capable of developing new and adaptable knowledge, rather than applying limited simplistic procedures or strategies, we need to foster what I term, learning innovation (English, 2018 ; Fig. 2 ). Such learning builds on core content to generate more powerful disciplinary knowledge and thinking processes that can, in turn, be adapted and applied to solving subsequent challenging problems.

Learning innovation
Figure 2 (adapted from McKenna, 2014 ) illustrates how learning innovation can be fostered through the growth of mathematical and STEM knowledge, together with STEM ways of thinking. The optimal adaptability corridor represents the growth of mathematical and STEM-based problem solving from novice to adaptable solver . Adaptable problem solvers (Hatano & Oura, 2003 ) have developed the flexibility, curiosity, and creativity to tackle novel problems—skills that are needed in jobs of the future (Denning & Noray, 2020 ; OECD, 2019 ). Without the disciplinary knowledge, ways of thinking, and engagement in challenging but approachable problems, a student risks remaining merely a routine problem solver—one who is skilled solely in applying previously taught procedures to solve sets of familiar well-worn problems, as typically encountered at school. Learning innovation remains a challenging and unresolved issue across curriculum domains and is proposed as central to dealing effectively with disruption.
Particularly rich experiences for developing learning innovation involve interdisciplinary modelling, incorporating critical mathematical modelling (e.g., Hallström and Schönborn, 2019 ; Lesh et al., 2013 ). As noted, a key feature of model-eliciting experiences is the affordances they provide all students to exhibit “extraordinary abilities to remember and transfer their tools to new situations” (Lesh et al., 2013 , p. 54). Modelling enables students to apply more sophisticated STEM concepts and generate solutions that extend beyond their usual classroom problems. Such experiences require different ways of thinking in problem solving as they deal with, for example, conflicting constraints and trade-offs, alternative paths to follow, and various tools and representations to utilise. In essence, interdisciplinary modelling may be regarded as a way of creating STEM concepts, with modeling and concept development being highly interdependent and mutually supportive (cf., Lesh & Caylor, 2007 ).
4 Concluding points
The ill-defined problems of today, coupled with unexpected disruptions across all walks of life, demand advanced problem-solving by all citizens. The need to update outmoded forms of problem solving, which fail to take into account increasing global challenges, has never been greater (Cowin, 2021 ). The ways-of-thinking framework has been proposed as a powerful means of enhancing problem-solving skills for dealing with today’s unprecedented game-changers. Specifically, critical thinking (including critical mathematical modelling and philosophical inquiry), systems thinking, and design-based thinking are advanced as collectively contributing to the adaptive and innovative skills required for problem success. It is argued that the pinnacle of this framework is learning innovation, which can be within reach of all students. Fostering students’ agency for developing learning innovation is paramount if they are to take some control of their own problem solving and learning (English, 2018 ; Gadanidis et al., 2016 ; Roberts et al., 2022 ).
Establishing a culture of empowerment and equity, with an asset-based approach, where the strengths of all students are recognised, can empower students as learners and achievers in an increasingly uncertain world (Celedón-Pattichis et al., 2018 ). Teacher actions that encourage students to express their ideas, together with a program of future-oriented mathematical and STEM-based problems, can nurture students’ problem-solving confidence and dispositions (Goldman & Zielezinski, 2016 ; Roberts et al., 2022 ). In particular, mathematical and STEM-based modelling has been advocated as a rich means of developing multiple ways of thinking that foster adaptive and innovative learners—learners with a propensity for developing new knowledge and skills, together with a willingness to tackle ill-defined problems of today and the future. Such propensity for dealing effectively with STEM-based problem solving is imperative, beginning in the earliest grades. The skills gained can thus be readily transferred across disciplines, and subsequently across career opportunities.
Data availability
The data sets generated during and/or analysed during the current study are available from the corresponding author on reasonable request.
Aguilar, M. S., & Castaneda, A. (2021). What mathematical competencies does a citizen need to interpret Mexico’s official information about the COVID-19 pandemic? Educational Studies in Mathematics . https://doi.org/10.1007/s10649-021-10082-9
Article Google Scholar
Aktoprak, A., & Hursen, C. (2022). A bibliometric and content analysis of critical thinking in primary education. Thinking Skills and Creativity, 44 , 101029.
Anhalt, C. O., Staats, S., Cortez, R., & Civil, M. (2018). Mathematical modeling and culturally relevant pedagogy. In Y. J. Dori, Z. R. Mevarech, & D. R. Baker (Eds.), Cognition, metacognition, and culture in STEM education (pp. 307–330). Springer. https://doi.org/10.1007/978-3-319-66659-4_14
Chapter Google Scholar
Ärlebäck, J. B., & Doerr, H. M. (2018). Students’ interpretations and reasoning about phenomena with negative rates of change throughout a model development sequence. ZDM Mathematics Education, 50 (1–2), 187–200.
Aviso, K. B., Yu, C. D., Lee, J.-Y., & Tan, R. R. (2022). P-graph optimization of energy crisis response in Leontief systems with partial substitution. Cleaner Energy and Technology, 9 , 100510. https://doi.org/10.1016/j.clet.2022.100510
Baioa, A. M., & Carreira, S. (2022). Mathematical thinking about systems—Students modeling a biometrics identity verification system. Mathematical Thinking and Learning.
Bakker, A., & Wagner, D. (2020). Pandemic: Lessons for today and tomorrow? Educational Studies in Mathematics, 104 , 1–4. https://doi.org/10.1007/s10649-020-09946-3
Barbosa, J. C. (2006). Mathematical modelling in classroom: A socio-critical and discursive perspective. ZDM Mathematics Education, 38 , 293–301. https://doi.org/10.1007/BF02652812
Base, G. (2001). In N. Harry (Ed.), The Water Hole. Abrs Inc.
Google Scholar
Benessiaa, A., & De Marchi, B. (2017). When the earth shakes … and science with it. The management and communication of uncertainty in the L’Aquila earthquake. Futures, 91 , 35–45.
Bezençon, E. (2020). Practising philosophy of mathematics with children: what, why and how? Philosophy of Mathematics Education Journal, 36 .
Bielik, T., Stephens, L., McIntyre, C., Damelin, D., & Krajcik, J. S. (2022). Supporting student system modelling practice through curriculum and technology design. Journal of Science Education and Technology, 31 , 217–231. https://doi.org/10.1007/s10956-021-09943-y
Blum, W., & Leiss, D. (2007). How do students and teachers deal with mathematical modelling problems? In C. Haines, P. L. Galbraith, W. Blum, & S. Khan (Eds.), Mathematical modelling (ICTMA12)—Education, engineering and economics. Horwood.
Borge, M. (2016). Systems thinking as a design problem. In R. A. Duschl & A. S. Bismack (Eds.), Reconceptualizing STEM education (pp. 68–80). Routledge.
Brady, C., Lesh, R., & Sevis, S., et al. (2015). Extending the reach of the models and modelling perspective: A course-sized research site. In G. A. Stillman (Ed.), Mathematical modelling in education research and practice (pp. 55–66). Springer.
Braund, M. (2021). Critical STEM Literacy and the COVID-19 Pandemic. Canadian Journal of Science, Mathematics, and Technology Education, 21 , 39–356. https://doi.org/10.1007/s42330-021-00150-w
Buchanan, R. (2019). Systems thinking and design thinking: the search for principles in the world we are making. She Ji the Journal of Design, Economics, and Innovation, 5 (2), 85–104.
Calvert, K., Forster, M., Hausberg, A., et al. (2017). Philosophizing with children in science and mathematics classes. In M. Rollins Gregory, J. Haynes, & K. Murris (Eds.), The Routledge international handbook of philosophy for children (pp. 191–199). Routledge.
Celedón-Pattichis, S., Lunney Borden, L., Pape, S. J., et al. (2018). Asset-based approaches to equitable mathematics education research and practice. Journal for Research in Mathematics Education, 49 (4), 373–389.
Charles, R. (1985). The role of problem solving. Arithmetic Teacher, 32 , 48–50.
Chin, D. B., Blair, K. P., Wolf, R. C., Conlin, L. D., Cutumisu, M., Pfaffman, J., & Schwartz, D. L. (2019). Educating and measuring choice: A test of the transfer of design thinking in problem solving and learning. Journal of the Learning Sciences, 28 (3), 337–380. https://doi.org/10.1080/10508406.2019.1570933
Cirillo, M., Bartell, T. G., & Wager, A. A. (2016). Teaching mathematics for social justice through mathematical modeling. In C. R. Hirsch & A. R. RothMcDuffie (Eds.), Mathematical modeling and modeling mathematics (pp. 87–96). National Council of Teachers of Mathematics.
Cowin, J. (2021). The fourth industrial revolution: Technology and education. Journal of Systemics, Cybernetics and Informatics, 19 (8), 53–63.
Crittenden, AB, Crittenden VI, Crittenden, WF (2017) Industry transformation via channel disruption. Journal of Marketing Channels , 24 (1–2):13–26.
Cunningham, C. M. (2018). Engineering in elementary STEM education: Curriculum design, instruction, learning, and assessment . Teachers College Press.
Curwin, M. S., Ardell, A., MacGillivray, L., & Lambert, R. (2018). Systems thinking in a second grade curriculum: Students engaged to address a statewide drought. Frontiers in Education . https://doi.org/10.3389/feduc.2018.00090
Dalal, M., Carberry, A., Archambault, L., et al. (2021). Developing a ways of thinking framework for engineering education research. Studies in Engineering Education, 1 (2), 108–125.
Damelin, D., Krajcik, J., Mcintyre, C., & Bielik, T. (2017). Students making systems models: An accessible approach. Science Scope, 40 (5), 78–82.
Daniel, M., Gagnon, M., & Auriac-Slusarczyk, E. (2017). Dialogical critical thinking in kindergarten and elementary school: Studies on the impact of philosophical praxis in pupils. In M. R. Gregory, J. Haynes, & K. Murris (Eds.), The Routledge international handbook of philosophy for children (pp. 236–244). Routledge.
Denning, D. J., & Noray, K. (2020). Earnings dynamics, changing job skills, and STEM careers. The Quarterly Journal of Economics, 135 (4), 1965–2005. https://doi.org/10.1093/qje/qjaa021
Dong, Y., Wang, J., Yang, Y., & Karup, P. M. (2020). Understanding intrinsic challenges to STEM instructional practices for Chinese teachers based on their beliefs and knowledge base. International Journal of STEM Education, 7 , 47. https://doi.org/10.1186/s40594-020-00245-0
Eleyyan, S. (2021). The future of education according to the fourth industrial revolution. Journal of Educational Technology and Online Learning, 4 (1), 23–30.
Elkin, M., Sullivan, A., & Bers, M. U. (2018). Books, butterflies, and ’bots: Integrating engineering and robotics into early childhood curricula. In L. D. English & T. Moore (Eds.), Early engineering learning (pp. 225–248). Springer.
Engel, M., Claessens, A., Watts, T., & Farkas, G. (2016). Mathematics content coverage andstudent learning in kindergarten. Educational Researcher , 45 (5), 293–300. https://doi.org/10.3102/0013189X16656841
English, L. D. (2013). Modeling as a vehicle for philosophical inquiry in the mathematics classroom. The Journal of Analytic Teaching and Philosophical Praxis, 34 (1), 46–57.
English, L. D. (2016). STEM education K-12: Perspectives on integration. International Journal of STEM Education, 3 (1), 1–12. https://doi.org/10.1186/s40594-016-0036-1
English, L. D. (2018). Disruption and learning innovation across STEM. Plenary presented at the 5th International Conference of STEM in Education , Brisbane. https://stem-in-ed2018.com.au/proceedings-2/
English, L. D. (2021a). Mathematical and interdisciplinary modeling in optimizing young children’s learning. In J. Suh, M. Wickstrom, & L. D. English (Eds.), Exploring mathematical modeling with young learners (pp. 3–24). Springer.
English, L. D. (2021b). Facilitating STEM integration through design. In J. Anderson & Y. Li (Eds.), Integrated approaches to STEM education: An international perspective (pp. 45–66). Springer.
English, L. D. (2022). Mathematical modeling and philosophical inquiry in the elementary school. In N. Kennedy (Ed.), Dialogical inquiry in mathematics teaching and learning: A philosophical approach. Lit Verlag.
English, L. D., Adams, R., & King, D. (2020). Learning by design across STEM education. In C. J. Johnson, M. M. Schroeder, T. Moore, & L. D. English (Eds.), Handbook of research on STEM education (pp. 76–96). Routledge.
English, L. D., & Gainsburg, J. (2016). Problem solving in a 21st-century mathematics curriculum. In L. D. English & D. Kirshner (Eds.), Handbook of international research in mathematics education (3rd ed., pp. 313–335). Taylor & Francis.
English, L. D., & Mousoulides, N. (2011). Engineering-based modelling experiences in the elementary classroom. In M. S. Khine & I. M. Saleh (Eds.), Models and modeling: Cognitive tools for scientific enquiry (pp. 173–194). Springer.
Ericson, J. D. (2022). Mapping the relationship between critical thinking and design thinking. Journal of the Knowledge Economy , 13 , 406–429.
Feriver, S., Olgan, R., Teksöz, G., & Barth, M. (2019). Systems thinking skills of preschool children in early childhood education contexts from Turkey and Germany. MDPI: Sustainability, 11 , 1478.
Gadanidis, G., Hughes, J. M., Minniti, L., & White, B. J. (2016). Computational thinking, Grade 1 students and the binomial theorem. Digital Experiences in Mathematics Education . https://doi.org/10.1007/s40751-016-0019-3
Gallagher, M. A., & Jones, J. P. (2021). Supporting students’ critical literacy: Mathematical modeling and economic decisions. In J. Suh, M. Wickstrom, & L. D. English (Eds.), Exploring mathematical modeling with young learners (pp. 373–388). Springer.
Gibbs, A. M., & Young Park, J. (2022). Unboxing mathematics: Creating a culture of modeling as critic. Educational Studies in Mathematics, 110 , 167–192. https://doi.org/10.1007/s10649-021-10119-z
Gillmeister, K. M. (2017). Development of early conceptions in systems thinking in an environmental context: An exploratory study of preschool students’ understanding of stocks and flows . State University of New York.
Goldman, S., & Kabayadondo, Z. (Eds.). (2017). Taking Design Thinking to School: How the technology of design can transform teachers, learners, and classrooms (pp. 3–19). Routledge.
Goldman, S., & Zielezinski, M. B. (2016). Teaching with design thinking: developing newvision and approaches to twenty-first century learning. In L. Annetta & J. Minogue (Eds.), Connecting science and engineering education practices in meaningful ways. Contemporary trends and issues in science education (p. 44). Springer. https://doi.org/10.1007/978-3-319-16399-4_10 .
Greer, B. (2009). Estimating Iraqi deaths: A case study with implications for mathematics education. ZDM Mathematics Education, 41 (1–2), 105–116. https://doi.org/10.1007/s11858-008-0147-3
Greer, B., Verschaffel, L., & Mukhopadhyay, S. (2007). Modeling for life: Mathematics and children’s experience. In W. Blum, W. Henne, & M. Niss (Eds.), Applications and modeling in mathematics education (ICMI study 14) (pp. 89–98). Kluwer.
Guzey, S. S., Ring-Whalen, E. A., Harwell, M., & Peralta, Y. (2019). Life STEM: A case study of life science learning through engineering design. International Journal of Science and Mathematics Education, 17 (1), 23–42.
Hallström, J., & Schönborn, K. J. (2019). Models and modelling for authentic STEM education: reinforcing the argument. International Journal of STEM Education , 6 , 22. https://doi.org/10.1186/s40594-019-0178-z .
Hatano, G., & Oura, Y. (2003). Commentary: Reconceptualizing school learning using insight from expertise research. Educational Researcher, 32 (8), 26–29.
Hjalmarson, M. A., Holincheck, N., Baker, C. K., & Galanti, T. M. (2020). Learning models and modeling across the STEM disciplines. In C. J. Johnson, M. M. Schroeder, T. Moore, & L. D. English (Eds.), Handbook of research on STEM education (pp. 223–233). Routledge.
Hmelo-Silver, C. E., Jordan, R., Eberbach, C., & Sinha, S. (2017). Systems learning with a conceptual representation: A quasi-experimental study. Instructional Science, 45 , 53–72. https://doi.org/10.1007/s11251-016-9392-y
Jablonka, E. (2020). Critical thinking in mathematics education. In S. Lerman (Ed.), Encyclopedia of mathematics education. Springer. https://doi.org/10.1007/978-3-030-15789-0_35
Jacobson, M. J., & Wilenski, U. (2022). Complex systems and the learning sciences: Educational, theoretical, and methodological implications. In K. Sawyer (Ed.), The Cambridge handbook of the learning sciences (pp. 504–522). Cambridge University Press. https://doi.org/10.1017/9781108888295.031
Kazemi, E. (2021). Mathematical modeling with young learners: A commentary. In J. Suh, M. Wickstrom, & L. D. English (Eds.), Exploring mathematical modeming with young learners (pp. 337–342). Springer.
Kelley, T. R., & Sung, E. (2017). Examining elementary school students’ transfer of learning through engineering design using think-aloud protocol analysis. Journal of Technology Education, 28 (2), 83–108.
Kelley, T. R., & Knowles, J. G. (2016). A conceptual framework for integrated STEMeducation. International Journal of STEM Education , 3 (1), 1–11. https://doi.org/10.1186/s40594-016-0046-z .
Kennedy, N. S. (2012). What are you assuming? Mathematics Teaching in the Middle School, 18 (2), 86–91.
King, D. T., & English, L. D. (2017). Engineering design in the primary school: Applying STEM concepts to build an optical instrument. International Journal of Science Education, 38 , 2762–2794.
Koh, J. H. L., Chai, C. S., Wong, B., & Hong, H.-Y. (2015). Design thinking for education: Conceptions and applications in teaching and learning . Springer.
Book Google Scholar
Kollosche, D. (2021). Styles of reasoning for mathematics education. Educational Studies in Mathematics , 107 (3), 471–486.
Kollosche, D., & Meyerhöfer, W. (2021). COVID-19, mathematics education, and the evaluation of expert knowledge. Educational Studies in Mathematics, 108 , 401–417. https://doi.org/10.1007/s10649-021-10097-2 .
Krause, C. M., Di Martino, P., & Moschkovich, J. N. (2021). Tales from three countries: Reflections during COVID-19 for mathematical education in the future. Educational Studies in Mathematics, 108 , 87–104. https://doi.org/10.1007/s10649-021-10066-9 .
Langman, C., Zawojewski, J., McNicholas, P., Cinar, A., Brey, E., Bilgic, M., & Mehdizadeh, H. (2019). Disciplinary learning from an authentic engineering context. Journal of Pre-College Engineering Education Research, 9 , 77–94.
Larson M (2017) Math education is STEM education! NCTM president’s message . Retrieved from https://www.nctm.org/News-and-Calendar/Messages-from-the-President/Archive/Matt-Larson/Math-Education-Is-STEM-Education .
Lesh, R., & Caylor, B. (2007). Introduction to the special issue: Modeling as application versus modeling as a way to create mathematics. International Journal of Computers for Mathematical Learning, 12 (3), 173–194.
Lesh, R., English, L. D., Riggs, C., & Sevis, S. (2013). Problem solving in the primary school (K-2). Mathematics Enthusiast, 10 (1), 35–60. https://doi.org/10.54870/1551-3440.1259 .
Lesh, R., & Zawojewski, J. S. (2007). Problem solving and modeling. In F. Lester (Ed.), Second handbook of research on mathematics teaching and learning (pp. 763–804). Information Age Publishing.
Lester, F., & Cai, F. (2016). Can mathematical problem solving be taught? In P. Felmer, E. Pehkonen, & J. Kilpatrick (Eds.), Posing and solving mathematical problems (pp. 117–136). Springer.
Li, Y, Schoenfeld A H (2019) Problematizing teaching and learning mathematics as ‘given’ in STEM education. International Journal of STEM Education, 6 , 44. https://doi.org/10.1186/s40594-019-0197-9 .
Lipman, M. (2003). Thinking in education (2nd ed.). Cambridge University Press.
Lipman, M. (2008). A life teaching thinking . The Institute for the Advancement of Philosophy for Children.
Lippard, C. N., Riley, K. L., & Mann, M. H. (2018). Encouraging the development of engineering habits of mind in prekindergarten learners. In L. D. English & T. J. Moore (Eds.), Early engineering learning (pp. 19–37). Springer.
Litzinger, T. A. (2016). Thinking about a system and systems thinking in engineering. In R. A. Duschl & A. S. Bismack (Eds.), Reconceptualizing STEM education (pp. 35–48). Routledge.
Lucas, B., Claxton, G., & Hanson, J. (2014). Thinking like an engineer: Implications for the education system. A report for the Royal Academy of Engineering Standing Committee for Education and Training.
Maass, K., Geiger, V., Romero Ariza, M., & Goos, M. (2019). The role of mathematics in interdisciplinary STEM education. ZDM Mathematics Education, 51 , 869–884. https://doi.org/10.1007/s11858-019-01100-5 .
Mayes, R. (2019). Quantitative reasoning and its role in interdisciplinarity. In B. Doig, J. Williams, D. Swanson, R. Borromeo Ferri, & P. Drake (Eds.), Interdisciplinary Mathematics Education. ICME-13 Monographs . Springer. https://doi.org/10.1007/978-3-030-11066-6_8 .
McKenna, A. F. (2014). Adaptive expertise and knowledge fluency in design and innovation. In A. Johri & B. M. Olds (Eds.), Cambridge handbook of engineering education research (pp. 227–242). Cambridge University Press.
Meadows, D. (2008). Thinking in systems: A primer (Chelsea Green Publishing). https://www.chelseagreen.com/product/thinking-in-systems .
Mukhopadhyay, S., & Greer, B. (2007). How many deaths? Education for statistical empathy. In B. Sriraman (Ed.), International perspectives on social justice in mathematics education (pp. 169–189). Information Age Publishing.
National Research Council (NRC). (2012). A framework for K-12 science education: Practices, crosscutting concepts, and core ideas . National Academies Press.
National Science and Technological Council. (2018). Charting a course for success: America’s strategy for STEM education. Committee on STEM Education of the National Science and Technological Council . https://www.whitehouse.gov/wp-content/uploads/2018/12/STEM-Education-Strategic-Plan-2018.pdf .
NGSS Lead States. (2013). Next generation science standards: For states, by states (The National Academic Press). http://www.nextgenscience.org/next-generation-science-standards .
Nichols, R. K., Burgh, G., & Fynes-Clinton, L. (2019). Reconstruction of thinking across the curriculum through the community of inquiry. In M. Rollins Gregory, J. Haynes, & K. Murris (Eds.), The Routledge international handbook of philosophy for children (pp. 245–252). Routledge.
OECD (2019). PISA 2022 Mathematics Framework . https://pisa2022-maths.oecd.org/ca/index.html .
O’Reilly, C., Devitt, A., & Hayes, N. (2022). Critical thinking in the preschool classroom—A systematic literature review. Thinking Skills and Creativity. https://doi.org/10.1016/j.tsc.2022.101110 .
Organisation for Economic Co-operation and Development (OECD). (2019). Education at a glance 2019: OECD indicators . OECD.
Organisation for Economic Co-operation and Development (OECD). (2022). Thinking Outside the box: The PISA 2022 Creative Thinking Assessment . OECD.
Oxford Economics (2022). Global economic model . https://www.oxfordeconomics.com/service/subscription-services/macro/global-economic-model/ .
P21 Partnership for 21st Century Learning (2015). http://www.p21.org/our-work/p21-framework .
Park, D.-Y., Park, M.-H., & Bates, A. B. (2018). Exploring young children’s understanding about the concept of volume through engineering design in a STEM activity: A case study. International Journal of Science and Mathematics Education, 16 (2), 275–294.
Rhodes, T., & Lancaster, K. (2020). Mathematical models as public troubles in COVID-19 infection control. Health Sociology Review, 29 , 177–194.
Rhodes, T., Lancaster, K., & Rosengarten, M. (2020). A model society: Maths, models and expertise in viral outbreaks. Critical Public Health, 30 (3), 253–256.
Roberts, T., Maiorca, C., Jackson, C., & Mohr-Schroeder, M. (2022). Integrated STEM as problem-solving practices. Investigations in Mathematics Learning . https://doi.org/10.1080/19477503.2021.2024721 .
Rollins Gregory, M., Haynes, J., & Murris, K. (Eds.). (2017). The Routledge international handbook of philosophy for children . Routledge.
Shaughnessy, M. (2013). By way of introduction: Mathematics in a STEM context. Mathematics Teaching in the Middle School , 18 (6):324.
Shin, N., Bowers, J., Roderick, S., McIntyre, C., Stephens, A. L., Eidin, E., et al. (2022). A framework for supporting systems thinking and computationalthinking through constructing models. Instructional Science , 50 (6), 933–960.
Slavit, D., Grace, E., & Lesseig, K. (2021). Student ways of thinking in STEM contexts: A focus on claim making and reasoning. School Science and Mathematics, 21 (8), 466–480. https://doi.org/10.1111/ssm.12501 .
Slavit, D., Lesseig, K., & Simpson, A. (2022). An analytic framework for understanding student thinking in STEM contexts. Journal of Pedagogical Research, 6 (2), 132–148. https://doi.org/10.33902/JPR.202213536
Stehle, S. M., & Peters-Burton, E. E. (2019). Developing student 21st century skills in selected exemplary inclusive STEM high schools. International Journal of STEM Education, 6 , 39. https://doi.org/10.1186/s40594-019-0192-1
Strimel, G. J., Bartholomew, S. R., Kim, E., & Zhang, L. (2018). An investigation of engineering design cognition and achievement in primary school. Journal for STEM Education Research . https://doi.org/10.1007/s41979-018-0008-0
Stroh, D. P. (2018). The systems orientation: From curiosity to courage . https://thesystemsthinker.com/the-systems-orientation-from-curiosity-to-courage/
Svensson, M. (2022). Cross-curriculum system concepts and models. In J. Hallström & P. J. Williams (Eds.), Teaching and learning about technological systems (pp. 53–72). Springer. https://doi.org/10.1007/978-981-16-7719-9_4
Teng, B., Wang, S., Shi, Y., Sun, Y., Wang, W., Hu, W., & Shi, C. (2022). Economic recovery forecasts under impacts of COVID-19. Economic Modelling, 110 , 105821. https://doi.org/10.1016/j.econmod.2022.105821
Tornroth, S., & WikbergNilsson, A. (2022). Design thinking for the everyday aestheticisation of urban renewable energy. Design Studies, 79 , 101096. https://doi.org/10.1016/j.destud.2022.101096
Turner, E., Bennett, A., Granillo, M., et al. (2022). Authenticity of elementary teacher designed and implemented mathematical modeling tasks. Mathematical Thinking and Learning: an International Journal, 2022 , 1–24.
Turner, E., Roth McDuffie, A., Aguirre, J., Foote, M. Q., Chapelle, C., Bennett, A., Granillo, M., & Ponnuru, N. (2021). Upcycling plastic bags to make jump ropes: Elementary students leverage experiences and funds of knowledge as they engage in a relevant, community oriented mathematical modeling task. In J. Suh, M. Wickstram, & L. English (Eds.), Exploring mathematical modeling with young learners (pp. 235–266). Springer.
Turner, E., Varley, M., Simic, K., & Diaz-Palomar, J. (2009). “Everything is math in the Whole World!”: Integrating critical and community knowledge in authentic mathematical investigations with elementary latina/o students. Mathematical Thinking and Learning: An International Journal, 11 (3), 136–157.
Tytler R (2016) Drawing to learn in STEM. Proceedings of the ACER Research Conference: Improving STEM learning: What will it take? (pp. 45-50). Australian Council for Educational Research.
van Breukelen, D. H. J., de Vries, M. J., & Schure, F. A. (2017). Concept learning by direct current design challenges in secondary education. International Journal of Technology and Design Education, 27 , 407–430.
York, S., Lavi, R., Dori, Y. J., & Orgill, M. (2019). Applications of systems thinking in STEM education. Journal of Chemical Education, 96 , 2742–2751.
Zawojewski, J., Hjalmarson, M., Bowman, K. J., & Lesh, R. (Eds.). (2008). Models and modeling in engineering education . Brill.
Download references
Acknowledgements
Sentiments expressed in this article have arisen from recent Australian Research Council grants # DP 220100303 and DP 150100120. Views expressed in this article are those of the author and not the Council.
Open Access funding enabled and organized by CAUL and its Member Institutions.
Author information
Authors and affiliations.
Queensland University of Technology, Brisbane, Australia
Lyn D. English
You can also search for this author in PubMed Google Scholar
Corresponding author
Correspondence to Lyn D. English .
Ethics declarations
Conflict of interest.
There are no financial or non-financial interests that are directly or indirectly related to this submission.
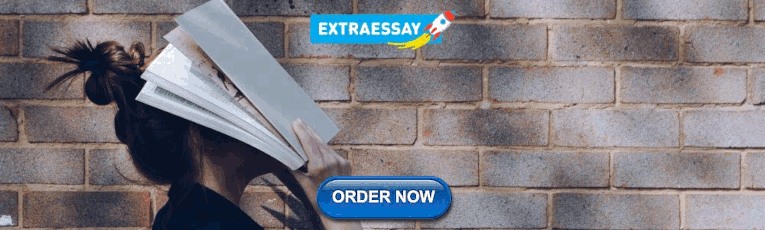
Additional information
Publisher's note.
Springer Nature remains neutral with regard to jurisdictional claims in published maps and institutional affiliations.
Rights and permissions
Open Access This article is licensed under a Creative Commons Attribution 4.0 International License, which permits use, sharing, adaptation, distribution and reproduction in any medium or format, as long as you give appropriate credit to the original author(s) and the source, provide a link to the Creative Commons licence, and indicate if changes were made. The images or other third party material in this article are included in the article's Creative Commons licence, unless indicated otherwise in a credit line to the material. If material is not included in the article's Creative Commons licence and your intended use is not permitted by statutory regulation or exceeds the permitted use, you will need to obtain permission directly from the copyright holder. To view a copy of this licence, visit http://creativecommons.org/licenses/by/4.0/ .
Reprints and permissions
About this article
English, L.D. Ways of thinking in STEM-based problem solving. ZDM Mathematics Education 55 , 1219–1230 (2023). https://doi.org/10.1007/s11858-023-01474-7
Download citation
Accepted : 16 February 2023
Published : 03 March 2023
Issue Date : December 2023
DOI : https://doi.org/10.1007/s11858-023-01474-7
Share this article
Anyone you share the following link with will be able to read this content:
Sorry, a shareable link is not currently available for this article.
Provided by the Springer Nature SharedIt content-sharing initiative
- Problem solving
- Mathematical modelling
- Critical thinking
- Systems thinking
- Design-based thinking
- Adaptive and innovative thinking
- Find a journal
- Publish with us
- Track your research
- STEM Ambassadors
- School trusts
- ITE and governors
- Invest in schools
- STEM careers inspiration
- Benefits and impact
- Our supporters
- Become a STEM Ambassador
- Request a STEM Ambassador
- Employer information
- Training and support
- STEM Ambassadors Partners
- Working with community groups
- Search icon
- Join the STEM Community
Thinking Through Mathematics
These resources, produced by The National Centre for Excellence in the Teaching of Mathematics (NCETM), have been designed to encourage distinctive ways of thinking and learning as part of the NCETM professional development package .
Analysing reasoning and solutions sets a number of problems about proportion and percentages and gives a range of student responses to each problem. The examples provide inspiration for further discussion about student misconceptions .
Classifying contains a number of cards each containing a different shapes. Students are asked to place the cards into categories. The task is inspiration for teachers to discuss how the teacher should proceed with the activity. The second activity, classifying using two-way tables, requires students to place the cards into a two-way table according to the shape’s properties.
Creating and solving problems explains the ‘doing’ and ‘undoing’ processes in mathematics giving three examples of a problem poser where a closed problem is posed and the problem solver where the problem is posed in a more open format.
Evaluat i ng mathematical statements gives a number of examples for which it has to be decided whether the statement is always true, sometimes true or never true. A further example is given in which each statement has to be categorised as true, false or unsure.
Interpreting multiple representations contains an example of an activity in which students are asked to take it in turns to match up cards. One set of cards contain decimal numbers, one set contain diagrams of shaded squares and one set contains arrows on a number line.
As part of the NCETM course teachers were asked to make a similar activity for another area of mathematics.
Show health and safety information
Please be aware that resources have been published on the website in the form that they were originally supplied. This means that procedures reflect general practice and standards applicable at the time resources were produced and cannot be assumed to be acceptable today. Website users are fully responsible for ensuring that any activity, including practical work, which they carry out is in accordance with current regulations related to health and safety and that an appropriate risk assessment has been carried out.
Show downloads
Subject(s) | Mathematics |
---|---|
Age | 7-11, 11-14, 14-16 |
Published | 2010 to 2019 |
Published by | |
Direct URL |
Share this resource
Did you like this resource.
Welcome to Leaf and STEM Learning!
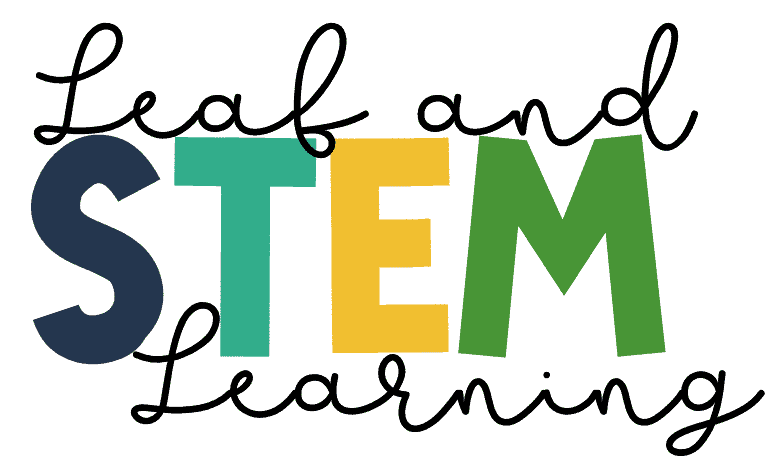
Solving Proportion Word Problems with Sentence Stems
- No Comments
- Math Professional Development , Teacher Tips

Teaching proportionality is a difficult task with any group of students, but with students who may be below their grade level in reading, teaching proportional relationships may seem to be nearly impossible. After years of working with English learners in the math classroom, a simple sentence stem may be all you need to bridge the gap when teaching your proportions unit this school year.
My students were always looking for patterns in word problems—quick fixes that would “tell” them exactly what they were supposed to be doing. As a teacher, this often made me crazy. The story problems just did not work like that. Once we started proportions, this student tactic was made even worse by the way the proportion problems were worded. My kids needed a pattern—a mathematically correct pattern—for analyzing proportion problems. They needed a signal that the problem that they were solving was not just about operations, but that they would need to find the information and set up a proportion.
How would they be able to tell when they are working on a problem that contained proportional relationships?
We were on the look out for questions that could fill this pattern:
When I have ________________, I also have ___________________.
Let’s look at an example from the proportionality interactive notebook set :
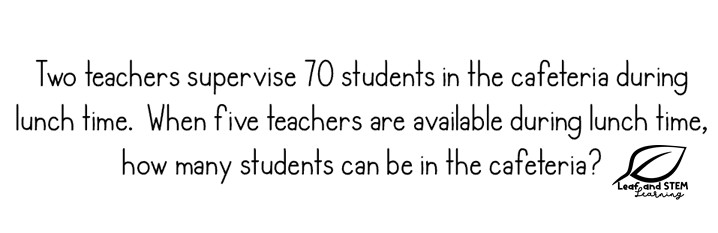
Can this word problem fill in the sentence stem? Yes, we can say that, “When I have 2 teachers, I also have 70 students.” Practice this pattern again and again with your students. Every time you are looking at a word problem, use this sentence stem with your students. As you repeat the stem, write the ratio.
Your students will start recognizing the ratio in word problems with this repeated practice.
Can your students write the proportion equation?
Once your students have mastered finding the ratio in the word problem, the next step is to add one more piece to the sentence stem.
When I have ___________________, I also have __________________, but I really have_________________.
Back to our previous example from the proportionality interactive notebook :
When I have 2 teachers, I also have 70 students, but I really have 5 teachers.
This new stem gives the students all the pieces they will need to write a proportionality equation.
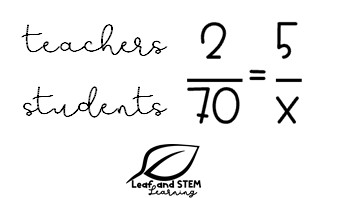
Before long, your students will be able to recognize proportional situations in word problems and analyze those problems to write proportion equations.

5th Grade Financial Literacy Activities
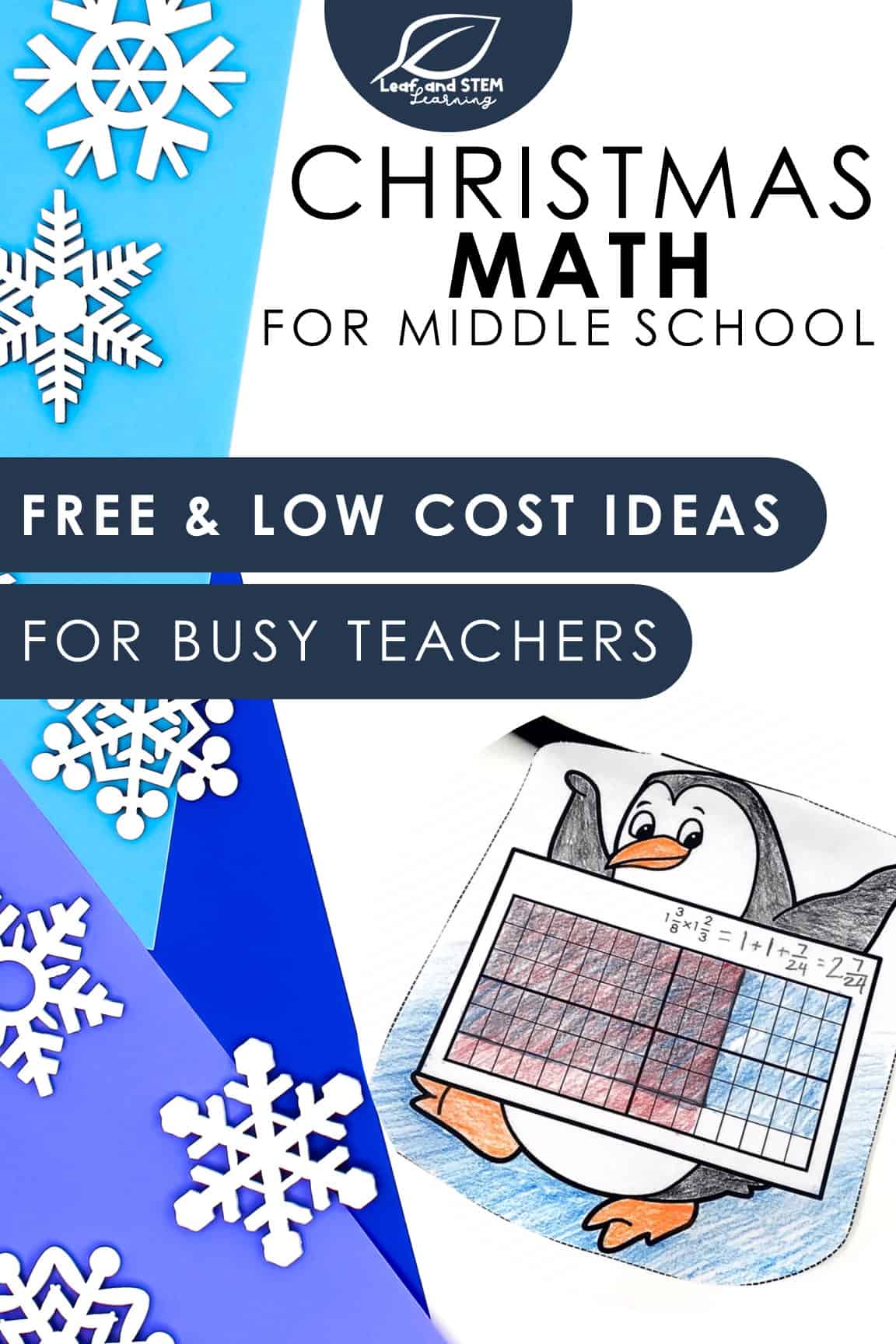
Christmas Math Activities for Middle Schoolers
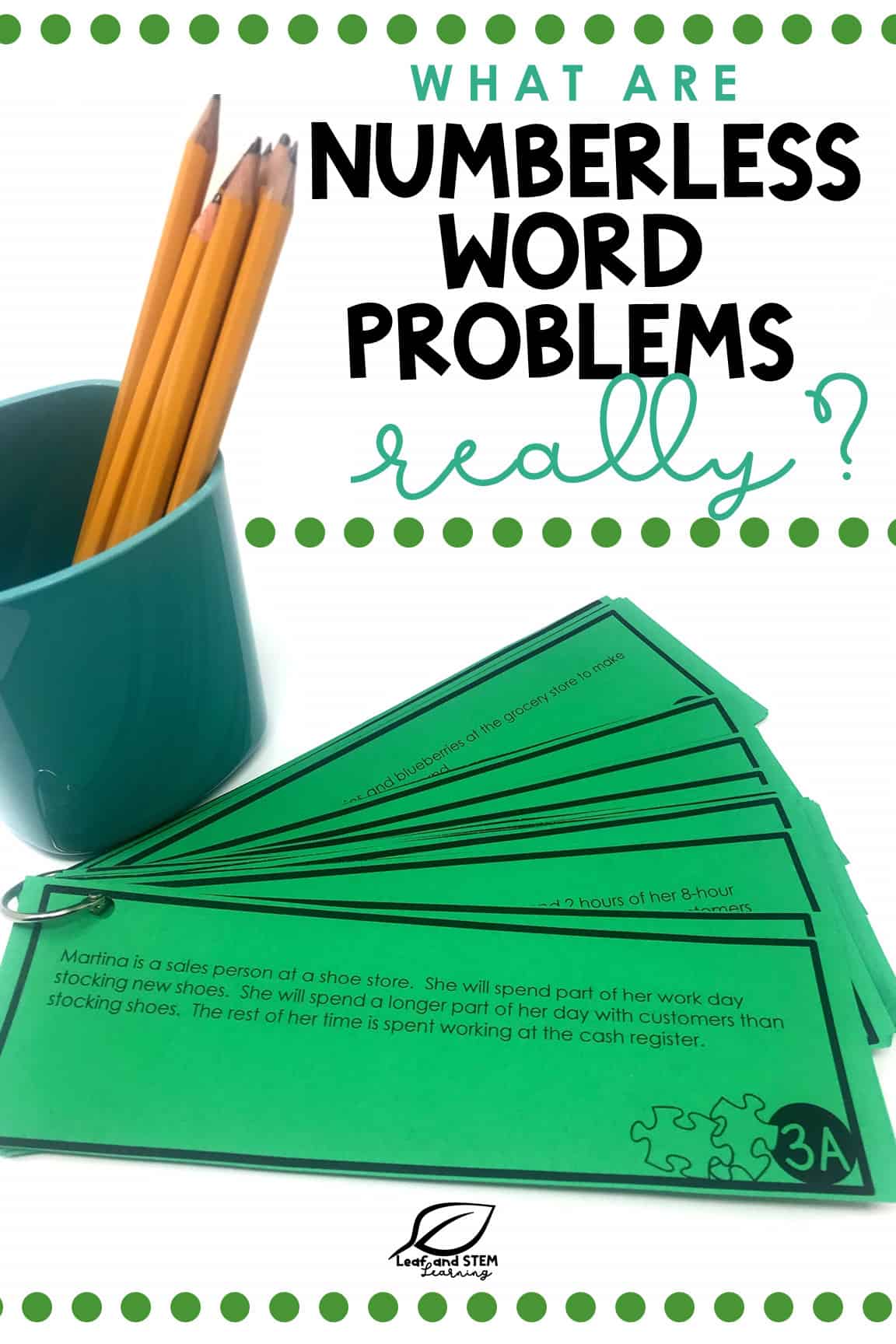
What are Numberless Word Problems Really?
Check out more posts, leave a comment cancel reply, ©2020 leaf and stem learning. all rights reserved. designed by ashley hughes..
- Grades 6-12
- School Leaders
Win a $100 gift card each day this month! 🎁
How To Use Sentence Stems (Plus 60+ Examples for Every Subject)
Sentence stems help students …
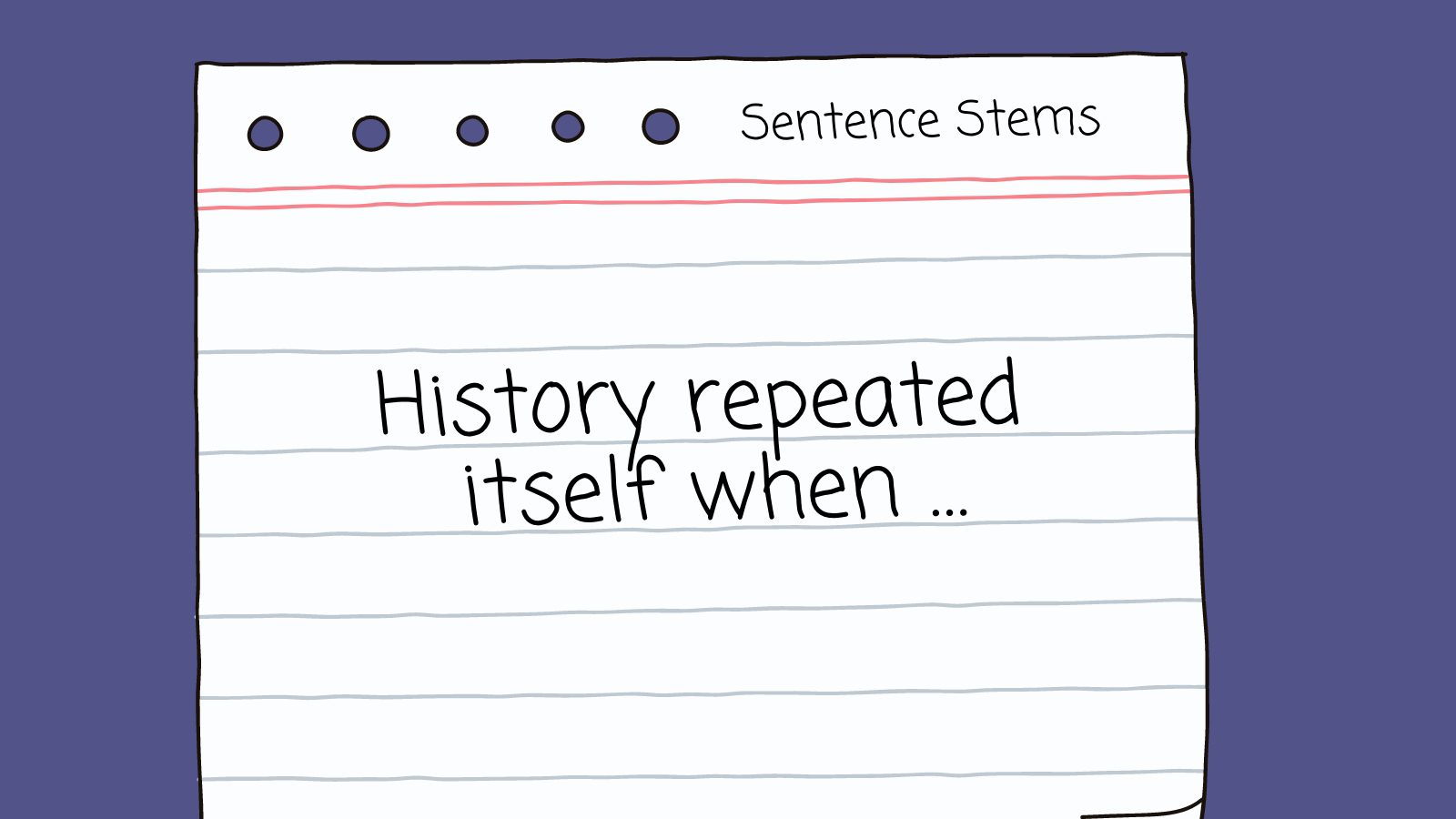
Some students have no trouble expressing their thoughts, whether verbally or in writing. Others, though, need some help to get started. Sentence stems—sometimes called sentence starters, sentence frames, or thinking stems—help them do just that. Here’s how they work.
How To Use Sentence Stems
Provide all students with a list of sentence stems they can use during discussions or when they’re writing. For fluent writers or speakers, these stems may not be necessary, but they’re always helpful to have on hand. You can give kids a handout, or post an anchor chart for them to refer back to.
Be sure to model them for kids, showing how to fill in the blanks. Practice both out loud and in writing, providing your own example and then asking for their take. It’s OK if they simply fill in the blanks when they first start using these stems. But over time, students should use them more as a jumping-off point, to delve deeper into the topic.
Tip: Don’t require sentence stems if students don’t need them. Some kids will do just fine having a discussion on their own, or getting started on a writing assignment. But encourage others to use them as much as they need to. Eventually, these stems will become automatic, giving students tools to help them become better communicators.
Language Arts Sentence Stems
- The author used this technique because …
- The narrator is reliable/unreliable because …
- Characters in this story started out … but changed to …
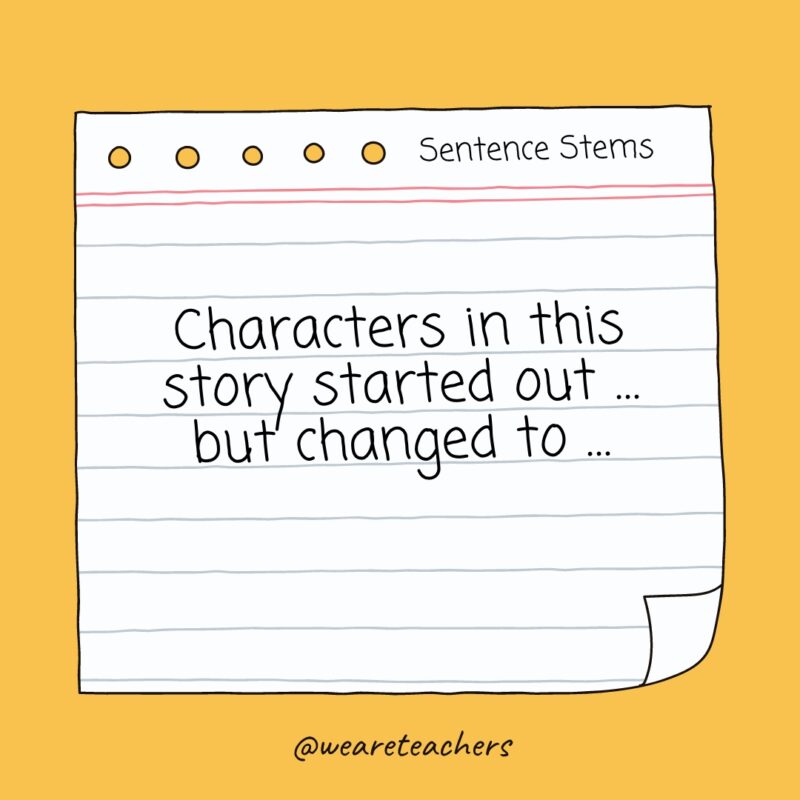
- The theme of this story is … which is shown by …
- This story reminds me of …
- If could change the end of this story, I would …
- I liked/disliked this character because …
- This story made me feel … when …
- [Character] and [character] are alike/different because …
- My favorite part was …
- The author wants us to believe …
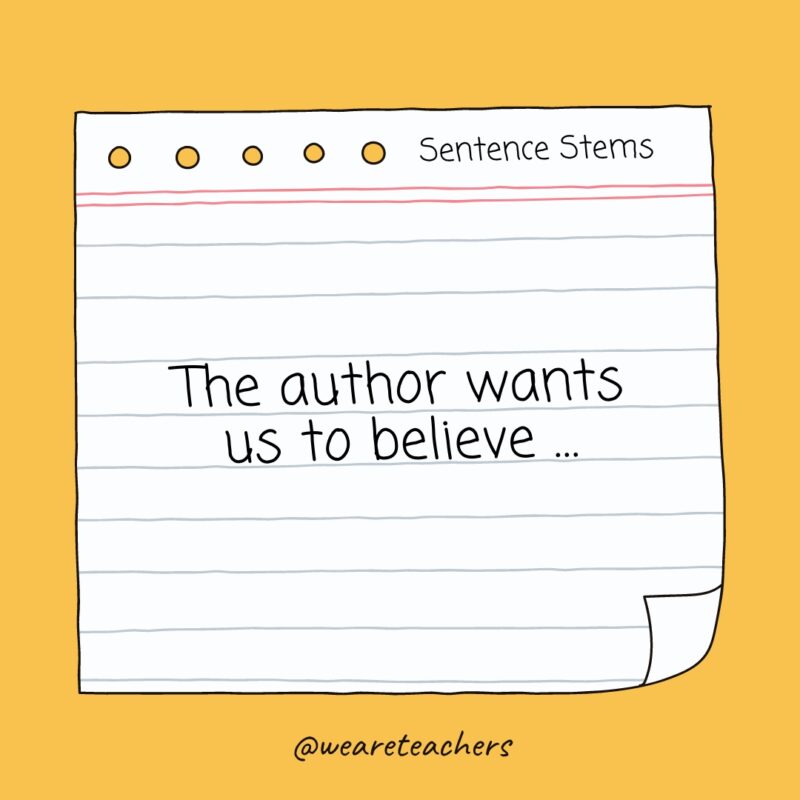
- Based on this text …
- The author proves their point by saying …
- I didn’t understand why …
- When I read … what I pictured in my head was …
Social Studies Sentence Stems
- These events are alike/different because …
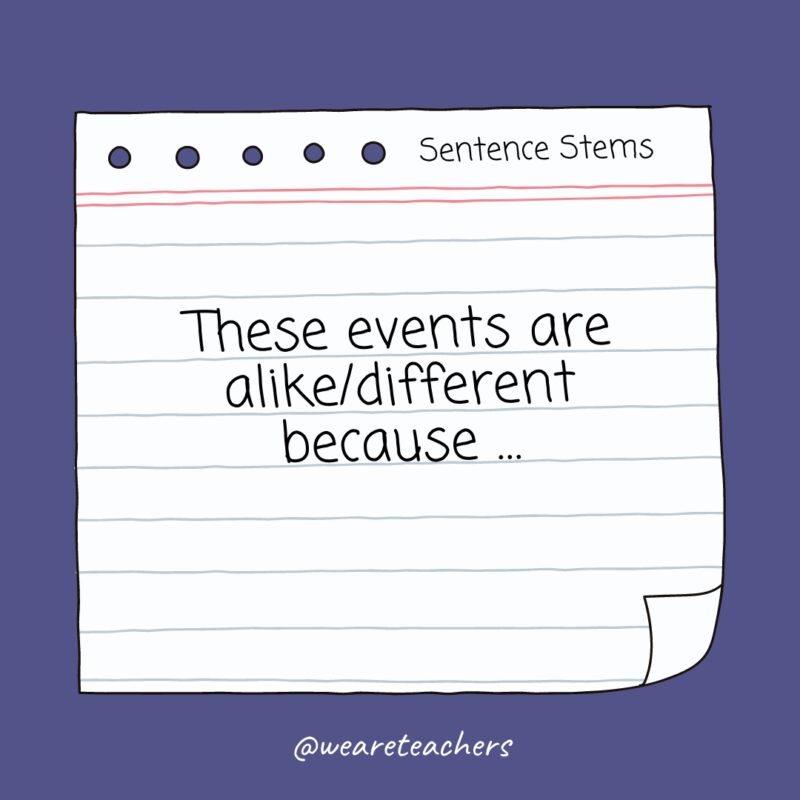
- If this happened today …
- If I lived then, I would have …
- This happened because …
- The effects of this were …
- I agree/disagree with … because …
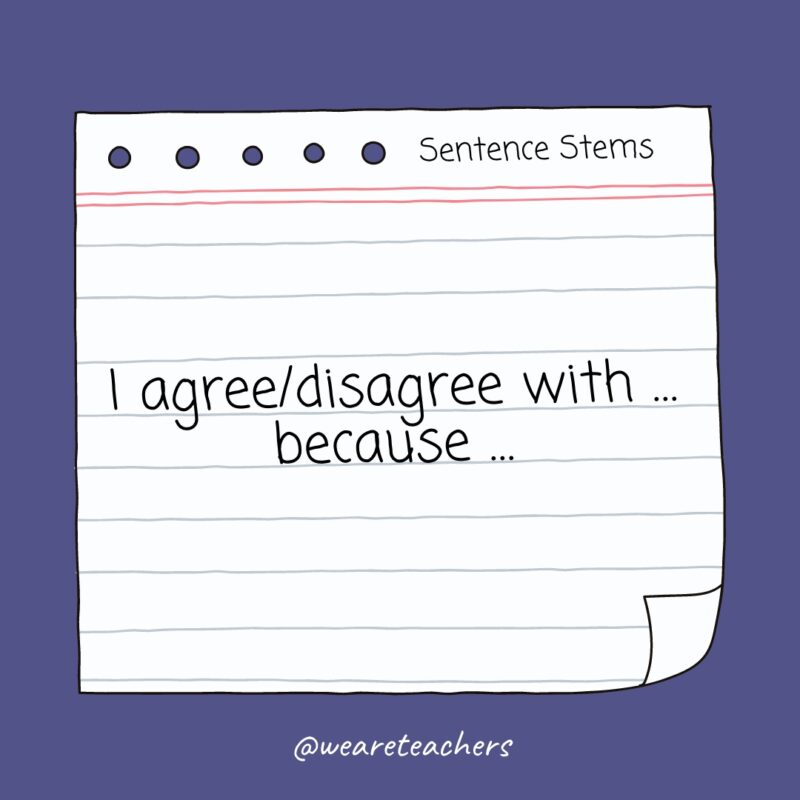
- I was surprised to learn …
- I don’t understand why …
- History repeated itself when …
- I feel inspired by …
Science Sentence Stems
- The data shows …
- This experiment proved … because …
- I believe … will happen because …
- I expect to see …
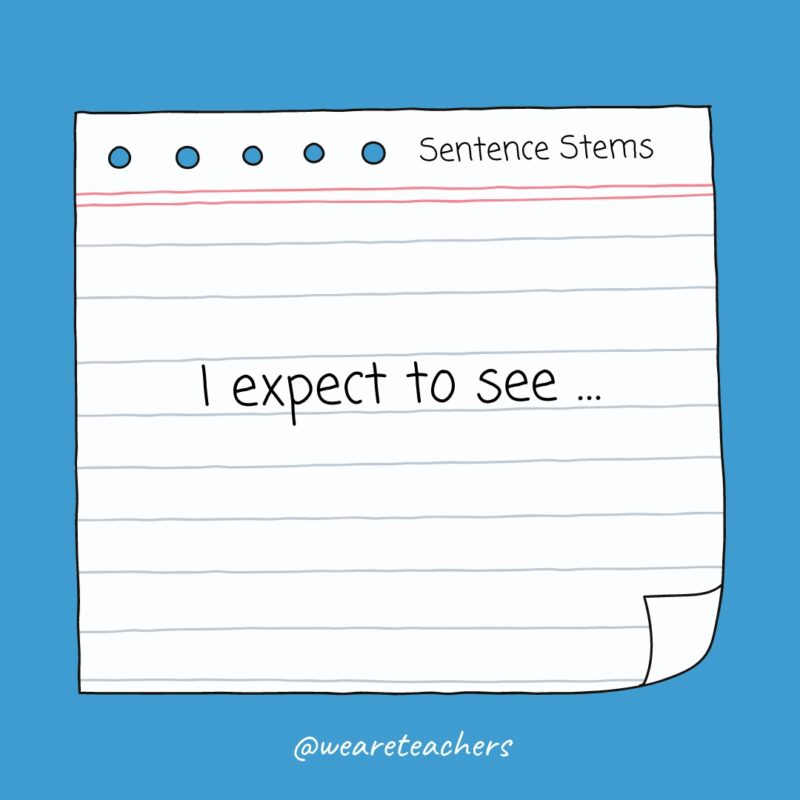
- The cause of this is …
- The effect of this is …
- I was surprised when …
- We can find out by …
- We could change the outcome by …
- I discovered …
- The pattern I observed was …
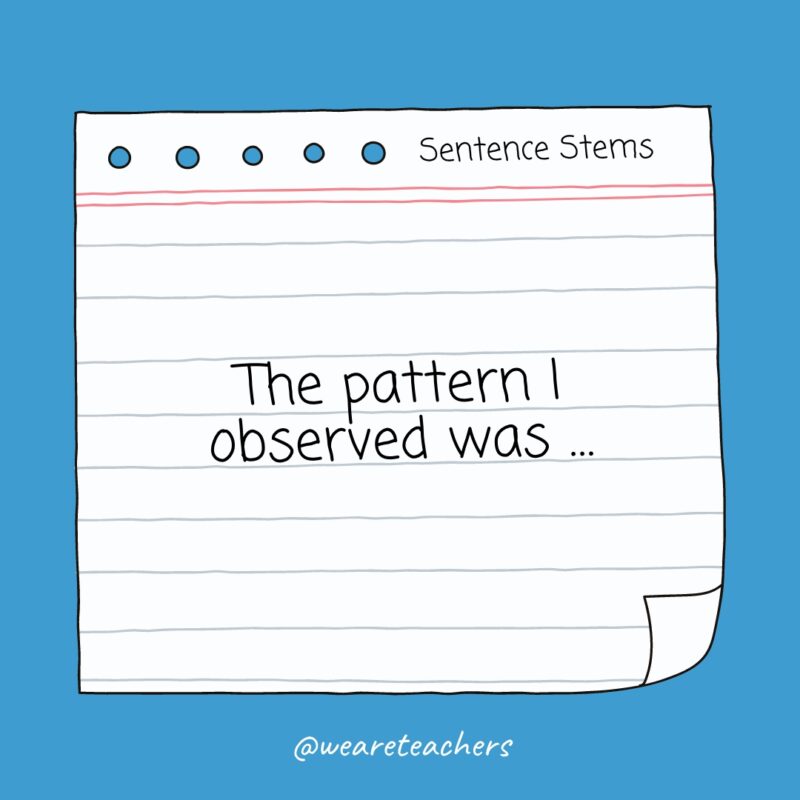
- My results show …
- I predict … because …
- The experiment was a success/failure because …
Math Sentence Stems
- To solve this problem, I need to …
- The important information is …
- I can check my work by …
- Another way to solve this problem is …
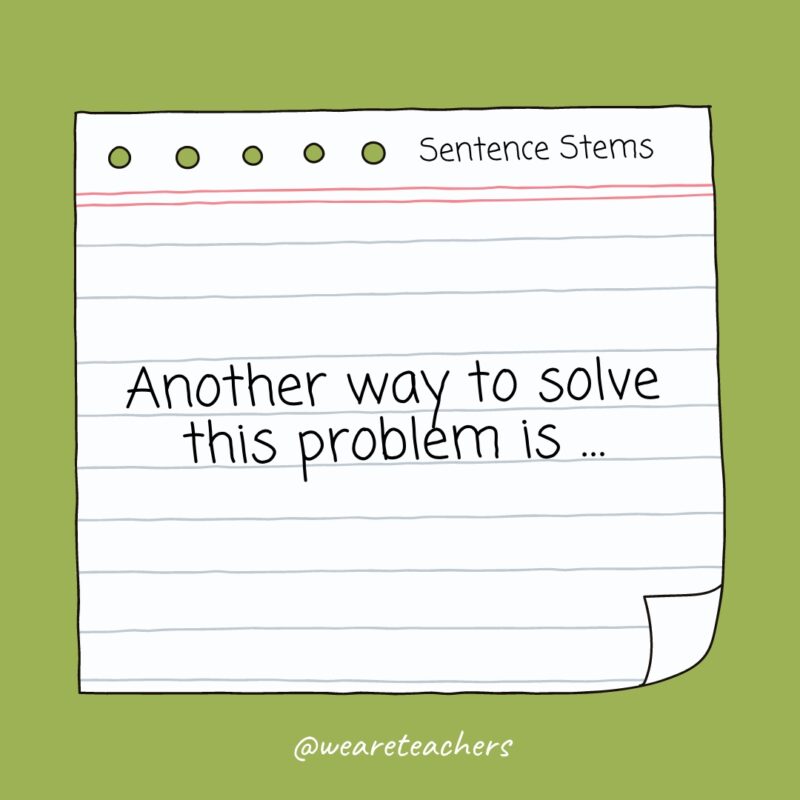
- First I … Then I … Finally I …
- I found the answer by …
- This problem reminded me of …
- I can use this skill in real life when …
- I got the wrong answer because …
- This solution makes/doesn’t make sense because …
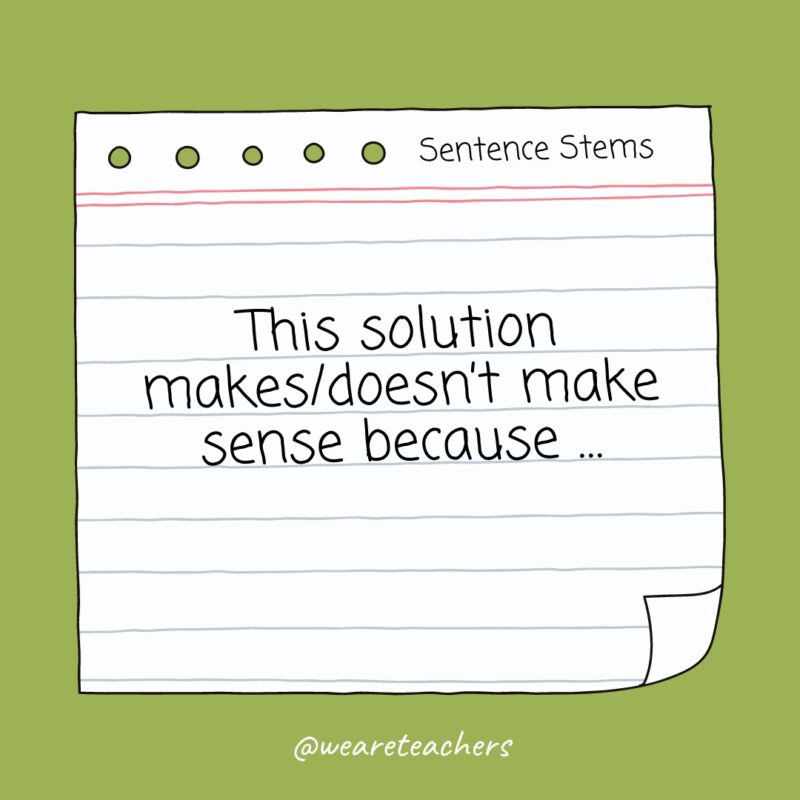
Discussion Sentence Stems
- When you said … I felt …
- I used to think … Now I think …
- I feel … when …
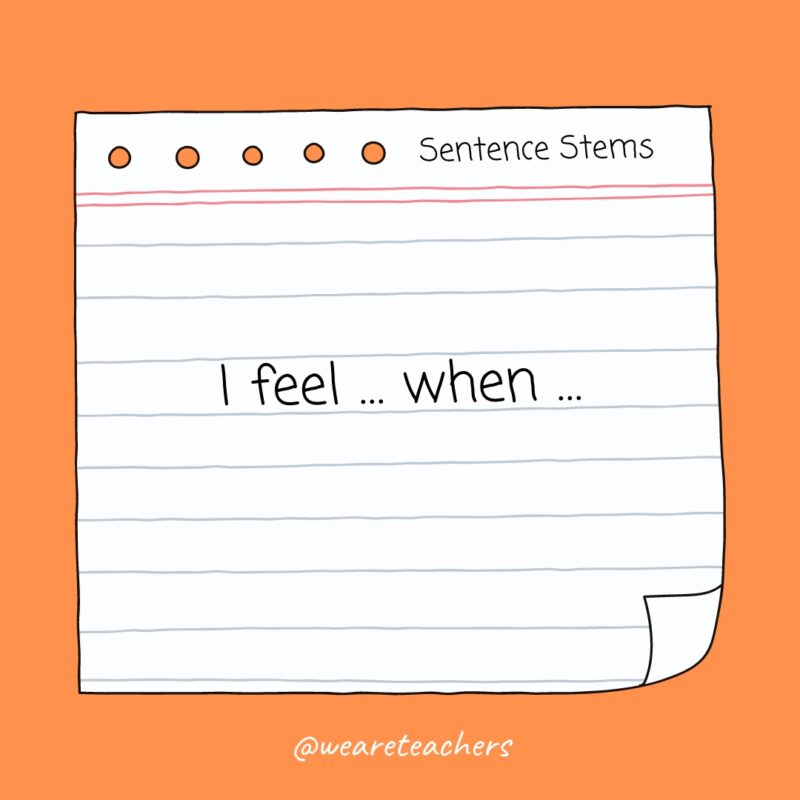
- We are alike/different because …
- I respect your opinion, but I disagree because …
- From my point of view …
- What I’m hearing you say is …
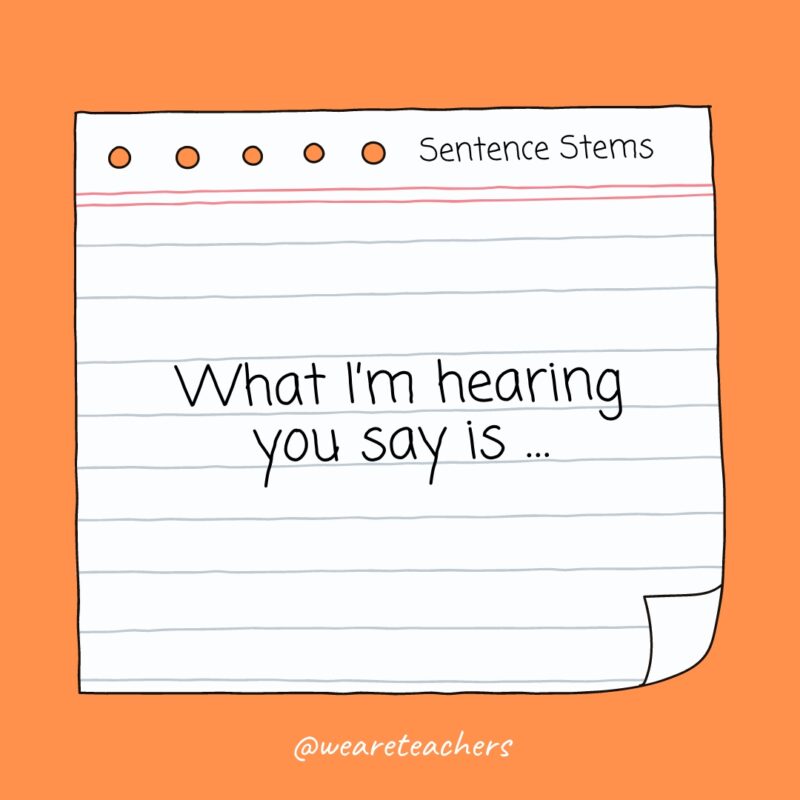
- If that happened to me, I would …
- What if we …
- I need some help with …
How do you use sentence stems? Come share your thoughts and ask for advice in the WeAreTeachers HELPLINE group on Facebook .
Plus, 40 must-have anchor charts for teaching writing of all kinds ., you might also like.
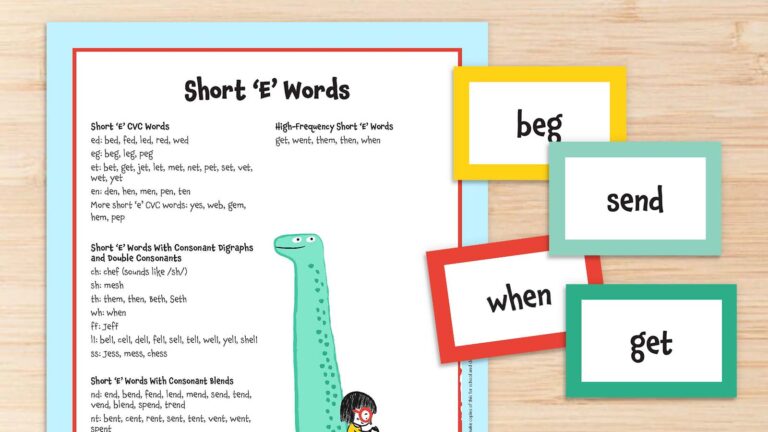
Short ‘E’ Words (List, Teaching Ideas, and Free Printables)
This tricky short vowel takes lots of practice! Continue Reading
Copyright © 2024. All rights reserved. 5335 Gate Parkway, Jacksonville, FL 32256
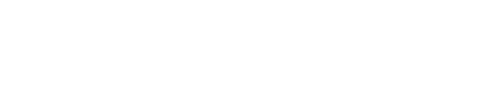
Mathematical Reasoning & Problem Solving
In this lesson, we’ll discuss mathematical reasoning and methods of problem solving with an eye toward helping your students make the best use of their reasoning skills when it comes to tackling complex problems.
Previously Covered:
- Over the course of the previous lesson, we reviewed some basics about chance and probability, as well as some basics about sampling, surveys, etc. We also covered some ideas about data sets, how they’re represented, and how to interpret the results.
Approaches to Problem Solving
When solving a mathematical problem, it is very common for a student to feel overwhelmed by the information or lack a clear idea about how to get started.
To help the students with their problem-solving “problem,” let’s look at some examples of mathematical problems and some general methods for solving problems:
Identify the following four-digit number when presented with the following information:
- One of the four digits is a 1.
- The digit in the hundreds place is three times the digit in the thousands place.
- The digit in the ones place is four times the digit in the ten’s place.
- The sum of all four digits is 13.
- The digit 2 is in the thousands place.
Help your students identify and prioritize the information presented.
In this particular example, we want to look for concrete information. Clue #1 tells us that one digit is a 1, but we’re not sure of its location, so we see if we can find a clue with more concrete information.
We can see that clue #5 gives us that kind of information and is the only clue that does, so we start from there.
Because this clue tells us that the thousands place digit is 2, we search for clues relevant to this clue. Clue #2 tells us that the digit in the hundreds place is three times that of the thousands place digit, so it is 6.
So now we need to find the tens and ones place digits, and see that clue #3 tells us that the digit in the ones place is four times the digit in the tens place. But we remember that clue #1 tells us that there’s a one somewhere, and since one is not four times any digit, we see that the one must be in the tens place, which leads us to the conclusion that the digit in the ones place is four. So then we conclude that our number is:
If you were following closely, you would notice that clue #4 was never used. It is a nice way to check our answer, since the digits of 2614 do indeed add up to be thirteen, but we did not need this clue to solve the problem.
Recall that the clues’ relevance were identified and prioritized as follows:
- clue #3 and clue #1
By identifying and prioritizing information, we were able to make the information given in the problem seem less overwhelming. We ordered the clues by relevance, with the most relevant clue providing us with a starting point to solve the problem. This method also utilized the more general method of breaking a problem into smaller and simpler parts to make it easier to solve.
Now let’s look at another mathematical problem and another general problem-solving method to help us solve it:
Two trees with heights of 20 m and 30 m respectively have ropes running from the top of each tree to the bottom of the other tree. The trees are 40 meters apart. We’ll assume that the ropes are pulled tight enough that we can ignore any bending or drooping. How high above the ground do the ropes intersect?
Let’s solve this problem by representing it in a visual way , in this case, a diagram:
You can see that we have a much simpler problem on our hands after drawing the diagram. A, B, C, D, E, and F are vertices of the triangles in the diagram. Now also notice that:
b = the base of triangle EFA
h = the height of triangle EFA and the height above the ground at which the ropes intersect
If we had not drawn this diagram, it would have been very hard to solve this problem, since we need the triangles and their properties to solve for h. Also, this diagram allows us to see that triangle BCA is similar to triangle EFC, and triangle DCA is similar to triangle EFA. Solving for h shows that the ropes intersect twelve meters above the ground.
Students frequently complain that mathematics is too difficult for them, because it is too abstract and unapproachable. Explaining mathematical reasoning and problem solving by using a variety of methods , such as words, numbers, symbols, charts, graphs, tables, diagrams, and concrete models can help students understand the problem better by making it more concrete and approachable.
Let’s try another one.
Given a pickle jar filled with marbles, about how many marbles does the jar contain?
Problems like this one require the student to make and use estimations . In this case, an estimation is all that is required, although, in more complex problems, estimates may help the student arrive at the final answer.
How would a student do this? A good estimation can be found by counting how many marbles are on the base of the jar and multiplying that by the number of marbles that make up the height of the marbles in the jar.
Now to make sure that we understand when and how to use these methods, let’s solve a problem on our own:
How many more faces does a cube have than a square pyramid?
Reveal Answer
The answer is B. To see how many more faces a cube has than a square pyramid, it is best to draw a diagram of a square pyramid and a cube:
From the diagrams above, we can see that the square pyramid has five faces and the cube has six. Therefore, the cube has one more face, so the answer is B.
Before we start having the same problem our model student in the beginning did—that is, being overwhelmed with too much information—let’s have a quick review of all the problem-solving methods we’ve discussed so far:
- Sort and prioritize relevant and irrelevant information.
- Represent a problem in different ways, such as words, symbols, concrete models, and diagrams.
- Generate and use estimations to find solutions to mathematical problems.
Mathematical Mistakes
Along with learning methods and tools for solving mathematical problems, it is important to recognize and avoid ways to make mathematical errors. This section will review some common errors.
Circular Arguments
These involve drawing a conclusion from a premise that is itself dependent on the conclusion. In other words, you are not actually proving anything. Circular reasoning often looks like deductive reasoning, but a quick examination will reveal that it’s far from it. Consider the following argument:
- Premise: Only an untrustworthy man would become an insurance salesman; the fact that insurance salesmen cannot be trusted is proof of this.
- Conclusion: Therefore, insurance salesmen cannot be trusted.
While this may be a simplistic example, you can see that there’s no logical procession in a circular argument.
Assuming the Truth of the Converse
Simply put: The fact that A implies B doesn’t not necessarily mean that B implies A. For example, “All dogs are mammals; therefore, all mammals are dogs.”
Assuming the Truth of the Inverse
Watch out for this one. You cannot automatically assume the inverse of a given statement is true. Consider the following true statement:
If you grew up in Minnesota , you’ve seen snow.
Now, notice that the inverse of this statement is not necessarily true:
If you didn’t grow up in Minnesota , you’ve never seen snow.
Faulty Generalizations
This mistake (also known as inductive fallacy) can take many forms, the most common being assuming a general rule based on a specific instance: (“Bridge is a hard game; therefore, all card games are difficult.”) Be aware of more subtle forms of faulty generalizations.
Faulty Analogies
It’s a mistake to assume that because two things are alike in one respect that they are necessarily alike in other ways too. Consider the faulty analogy below:
People who absolutely have to have a cup of coffee in the morning to get going are as bad as alcoholics who can’t cope without drinking.
False (or tenuous) analogies are often used in persuasive arguments.
Now that we’ve gone over some common mathematical mistakes, let’s look at some correct and effective ways to use mathematical reasoning.
Let’s look at basic logic, its operations, some fundamental laws, and the rules of logic that help us prove statements and deduce the truth. First off, there are two different styles of proofs: direct and indirect .
Whether it’s a direct or indirect proof, the engine that drives the proof is the if-then structure of a logical statement. In formal logic, you’ll see the format using the letters p and q, representing statements, as in:
If p, then q
An arrow is used to indicate that q is derived from p, like this:
This would be the general form of many types of logical statements that would be similar to: “if Joe has 5 cents, then Joe has a nickel or Joe has 5 pennies “. Basically, a proof is a flow of implications starting with the statement p and ending with the statement q. The stepping stones we use to link these statements in a logical proof on the way are called axioms or postulates , which are accepted logical tools.
A direct proof will attempt to lay out the shortest number of steps between p and q.
The goal of an indirect proof is exactly the same—it wants to show that q follows from p; however, it goes about it in a different manner. An indirect proof also goes by the names “proof by contradiction” or reductio ad absurdum . This type of proof assumes that the opposite of what you want to prove is true, and then shows that this is untenable or absurd, so, in fact, your original statement must be true.
Let’s see how this works using the isosceles triangle below. The indirect proof assumption is in bold.
Given: Triangle ABC is isosceles with B marking the vertex
Prove: Angles A and C are congruent.
Now, let’s work through this, matching our statements with our reasons.
- Triangle ABC is isosceles . . . . . . . . . . . . Given
- Angle A is the vertex . . . . . . . . . . . . . . . . Given
- Angles A and C are not congruent . . Indirect proof assumption
- Line AB is equal to line BC . . . . . . . . . . . Legs of an isosceles triangle are congruent
- Angles A and C are congruent . . . . . . . . The angles opposite congruent sides of a triangle are congruent
- Contradiction . . . . . . . . . . . . . . . . . . . . . . Angles can’t be congruent and incongruent
- Angles A and C are indeed congruent . . . The indirect proof assumption (step 3) is wrong
- Therefore, if angles A and C are not incongruent, they are congruent.
“Always, Sometimes, and Never”
Some math problems work on the mechanics that statements are “always”, “sometimes” and “never” true.
Example: x < x 2 for all real numbers x
We may be tempted to say that this statement is “always” true, because by choosing different values of x, like -2 and 3, we see that:
Example: For all primes x ≥ 3, x is odd.
This statement is “always” true. The only prime that is not odd is two. If we had a prime x ≥ 3 that is not odd, it would be divisible by two, which would make x not prime.
- Know and be able to identify common mathematical errors, such as circular arguments, assuming the truth of the converse, assuming the truth of the inverse, making faulty generalizations, and faulty use of analogical reasoning.
- Be familiar with direct proofs and indirect proofs (proof by contradiction).
- Be able to work with problems to identify “always,” “sometimes,” and “never” statements.
Facebook Twitter Email Youtube Pinterest LinkedIn
- What's New on CADREK12.org
- Announcements
- DRK-12 Projects
- DRK-12 Project Members
- DRK-12 Community Voices Blog
- CADRE Fellows
- CADRE Postdocs
- NSF CAREER Program & DRK-12
- Early Career Resources
- Early Career News & Opportunities
- NSF Proposal Toolkit
- Project Management Toolkit
- Dissemination Toolkit
- Research Toolkit
- Spotlights on STEM Education Research
- CADRE Products
- DRK-12 Research and Products
- Educator Resources
- CADRE, NSF & NSF Network Events
- About CADRE
- STEM Smart Initiative
Problem Solving in STEM Education
In this Spotlight...
- Animated Contrasting Cases to Improve Procedural and Conceptual Knowledge in Geometry (AC²inG) (PI: Erin Krupa)
- Building Informed Designers (PI: Blake Hylton)
- CAREER: Noticing and Using Students’ Prior Knowledge in Problem-Based Instruction (PI: Gloriana González)
- COnceptual Model-based Math Intervention Tutor (COMMIT) (PI: Yan Ping Xin)
- DEAP: Developing and Evaluating Assessments of Problem Solving (PIs: Jonathan Bostic, Toni Sondergeld)
- Ed+gineering: An Interdisciplinary Partnership Integrating Engineering into Elementary Teacher Preparation Programs (PI: Jennifer Kidd)
- FLECKS: Fostering Collaborative Computer Science Learning with Intelligent Virtual Companions for Upper Elementary Students (PIs: Kristy Boyer, Eric Wiebe)
- Improving Grades 6-8 Students' Mathematics Achievement in Modeling and Problem Solving through Effective Sequencing of Instructional Practices (PI: Joe Champion)
- Mathematical and Computational Methods for Planning a Sustainable Future (PS-Future) (PI: Margaret Cozzens)
- Mathematical Learning via Architectural Design and Modeling Using E-Rebuild (PI: Fengfeng Ke)
- Proportions Playground (PI: Chandra Orrill)
- Science and Engineering Education for Infrastructure Transformation (PI: Charles Xie)
- Systemic Transformation of Inquiry Learning Environments for STEM (PI: Ellen Meier)
- Zoombinis: The Implementation Research Study of a Computational Thinking Game for Upper Elementary and Middle School Learners (PI: Jodi Asbell-Clarke)
Additional Resources
Featured projects, animated contrasting cases to improve procedural and conceptual knowledge in geometry (ac²ing) (nsf #1907745).
PI: Erin Krupa | Co-PI: Jon Star Target Audience: Middle school students and teachers STEM Discipline: Mathematics

In our materials, two fictional students each present a unique solution strategy to a geometry problem. Middle school students analyze both methods and discuss similarities, differences, strengths, and weaknesses of each strategy. This research is based on positive outcomes of using contrasting cases to support the learning of algebra. The AC2inG project tests the conjecture that utilizing contrasting cases is a viable strategy for improving the learning of geometry. Further, AC2inG materials will be digital, so students will see the concepts come to life through rich visual animations.
Theoretical Framework:
- Multiple Solution Strategies : Mathematics education literature often advocates for the use of multiple solution strategies or tools to approach a mathematics problem (Dhombres, 1993; House & Coxford, 1995; NCTM, 2000; Polya, 1973, 1981; Pólya, 1963; A. H. Schoenfeld, 1983, 1988; Vinner, 1989).
- Contrasting Cases as Learning Tool : Goldstone, Day, and Son (2010) stated, “research has demonstrated that the simple act of comparing two things can produce important changes in our knowledge” (p. 103). There is empirical support from cognitive scientist literature for the use of comparing contrasting examples for learning about business negotiations (Gentner et al., 2003), heat flow in science (Kurtz et al., 2001), children’s learning (Loewenstein & Gentner, 2001; Namy & Gentner, 2002), and in studies of infants 4 to 6-months old (Oakes & Ribar, 2005).
- Contrasting Cases in Mathematics Education : In mathematics education, research on comparing has proven effective in learning: estimation (Star & Rittle-Johnson, 2008, March, 2009), the concept of an altitude for a triangle (Guo & Pang, 2011), and equation solving (Rittle-Johnson & Star, 2007, 2009; Rittle-Johnson, Star, & Durkin, 2012). Aside from the work of Star and colleagues, there are very few experimental classroom-based studies on the effects of the Contrasting Cases approach on gains in students’ knowledge, and only one such study exists in geometry (Guo & Pang, 2011).
Methodology: Overall, we are utilizing design-based research as we develop and test the materials (Brown, 1992; Cobb, Confrey, diSessa, Lehrer, & Schauble, 2003; Collins, 1992; Schoenfeld, 2006). We used a theory-driven process to design our materials and will use a data-driven process to iteratively refine them (Shavelson & Towne, 2002). Through a series of revisions and implementations, we will test and refine our conjectures about how, and what, students learn when interacting with the geometry cases, with the ultimate goal of improving their procedural and conceptual knowledge.
To obtain the data that will inform our revisions, we will use a quasi-experimental design that is similar to prior Contrasting Cases work in algebra (e.g., Rittle-Johnson & Star, 2007); half the students will be in the Sequential group and the other half in the Compare group. The Sequential group will be presented with a page with one student’s solution to a problem, a discussion sheet, a separate page with another student’s solution, and a second discussion sheet. The Compare group will be presented with the supplemental curriculum that includes: a page with one student’s solution to a problem, a page with another student’s solution to a problem, an in-depth side-by-side comparison sheet of the two solutions, a discussion sheet, and a ‘closure’ page.
All students will be administered lesson-level, unit-level, and course-level pre- and post-assessments. In addition, students will be allowed to write or draw on the AC2inG instructional materials, and all lessons will be audio-recorded. After instruction with the animated contrasting cases, we will conduct and audio-record interviews with small groups of students in order to explore students’ geometric thinking. A mixed-methods approach will be used to analyze the data that is collected in order to provide evidence of effectiveness of the supplemental materials on students’ procedural and conceptual knowledge of geometry.
Current Findings Related to Problem Solving: We are in the first of three years of the grant. To date, we have created paper-based curricular materials for all 8th grade geometry units: Angles, Volume, Transformations, and Pythagorean Theorem. We have created animation scripts that are currently under the third round of revisions with our digital animator, and we hope to have these finalized in time for the 2020-2021 school year.
Key Challenge: The most obvious current challenge is the COVID-19 pandemic. When schools closed, we were unable to implement our materials with students. To overcome this, we are prepared to conduct think-aloud interviews with students engaging in our digital materials once those have been finalized.
Products: Project Website | Curricular Materials
Building Informed Designers (NSF #1812823)
PI: J. Blake Hylton | Co-PIs: Todd France , Patrick Herak , Bruce Wellman Target Audience: Grades 9-12 STEM Discipline: Infusing engineering content into physics, chemistry, biology, and physical science curricula
Description: This project is developing engineering problem framing activities for high school science classrooms and associated professional development for teachers. Teacher participants are brought in for a three day workshop, where they receive training on engineering content, complete an engineering design experience, walk through the materials, and work to develop an implementation plan for connecting the engineering content to their regular science content. Teachers meet with a team member for continued mentorship at least twice more as they work to enact their implementation plan. Curricular materials are currently undergoing a transformation towards a hybrid synchronous/asynchronous mode using interactive modules deployed via LMS. Materials are being developed for several different problem contexts, to allow tailoring to regular course content, and with multiple levels of proficiency, to allow students to revisit similar content at a higher level in later courses in the curriculum. In addition to analyzing student artifacts, the project also captures student attitudes about engineering as a career path, using the STEM-CIS instrument, and about engineering design, using a modified version of an established instrument based on expectancy-value theory. The project goal is to explore how students' attitudes and abilities shift through repeated exposure across the high school curriculum.
Theoretical Framework & Methodology: Student attitudes about engineering design are explored using a survey based on expectancy-value theory. Artifacts are coded to identify key traits and correlated with survey results. Students are also surveyed using the STEM-CIS to explore interest in engineering as a career path. In both cases, trends are examined over time as students encounter additional interventions throughout their high school curriculum.
Key Challenges: There have been two core challenges encountered on this project. First, cooperation of the district professional development coordinator is critical to ensuring access to teachers. We have learned that a formal documentation of this agreement and the expectations as to when teachers will be allowed to participate in project-related activities rather than district mandated activities is critical to success. Second, our initial model for the modules has changed significantly, in part due to observations during piloting and in part due to COVID-19. This has delayed publication of findings and materials.
Products: We hope to have a full suite of field-tested modules available next year.
CAREER: Noticing and Using Students’ Prior Knowledge in Problem-Based Instruction (NSF #1253081)
PI: Gloriana González Target Audience: High school geometry, high-need schools STEM Discipline: Mathematics
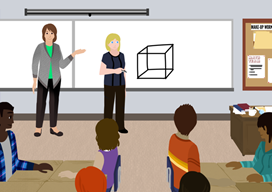
Products: We developed 4 geometry problems situated in realistic contexts for high school students to use their prior knowledge. Our publications show examples of ways in which they draw on their prior knowledge during problem-solving. We also developed a set of animations with examples of how teachers can use that prior knowledge during a problem-based lesson and guidance for engaging in a lesson study cycle using the problems as a source. The project website has the problems, the animations, and a list of publications; a total of 13 journal publications (and 1 in-press), 2 book chapters (1 in press), and more than 20 presentations.
COnceptual Model-based Math Intervention Tutor (COMMIT) (NSF #1503451)
PI: Yan Ping Xin | Co-PIs: Yingjie Victor Chen, Signe Kastberg Target Audience: Second, third, fourth grade students with learning difficulties in mathematics STEM Discipline: Mathematics
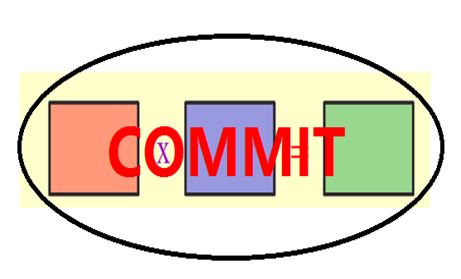
Current Findings Related to Problem Solving: We conducted a RCT study involving 18 students with LDM to investigate the effects of the web-based computer tutor, COMMIT, on enhancing mathematical word problem-solving performance of students with LDM. Findings from this study indicated that COMMIT, which emphasizes mathematical model-based problem-solving, boosted participants’ performance above and beyond the business-as-usual (BAU) group. That is, although both groups improved their performance from pre- to posttest, the improvement rate of the students in the COMMIT group (effect size [ES] COMPS = 1.88) is much larger than that of the students in the BAU group (ES BAU = 0.98). Using gain scores as the measure, the ES between the two groups was 1.32 favoring the COMPS group. Additionally, the COMMIT group also took the Mathematics Problem Solving subset of the Stanford Achievement Test (SAT-10; Harcourt Assessment, 2004) before and after the COMMIT intervention. Results on SAT scores indicate that five out of nine (56%) participants in the COMPS-A group improved their SAT percentile rank after the intervention.
Key Challenge: One challenge in conducting this type of work is the interdisciplinary nature of the work. The development of educational technologies requires the expertise of a diverse group of scholars who draw from different views of teaching and learning. Creating effective interfaces for learners and teachers demands that project team members develop a negotiated view of teaching and learning that can be drawn upon in the design of instructional activities, the programming of tools, and the implementation of the educational technology. Our engagement in conversations of views of teaching and learning overtime eventually result in a shared view.
Products: Project Website | 2020 STEM for All Video Showcase Entry
National/Internationally Refereed Journal Articles
- Xin, Y. P., Kim, S. J., Lei, Q., Wei, S., Liu, B., Wang, W., Kastberg, S., Chen, Y., Yang, X., Ma, X., Richardson, S. E. (2020). The impact of a conceptual model-based intervention program on math problem-solving performance of at-risk English learners. Reading and Writing Quarterly, 36 (2), 104-123. https://www.tandfonline.com/doi/full/10.1080/10573569.2019.1702909
- Xin, Y. P., Park, J., Tzur, R., & Si, L. (2020). The impact of a conceptual model-based mathematics computer tutor on multiplicative reasoning and problem-solving of students with learning disabilities. The Journal of Mathematical Behavior, 58. available online Feb 27, 2020. https://doi.org/10.1016/j.jmathb.2020.100762
- Xin, Y. P. (2019). The effect of a conceptual model-based approach on “additive” word problem solving of elementary students who are struggling in mathematics. ZDM : Mathematics Education, 51 (1), 139-150. DOI: 10.1007/s11858-018-1002-9
- Xin, Y. P.,Chiu, M. M., Tzur, R. Ma, X., Park, J., Yang, X. (2019). Discourse-oriented instruction: How does a teacher’s talk affect math problem solving and reasoning of students with Learning disabilities. Learning Disability Quarterly, 43 , 43-56 doi.org/10.1177/0731948719858707 ".
- Xin, Y. P., Tzur, Si, L. Hord, C., Liu, J., Park, J. Y. (2017). An intelligent tutor-assisted math problem-solving intervention program for students with learning difficulties. Learning Disability Quarterly, 40(1), 4-16 .
- Xin, Y. P., Liu, J., Jones, S., Tzur, R., SI, L. (2016). A preliminary discourse analysis of constructivist-oriented math instruction for a student with learning disabilities. The Journal of Educational Research, 109 (4), 436-447. DOI:10.1080/00220671.2014.979910
- Xin, Y. P. & Hord, C. (2013). Conceptual model-based teaching to facilitate geometry learning of students who struggle in mathematics. Journal of Scholastic Inquiry: Education, 1 (1), 147-160.
- Hord, C. & Xin, Y. P. (2013). Intervention research for helping elementary school students with math learning difficulties understand and solve word problems: 1996-2010. Learning Disabilities: A Multidisciplinary Journal, 19 (1), 3-17.
- Zhang, D, Xin, Y. P., & Si, L. (2013). Transition from intuitive to advanced strategies in multiplicative reasoning for students with math difficulties. The Journal of Special Education, 47 (1), 50-64.
- Xin, Y. P., Si, L., Hord, C., Zhang, D., Cetintas, S., & Park. J. Y. (2012). The effects of computer-assisted instruction in teaching Conceptual Model-Based problem solving. Learning Disabilities: A Multidisciplinary Journal, 18 (2), 71-85.
- Xin, Y. P., Zhang, D., Park, J. Y., Tom, K., Whipple, A., & Si, L. (2011). A comparison of two mathematics problem-solving strategies: Facilitate algebra-readiness. The Journal of Educational Research, 104 , 381-395.
- Cetintas, S, Si, L., Xin, Y. P., Zhang, D., Park, J. Y. & Tzur, R. (2010). A joint probabilistic classification model of relevant and irrelevant sentences in mathematical word problems. Journal of Educational Data Mining, 2 (1), 83-101.
- Cetintas, S., Si, L., Xin, Y. P., and Hord, C. (2010). Automatic detection of off-task behaviors in intelligent tutoring systems with machine learning techniques. IEEE Transactions on Learning Technologies, 3 (3), 228-236.
- Xin, Y. P. & Zhang, D. (2009). Exploring a conceptual model-based approach to teaching situated word problems. The Journal of Educational Research, 102 (6), 427-441.
- Xin, Y. P. (2008). The effect of schema-based instruction in solving word problems: An emphasis on pre-algebraic conceptualization of multiplicative relations. Journal for Research in Mathematics Education, 39 , 526-551.
- Xin, Y. P., Wiles, B., & Lin, Y. (2008). Teaching conceptual model-based word-problem story grammar to enhance mathematics problem solving. The Journal of Special Education, 42 , 163-178.
Peer-Reviewed Proceedings
- Kim, S., Kastberg, S. Xin, Y. P. Chen Y. & Wei, S. (2018). Development of the composite unit in additive problem solving of student with mathematics difficulty in a computer based-learning environment. In Hodges, T.E., Roy, G. J., & Tyminski, A. M. (Eds.). (2018). Proceedings of the 40th annual meeting of the North American Chapter of the International Group for the Psychology of Mathematics Education ( p.1280 ). Greenville, SC: University of South Carolina & Clemson University.
- Lei, Q., Xin, Y., P., Morita-Mullaney, T., & Tzur, R. (2018). Analyzing a discourse of scaffolds for mathematics instruction for an ELL with learning disabilities. In Hodges, T.E., Roy, G. J., & Tyminski, A. M. (Eds.). (2018). Proceedings of the 40th annual meeting of the North American Chapter of the International Group for the Psychology of Mathematics Education (pp. 460-467). Greenville, SC: University of South Carolina & Clemson University.
- Wei, S., Xin,Y.P., & Chen,Y. (2020). Visualizing Students' Eye Movement to Understand Their Problem-Solving Process". Accepted by the Proceedings of the 22nd International Conference on Human Computer Interaction, Copenhagen, Denmark.
- Wei, S., Lei, Q., Chen, Y., Xin, Y. P., Kastberg, S., & Kim, S. (2018). Evaluating the effects of highlighting text animations on the attention distribution of students with math learning difficulties. Proceedings of the 2018 American Society for Engineering Education (ASEE) Conference.
- Wei, S., Chen, Y., Xin, Y. P., Kastberg, S. (2018). An exploratory approach to analyzing students’ eye movements when solving math problems. In Hodges, T.E., Roy, G. J., & Tyminski, A. M. (Eds.). (2018). Proceedings of the 40th annual meeting of the North American Chapter of the International Group for the Psychology of Mathematics Education (pp. 1259-1262). Greenville, SC: University of South Carolina & Clemson University.
- Xin, Y. P., Kim; S., Kastberg, S., Chen, Y., Liu, B., Lei, Q., Wang, W., Richardson, S. E., Wei, S. (2018). The effect of a computer-assisted model-based problem-solving program for students with learning difficulties in mathematics. In Hodges, T.E., Roy, G. J., & Tyminski, A. M. (Eds.). (2018). Proceedings of the 40th annual meeting of the North American Chapter of the International Group for the Psychology of Mathematics Education ( pp.1267-1270 ). Greenville, SC: University of South Carolina & Clemson University.
- Xin, Y. P., Kastberg, Si., & V. Chen. (2017). Conceptual Model-based Problem Solving: A response to intervention program for students with LDM. In E. Galindo & J. Newton (Eds.) Proceedings of the 39th annual meeting of the North American Chapter of the International Group for the Psychology of Mathematics Education . Indianapolis, IN: Hoosier Association of Mathematics Teacher Educators, pp. 326.
- Xin, Y. P., Yang, X., Tzur, R. Park J., Ma. X (2016). PGBM-COMPS math problem-solving program: promote independent problem solving of students with LD. Proceedings of 13th International Congress on Mathematical Education (ICME), Hamburg Germany.
DEAP: Developing and Evaluating Assessments of Problem Solving (NSF #s 1720646 , 1720661 )
PIs: Jonathan Bostic , Toni Sondergeld | Co-PI: Gabriel Matney Target Audience: Researchers interested in assessment development and use in STEM contexts; grades 3-8 mathematics teachers and school administrators STEM Discipline: Mathematics
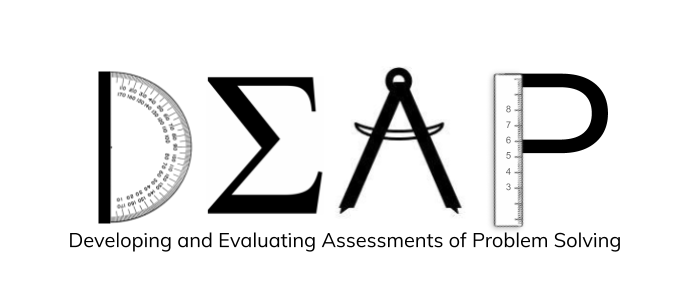
Theoretical Framework: All PSM items are problems for students to solve, rather than exercises. For this project, problems are characterized as tasks meeting the following criteria: (a) it is unknown whether a solution exists, (b) a solution pathway is not readily determined, and (c) there exists more than one way to answer the task (Schoenfeld, 2011). PSM tasks are word problems, thus we draw upon a framework from Verschaffel and colleagues (1999) such that the word problems are (a) open, (b) developmentally complex, and (c) realistic tasks for an individual. Open tasks can be solved using multiple developmentally-appropriate strategies. Complex tasks are not readily solvable by a respondent and require productive thinking. Realistic tasks may draw upon real-life experiences, experiential knowledge, and/or believable events. Problems are distinct from exercises, whereas exercises are tasks intended to promote efficiency with a known procedure (Kilpatrick et al., 2001). Further, problem solving goes beyond the type of thinking needed to solve exercises (Mayer & Wittrock, 2006) and occurs when the task is a problem, not an exercise (Schoenfeld, 2011).
Methodology: Once initial items are developed for PSMs, a systematic process is undertaken to collect five types of validity evidence, as suggested in The Standards for Educational and Psychological Testing (AERA et al., 2014): test content, response process, internal structure, relationship to other variables, and consequential. These sources of validity evidence inform continued or discontinued use of an item and whether additional items need to be developed. Test content validity evidence is collected through subject matter experts, including mathematics teachers, mathematics educators, and mathematicians, evaluating item-to-construct alignment through qualitative (logical or empirical) methods (Sireci & Faulkner-Bond, 2014). One-on-one and whole-class think alouds (Bostic et al., 2020) with typical student participants are used to inform response process validity evidence to ensure participant responses align with the test construct (Padilla & Benitez, 2014). For DEAP, internal structure validity evidence is assessed psychometrically using Rasch (1960) methods to explore instrument dimensionality, measurement invariance, and instrument reliability (Rios & Wells, 2014). Relationship to other variables validity evidence uses statistical testing to determine if PSM outcomes are associated with hypothesized variables (Beckman et al., 2005). Finally, consequential validity evidence (or bias) is examined through interviews to see how participants perceived the assessment to have impacted them (Bostic & Sondergeld, 2015) and through Rasch differential item functioning (DIF) analyses. Taken collectively, this evidence fuels the validity argument, which communicates how PSM users know score interpretations are appropriately grounded in sound claims and validity evidence.
Current Findings Related to Problem Solving: Initial findings show that our team has successfully developed three problem-solving measures (PSM 3-5) that assess students’ abilities to solve CCSSM grade-level aligned problems. Each assessment has been explored rigorously through multiple validation studies to produce meaningful measures and score interpretations for researchers and schools. To date, anchor items used for linking assessments to each other have been initially tested and overall demonstrate acceptable for continued use. In our final year, we will test the vertical equating linking to ensure our operationalized construct of problem-solving ability from grades 3 to 8 has been appropriately measured in the anticipated manner.
Key Challenge: Rigorous assessment development and validation is time intensive as it takes multiple cycles of creating and revising. Additionally, this work takes expertise from many different domains – subject matter experts, psychometricians, and school partners. It has taken our team many years of working together with diverse districts to construct a suitable test development process to conduct this type of work effectively and efficiently.
Products:
- Bostic, J., Matney, G., Sondergeld, T., & Stone, G. (2020, April). Validation as design-based research: Implications for practice and theory. Paper presented at annual meeting of the annual meeting of the American Education Research Association. San Francisco, CA.
- Bostic, J., Matney, G., Sondergeld, T., & Stone, G. (2020, April). Developing a series of problem-solving measures for elementary students. Paper presented at annual meeting of the annual meeting of the American Education Research Association. San Francisco, CA.
- Bostic, J., Matney, G., Sondergeld, T., & Stone, G. (2020, March). Measuring what we intend: A validation argument for the grade 5 problem-solving measure (PSM5). Validation: A Burgeoning Methodology for Mathematics Education Scholarship . In J. Cribbs & H. Marchionda (Eds.), Proceedings of the 47th Annual Meeting of the Research Council on Mathematics Learning (pp. 59-66). Las Vegas, NV.
- Bostic, J., Matney, G., Sondergeld, T., & Stone, G. (2019, July). Developing a problem-solving measure for grade 4. In M. Graven, H. Venkat, A. Essien, & P. Vale (Eds.), Proceedings of the 43 rd Meeting of the International Group for the Psychology of Mathematics Education (Vol. 4, p 4-104). Pretoria, South Africa. https://www.up.ac.za/media/shared/705/pme43/Proceedings/PME43Volume-4-proceedings-oc_pp.pdf
- Bostic, J.(2019, October). We can do better! Intersection Points, 44 (6), p. 3-4.
- Bostic, J., Matney, G., Sondergeld, T., & Stone, G. (2019, February). Validation: A Burgeoning Methodology for Mathematics Education Scholarship . In A. Sanogo & J. Cribbs (Eds.), Proceedings of the 46th Annual Meeting of the Research Council on Mathematics Learning (pp. 43-50). Charlotte, NC.
- Bostic, J.,Matney, G., Sondergeld, T., & Stone, G. (2018, November). Content validity evidence for new problem-solving measures (PSM3, PSM4, and PSM5). In T. Hodges, G. Roy, & A. Tyminski (Eds.), Proceedings for the 40 h Annual Meeting of the North American Chapter of the International Group for the Psychology of Mathematics Education (pp. 1641). Greenville, SC.
- Sondergeld, T. A. (2020). Shifting sights on STEM education instrumentation development: The importance of moving validity evidence to the forefront rather than a footnote. School Science and Mathematics Journal. https://onlinelibrary.wiley.com/doi/abs/10.1111/ssm.12410?af=R
- Sondergeld, T., Stone, G., Kruse, L., Bostic, J., & Matney, G. (2020, April). Evaluating Dichotomous and Partial-Credit Scoring within a Constructed-Response Assessment: Is More Information Always Psychometrically Better? Paper presented at annual meeting of the annual meeting of the American Education Research Association. San Francisco, CA.
- Sondergeld, T., Stone, G., Bostic, J., & Matney, G., (2019, July). Validity in a different context: Exploring relations to other variables evidence. In M. Graven, H. Venkat, A. Essien, & P. Vale (Eds.), Proceedings of the 43 rd Meeting of the International Group for the Psychology of Mathematics Education (Vol. 4, p. 4-104). Pretoria, South Africa. https://www.up.ac.za/media/shared/705/pme43/Proceedings/PME43Volume-4-proceedings-oc_pp.pdf
- Stone, G. E., Sondergeld, T. A., Bostic, J., & Matney, G. (2019, July). Validity in a different context: Exploring relationship to other variables validity evidence. In M. Graven, H. Venkat, A. Essien, & P. Vale (Eds.), Proceedings of the 43 rd Meeting of the International Group for the Psychology of Mathematics Education (Vol. 4, p. 4-104). Pretoria, South Africa. https://www.up.ac.za/media/shared/705/pme43/Proceedings/PME43Volume-4-proceedings-oc_pp.pdf
Ed+gineering: An Interdisciplinary Partnership Integrating Engineering into Elementary Teacher Preparation Programs (NSF #1908743)
PI: Jennifer Kidd | Co-PIs: Kristie Gutierrez, Krishnanand Kaipa, Pilar Pazos-Lago, Stacie Ringleb Target Audience: Our project partners undergraduate education and engineering students to teach engineering lessons to 4th and 5th graders. STEM Discipline: Engineering
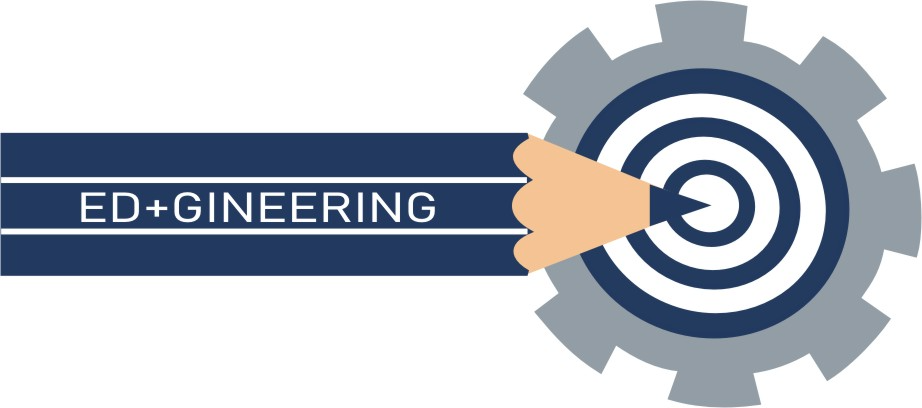
Ed+gineering’s goal is to increase PSTs’ engineering confidence and engineering students’ ability to communicate effectively in interdisciplinary contexts. Teaching engineering lessons in a supported context helps increase PSTs’ self-efficacy and intention to teach engineering. Engineering students who seek out and value the contributions of non-technical peers are well positioned to address complex global challenges.
Products: Coming Soon! Project Website
Project Videos
- 2020 STEM for All Video Showcase Entry
- Teaching robotics while quarantined: A profile of one team’s journey (Ed+gineeringODU Spring 2020)
- ODU National Active Learning Day
- Engineering Lessons Project 2016 @ ODU
- WOW Club 2018
ASEE 2020 Conference Presentations
- What do Undergraduate Engineering Students and Pre-service Teachers Learn by Collaborating and Teaching Engineering and Coding Through Robotics?
- The First Year of an Undergraduate Service Learning Partnership to Enhance Engineering Education and Elementary Pre-Service Teacher Education
- Enhancing Teamwork Skills Through an Engineering Service-learning Collaboration
- Partnering Undergraduate Engineering Students with Preservice Teachers to Design and Teach an Elementary Engineering Lesson Through Ed+gineering
FLECKS: Fostering Collaborative Computer Science Learning with Intelligent Virtual Companions for Upper Elementary Students (NSF #s 1721000 , 1721160 )
PIs: Kristy Boyer , Eric Wiebe | Co-PI: Collin Lynch Target Audience: Upper elementary (Grades 4-5) students, especially populations that historically have been underrepresented in Computer Science STEM Discipline: Computer Science
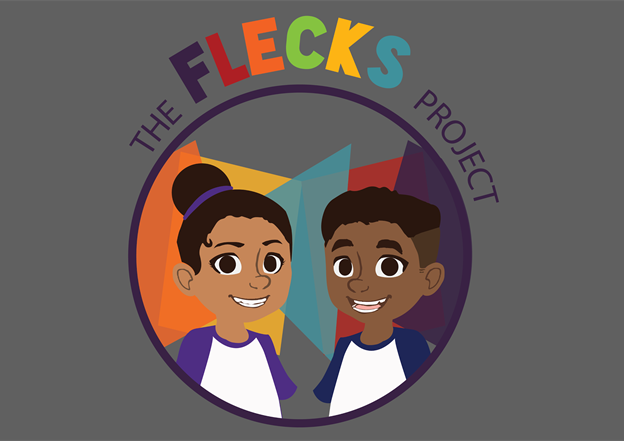
Theoretical Framework: The theoretical work of Mercer and colleagues (1999; 2002; 2004) has informed both our analysis of collaborative work and the goals for more productive collaboration. In addition, we are also interested in how dyads of students regulate their collaboration. This theoretical lens is informed by the work of noted scholars in the field (Hadwin, Wozney, & Pontin, 2005; Janssen, Erkens, Kirschner, & Kanselaar, 2010; and Kumpulainen & Mutanen, 1999).
Methodology: The primary approach to analyzing student discourse has been qualitative analysis of video and audio recordings of student work, guided by rubrics designed through the lens of the above-mentioned theoretical frameworks. In addition, we make extensive use of participatory design techniques in the development of our prototype learning environments. More recently, we have also been utilizing Epistemic Network Analysis (ENA; Shaffer, Collier & Ruis, 2016) to further unpack students’ discursive practices.
Current Findings Related to Problem Solving: Productive collaborative problem solving does not just happen, especially with younger students. Efficacious practices have to be modeled and supported by teachers and intelligent systems. We have learned that interpersonal dynamics will often lead students away from productive problem-solving and into unproductive conflict if not properly supported. Similarly, students need to be taught how to employ questioning techniques that move beyond low level questioning, where they are spurring deeper thinking on the part of their partner as they explain and defend their strategies and solutions.
Key Challenge: One of our challenges is the development of activities and design of a learning environment that serves and supports a diverse audience of students. In addition, elementary teachers have historically not taught computer science or collaborative problem solving in technology-rich environments. We have had to devote effort to properly supporting teachers and seeking feedback from a broad range of students through our design and development process.
- Tsan, J., Vandenberg, J., Fu, X., Wilkinson, J., Boulden, D., Wiebe, E., Lynch, C., & Boyer, K. E. (June, 2019). Conflicts and collaboration: A study of upper elementary students solving computer science problems. International Conference on Computer Supported Collaborative Learning (CSCL 2019) . Lyon, France.
- Tsan, J., Vandenberg, J., Fu, X., Wilkinson, J., Boulden, D., Boyer, K. E., ... & Wiebe, E. (February, 2019). An investigation of conflicts between upper-elementary pair programmers. Proceedings of the 50th ACM Technical Symposium on Computer Science Education (pp. 1264-1264). ACM.
- Tsan, J., Vandenberg, J., Zakaria, Z., Wiggins, J. B., Webber, A. R., Bradbury, A., Lynch, C., Wiebe, E., Boyer, K. E., (2020, March) A comparison of two pair programming configurations for upper elementary students. Proceedings of the 51st ACM Technical Symposium on Computer Science Education (SIGCSE ’20) , Portland, OR (pp. 346-352).
- Vandenberg, J., Tsan, J., Boulden, D. C., Zakaria, Z., Lynch, C., Boyer, K. E., & Wiebe, E. (2020). Elementary students' understanding of CS terms. ACM Transactions on Computing Education (TOCE), 20 (3). https://dl.acm.org/doi/pdf/10.1145/3386364
- Vandenberg, J., Tsan, J., Zakaria, Z., Boulden, D. C., Boyer, K. E., Lynch, C., & Wiebe, E. N. (2020, April). Elementary learners’ regulation in computer-supported collaborative learning environments . Paper accepted for presentation at the annual meeting of the American Educational Research Association (AERA), San Francisco, CA.
- Wiggins, J., Wilkinson, J., Lynch, C., Wiebe, E., & Boyer, K. E. (June, 2019). From doodles to designs: Participatory edagogical agent design with elementary students. Proceedings of the 18th ACM International Conference on Interaction Design and Children (IDC 2019) (pp. 642-647). ACM. doi: 10.1145/3311927.3325321
- Zakaria. Z., Boulden. D. C., Vandenberg, J., Tsan, J., Lynch, C. Boyer, K. E. & Wiebe, E. N. (April, 2019). Elementary students’ collaborative practices in side-by-side programming . AERA Annual Meeting, Toronto, CA.
- Zakaria, Z., Vandenberg, J., Boulden, D. C., Tsan, J., Boyer, K. E., Lynch, C., & Wiebe, E. N. (2020, April). Feedback to improve collaboration in pair programming . Paper accepted for presentation at the annual meeting of the American Educational Research Association (AERA), San Francisco, CA.
- Zakaria, Z., Wiebe, E., Boulden, D., Tsan, J., Vandenberg, J., Lynch, C. & Boyer, K. (June, 2019). Collaborative talk across two pair-programming configurations. International Conference on Computer Supported Collaborative Learning (CSCL 2019) . Lyon, France.
Improving Grades 6-8 Students' Mathematics Achievement in Modeling and Problem Solving through Effective Sequencing of Instructional Practices (NSF #1907840)
PI: Joe Champion | Co-PIs: Michele Carney , Samuel Coskey , Ya Mo, Keith Thiede Target Audience: Grades 6-8 students STEM Discipline: Mathematics
Theoretical Framework: ROOT aims to adopt and extend features of effective mathematics instruction as outlined by Hiebert and Grouws (2007). The Explicit Attention to Concepts (EAC) and Students’ Opportunities to Struggle (SOS) conceptual framework was successfully used by Stein and colleagues (2017) for large-scale classroom teacher research, and our project has been heavily invested in extending Stein’s work through a combination of video analysis, surveys, and student achievement data.
Key Challenge: ROOT addresses the key challenge of conducting classroom intervention research that both offers support and choice for teachers to innovate in their local context and generates transferable research evidence about how instructional strategies can improve student learning.
Product: Project Website | PD Modules One , Two , Three | EAC & SOS Guide
Mathematical and Computational Methods for Planning a Sustainable Future (PS-Future) (NSF #1503414)
PI: Margaret Cozzens | Co-PIs: Tamra Carpenter , Rebecca Jordan Target Audience: High school STEM Disciplines: Mathematics, Environmental Science, Biology, Computing, Social Sciences
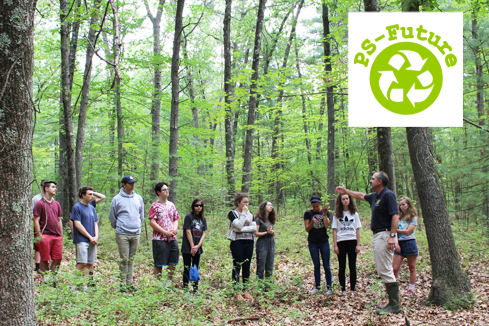
Project research and evaluation studies investigated and validated our belief that short (roughly one week) modules can positively impact students’: 1) engagement in mathematics and computing by immersion in sustainability topics of personal relevance; 2) confidence in using cross-cutting skills in mathematical and computational methods for scientific inquiry; and 3) learning of the STEM practices and modes of inquiry necessary to become the next generation of interdisciplinary problem solvers.
Theoretical Framework & Methodology: Given the increased demand for inter- and cross-disciplinary thinking, especially in environmental and sustainability related fields, special attention needs to be given to the means by which these complex environmental problems are understood. We feel that thinking within complex systems is essential for learners to grasp the non-linear and dynamic nature of real world problems and have focused our PS Futures learning modules on the quantitative reasoning and operations associated with sustainability related problems. Our work is nested in theory that suggests that learning is mediated by the tools and outcomes in an activity system surrounding the learners and that learners need to collaboratively attend to abstractions that can help encourage learning transfer (e.g., Palincsar 1998; Bransford et al. 1999). The tools and outcomes in our modules integrate social and scientific concepts embedded in mathematical operations that students encounter in their math and environmental science courses. We use mental models made visible through a specific conceptual representation that helps the learners to tie together the multiple ideas encountered in the modules. Because models often include a small number of semantic representations, individuals coming from different backgrounds, classrooms, and engaging with different task structures, once familiar with model terms, can communicate their ideas in a standardized space that can be compared across contexts.
Current Findings Related to Problem Solving: We were able to code data from 84 pre-module and 147 post-module models in which learners were asked to tie together ideas and transfer knowledge to a novel context. In this way, these learners can describe on a system level the mathematical strategies necessary to understand the environmental problem posed. From these models a number of trends emerged. First, and not surprisingly, the models were more accurate following instruction. Second, the post-module models tended to represent more causal agents, which suggests an increased understanding of how specific phenomena are driven by underlying mechanisms versus specific parts. In this way, we argue that as learners encounter novel spaces where similar thinking strategies are warranted, they are more likely to implement their more accurate mathematical practices. To support this contention, we found that when students represented greater mechanism they tended to do so at a more abstract scale, which tend to be more applicable across environments. While at this point only a speculation, there is enough evidence to suggest deeper investigation would be enlightening.
Key Challenges: As we were finishing publication of the modules, we discovered that the Cornell Ornithology Center was no longer supporting the “Yardmap” software they had developed, and by January 31, 2020, they had pulled it entirely from their website. One of the modules, called Life on the Edge, made extensive use of the software by students in designing suitable habitats. This section had to be rewritten and a new methodology found for developing and representing animal habitats. Fortunately, we were able to use Google Earth to accomplish all of tasks required. Google Earth is available to students through Google Classroom or independently. This work was completed in early June, completing the module development.
A typical challenge for this type of work is that of implementing a complex pre-post test project evaluation that involves models versus finite-scope types of questionnaires. That is to say, learners have the open space to model whatever aspect of the novel system, which can result in numerous possibilities that may reflect classroom-specific detail versus across-classroom ideas.
Doing this type of work, requires that the classroom instructors invest similar amounts of time and intellectual energy to the task which is not always feasible given the incredibly dynamic classroom space.
Products: Instructional Modules | Project Website | 2018 STEM for All Video Showcase Entry
Mathematical Learning via Architectural Design and Modeling Using E-Rebuild (NSF #1720533)
PI: Fengfeng Ke | Co-PIs: Russell Almond, Kathleen Clark, Gordon Erlebacher, Valerie Shute Target Audience: Middle school students STEM Disciplines: Mathematics
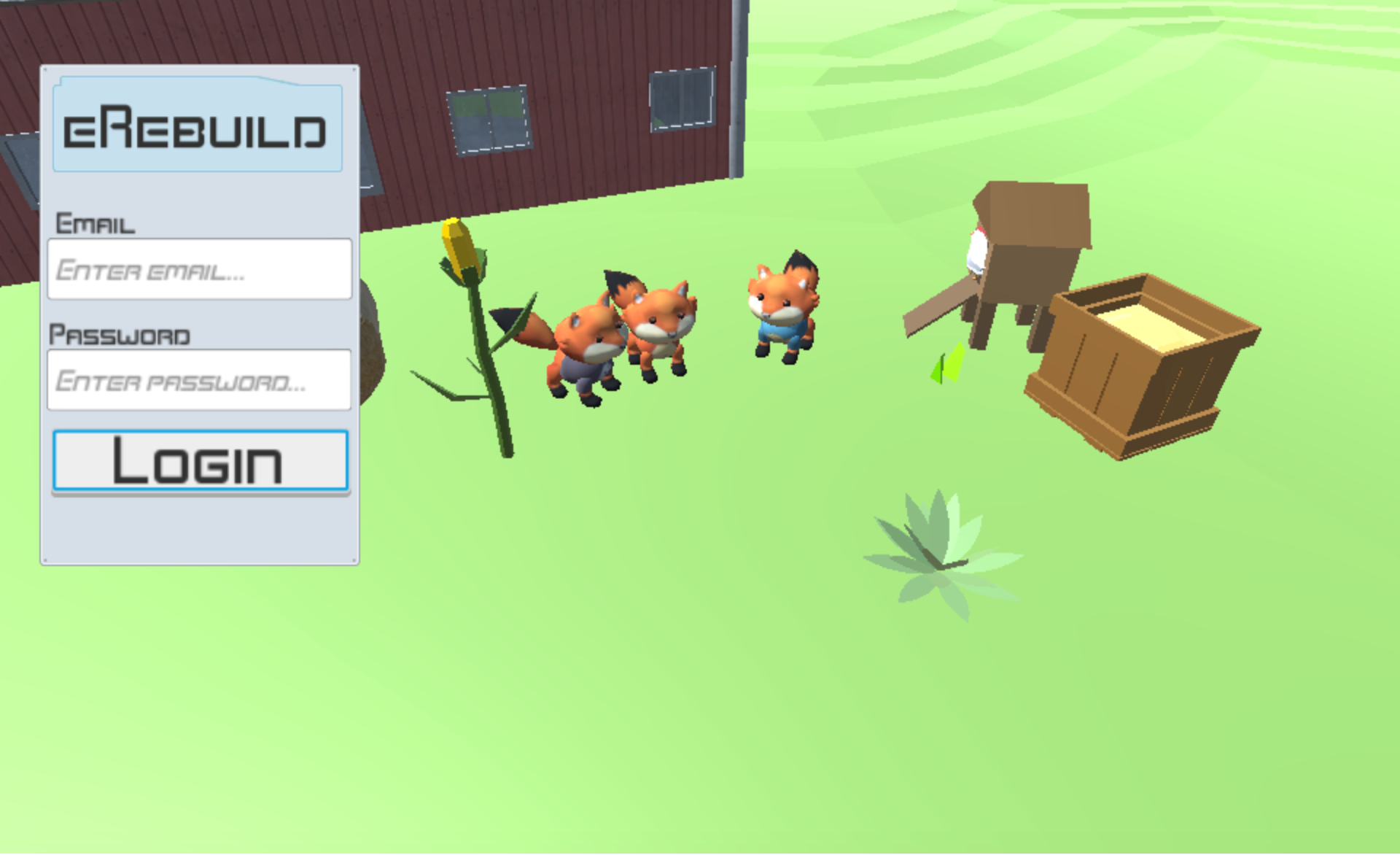
Theoretical Framework: Realistic mathematics education, learning through making and design, constructionism, and learning with multiple representations.
Methodology: The project uses a design-based research approach to gather data from students and teachers that will inform the design of the learning environment. The qualitative and quantitative data will also be used to understand what students are learning as they play the game and how teachers are interacting with their students.
Current Findings Related to Problem Solving: The initial findings of a recent mixed-method infield study indicate a positive effect and an advantage of using E-Rebuild in the classroom, in comparison with a business-as-usual control group, on the math problem-solving test performance of middle school students. There was a significant improvement with a large effect size from pretest to posttest by the E-Rebuild participants in the math problem-solving test performance. Controlling for the pretest performance, the E-Rebuild group significantly outperformed the control group in the post-treatment math problem-solving test with a medium-large effect size. The qualitative behavior analysis of participants’ gameplay processes also indicated a trend of increased engagement in mathematical problem-solving actions and the development of mindfulness with positive attitudes toward mathematical reasoning and conception.
Products: E-Rebuild Game, Tutorial, & Demos
Proportions Playground (NSF #1621290)
PI: Chandra Orrill Target Audience: Middle school teachers STEM Disciplines: Mathematics
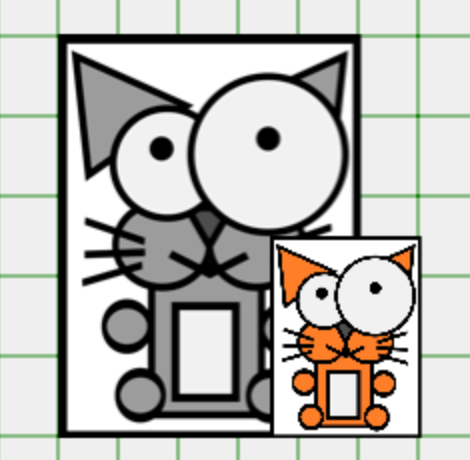
Theoretical Framework: Our work is grounded in two frameworks. The first is Knowledge in Pieces (from diSessa) which allows us a lens to think about how adults understand proportional relationships. While teachers may have a wide range of understandings, those understandings may not be interconnected in ways that support problem solving or the teachers may be missing key knowledge resources. The items we’ve developed attempt to push on making connections between independent knowledge resources, thus deepening teachers’ knowledge bases.
Our other framework is playing with math. This means engaging in problem solving in a way that relies on making and testing conjectures and math arguments that can be reasoned about, tested, illustrated, and explained through the use of dynamic environments. This necessarily occurs in a safe space built on discussion driven by challenges, explorations, and mysteries that are perceived to be worthwhile to engage in and to discuss. Worthwhile tasks are those that are relevant to classroom teaching, allow argument, often feature ambiguity (because that’s where argument can happen), and are open middle and/or open ended.
Methodology: Because this is an exploratory project, we have been using traditional qualitative methods relying primarily on videotaping professional development sessions.
Current Findings Related to Problem Solving: Two critical findings have emerged. First, the teachers love this approach. They rate the experiences very highly, report high engagement, and leave the PD smiling. Teachers want to be challenged, and this approach challenges them. Second, tasks need to be ambiguous to generate the playful exploration necessary to engage in this learning. This is very different from traditional views in which math problems are made as precise as possible. In our conversations, we explicitly discuss the need for precision by building from the perturbation that emerged from the ambiguity.
Products: Proportions Playground Toys | Proportions Playground Tasks
Science and Engineering Education for Infrastructure Transformation (NSF #1721054)
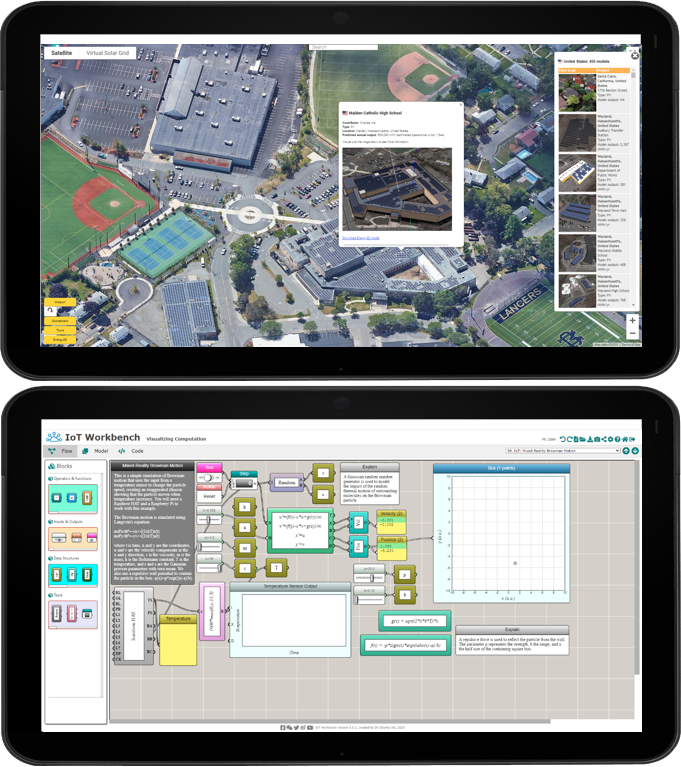
Description: Future sustainable and resilient infrastructure will be powered by renewable energy, be able to respond intelligently to changes in the environment, and support smart and connected communities. The transformation of our infrastructure calls for millions of problem solvers that our education system must cultivate. One way to fill the gap is to build cyberlearning infrastructures that enable students to learn how to solve those problems in the classroom with STEM. This project has developed two such programs, the Virtual Solar Grid and the IoT Workbench. These programs create rich problem-solving activities with sound pedagogies at the intersection of science, engineering, and computation and in the context of solar power engineering and cyber-physical systems. In the past year, we conducted research with 132 high school students and 52 middle school students with highly diverse socioeconomic backgrounds. The results show that students improve their science understanding while acquiring problem-solving abilities such as claim-evidence-reasoning, engineering design, and computational modeling. One of the teachers spoke highly of the solar energy project. “This is the first time of the year that all 16 pairs of eyes paid attention with invested attitudes,” referring to one of her classes that was relatively underperforming.
Products: Virtual Solar Grid | IoT Workbench
Systemic Transformation of Inquiry Learning Environments for STEM (NSF #2010530)
PI: Ellen Meier | Co-PI: Bryan Keller Target Audience: Grades 3-8, Urban Schools STEM Discipline: Transdisciplinary STEM; STEM as a meta-discipline; cross-cutting concepts in STEM, which can involve other disciplines beyond science, technology, engineering, and mathematics.
With the NSF funding, the project team will study the utility and feasibility of the model in an effort to streamline the approach and to create broader access to high-quality professional learning. Over the course of the project, Grades 3-8 teachers from twenty-five schools in New York City and New Haven, CT will participate in a multi-year online workshop and coaching series to design and implement rigorous and culturally relevant learning experiences focused on cross-cutting concepts across the STEM disciplines. Complementary work with school-based administrators and instructional coaches will support leaders in strategically supporting the change process.
Products: The systemic work leverages the Innovating Instruction approach to professional learning developed by the Center for Technology and School Change at Teachers College, Columbia University. A publically available version is available on the Center’s website .
Zoombinis: The Implementation Research Study of a Computational Thinking Game for Upper Elementary and Middle School Learners (NSF #1502882)
PI: Jodi Asbell-Clarke | Co-PIs: Teon Edwards , Elizabeth Rowe Target Audience: National, Grades 3-8; Sub-study on students with IEPs or 504s for neurodiversity Discipline/Focus: Computational Thinking
Zoombinis engages learners in problem-solving practices such as Problem Decomposition, Pattern Recognition, Abstraction, and Algorithm Design. We provide “bridge activities” that helps teachers connect the implicit CT problem-solving practices in the game to explicit classroom problem-solving in other subjects such as science and math.
We studied how teachers use the learning game, Zoombinis , to solve problems using Computational Thinking (CT) practices. While students in grades 3-8 solve fun logic puzzles in the game, teachers connect those practices to problem-solving in STEM and everyday activities.
Theoretical Framework: We draw from theory that considers CT as a specific form of problem-solving to study problem solving in the game Zoombinis . Our research goal was to examine how students build CT practices such as problem decomposition, pattern recognition, abstraction, and algorithm design within the context of the increasingly complex logic puzzles in the game.
We also use theory from game-based learning where students build implicit skills and knowledge through engaged problem-solving. This framework differentiates between explicit knowledge, what wecan express, from implicit knowing, which is what we are able to do . Implicit knowledge is, by definition, largely unexpressed by the learner yet is considered foundational to all knowledge. Explicit knowledge is what educators typically attempt to measure in learning assessments. Games present a rich opportunity to support and measure implicit learning because players are often immersed in problem-solving situations where they experiment with the mechanics to understand the rule system, using trial and error with helpful feedback and rewards for motivation and sustain engagement.
We built educational data mining models as a form of embedded implicit assessments, assessments that could measure CT practices using the game log data generated by students’ gameplay. One component of our research study was to examine the validity of these detectors as assessments of students’ CT. Another component of the research was to examine the relationship between students’ activity in the game, teachers’ activity in the class, and the outcomes of students’ CT practices. Our previous research has shown that teachers are key to bridging implicit game-based learning to explicit classroom learning, so we provided teachers with bridging activities and support while they implemented the game in their classes.
Methodolgy: We used a combination of research methods, including educational data mining on game data logs, cluster analysis on teacher logs of classroom activity, and multilevel modeling, to determine the impact of the duration and nature of student gameplay, as well as the extent and nature of classroom activity, on student CT practices.
To develop and validate implicit learning assessments using Zoombinis log data, we designed automated detectors of CT practices within Zoombinis game play. We collected video and screencapture along with data logs from a wide variety of Zoombinis players. After extensive observations and human-labelling of the data, we build educational data mining (EDM) modes to detect those strategies automatically in student gameplay. These automated detectors provide real-time assessment of CT practices students build implicitly within the game.
To validate the detectors as measures of CT, we correlated the detectors with external measures of students’ CT practices. Most CT practice detectors were significantly correlated with these external measures.
To serve as external pre- and post-assessments of CT, the authors worked with a game-based learning company to design Interactive Assessments of CT (IACT)—a set of online, non-coding, non-textual logic puzzles to assess CT practices in upper elementary and middle school.
Current Findings Related to Problem Solving: Automated detectors of gameplay CT practices built for this research were significant predictors of external post-assessment scores, and thus show promise as implicit assessments of CT practices within gameplay .
Students with high duration of gameplay and high gameplay CT practices scored highest on external post-assessment of CT practices, when accounting for pre-assessment scores. This research suggests that Zoombinis is an effective CT learning tool and CT assessment tool for elementary- and middle-school students.
In a small sub-study of classes where we were able to collect students’ IEP status, we found that when teachers used a combination of Zoombinis and classroom bridging activities the outcomes of students with IEP status are comparable to their peers.
Key Challenge: Our biggest challenge was the lack of available external assessments in CT that met the diverse needs of our target audience. We designed our own assessments because we could not find established instruments that did not rely on significant text or coding, which may have been a barrier for some students. In a larger study using an augmented sample, we found moderate evidence of concurrent validity and strong evidence of test-retest reliability for IACT, so we used these for outcome measures in our studies. The research findings would be stronger with a more psychometrically-validated external assessment instrument that also accessible to a broad audience.
Products: Zoombinis Game | Zoombinis Apps ( Apple Store, Google Play )
- Asbell-Clarke, J., Rowe, E., Almeda, V., Edwards, T., Bardar, E., Gasca, S., Baker, R.S., & Scruggs, R. (under review). The Development of Students’ Computational Thinking Practices in Elementary- and Middle-School Classes using the Learning Game, Zoombinis.
- Asbell-Clarke, J. ,Rowe, E. , Almeda, V., Gasca, S. , Edwards, T. , Bardar, E. ,Shute, V. , and Ventura, M. (under review). Interactive Assessments of CT (IACT): Digital Interactive Logic Puzzles to Assess Computational Thinking in Grades 3–8.
- Asbell-Clarke, J., Rowe, E. , Almeda, V., Edwards, T. , Bardar, E. (under review). Bridging the Gap: Using the Computational Thinking Game, Zoombinis , to Support Neurodiverse Learners.
- Rowe, E., Asbell-Clarke, J., & Almeda, M. Scruggs, R., Baker, R.S., Bardar,E. & Gasca, S. (under review) Assessing Implicit Computational Thinking in Zoombinis Puzzle Gameplay. Submitted to a special issue of Computers & Human Behavior on Learning Analytics and Assessment.
- Almeda, M., Rowe, E., Asbell-Clarke, J., Baker, R., Scruggs, R., Bardar, E., & Gasca, S. (2019, October). Modeling Implicit Computational Thinking in Zoombinis Mudball Wall Gameplay. Paper submitted to the Technology, Mind, and Society conference, October, Washington D.C.
- Rowe, E., Asbell-Clarke, J., Almeda, M., Bardar, E., Baker, R. S., & Scruggs, R., (2019). Advancing Research in Game-Based Learning Assessment: Tools and Methods for Measuring Implicit Learning. In E. Kennedy & J. Qian (Eds.) Advancing Educational Research with Emerging Technology. IGI Global.
- Rowe, Rowe, E., Asbell-Clarke, J., & Baker, R. (2019, April). Game-based measures of implicit learning. Structured poster session organized by Y.J. Kim titled Game-Based Assessment: How Has the Field Matured over the Past 10 years? AERA Annual Meeting, Toronto.
- Rowe, E., Asbell-Clarke, J., Baker, R., Gasca, S., Bardar, E., & Scruggs, R. (2018, April). Labeling Implicit Computational Thinking in Pizza Pass Gameplay. Late-breaking work presented at the ACM SIGCHI Conference on Human Factors in Computing Systems (CHI 18), Montreal. https://doi.org/10.1145/3170427.3188541
- Rowe, E., Asbell-Clarke, J., Baker, R., Gasca, S., Bardar, E., & Scruggs, R. (2017, April). Labeling Implicit Computational Thinking in Pizza Pass Gameplay. Late-breaking work presented at the ACM SIGCHI Conference on Human Factors in Computing Systems (CHI 18), Montreal. https://doi.org/10.1145/3170427.3188541
- Shute, V. J., Sun, C., & Asbell-Clarke, J. (2017). Demystifying computational thinking . Educational Research Review, 22, 142-158.
- Rowe, E., Asbell-Clarke, J., Cunningham, K. & Gasca, S. (2017, October). Assessing implicit computational thinking in Zoombinis gameplay: Pizza Pass, Fleens, and Bubblewonder Abyss. Work-in-progress presented at the ACM SIGCHI Annual Symposium on Computer-Human Interaction in Play, Amsterdam.
- Rowe, E., Asbell-Clarke, J., Gasca, S., & Baker, R. (2017, October). Computational thinking in Zoombinis gameplay. Spotlight session at the 8th Digital Media & Learning Conference in Irvine, CA.
- Rowe, E., Asbell-Clarke, J., Gasca, S., & Cunningham, K. (2017, August). Assessing implicit computational thinking in Zoombinis gameplay. Poster presented at the International Conference on the Foundations of Digital Games in Hyannis, MA.
- Rowe, E., Asbell-Clarke, J., Gasca, S., & Baker, R. (2017, April). Computational Thinking in Zoombinis Gameplay. Poster presented at the Cyberlearning Conference in Arlington, VA.
- Algebra Education
- Argumentation, Critique, & Other Discursive STEM Practices
- Computer Science & Computational Thinking
- Broadening Youth Participation in Computer Science & Engineering
- Collaborative Learning
- Computational Thinking
Brown, A. L. (1992). Design experiments: Theoretical and methodological challenges in creating complex interventions in classroom settings. The Journal of the Learning Sciences , 2 (2), 141-178.
Cobb, P., Confrey, J., DiSessa, A., Lehrer, R., & Schauble, L. (2003). Design experiments in educational research. Educational Researcher , 32 (1), 9-13.
Collins, A. (1992). Towards a design science of education. In E. Scanlon & T. O'Shea (Eds.), New directions in educational technology (pp. 15-22). Berlin: Springer
Dhombres, J. (1993). Is one proof enough? Travels with a mathematician of the baroque period. Educational Studies in Mathematics , 24 (4), 401-419.
Hadwin, A. F., Wozney, L., & Pontin, O. (2005). Scaffolding the appropriation of self-regulatory activity: A socio-cultural analysis of changes in teacher–student discourse about a graduate research portfolio. Instructional Science, 33 (5–6), 413–450.
Gentner, D., Loewenstein, J., & Thompson, L. (2003). Learning and transfer: A general role for analogical encoding. Journal of Educational Psychology , 95 (2), 393.
Goldstone, R. L., Day, S., & Son, J. Y. (2010). Comparison. In Towards a theory of thinking (pp. 103-121). Springer, Berlin, Heidelberg.
Guo, J. P., & Pang, M. F. (2011). Learning a mathematical concept from comparing examples: The importance of variation and prior knowledge. European Journal of Psychology of Education , 26 (4), 495-525.
Hiebert, J., & Grouws, D. A. (2007). The effects of classroom mathematics teaching on students’ learning. In F. K. Lester (Ed.), Second handbook of research on mathematics teaching and learning (pp. 371-404). Greenwich, CT: Information Age
House, P. A., & Coxford, A. F. (1995). Connecting Mathematics across the Curriculum.1995 Yearbook . National Council of Teachers of Mathematics. Reston, VA.
Janssen, J., Erkens, G., Kirschner, P. A., & Kanselaar, G. (2012). Task-related and social regulation during online collaborative learning. Metacognition and Learning, 7 (1), 25–43.
Loewenstein, J., & Gentner, D. (2001). Spatial mapping in preschoolers: Close comparisons facilitate far mappings. Journal of Cognition and Development , 2 (2), 189-219.
Kumpulainen, K., & Mutanen, M. (1999). The situated dynamics of peer group interaction: An introduction to an analytic framework. Learning and Instruction, 9 (5), 449-473.
Kurtz, K. J., Miao, C. H., & Gentner, D. (2001). Learning by analogical bootstrapping. The Journal of the Learning Sciences , 10 (4), 417-446.
Mercer, N. (2002). Words and minds: How we use language to think together. Routledge.
Mercer, N., Dawes, L., Wegerif, R., & Sams, C. (2004). Reasoning as a scientist: Ways of helping children to use language to learn science. British Educational Research Journal, 30 (3), 359-377.
Mercer, N., Wegerif, R., & Dawes, L. (1999). Children's talk and the development of reasoning in the classroom. British Educational Research Journal, 25 (1), 95-111.
Namy, L. L., & Gentner, D. (2002). Making a silk purse out of two sow's ears: Young children's use of comparison in category learning. Journal of Experimental Psychology: General , 131 (1), 5.
National Council of Teachers of Mathematics. (2000). Principles and standards for school mathematics . Reston, VA: NCTM.
National Governors Association. (2010). Common core state standards . Washington, DC.
Oakes, L. M., & Ribar, R. J. (2005). A comparison of infants' categorization in paired and successive presentation familiarization tasks. Infancy , 7 (1), 85-98.
Pólya, G. (1963). Induction and analogy in mathematics . Princeton University Press.
Polya, G. (1973). How to solve it 2nd . New Jersey: Princeton University.
Polya, G. (1963). Induction and analogy in mathematics . Princeton University Press.
Rittle-Johnson, B., & Star, J. R. (2007). Does comparing solution methods facilitate conceptual and procedural knowledge? An experimental study on learning to solve equations. Journal of Educational Psychology , 99 (3), 561.
Rittle-Johnson, B., & Star, J. R. (2009). Compared with what? The effects of different comparisons on conceptual knowledge and procedural flexibility for equation solving. Journal of Educational Psychology , 101 (3), 529.
Rittle-Johnson, B., Star, J. R., & Durkin, K. (2009). The importance of prior knowledge when comparing examples: Influences on conceptual and procedural knowledge of equation solving. Journal of Educational Psychology , 101 (4), 836.
Schoenfeld, A. H. (1983). Beyond the purely cognitive: Belief systems, social cognitions, and metacognitions as driving forces in intellectual performance. Cognitive science , 7 (4), 329-363.
Schoenfeld, A. H. (1988). When good teaching leads to bad results: The disasters of'well-taught'mathematics courses. Educational Psychologist , 23 (2), 145-166.
Schoenfeld, A. H. (2006). Design experiments. Handbook of Complementary Methods in Education Research , 193-206.
Shaffer, D. W., Collier, W., & Ruis, A. R. (2016). A tutorial on epistemic network analysis: Analyzing the structure of connections in cognitive, social, and interaction data. Journal of Learning Analytics, 3 (3), 9–45.
Shavelson, R. J., & Towne, L. (Eds.). (2002). Scientific research in education. Committee on Scientific Principles for Education Research. Washington, DC: National Research Council.
Star, J. R., & Rittle-Johnson, B. (2008). Flexibility in problem solving: The case of equation solving. Learning and Instruction , 18 (6), 565-579.
Star, J. R., & Rittle-Johnson, B. (2009). It pays to compare: An experimental study on computational estimation. Journal of Experimental Child Psychology , 102 (4), 408-426.
Vinner, S. (1989). The Avoidance of Visual Considerations in Calculus Students. Focus on Learning Problems in Mathematics , 11 , 149-56.
Xin, Y. P., Wiles, B., & Lin, Y. Y. (2008). Teaching Conceptual Model—Based Word Problem Story Grammar to Enhance Mathematics Problem Solving. The Journal of Special Education , 42 (3), 163-178.
- International
- Education Jobs
- Schools directory
- Resources Education Jobs Schools directory News Search
Maths Sentence Stems for display
Subject: Mathematics
Age range: 7-11
Resource type: Visual aid/Display

Last updated
30 July 2020
- Share through email
- Share through twitter
- Share through linkedin
- Share through facebook
- Share through pinterest
A set of sentence stems to support children in their justification of reasoning and understanding of mathematical problems.
Creative Commons "Sharealike"
Your rating is required to reflect your happiness.
It's good to leave some feedback.
Something went wrong, please try again later.
This resource hasn't been reviewed yet
To ensure quality for our reviews, only customers who have downloaded this resource can review it
Report this resource to let us know if it violates our terms and conditions. Our customer service team will review your report and will be in touch.
Not quite what you were looking for? Search by keyword to find the right resource:
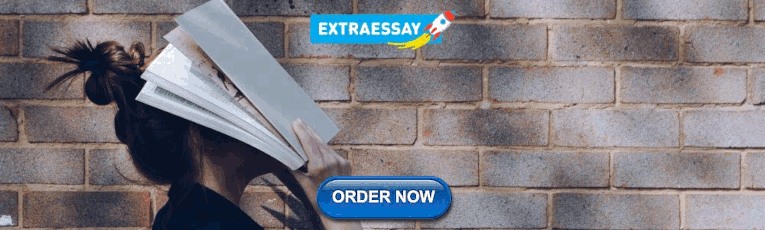
IMAGES
VIDEO
COMMENTS
Stem sentences can be used to: Express a key concept. Generalise a key concept. Provide a template for discussions or explanations. Each time learners repeat a stem sentence correctly, it helps embed the concept. Sentence stems are a fantastic resource when using an ' I do, we do, you do ' teaching pedagogy.
In addition to sentence starters and frames that support students in sharing and thinking deeply about their own work, different types of sentence starters and frames can support peer discussion and peer critique of mathematics problem-solving. For example, sentence starters such as, "Can you explain how you…" and, "I wonder why you ...
A sentence stem, also known as a sentence frame, is part of a structured sentence with missing parts to encourage kids to communicate their ideas. They are used in a range of topics to provide clarity or to generalise concepts. These can be used across core subjects such as math and English, in addition to other subjects such as languages ...
An effective stem sentence allows children to practise reasoning by changing variables and using precise and technical vocabulary. Stem sentences can also be used to arrive at a generalisation or general rule. For example, when children in UKS2 build on their understanding of 'factor x factor = product', they explore how many factors a ...
5. Track progress: Monitor the students' use of sentence stems over time and provide feedback and reinforcement on areas where they may need further assistance. 60+ Sentence Stem Examples for Every Subject. Mathematics: 1. "I noticed a pattern when I saw…". 2. "The formula changes if we…". 3.
Encourage students to use sentence stems to explain their problem-solving processes, the strategies they chose, and why. This can help demystify complex problems and foster a growth mindset toward tackling challenging questions. Examples: "I approached this problem by ___ because…" "A mistake I encountered was ___ and I fixed it by…"
These can be used to state a fact, explain a thought process, or give an answer to a problem. They generally come in three different types: Stating a key concept. Stating a generalised concept. Explaining thoughts and ideas. Stem sentences are designed to improve the comprehension of maths problems and concepts.
Balancing disciplinary knowledge and practical reasoning in problem solving is needed for meaningful learning. In STEM problem solving, science subject matter with associated practices often appears distant to learners due to its abstract nature. Consequently, learners experience difficulties making meaningful connections between science and their daily experiences. Applying Dewey's idea of ...
ents four tens. The '2' is in the tens column- it represents two tens.DecimalsThe whole is divided into ten/ a hundred equal. parts and ___ of them is/ are shaded; this is ___ tenth(s)/ hundred(s) of the whole.If a digit is moved one/ two column(s) to the left, the numbe.
Maths Sentence Stems KS2 PDF A simple, A4 wordmat providing a range of sentence stems to support students effectively explain their mathematical reasoning. Sentence stems are grouped based on the structure / scenario most appropriate. This resource is appropriate for all of KS2 based on National Curriculum objectives. White- problem solving.
20 MASTERY STEM SENTENCE STARTERS. Subject: Mathematics. Age range: 7-11. Resource type: Visual aid/Display. File previews. pptx, 99.97 KB. Maths STEM sentences. help support all abilities in KS1 and KS2 to explain their reasoning and problem solving strategies. find more at uniqueclassrooms.com.
For example, quantitative reasoning, which is critical to integrated STEM problem solving, is frequently "misrepresented, underdeveloped, and ignored in STEM classrooms" (Mayes, 2019, p. 113). Likewise, Tytler ( 2016 ) warned that there needs to be an explicit focus on the mathematical concepts and thinking processes that arise in STEM ...
Creating and solving problems explains the 'doing' and 'undoing' processes in mathematics giving three examples of a problem poser where a closed problem is posed and the problem solver where the problem is posed in a more open format. Evaluating mathematical statements gives a number of examples for which it has to be decided whether ...
Yes, we can say that, "When I have 2 teachers, I also have 70 students.". Practice this pattern again and again with your students. Every time you are looking at a word problem, use this sentence stem with your students. As you repeat the stem, write the ratio. Your students will start recognizing the ratio in word problems with this ...
Be sure to model them for kids, showing how to fill in the blanks. Practice both out loud and in writing, providing your own example and then asking for their take. It's OK if they simply fill in the blanks when they first start using these stems. But over time, students should use them more as a jumping-off point, to delve deeper into the topic.
Explaining mathematical reasoning and problem solving by using a variety of methods, such as words, numbers, symbols, charts, graphs, tables, diagrams, and concrete models can help students understand the problem better by making it more concrete and approachable. Let's try another one.
In that paper he produces this pyramid: This is important for two reasons: 1) It splits up reasoning skills and problem solving into two different entities. 2) It demonstrates that fluency is not something to be rushed through to get to the 'problem solving' stage but is rather the foundation of problem solving.
Problem solving provides a powerful context for teaching and learning STEM+C concepts and practices and draws on skills, approaches, and ways of thinking that can be applied across disciplines. This Spotlight showcases thirteen projects that are researching and developing innovations that support and leverage problem solving in computer science, engineering, mathematics, and science.
This document contains a list of appropriate sentence stems and vocabulary for place value for Years 1 to 6. Sentence stems are used as a way for pupils to communicate and clarify mathematical conceptual ideas or generalisations. Using a common language both across a topic and the primary phase avoids confusion and encourages the use of ...
Maths Sentence Stems KS2 PDF A simple, A4 wordmat providing a range of sentence stems to support students effectively explain their mathematical reasoning. Sentence stems are grouped based on the structure / scenario most appropriate. This resource is appropriate for all of KS2 based on National Curriculum objectives. Sentence Stem Types.
20 STEM sentences to support problem solving and reasoning 20 STEM sentences to support problem solving and reasoning SKU: £1.75. £1.75. Unavailable per item 20 Maths STEM sentences . help support all abilities in KS1 and KS2 to explain their reasoning and problem solving strategies. Facebook; Pinterest; Google+; Quantity. Add to Cart ...
pdf, 1.05 MB. A free maths reasoning progression document for primary mathematics including progression of key skills, sentence starters and questioning support. Suitable for use alongside schemes of learning such as White Rose Maths to support children in their learning progression in reasoning. A display resource of maths stem sentence speech ...
Introducing AI concepts early enhances STEM aptitude, developing critical skills like coding and problem-solving. Pankaj Kumar emphasizes AI's benefits in personalized learning experiences ...
Maths Sentence Stems KS1 PDF A simple, A4 wordmat providing a range of sentence stems to support students effectively explain their mathematical reasoning. Sentence stems are grouped based on the structure / scenario most appropriate. This resource is appropriate for all of KS1 based on National Curriculum objectives. White- problem solving.
Reasoning Sentence Starters. Subject: Mathematics. Age range: 7-11. Resource type: Visual aid/Display. File previews. pdf, 208.58 KB. FREEBIE! 20 speech bubbles with sentence starters to help prompt reasoning discussion and recording during maths lessons. 4 different designs. Perfect for your working wall display!
Maths Sentence Stems for display. Subject: Mathematics. Age range: 7-11. Resource type: Visual aid/Display. File previews. pdf, 89.78 KB. A set of sentence stems to support children in their justification of reasoning and understanding of mathematical problems. Creative Commons "Sharealike".