Information
- Author Services
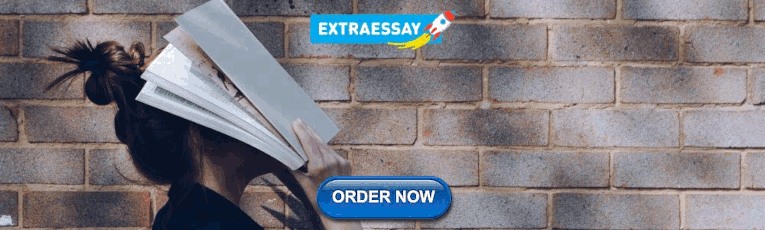
Initiatives
You are accessing a machine-readable page. In order to be human-readable, please install an RSS reader.
All articles published by MDPI are made immediately available worldwide under an open access license. No special permission is required to reuse all or part of the article published by MDPI, including figures and tables. For articles published under an open access Creative Common CC BY license, any part of the article may be reused without permission provided that the original article is clearly cited. For more information, please refer to https://www.mdpi.com/openaccess .
Feature papers represent the most advanced research with significant potential for high impact in the field. A Feature Paper should be a substantial original Article that involves several techniques or approaches, provides an outlook for future research directions and describes possible research applications.
Feature papers are submitted upon individual invitation or recommendation by the scientific editors and must receive positive feedback from the reviewers.
Editor’s Choice articles are based on recommendations by the scientific editors of MDPI journals from around the world. Editors select a small number of articles recently published in the journal that they believe will be particularly interesting to readers, or important in the respective research area. The aim is to provide a snapshot of some of the most exciting work published in the various research areas of the journal.
Original Submission Date Received: .
- Active Journals
- Find a Journal
- Proceedings Series
- For Authors
- For Reviewers
- For Editors
- For Librarians
- For Publishers
- For Societies
- For Conference Organizers
- Open Access Policy
- Institutional Open Access Program
- Special Issues Guidelines
- Editorial Process
- Research and Publication Ethics
- Article Processing Charges
- Testimonials
- Preprints.org
- SciProfiles
- Encyclopedia
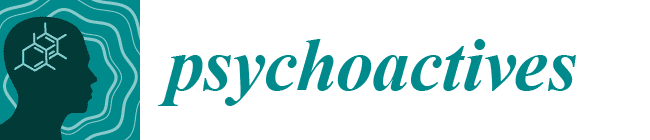
Article Menu
- Subscribe SciFeed
- Recommended Articles
- Author Biographies
- Google Scholar
- on Google Scholar
- Table of Contents
Find support for a specific problem in the support section of our website.
Please let us know what you think of our products and services.
Visit our dedicated information section to learn more about MDPI.
JSmol Viewer
Neurobiological theories of addiction: a comprehensive review.
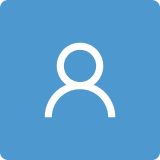
1. Introduction
2. opponent-process theory—solomon and corbit (1974), 3. dopaminergic hypothesis of addiction—wise (1980), 4. incentive sensitization theory—robinson and berridge (1993), 5. habit and compulsion theory—robbins and everitt (1999); everitt and robbins (2005), 6. allostasis theory of addiction—koob and le moal (1997), 7. new approaches to the study of the addictive process: neuroinflammation, 8. conclusions, author contributions, conflicts of interest.
- Drugs, Brains, and Behavior: The Science of Addiction. NIDA, National Institute of Health. Available online: https://nida.nih.gov/research-topics/addiction-science/drugs-brain-behavior-science-of-addiction (accessed on 10 January 2024).
- Birkeland, B.; Foster, K.; Selbekk, A.S.; Høie, M.M.; Ruud, T.; Weimand, B. The quality of life when a partner has substance use problems: A scoping review. Health Qual. Life Outcomes 2018 , 16 , 219. [ Google Scholar ] [ CrossRef ] [ PubMed ]
- Daley, D.C. Family and social aspects of substance use disorders and treatment. J. Food Drug Anal. 2013 , 21 , S73–S76. [ Google Scholar ] [ CrossRef ] [ PubMed ]
- Ferrer-Pérez, C.; Miñarro, J. From crayfish to humans: An evolutionary perspective of addiction. Metode Sci. Stud. J. 2022 , 12 , 57–61. [ Google Scholar ]
- Volkow, N.D.; Michaelides, M.; Baler, R. The neuroscience of drug reward and addiction. Physiol. Rev. 2019 , 99 , 2115–2140. [ Google Scholar ] [ CrossRef ]
- Feltenstein, M.W.; See, R.E.; Fuchs, R.A. Neural substrates and circuits of drug addiction. Cold Spring Harb. Perspect. Med. 2021 , 11 , a039628. [ Google Scholar ] [ CrossRef ]
- Cheron, J.; Kerchove d’Exaerde, A.D. Drug addiction: From bench to bedside. Transl. Psychiatry 2021 , 11 , 424. [ Google Scholar ] [ CrossRef ] [ PubMed ]
- Montagud-Romero, S.; Blanco-Gandía, M.C.; Reguilón, M.D.; Ferrer-Pérez, C.; Ballestín, R.; Miñarro, J.; Rodríguez-Arias, M. Social defeat stress: Mechanisms underlying the increase in rewarding effects of drugs of abuse. Eur. J. Neurosci. 2018 , 48 , 2948–2970. [ Google Scholar ] [ CrossRef ]
- Uhl, G.R.; Koob, G.F.; Cable, J. The neurobiology of addiction. Ann. N. Y. Acad. Sci. 2019 , 1451 , 5–28. [ Google Scholar ] [ CrossRef ]
- Koob, G.F.; Volkow, N.D. Neurobiology of addiction: A neurocircuitry analysis. Lancet Psychiatry 2016 , 3 , 760–773. [ Google Scholar ] [ CrossRef ]
- Suzuki, S.; Mell, M.M.; O’Malley, S.S.; Krystal, J.H.; Anticevic, A.; Kober, H. Regulation of craving and negative emotion in alcohol use disorder. Biol. Psychiatry Cogn. Neurosci. Neuroimaging 2020 , 5 , 239–250. [ Google Scholar ] [ CrossRef ]
- Koob, G.F.; Volkow, N.D. Neurocircuitry of addiction. Neuropsychopharmacology 2010 , 35 , 217–238. [ Google Scholar ] [ CrossRef ]
- Volkow, N.D.; Morales, M. The brain on drugs: From reward to addiction. Cell 2015 , 162 , 712–725. [ Google Scholar ] [ CrossRef ]
- Nestler, E.J. Molecular mechanisms of drug addiction. Neuropharmacology 2004 , 47 , 24–32. [ Google Scholar ] [ CrossRef ]
- Solomon, R.L.; Corbit, J.D. An opponent-process theory of motivation: I. Temporal dynamics of affect. Psychol. Rev. 1974 , 81 , 119. [ Google Scholar ] [ CrossRef ]
- Koob, G.F.; Moal, M.L. Drug abuse: Hedonic homeostatic dysregulation. Science 1997 , 278 , 52–58. [ Google Scholar ] [ CrossRef ]
- Olds, J.; Milner, P. Positive reinforcement produced by electrical stimulation of septal area and other regions of rat brain. J. Comp. Physiol. Psychol. 1954 , 47 , 419. [ Google Scholar ] [ CrossRef ]
- Wise, R.A. Dopamine and reward: The anhedonia hypothesis 30 years on. Neurotox. Res. 2008 , 14 , 169–183. [ Google Scholar ] [ CrossRef ]
- Stein, L. Norepinephrine reward pathways: Role in self-stimulation, memory consolidation, and schizophrenia. In Nebraska Symposium on Motivation ; University of Nebraska Press: Lincoln, NE, USA, 1974. [ Google Scholar ]
- Wise, R.A. Action of drugs of abuse on brain reward systems. Pharmacol. Biochem. Behav. 1980 , 13 , 213–223. [ Google Scholar ] [ CrossRef ]
- Yokel, R.A.; Wise, R.A. Increased lever pressing for amphetamine after pimozide in rats: Implications for a dopamine theory of reward. Science 1975 , 187 , 547–549. [ Google Scholar ] [ CrossRef ]
- De Wit, H.; Wise, R.A. Blockade of cocaine reinforcement in rats with the dopamine receptor blocker pimozide, but not with the noradrenergic blockers phentolamine or phenoxybenzamine. Can. J. Psychol./Rev. Can. Psychol. 1977 , 31 , 195. [ Google Scholar ] [ CrossRef ] [ PubMed ]
- Wise, R.A.; Spindler, J.; Legault, L. Major attenuation of food reward with performance-sparing doses of pimozide in the rat. Can. J. Psychol./Rev. Can. Psychol. 1978 , 32 , 77. [ Google Scholar ] [ CrossRef ]
- Wise, R.A.; Rompre, P.P. Brain dopamine and reward. Annu. Rev. Psychol. 1989 , 40 , 191–225. [ Google Scholar ] [ CrossRef ]
- Nutt, D.J.; Lingford-Hughes, A.; Erritzoe, D.; Stokes, P.R. The dopamine theory of addiction: 40 years of highs and lows. Nat. Rev. Neurosci. 2015 , 16 , 305–312. [ Google Scholar ] [ CrossRef ]
- Volkow, N.D.; Wang, G.J.; Fowler, J.S.; Thanos, P.; Logan, J.; Gatley, S.J.; Gifford, A.; Ding, Y.; Pappas, N. Brain DA D2 receptors predict reinforcing effects of stimulants in humans: Replication study. Synapse 2002 , 46 , 79–82. [ Google Scholar ] [ CrossRef ]
- Volkow, N.D.; Wang, G.J.; Fowler, J.S.; Tomasi, D.; Telang, F.; Baler, R. Addiction: Decreased reward sensitivity and increased expectation sensitivity conspire to overwhelm the brain’s control circuit. Bioessays 2010 , 32 , 748–755. [ Google Scholar ] [ CrossRef ]
- Dalley, J.W.; Fryer, T.D.; Brichard, L.; Robinson, E.S.; Theobald, D.E.; Lääne, K.; Peña, Y.; Murphy, E.; Shah, Y.; Probst, K.; et al. Nucleus accumbens D2/3 receptors predict trait impulsivity and cocaine reinforcement. Science 2007 , 315 , 1267–1270. [ Google Scholar ] [ CrossRef ]
- Robinson, T.E.; Berridge, K.C. The neural basis of drug craving: An incentive-sensitization theory of addiction. Brain Res. Rev. 1993 , 18 , 247–291. [ Google Scholar ] [ CrossRef ]
- Wightman, R.M.; Robinson, D.L. Transient changes in mesolimbic dopamine and their association with ‘reward’. J. Neurochem. 2002 , 82 , 721–735. [ Google Scholar ] [ CrossRef ]
- Robbins, T.W.; Everitt, B.J. Drug addiction: Bad habits add up. Nature 1999 , 398 , 567–570. [ Google Scholar ] [ CrossRef ] [ PubMed ]
- Everitt, B.J.; Robbins, T.W. Neural systems of reinforcement for drug addiction: From actions to habits to compulsion. Nat. Neurosci. 2005 , 8 , 1481–1489. [ Google Scholar ] [ CrossRef ] [ PubMed ]
- Everitt, B.J.; Robbins, T.W. From the ventral to the dorsal striatum: Devolving views of their roles in drug addiction. Neurosci. Biobehav. Rev. 2013 , 37 , 1946–1954. [ Google Scholar ] [ CrossRef ] [ PubMed ]
- Verdejo-García, A.; Lawrence, A.J.; Clark, L. Impulsivity as a vulnerability marker for substance-use disorders: Review of findings from high-risk research, problem gamblers and genetic association studies. Neurosci. Biobehav. Rev. 2008 , 32 , 777–810. [ Google Scholar ] [ CrossRef ] [ PubMed ]
- Koob, G.F.; Schulkin, J. Addiction and stress: An allostatic view. Neurosci. Biobehav. Rev. 2019 , 106 , 245–262. [ Google Scholar ] [ CrossRef ]
- Fischer, K.D.; Knackstedt, L.A.; Rosenberg, P.A. Glutamate homeostasis and dopamine signaling: Implications for psychostimulant addiction behavior. Neurochem. Int. 2021 , 144 , 104896. [ Google Scholar ] [ CrossRef ]
- Gipson, C.D.; Rawls, S.; Scofield, M.D.; Siemsen, B.M.; Bondy, E.O.; Maher, E.E. Interactions of neuroimmune signaling and glutamate plasticity in addiction. J. Neuroinflammation 2021 , 18 , 56. [ Google Scholar ] [ CrossRef ]
- Szumlinski, K.K.; Lominac, K.D.; Campbell, R.R.; Cohen, M.; Fultz, E.K.; Brown, C.N.; Miller, B.W.; Quadir, S.Q.; Martin, D.; Thompson, A.B.; et al. Methamphetamine Addiction Vulnerability: The Glutamate, the Bad, and the Ugly. Biol. Psychiatry 2017 , 81 , 959–970. [ Google Scholar ] [ CrossRef ]
- Pfau, M.L.; Ménard, C.; Russo, S.J. Inflammatory mediators in mood disorders: Therapeutic opportunities. Annu. Rev. Pharmacol. Toxicol. 2018 , 58 , 411–428. [ Google Scholar ] [ CrossRef ]
- Ménard, C.; Pfau, M.L.; Hodes, G.E.; Russo, S.J. Immune and neuroendocrine mechanisms of stress vulnerability and resilience. Neuropsychopharmacology 2017 , 42 , 62–80. [ Google Scholar ] [ CrossRef ]
- Koo, J.W.; Wohleb, E.S. How stress shapes neuroimmune function: Implications for the neurobiology of psychiatric disorders. Biol. Psychiatry 2021 , 90 , 74–84. [ Google Scholar ] [ CrossRef ] [ PubMed ]
- Buckley, P.F. Neuroinflammation and schizophrenia. Curr. Psychiatry Rep. 2019 , 21 , 72. [ Google Scholar ] [ CrossRef ]
- Hofford, R.S.; Russo, S.J.; Kiraly, D.D. Neuroimmune mechanisms of psychostimulant and opioid use disorders. Eur. J. Neurosci. 2019 , 50 , 2562–2573. [ Google Scholar ] [ CrossRef ] [ PubMed ]
- Dantzer, R. Neuroimmune interactions: From the brain to the immune system and vice versa. Physiol. Rev. 2018 , 98 , 477–504. [ Google Scholar ] [ CrossRef ] [ PubMed ]
- Pascual, O.; Ben Achour, S.; Rostaing, P.; Triller, A.; Bessis, A. Microglia activation triggers astrocyte-mediated modulation of excitatory neurotransmission. Proc. Natl. Acad. Sci. USA 2012 , 109 , E197–E205. [ Google Scholar ] [ CrossRef ] [ PubMed ]
- Shariq, A.S.; Brietzke, E.; Rosenblat, J.D.; Barendra, V.; Pan, Z.; McIntyre, R.S. Targeting cytokines in reduction of depressive symptoms: A comprehensive review. Prog. Neuro-Psychopharmacol. Biol. Psychiatry 2018 , 83 , 86–91. [ Google Scholar ] [ CrossRef ]
- Harricharan, R.; Abboussi, O.; Daniels, W.M. Addiction: A dysregulation of satiety and inflammatory processes. Prog. Brain Res. 2017 , 235 , 65–91. [ Google Scholar ]
- Nennig, S.E.; Schank, J. The role of NFkB in drug addiction: Beyond inflammation. Alcohol Alcohol. 2017 , 52 , 172–179. [ Google Scholar ] [ CrossRef ]
- Magrone, T.; Jirillo, E. Drugs of abuse induced-subversion of the peripheral immune response and central glial activity: Focus on novel therapeutic approaches. Endocr. Metab. Immune Disord.-Drug Targets (Former. Curr. Drug Targets-Immune Endocr. Metab. Disord.) 2019 , 19 , 281–291. [ Google Scholar ] [ CrossRef ]
- Anand, S.K.; Ahmad, M.H.; Sahu, M.R.; Subba, R.; Mondal, A.C. Detrimental effects of alcohol-induced inflammation on brain health: From neurogenesis to neurodegeneration. Cell. Mol. Neurobiol. 2023 , 43 , 1885–1904. [ Google Scholar ] [ CrossRef ]
- Montesinos, J.; Pascual, M.; Pla, A.; Maldonado, C.; Rodríguez-Arias, M.; Miñarro, J.; Guerri, C. TLR4 elimination prevents synaptic and myelin alterations and long-term cognitive dysfunctions in adolescent mice with intermittent ethanol treatment. Brain Behav. Immun. 2015 , 45 , 233–244. [ Google Scholar ] [ CrossRef ]
- Ferrer-Pérez, C.; Martinez, T.E.; Montagud-Romero, S.; Ballestín, R.; Reguilón, M.D.; Miñarro, J.; Rodríguez-Arias, M. Indomethacin blocks the increased conditioned rewarding effects of cocaine induced by repeated social defeat. PLoS ONE 2018 , 13 , e0209291. [ Google Scholar ] [ CrossRef ]
- Ferrer-Pérez, C.; Reguilón, M.D.; Miñarro, J.; Rodríguez-Arias, M. Oxytocin signaling as a target to block social defeat-induced increases in drug abuse reward. Int. J. Mol. Sci. 2021 , 22 , 2372. [ Google Scholar ] [ CrossRef ] [ PubMed ]
- Haass-Koffler, C.L.; Bartlett, S.E. Stress and addiction: Contribution of the corticotropin releasing factor (CRF) system in neuroplasticity. Front. Mol. Neurosci. 2012 , 5 , 91. [ Google Scholar ] [ CrossRef ]
- Han, X.; DeBold, J.F.; Miczek, K.A. Prevention and reversal of social stress-escalated cocaine self-administration in mice by intra-VTA CRFR1 antagonism. Psychopharmacology 2017 , 234 , 2813–2821. [ Google Scholar ] [ CrossRef ]
- Montagud-Romero, S.; Miñarro, J.; Rodríguez-Arias, M. Unravelling the neuroinflammatory mechanisms underlying the effects of social defeat stress on use of drugs of abuse. In Neuroscience of Social Stress ; Springer International Publishing: Cham, Switzerland, 2021; pp. 153–180. [ Google Scholar ]
Click here to enlarge figure
Theory | Contributions | Limitations |
---|---|---|
| The theory provides a comprehensive explanation addressing both the initial pleasure phase and the subsequent aversive phase in the addictive process. It offers an understanding of the persistence of emotional effects over time, explaining the challenge of overcoming addiction. Recognizes the importance of time and repetition in the development and maintenance of addiction, highlighting the dynamic nature of the process. It proposes concepts such as tolerance and habituation. It can be applied to various substances and addictive behaviors, providing a solid theoretical foundation for understanding different types of addictions. | The theory might oversimplify the complexity of psychological and neurobiological processes involved in addiction. The theory focuses more on emotional response processes than on the underlying causes of addiction. The theory may be perceived as a unidirectional approach by primarily emphasizing the oppositional process after the initial pleasure, without considering other intervening factors. |
| The hypothesis highlights the central role of dopamine in brain circuits associated with reward, providing a foundation for understanding the hedonic component of addiction. It provides a clear and specific explanation of how dopamine influences reward and reinforcement processes. It can be applied to a wide variety of addictive substances, offering a useful theoretical framework for understanding addiction to different drugs. The hypothesis has received support through studies demonstrating changes in dopamine levels in relation to the administration of addictive substances and reward-seeking behaviors. | By primarily focusing on dopamine, the hypothesis may oversimplify the complexity of neurochemical and behavioral factors involved in addiction. It does not adequately address psychosocial and contextual factors that also play crucial roles in the development and maintenance of addiction. Dopaminergic response can vary significantly among individuals, suggesting that factors beyond dopamine may be equally important. Although dopamine is implicated in reward, the hypothesis cannot always accurately predict the development and course of addiction in all cases. |
| The theory emphasizes the significance of sensitization to stimuli associated with drugs, providing insight into how motivation for seeking addictive substances develops. It offers an explanation of how sensitization can persist over time, contributing to an understanding of the chronic nature of addiction. The theory incorporates the motivational dimension, highlighting the transition from initial pleasure-seeking to motivated and persistent drug seeking. Applicable to various addictive substances, providing a broad theoretical framework that is applicable to different types of addictions. | The theory centers more on motivation for seeking addictive substances than addressing the underlying causes of addiction, potentially limiting overall understanding. The theory may not adequately address relapse processes and factors contributing to relapse in addiction. |
| The theory focuses on brain circuits and neuronal structures associated with habits and compulsions, providing a solid neurobiological foundation. Adaptability to different types of addictions: applicable to a variety of substances and addictive behaviors, providing a broad and applicable theoretical framework. The theory considers the influence of the environment and learning in the formation and persistence of habits and compulsions, enriching the perspective. | By focusing on habits and compulsions, the theory might oversimplify the diversity of factors involved in different types of addictions. This theory would not explain why addicts find it impossible to control consumption behavior and why they persist despite the severe consequences of their addiction. It may not comprehensively address the initial motivations leading to addiction, focusing more on the later phases of the addictive cycle. While considering the environment, the theory might lack specificity in precisely how environmental factors influence the formation of habits and compulsions. |
| The theory centers on allostatic processes, providing a perspective that highlights the continuous adaptation of the neurobiological system in response to drug-related demands. Offers an explanation of long-term neurobiological changes associated with addiction, addressing the need to comprehend dynamics over time. Considers stress and stress factors as significant elements in addiction, expanding the understanding beyond substances themselves. The theory addresses the importance of allostatic processes in the relapse cycle, providing a comprehensive view of addictive processes. | Although it considers stress, it might not comprehensively address psychosocial and environmental factors that are also crucial in addiction. By strongly emphasizing neurobiological aspects, the theory might overlook some important psychological and social aspects of addiction. The response to the neuroadaptive mechanisms can vary significantly among individuals. |
Research on neuroinflammation represents an emerging approach in understanding addiction, providing new insights into underlying biological processes. Addresses the interaction between the immune system and the central nervous system, potentially enriching the understanding of addiction beyond traditional aspects. Research on neuroinflammation could lead to the identification of new therapeutic targets for addiction treatment, expanding available options. Understanding neuroinflammation could have significant implications for addressing comorbidities associated with addiction and neuropsychiatric disorders. | The interaction between neuroinflammation and addiction is complex, making it challenging to identify clear causal relationships and specific mechanisms. While ongoing research exists, solid empirical evidence regarding the precise contribution of neuroinflammation to addiction may still be limited. Some neuroinflammation approaches may focus on later phases of the addictive process, leaving gaps in understanding initial events and predisposition. The clinical application of neuroinflammation research may face challenges, from identifying effective interventions to practical implementation in clinical settings. |
The statements, opinions and data contained in all publications are solely those of the individual author(s) and contributor(s) and not of MDPI and/or the editor(s). MDPI and/or the editor(s) disclaim responsibility for any injury to people or property resulting from any ideas, methods, instructions or products referred to in the content. |
Share and Cite
Ferrer-Pérez, C.; Montagud-Romero, S.; Blanco-Gandía, M.C. Neurobiological Theories of Addiction: A Comprehensive Review. Psychoactives 2024 , 3 , 35-47. https://doi.org/10.3390/psychoactives3010003
Ferrer-Pérez C, Montagud-Romero S, Blanco-Gandía MC. Neurobiological Theories of Addiction: A Comprehensive Review. Psychoactives . 2024; 3(1):35-47. https://doi.org/10.3390/psychoactives3010003
Ferrer-Pérez, Carmen, Sandra Montagud-Romero, and María Carmen Blanco-Gandía. 2024. "Neurobiological Theories of Addiction: A Comprehensive Review" Psychoactives 3, no. 1: 35-47. https://doi.org/10.3390/psychoactives3010003
Article Metrics
Article access statistics, further information, mdpi initiatives, follow mdpi.
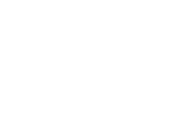
Subscribe to receive issue release notifications and newsletters from MDPI journals
- Open access
- Published: 02 December 2021
Dopamine, behavior, and addiction
- Roy A. Wise ORCID: orcid.org/0000-0002-9115-6654 1 , 2 &
- Chloe J. Jordan 3
Journal of Biomedical Science volume 28 , Article number: 83 ( 2021 ) Cite this article
30k Accesses
39 Citations
3 Altmetric
Metrics details
Addictive drugs are habit-forming. Addiction is a learned behavior; repeated exposure to addictive drugs can stamp in learning. Dopamine-depleted or dopamine-deleted animals have only unlearned reflexes; they lack learned seeking and learned avoidance. Burst-firing of dopamine neurons enables learning—long-term potentiation (LTP)—of search and avoidance responses. It sets the stage for learning that occurs between glutamatergic sensory inputs and GABAergic motor-related outputs of the striatum; this learning establishes the ability to search and avoid. Independent of burst-firing, the rate of single-spiking—or “pacemaker firing”—of dopaminergic neurons mediates motivational arousal. Motivational arousal increases during need states and its level determines the responsiveness of the animal to established predictive stimuli. Addictive drugs, while usually not serving as an external stimulus, have varying abilities to activate the dopamine system; the comparative abilities of different addictive drugs to facilitate LTP is something that might be studied in the future.
Addictive drugs are habit-forming. Here we use the phase “habit-forming broadly [ 1 ] to refer to the entire progression toward the stimulus–response end habits [ 2 ] discussed in the specialist literature.Rewards are habit-forming because predictive stimuli—reward-predictors as well as punishment-predictors—come to cause dopaminergic burst-firing, and burst-firing enhances or enables the separate development of long-term potentiation (LTP) and long-term depression (LTD) of learned connections between other systems: glutamatergic input pathways and GABAergic output pathways [ 3 ]. The primary source of these is in the striatum; the striatum receives sensory inputs from the cortex and sends motor-related outputs that are essential for food-searching and punishment-avoidance. The primary evidence for dopaminergic involvement in reward-driven learning comes from studies of genetically altered mice that cannot synthesize dopamine in the brain. These mice appear normal when born, but they fail to learn food-seeking and, after weaning, die of starvation unless they are force-fed [ 4 ]. Such animals have only unconditioned reflexes—“consummatory” reflexes [ 5 ]—and, having not learned to feed,—an “appetitive” response [ 5 ]—also fail to learn to seek or avoid other rewards. That dopamine is critical for such learning is evident from the dopaminergic recordings of Schultz and colleagues [ 6 , 7 ] and from recent optogenetic studies that confirm dopaminergic activation as rewarding [ 8 , 9 , 10 , 11 ].
Dopamine-deficient animals
Dopamine-deficient animals are born with minimal—learned in utero—knowledge of the environment. These animals have normal reflexes: they can swallow food if it is placed in their mouth [ 12 ] and they escape from aversive stimuli [ 13 , 14 , 15 ]. They do not, however, learn to search for rewards or avoid aversive stimuli [ 4 , 16 ]. They must learn to approach the environmental cues that guide behavior, leading to food and other rewards [ 17 , 18 ], just as they must learn to avoid predictive stimuli that warn of punishers. Learned approach to rewards is an appetitive behavior and is essential for addiction as well as for feeding; indeed, the strongest evidence of drug self-administration involved responses to predictors because the animals, in these cases, rarely have sensory contact with the drug itself [ 19 ].
Dopaminergic burst-firing enables environmental learning
Genetically modified neonates that cannot synthesize dopamine in the brain [ 4 ], like adult animals with their dopamine systems lesioned [ 16 ] or blocked pharmacologically [ 20 , 21 , 22 ]—dopamine challenged animals—fail to find and eat external foods [ 20 , 22 ] and fail to seek and consume addictive drugs [ 3 , 23 , 24 , 25 ] or other rewards [ 21 , 26 , 27 ].
The dopamine system is activated by three kinds of external stimuli: rewarding stimuli, punishing stimuli, and novel stimuli. When activated by rewards or punishers, portions of the dopamine system discharge in bursts [ 6 , 28 , 29 , 30 , 31 , 32 ], whereas other portions are inhibited [ 33 , 34 , 35 ]. Dopaminergic burst discharges involve two or more linked spikes with progressively decreasing amplitude and short inter-discharge intervals (about 60 ms between the first and second discharge and about 120 ms between subsequent discharges) [ 36 ]. These discharges cause very local accumulations of dopamine in the striatum, at local peaks of 100 nM or greater concentration, as measured by fast-scan cyclic voltammetry (FSCV) [ 37 ].
The primary sensory inputs to the dopamine system—driving this release—are glutamatergic and perhaps cholinergic terminals from cell bodies of the latero-dorsal and pedunculopontine tegmental nuclei [ 38 , 39 ]; similar burst firing can be activated as well by direct glutamatergic stimulation of dopaminergic neurons in isolated brain slices [ 40 ]. In dopamine-intact animals, dopaminergic neurons burst-fire in response not just to rewards or punishers but also to stimuli that reliably precede—and thus predict—rewards and punishers [ 6 , 7 , 41 ].
The burst-firing in response to predictors of rewards or punishers develops with age, as the animal learns about the environment. The burst-responses should not really be seen as travelling from the unconditioned rewards and punishers to their predictors; rather, the process of burst-firing develops anew in response to predictors that involve a Hebbian mechanism [ 42 , 43 ]. Hebb has postulated a mechanism by which repeated synaptic input from a (predictor) cell that reliably precedes another (reward) neuron becomes linked to its target. As responses to predictors develop, the burst-responding in response to the actual rewards or punishers is temporarily lost; responsiveness, however—in this case inhibition of firing—appears when the reward or punisher fails to appear at the expected time [ 44 ]. When burst-firing develops in response to reward-predictors it enables cellular learning in surrounding synapses; these are glutamate-GABA synapses localized within microns of the sites of dopamine release.
Burst-firing of the dopamine system is only a first step in the learning; the formation of the synapses for searching develops in other cellular elements. Dopamine bursting enables development of long-term potentiation (LTP) and long-term depression (LTD), and, in the striatum, this occurs between glutamatergic sensory inputs and GABAergic motor-related outputs [ 45 , 46 ]. Dopamine in the striatum reaches and binds to high-affinity D2 dopamine receptors and low-affinity D1 receptors [ 48 , 49 ]. At high affinity D2 receptors significant binding occurs, making D2 receptors particularly sensitive to phasic decreases in dopamine release. At low affinity D1 receptors less dopamine should be bound, making D1 receptors particularly sensitive to phasic increases in dopamine release. Movements result when D1 receptors are activated and inhibition of movement results when D2 receptors are activated [ 9 ]. In behaving animals, activation of D1 and D2 are momentary complements; their activations occur concurrently [ 50 ]. Concurrent activation presumably involves activating one subset of muscles (D1) to do something while inhibiting (D2) other sets of muscles, antagonistic muscles, that would normally interfere with the elicited action. The reward-predicting stimuli that lead an animal to anticipate rewards—both natural rewards and drug rewards—are established by this kind of learning [ 3 , 25 ].
Dopaminergic pacemaker-firing modulates motivation
Whereas burst responding of the dopamine system is elicited by external stimuli, dopaminergic single discharges also spontaneously occur; these discharges are identified as pacemaker firing because they result from a depolarizing current within the dopaminergic cells themselves [ 51 ]. Such discharges can be seen in brain slice preparations even when, in vitro, excitatory inputs have been eliminated [ 40 ]. Pacemaker firing is slower than burst firing; it occurs at about half the frequency of burst-firing [ 51 ]. The rate of pacemaker firing is modulated by two sources: by increases or decreases of inhibitory inputs from GABA-containing cells [ 52 ] and by hormones and peptides that act at receptors on dopaminergic neurons themselves [ 53 , 54 , 55 ] or that act through their inputs [ 55 , 56 , 57 ]. The tonic modulation of the dopamine system—pacemaker firing, supplemented by episodes of burst-firing—is a correlate of, presumably a cause of, motivational arousal.
Motivational arousal is a state variable; it regulates readiness to respond to external stimuli. While rewards and punishers elicit responses regardless of emotional state, it is predictors of rewards or punishers that depend on motivational arousal. In resting animals, it is pacemaker firing that varies as a function of internal state and determines when, and to what degree, the animal responds to reward-predictors. Burst-firing can also influence motivational arousal; consider the behavior of an animal when a pheromone-emitting conspecific passes nearby. Motivational arousal varies over time and, in resting animals, determines when a previously sated animal starts to become hungry and interested in seeking food.
In a resting animal, the release of dopamine is detected historically by microdialysis [ 58 ]. Baseline levels of dopamine are estimated to be around 5 nM [ 59 , 60 ]; microdialysis can measure dopamine levels this low and much lower; microdialysis—in tetrodotoxin-treated animals—can measure dopamine at 1% of baseline levels [ 61 ]. However, microdialysis is an insensitive measure, averaging rather than giving data from single cells; it involves the sampling of extracellular fluids through large (~ 250 microns) push–pull cannulae in the brain, in contact with many dopamine terminals, and it usually gives averages taken over minutes or tens of minutes. One possibility is that basal dopamine levels are near 5 nM at all points throughout the striatum; alternatively, it is possible that microdialysis simply reflects the average of large fluctuations around some unknown actual baseline level. In contrast, however, the alternative—FSCV, for example—measures individual elevations and does not have the sensitivity to detect the low levels of dopamine in resting animals; it is insensitive to dopamine at concentrations below 20 nM [ 37 ] and uses “background subtraction” to isolate dopamine fluctuations from noise [ 62 ]. FSCV measures peak concentrations that are isolated both in localization and in time. Because the degree of temporal and spatial heterogeneity is not known, it is not clear the degree to which these isolated dopamine peaks contribute to the motivational arousal in active animals. More recently developed techniques involving optical technology, calcium imaging, and genetically-encoded fluorescent protein sensors [ 63 ] will give us better methods for assessing pacemaker dopamine discharge.
The evidence implicating a causal role of dopamine in motivational function comes from experiments where the dopamine system has been experimentally manipulated [ 25 ]. These include the following: Animals with partial dopamine depletion show reduced energy in learned tasks [ 64 ]. Parkinsonian patients with decreased dopamine levels have deficits in speed of hand movements [ 65 ] and in willingness to squeeze a dynamometer [ 66 ]; when dopamine is replaced by L-DOPA administration, these symptoms decrease [ 66 ]. Amphetamine, which augments dopamine release, causes humans to increase effort for monetary rewards [ 67 ]. A dopamine uptake inhibitor that doubles baseline dopamine levels increases willingness to work for high-carbohydrate pellets [ 68 ]. Restoring dopamine by re-establishing synthesis in dopaminergic neurons restores locomotion and food-seeking in dopamine-deficient mice [ 69 , 70 ].
The fact that dopamine-depleted animals already have responses to rewards and punishers allows a stronger definition of motivation than has been offered in the past; the level of motivation varies with responsiveness to predictive stimuli in the environment. The distinction here is between predictive stimuli that lead toward or away from rewards or punishers and rewarding and punishing stimuli themselves, to which dopamine-depleted animals continue to respond.
Motivation is not a linear function of dopamine levels and may vary with noradrenergic as well as dopaminergic motivation. Motivation is low when dopamine levels are low, and it increases as dopamine levels start to increase. However, when dopamine levels are doubled or tripled—such levels as are induced by self-administered doses of amphetamine [ 71 ], cocaine [ 72 ], or opiates [ 73 ]—motivation is lost [ 74 ]. Thus the relation that links dopamine level with motivation appears to be a classic “U”-shaped function; such functions have traditionally been associated with arousal and motivation [ 75 , 76 ].
Predictive cues can become aversive
Wheeler and colleagues have suggested conditions in which cocaine-predictive cues can become associated with negative affect [ 77 , 78 , 79 ]. The first of these papers discussed “cocaine predictive” cues, but the second paper more correctly characterized them as cues of “delayed cocaine delivery.” The parameters of establishing the association of a sweet-tasting substance with aversive conditioning are of particular interest, in part because people who use addictive drugs sometimes appear to do so in anticipation of, or in fear of, expected aversive symptoms.
In the Wheeler studies, animals were given series of 30 or 45 min-long, intra-oral taste stimuli that preceded 2 h sessions of intravenous saline or cocaine self-administration. After several days of training the facial expression elicited by the taste cues [ 77 ] and the effect of these cues on dopamine release [ 78 ] were determined. A taste cue that preceded subsequent saline self-administration caused licking and lateral tongue movements—these are responses driven by sweet solutions—whereas cues that predicted delayed cocaine self-administration had come to cause gagging and gaping—the responses to aversive quinine solutions—[ 77 ]. Moreover, the cue predicting saline self-administration increased dopamine release, whereas the cue predicting cocaine self-administration inhibited dopamine release [ 77 ]. The critical factor here is that it was the predictor of delayed cocaine availability that became aversive. Delayed cocaine availability is not well associated with dopamine release; dopamine release is directly controlled by what happens in seconds after the prediction [ 44 ]. The immediate consequence—for the dopamine system—of the cue that predicted delayed cocaine was the absence of dopamine it caused after training with series of 29 or 44 one-minute cue exposures.
Dopamine and addictive drugs
Roles for dopamine in reward theory [ 80 , 81 , 82 ] and a role of reward in addiction [ 83 ] were established shortly after dopamine was established as a neurotransmitter. Dopamine was first identified with reward function from anatomical [ 84 ] and pharmacological evidence [ 85 , 86 , 87 , 88 , 89 ]; it was subsequently implicated as well in motivational function [ 90 , 91 , 92 ]. Dopamine has broadly been associated with the rewarding effects of addictive drugs, particularly in the process of establishing habitual drug intake [ 24 , 93 , 94 ]. However, dopamine plays strongly established roles in the addictive properties of some drugs but is implicated by minimal evidence in others.
Amphetamine and cocaine The role of dopamine in the rewarding effects of the psychomotor stimulants—amphetamine and cocaine—are strongly established. Self-administered doses of amphetamine [ 71 ] or cocaine [ 72 ] elevate dopamine levels over four-fold. Dopamine antagonists at high doses block amphetamine and cocaine self-administration [ 86 , 95 , 96 ]. At low doses the antagonists cause compensatory increases in responding, suggesting that the rewarding effects of the stimulants has been attenuated [ 86 , 95 , 96 ]. Dopamine-selective lesions cause immediate loss of cocaine self-administration when the lesions are complete [ 97 ] and temporary loss when they are incomplete [ 98 ]. These lesioned animals continue to lever-press for the direct dopamine agonist, apomorphine, following these lesions, confirming that the lesioned animals remember their training history and have normal motor capacity [ 97 , 98 ]. Finally, cocaine and amphetamine induces long-term synaptic changes in glutamate-GABA synapses in the striatum [ 99 , 100 , 101 ].
Opiates Heroin self-administration is also clearly dopamine-dependent. It more than triples resting dopamine levels [ 73 ], and while the role of dopamine in opiate addiction has been questioned [ 102 ], evidence from intravenous heroin self-administration studies makes it clear that animals usually request additional heroin each time their dopamine levels fall below about twice-normal levels [ 103 ]. An important possibility in experiments blocking opiate self-administration with dopamine antagonists is that the antagonists act not only at post-synaptic receptors but also at dopamine autoreceptors [ 104 ] where they increase dopamine firing and dopamine release. By increasing dopamine release—as heroin alone does not—dopamine antagonists elevate extracellular dopamine at the nerve terminal, desensitizing the system to the antagonist and, in this case, requiring more heroin to be effective. In any case, dopamine antagonists do block opiate self-administration [ 102 ]; the lack of compensatory increases in responding for heroin following low doses of dopamine antagonists [ 102 ] does not [ 105 ] rule out a role for dopamine in opiate reward. Studies of opiate-conditioned place preferences adds to the evidence that opiates are habit-forming—place-preferences address the first element of search-habits, the locomotion to the place where drugs are available—and that their habit-forming effects are blocked by dopamine antagonists [ 106 , 107 ].
Nicotine Self-administration of nicotine also appears to be dopamine-dependent. Nicotine self-administration causes burst-firing of dopaminergic neurons [ 108 , 109 ] and elevates dopamine levels to 150–200% of baseline [ 110 ]. It is disrupted by selective dopaminergic antagonists [ 111 ] and selective neurochemical lesions [ 112 ]. Nicotine acts at sites and on receptors expressed by dopamine neurons and inhibitory controllers of dopamine neurons, such as local GABAergic cells within the ventral tegmental area (VTA). Deletion of nicotinic receptor subunits, such as β2, abolishes nicotine-induced dopamine release and attenuates nicotine self-administration, and re-expression of β2 restores nicotine’s rewarding effects [ 113 , 114 , 115 ]. Nicotine causes conditioned place preferences; this is blocked with dopamine antagonists [ 116 ]. Nicotine enables LTP in glutamatergic inputs to the dopamine system and primes the ability of cocaine to induce LTP in the amygdala [ 117 , 118 ], a structure anatomically related to the striatum [ 119 ].
Ethanol The evidence that dopamine is important for the rewarding effects of ethanol is also substantial but weaker than that supporting dopamine involvement in stimulant or opiate reward. Part of the problem is that we still have no animal model of self-administration that is sufficient to maintain intoxication [ 120 ]; rats can be induced to drink alcohol [ 121 , 122 , 123 , 124 ], and animals can be made physically dependent on alcohol [ 125 , 126 ], however, even in already dependent rats, voluntary self-administration that maintains dependence is not seen. Ethanol (and ethanol withdrawal) increases burst-firing in dopaminergic animals [ 127 , 128 ]; ethanol also increases pacemaker dopaminergic firing [ 129 ]. Ethanol can increase dopamine levels to 150–200% of baseline [ 94 ], and increases dopamine cell burst-firing as well as pacemaker-like firing in the VTA; note, however, that a subset of VTA dopamine neurons are instead inhibited by ethanol [ 128 ] and this might also be important.
Dopamine antagonists decrease lever-pressing for ethanol in a sucrose-fading procedure [ 130 , 131 ]; this is done in animals that were experienced with ethanol and during intervals of alcohol deprivation. However, the case of alcohol is unusual. In a conditioned place preference study, alcohol is reported to be dopamine-dependent in alcohol-naive animals but not in withdrawn, experienced, animals [ 132 ]. One possibility is that a dopamine-independent pathway is also involved in ethanol reinforcement [ 132 , 133 ]. Ethanol enhances synaptic plasticity in the striatum [ 101 ].
Cannabis There are many cannabinoids, some of which have psychoactive effects and remain to be studied. The primary psychoactive ingredient in marijuana is ∆9 -tetrahydrocannabinol (THC). While some studies have reported that this agent is self-administered intravenously by rodents [ 134 ] and non-human primates [ 135 ] and increases striatal dopamine levels [ 136 , 137 , 138 , 139 ], other studies suggest that THC is not very rewarding in other animals, such that THC self-administration is modest and difficult to sustain [ 140 , 141 ]. Newer rodent models of edible or vaporized THC self-administration hold promise [ 142 , 143 ]. However, species differences in cannabinoid receptor expression and distribution, particularly in the VTA, may underlie differences in the rewarding effects of THC between humans, non-human primates and rodents [ 144 ].
THC is an unusual agent; two of its endogenous analogues—anandamide, 2-arachidonylglycerol—are expressed by dopaminergic (and other) neurons and are released when dopaminergic neurons fire; they influence dopamine turnover through actions on inputs to the dopamine system [ 145 , 146 ]. THC is self-administered into two dopamine-rich regions, the posterior VTA where mesolimbic dopamine cell bodies are found and the posterior ventral striatum, where terminals of that system terminate [ 147 ]; these sites of action implicate THC’s actions on the dopamine system in reward function and the use of central drug self-administration suggests that site-specificity is important here.
Barbiturates and benzodiazepines Much less is known about self-administered doses of barbiturates or benzodiazepines. Barbiturates [ 148 , 149 ] and benzodiazepines [ 150 , 151 ] are self-administered both intravenously and intracranially into the VTA [ 152 , 153 ] by animals. Benzodiazepines increase VTA dopamine neuron firing and induce LTP in glutamatergic inputs to VTA dopamine neurons through positive modulation of local GABA A receptors [ 154 , 155 , 156 , 157 ]. At experimenter-selected doses they elevate dopamine levels [ 158 , 159 , 160 , 161 ] and it has been suggested that they are addictive for this reason [ 24 ].
Caffeine Caffeine is self-administered by animals [ 148 , 162 , 163 ] and produces conditioned flavor preferences (low doses) or conditioned place aversions (high doses) in rats when injected intraperitoneally or directly into the VTA [ 164 ]. A dopamine antagonist injected into the shell of the ventral striatum blocks these place preferences, whereas the antagonist injected into the core of the ventral striatum blocks the conditioned aversive effects [ 165 ]. Volatized, inhaled caffeine increases extracellular dopamine levels in the nucleus accumbens shell [ 166 ]. The mechanism by which caffeine does so is not clear. The main actions of caffeine are mediated through actions at adenosine receptors that form heteromers with dopamine receptors. However, in human Positron Emission Tomography (PET) studies, caffeine increases D2/D3 receptor availability in the ventral striatum, suggesting caffeine alone does not directly increase dopamine levels in this region [ 167 ]. Other studies suggest that caffeine enhances the rewarding effects of other manipulations, such as exercise [ 168 ] or ethanol consumption [ 65 , 169 ].
Conclusions
Learned behavior—perhaps all or almost-all learned behavior—depends on dopamine function; dopamine deficient animals fail to learn to search for food or other rewards and fail to learn to avoid predictable punishers. Dopamine neurons discharge in bursts when triggered by external stimuli, and this burst-firing enables formation of potentiated glutamate-GABA signaling that is critical for learned searching. Dopamine neurons also discharge in slower single-impulse pacemaker firing and the rate of this firing appears to determine motivation in resting (inanimate) animals. The ability of addictive drugs to cause burst-like discharges in the dopamine system is the broadly assumed correlate of addiction, but the direct evidence for this assumption is linked strongly to amphetamine, cocaine, and opiates; the evidence is weaker for nicotine and alcohol, cannabis, barbiturates, benzodiazepines, and caffeine. The abilities of different addictive drugs to enable long-term potentiation and facilitate habit formation via dopaminergic mechanisms should be compared in future studies.
Availability of data and materials
Not applicable.
Singer BF, Fadanelli M, Kawa AB, Robinson TE. Are cocaine-seeking “habits” necessary for the development of addiction-like behavior in rats? J Neurosci. 2018;38(1):60–73.
Article CAS PubMed PubMed Central Google Scholar
Balleine BW, Dickinson A. Goal-directed instrumental action: contingency and incentive learning and their cortical substrates. Neuropharmacology. 1998;37(4–5):407–19.
Article CAS PubMed Google Scholar
Wise RA. Dopamine, learning and motivation. Nat Rev Neurosci. 2004;5:483–94.
Palmiter RD. Dopamine signaling in the dorsal striatum is essential for motivated behaviors: lessons from dopamine-deficient mice. Ann NY Acad Sci. 2008;1129:35–46.
Craig W. Appetites and aversions as constituents of instincts. Biol Bull. 1918;34:91–107.
Article Google Scholar
Ljungberg T, Apicella P, Schultz W. Responses of monkey dopamine neurons during learning of behavioral reactions. J Neurophysiol. 1992;67:145–63.
Schultz W, Apicella P, Ljungberg T. Responses of monkey dopamine neurons to reward and conditioned stimuli during successive steps of learning a delayed response task. J Neurosci. 1993;13:900–13.
Witten IB, Steinberg EE, Lee SY, Davidson TJ, Zalocusky KA, Brodsky M, et al. Recombinase-driver rat lines: tools, techniques, and optogenetic application to dopamine-mediated reinforcement. Neuron. 2011;72:721–33.
Kravitz AV, Tye LD, Kreitzer AC. Distinct roles for direct and indirect pathway striatal neurons in reinforcement. Nat Neurosci. 2012;15(6):816–8.
Ilango A, Kesner AJ, Keller KL, Stuber GD, Bonci A, Ikemoto S. Similar roles of substantia nigra and ventral tegmental dopamine neurons in reward and aversion. J Neurosci. 2014;34(3):817–22.
Steinberg EE, Boivin JR, Saunders BT, Witten IB, Deisseroth K, Janak PH. Positive reinforcement mediated by midbrain dopamine neurons requires D1 and D2 receptor activation in the nucleus accumbens. PLoS ONE. 2014;9(4):e94771.
Article PubMed PubMed Central Google Scholar
Zhou QY, Palmiter RD. Dopamine-deficient mice are severely hypoactive, adipsic, and aphagic. Cell. 1995;83(7):1197–209.
Cooper BR, Breese GR, Grant LD, Howard JL. Effects of 6-hydroxydopamine treatments on active avoidance responding: evidence for involvement of brain dopamine. J Pharmacol Exp Ther. 1973;185(2):358–70.
CAS PubMed Google Scholar
Fibiger HC, Phillips AG, Zis AP. Deficits in instrumental responding after 6-hydroxydopamine lesions of the nigro-neostriatal dopaminergic projection. Pharmacol Biochem Behav. 1974;2(1):87–96.
Barbano MF, Wang HL, Zhang S, Miranda-Barrientos J, Estrin DJ, Figueroa-González A, et al. VTA glutamatergic neurons mediate innate defensive behaviors. Neuron. 2020;107(2):368-82.e8.
Ungerstedt U. Adipsia and aphagia after 6-hydroxydopamine induced degeneration of the nigro-striatal dopamine system. Acta Physiol Scand. 1971;367:95–122.
Article CAS Google Scholar
Bindra D. Neuropsychological interpretation of the effects of drive and incentive-motivation on general activity and instrumental behavior. Psychol Rev. 1968;75:1–22.
Bolles RC. Reinforcement, expectancy, and learning. Psychol Rev. 1972;79:394–409.
Wise RA. Brain reward circuitry: Insights from unsensed incentives. Neuron. 2002;36:229–40.
Wise RA, Spindler J, deWit H, Gerber GJ. Neuroleptic-induced “anhedonia” in rats: pimozide blocks reward quality of food. Science. 1978;201(4352):262–4.
Gerber GJ, Sing J, Wise RA. Pimozide attenuates lever pressing for water reinforcement in rats. Pharmacol Biochem Behav. 1981;14(2):201–5.
Wise RA, Schwartz HV. Pimozide attenuates acquisition of lever pressing for food in rats. Pharmacol Biochem Behav. 1981;15:655–6.
Wise RA. Neuroleptics and operant behavior: the anhedonia hypothesis. Behav Brain Sci. 1982;5:39–87.
Wise RA, Bozarth MA. A psychomotor stimulant theory of addiction. Psychol Rev. 1987;94(4):469–92.
Wise RA, Robble MA. Dopamine and addiction. Ann Rev Psychol. 2020;71:79–106.
Robinson DL, Phillips PE, Budygin EA, Trafton BJ, Garris PA, Wightman RM. Sub-second changes in accumbal dopamine during sexual behavior in male rats. NeuroReport. 2001;12(11):2549–52.
El-Guebaly N, Mudry T, Zohar J, Tavares H, Potenza MN. Compulsive features in behavioural addictions: the case of pathological gambling. Addiction (Abingdon, England). 2012;107(10):1726–34.
Strecker RE, Jacobs BL. Substantia nigra dopaminergic unit activity in behaving cats: effect of arousal on spontaneous discharge and sensory evoked activity. Brain Res. 1985;361(1–2):339–50.
Schultz W. Responses of midbrain dopamine neurons to behavioral trigger stimuli in the monkey. J Neurophysiol. 1986;56:1439–61.
Horvitz JC, Stewart T, Jacobs BL. Burst activity of ventral tegmental dopamine neurons is elicited by sensory stimuli in the awake cat. Brain Res. 1997;759(2):251–8.
Brischoux F, Chakraborty S, Brierley DI, Ungless MA. Phasic excitation of dopamine neurons in ventral VTA by noxious stimuli. PNAS. 2009;106(12):4894–9.
Matsumoto M, Hikosaka O. Two types of dopamine neuron distinctly convey positive and negative motivational signals. Nature. 2009;459(7248):837–41.
Matsumoto H, Tian J, Uchida N, Watabe-Uchida M. Midbrain dopamine neurons signal aversion in a reward-context-dependent manner. eLife. 2016;5.
Juarez B, Han MH. Diversity of dopaminergic neural circuits in response to drug exposure. Neuropsychopharmacology. 2016;41(10):2424–46.
Verharen JPH, Luijendijk MCM, Vanderschuren L, Adan RAH. Dopaminergic contributions to behavioral control under threat of punishment in rats. Psychopharmacology. 2020;237(6):1769–82.
Grace AA, Bunney BS. The control of firing pattern in nigral dopamine neurons: burst firing. J Neurosci. 1984;4:2877–90.
Owesson-White CA, Roitman MF, Sombers LA, Belle AM, Keithley RB, Peele JL, et al. Sources contributing to the average extracellular concentration of dopamine in the nucleus accumbens. J Neurochem. 2012;121(2):252–62.
Floresco SB, West AR, Ash B, Moore H, Grace AA. Afferent modulation of dopamine neuron firing differentially regulates tonic and phasic dopamine transmission. Nat Neurosci. 2003;6(9):968–73.
Lodge DJ, Grace AA. The laterodorsal tegmentum is essential for burst firing of ventral tegmental area dopamine neurons. PNAS. 2006;103(13):5167–72.
Grace AA, Onn SP. Morphology and electrophysiological properties of immunocytochemically identified rat dopamine neurons recorded in vitro. J Neurosci. 1989;9(10):3463–81.
Kim KM, Baratta MV, Yang A, Lee D, Boyden ES, Fiorillo CD. Optogenetic mimicry of the transient activation of dopamine neurons by natural reward is sufficient for operant reinforcement. PLoS ONE. 2012;7(4):e33612.
Hebb DO. The organization of behavior. New York: Wiley; 1949.
Google Scholar
Hebb DO. Essay on mind. New York: Erlbaum; 1980.
Schultz W, Dayan P, Montague PR. A neural substrate of prediction and reward. Science. 1997;275:1593–9.
Centonze D, Picconi B, Gubellini P, Bernardi G, Calabresi P. Dopaminergic control of synaptic plasticity in the dorsal striatum. Eur J Neurosci. 2001;13:1071–7.
Calabresi P, Picconi B, Tozzi A, Di Filippo M. Dopamine-mediated regulation of corticostriatal synaptic plasticity. Trends Neurosci. 2007;30(5):211–9.
Surmeier DJ, Ding J, Day M, Wang Z, Shen W. D1 and D2 dopamine-receptor modulation of striatal glutamatergic signaling in striatal medium spiny neurons. Trends Neurosci. 2007;30(5):228–35.
Richfield EK, Penney JB, Young AB. Anatomical and affinity state comparisons between dopamine D1 and D2 receptors in the rat central nervous system. Neuroscience. 1989;30(3):767–77.
Neve KA, Neve RL. Molcular biology of dopamine receptors. In: Neve KA, Neve RL, editors. The dopamine receptors. Totowa: Humana Press; 1997. p. 27–76.
Chapter Google Scholar
Cui G, Jun SB, Jin X, Pham MD, Vogel SS, Lovinger DM, et al. Concurrent activation of striatal direct and indirect pathways during action initiation. Nature. 2013;494(7436):238–42.
Grace AA, Bunney BS. The control of firing pattern in nigral dopamine neurons: single spike firing. J Neurosci. 1984;4(11):2866–76.
Grace AA. The regulation of dopamine neuron activity as determine by in vivo and in vitro intracellular recordings. In: Chiodo LA, Freeman A, editors. Neurophysiology of dopaminergic systems: current status and Clinical perspectives. Detroit: Lakeshor Publishing; 1987. p. 1–66.
Figlewicz DP, Evans SB, Murphy J, Hoen M, Baskin DG. Expression of receptors for insulin and leptin in the ventral tegmental area/substantia nigra (VTA/SN) of the rat. Brain Res. 2003;964(1):107–15.
Fulton S, Pissios P, Manchon RP, Stiles L, Frank L, Pothos EN, et al. Leptin regulation of the mesoaccumbens dopamine pathway. Neuron. 2006;51(6):811–22.
Jerlhag E, Egecioglu E, Dickson SL, Engel JA. Glutamatergic regulation of ghrelin-induced activation of the mesolimbic dopamine system. Addiction Biol. 2011;16(1):82–91.
Leinninger GM, Jo YH, Leshan RL, Louis GW, Yang H, Barrera JG, et al. Leptin acts via leptin receptor-expressing lateral hypothalamic neurons to modulate the mesolimbic dopamine system and suppress feeding. Cell Metab. 2009;10(2):89–98.
Mietlicki-Baase EG, Ortinski PI, Rupprecht LE, Olivos DR, Alhadeff AL, Pierce RC, et al. The food intake-suppressive effects of glucagon-like peptide-1 receptor signaling in the ventral tegmental area are mediated by AMPA/kainate receptors. Amer J Physiol Endocrinol Metab. 2013;305(11):E1367–74.
Tossman U, Ungerstedt U. Microdialysis in the study of extracellular levels of amino acids in the rat brain. Acta Physiol Scand. 1986;128(1):9–14.
Parsons LH, Justice JB. Extracellular concentration and in vivo recovery of dopamine in the nucleus accumbens using microdialysis. J Neurochem. 1992;58:212–8.
Sam PM, Justice JB Jr. Effect of general microdialysis-induced depletion on extracellular dopamine. Anal Chem. 1996;68(5):724–8.
Westerink BHC, Tuntler J, Damsma G, Rollema H, De Vries JB. The use of tetrodotoxin for the characterization of drug-enhanced dopamine release in conscious rats studied by brain dialysis. Naunyn-Schmiedeberg’s Arch Pharmacol. 1987;336:502–7.
Howell JO, Kuhr WG, Ensman RE, Wightman RM. Background subtraction for rapid scan voltammetry. J Electroanalytical Chem Interfacial Electrochem. 1986;209:77–90.
Yocky AG, Covey DP. Evolution of in vivo dopamine monitoring techniques. Pharmacol Biochem Behav. 2021;200:173078.
Salamone JD, Correa M, Farrar A, Mingote SM. Effort-related functions of nucleus accumbens dopamine and associated forebrain circuits. Psychopharmacology. 2007;191(3):461–82.
Mazzoni P, Hristova A, Krakauer JW. Why don’t we move faster? Parkinson’s disease, movement vigor, and implicit motivation. J Neurosci. 2007;27(27):7105–16.
Chong TT, Bonnelle V, Manohar S, Veromann KR, Muhammed K, Tofaris GK, et al. Dopamine enhances willingness to exert effort for reward in Parkinson’s disease. Cortex. 2015;69:40–6.
Wardle MC, Treadway MT, Mayo LM, Zald DH, de Wit H. Amping up effort: effects of d-amphetamine on human effort-based decision-making. J Neurosci. 2011;31(46):16597–602.
Yohn SE, Errante EE, Rosenbloom-Snow A, Somerville M, Rowland M, Tokarski K, et al. Blockade of uptake for dopamine, but not norepinephrine or 5-HT, increases selection of high effort instrumental activity: implications for treatment of effort-related motivational symptoms in psychopathology. Neuropharmacol. 2016;109:270–80.
Szczypka MS, Mandel RJ, Donahue BA, Snyder RO, Leff SE, Palmiter RD. Viral gene delivery selectively restores feeding and prevents lethality of dopamine-deficient mice. Neuron. 1999;22(1):167–78.
Heusner CL, Hnasko TS, Szczypka MS, Liu Y, During MJ, Palmiter RD. Viral restoration of dopamine to the nucleus accumbens is sufficient to induce a locomotor response to amphetamine. Brain Res. 2003;980(2):266–74.
Ranaldi R, Pocock D, Zereik R, Wise RA. Dopamine fluctuations in the nucleus accumbens during maintenance, extinction, and reinstatement of intravenous D-amphetamine self- administration. J Neurosci. 1999;19(10):4102–9.
Wise RA, Newton P, Leeb K, Burnette B, Pocock P, Justice JB. Fluctuations in nucleus accumbens dopamine concentration during intravenous cocaine self-administration in rats. Psychopharmacology. 1995;120:10–20.
Wise RA, Leone P, Rivest R, Leeb K. Elevations of nucleus accumbens dopamine and DOPAC levels during intravenous heroin self-administration. Synapse. 1995;21(2):140–8.
Wise RA. Cognitive factors in addiction and nucleus accumbens function: some hints from rodent models. Psychobiology. 1999;27:300–10.
Hebb DO. Drives and the C.N.S. (conceptual nervous system). Psychol Rev. 1955;62:243–54.
Malmo RB. Activation: a neuropsychological dimension. Psychol Rev. 1959;66:367–86.
Wheeler RA, Twining RC, Jones JL, Slater JM, Grigson PS, Carelli RM. Behavioral and electrophysiological indices of negative affect predict cocaine self-administration. Neuron. 2008;57(5):774–85.
Wheeler RA, Aragona BJ, Fuhrmann KA, Jones JL, Day JJ, Cacciapaglia F, et al. Cocaine cues drive opposing context-dependent shifts in reward processing and emotional state. Biol Psychiatry. 2011;69(11):1067–74.
Wheeler DS, Robble MA, Hebron EM, Dupont MJ, Ebben AL, Wheeler RA. Drug predictive cues activate aversion-sensitive striatal neurons that encode drug seeking. J Neurosci. 2015;35(18):7215–25.
Wise RA. Catecholamine theories of reward: a critical review. Brain Res. 1978;152(2):215–47.
Fibiger HC. Drugs and reinforcement mechanisms: a critical review of the catecholamine theory. Ann Rev Pharmacol Toxicol. 1978;18:37–56.
Wise RA. Dopamine and reward: the anhedonia hypothesis 30 years on. Neurotox Res. 2008;14:169–83.
Pickens R, Harris WC. Self-administration of d-amphetamine by rats. Psychopharmacologia. 1968;12:158–63.
Crow TJ. A map of the rat mesencephalon for electrical self-stimulation. Brain Res. 1972;36:265–73.
Lippa AS, Antelman SM, Fisher AE, Canfield DR. Neurochemical mediation of reward: a significant role for dopamine. Pharmacol Biochem Behav. 1973;1:23–8.
Yokel RA, Wise RA. Increased lever pressing for amphetamine after pimozide in rats: implications for a dopamine theory of reward. Science. 1975;187:547–9.
Fouriezos G, Wise RA. Pimozide-induced extinction of intracranial self-stimulation: response patterns rule out motor or performance deficits. Brain Res. 1976;103(2):377–80.
Wasserman EM, Gomita Y, Gallistel CR. Pimozide blocks reinforcement but not priming from MFB stimulation in the rat. Pharmacol Biochem Behav. 1982;17:783–7.
Ettenberg A, Camp CH. Haloperidol induces a partial reinforcement extinction effect in rats: implications for a dopamine involvement in food reward. Pharmacol Biochem Behav. 1986;25:813–21.
Salamone JD, Cousins MS, Bucher S. Anhedonia or anergia? Effects of haloperidol and nucleus accumbens dopamine depletion on instrumental response selection in a T-maze cost/benefit procedure. Behav Brain Res. 1994;65:221–9.
Ikemoto S, Panksepp J. Dissociations between appetitive and consummatory responses by pharmacological manipulations of reward-relevant brain regions. Behav Neurosci. 1996;110(2):331–45.
Wyvell CL, Berridge KC. Intra-accumbens amphetamine increases the conditioned incentive salience of sucrose reward: enhancement of reward “wanting” without enhanced “liking” or response reinforcement. J Neurosci. 2000;20:8122–30.
Wise RA. The neurobiology of craving: implications for the understanding and treatment of addiction. J Abnorm Psychol. 1988;97(2):118–32.
Di Chiara G, Imperato A. Drugs abused by humans preferentially increase synaptic dopamine concentrations in the mesolimbic system of freely moving rats. PNAS. 1988;85:5274–8.
Yokel RA, Wise RA. Attenuation of intravenous amphetamine reinforcement by central dopamine blockade in rats. Psychopharmacology. 1976;48:311–8.
de Wit H, Wise RA. Blockade of cocaine reinforcement in rats with the dopamine receptor blocker pimozide, but not with the noradrenergic blockers phentolamine or phenoxybenzamine. Can J Psychol. 1977;31(4):195–203.
Article PubMed Google Scholar
Roberts DCS, Koob GF, Klonoff P, Fibiger HC. Extinction and recovery of cocaine self-administration following 6-OHDA lesions of the nucleus accumbens. Pharmacol Biochem Behav. 1980;12:781–7.
Roberts DCS, Corcoran ME, Fibiger HC. On the role of ascending catecholaminergic systems in intravenous self-administration of cocaine. Pharmacol Biochem Behav. 1977;6:615–20.
Kombian SB, Malenka RC. Simultaneous LTP of non-NMDA- and LTD of NMDA-receptor-mediated responses in the nucleus accumbens. Nature. 1994;368:242–6.
Nishioku T, Shimazoe T, Yamamoto Y, Nakanishi H, Watanabe S. Expression of long-term potentiation of the striatum in methamphetamine-sensitized rats. Neurosci Lett. 1999;268(2):81–4.
Avchalumov Y, Trenet W, Piña-Crespo J, Mandyam C. SCH23390 reduces methamphetamine self-administration and prevents methamphetamine-induced striatal LTD. Int J Mol Sci. 2020;21(18):6491.
Article CAS PubMed Central Google Scholar
Ettenberg A, Pettit HO, Bloom FE, Koob GF. Heroin and cocaine intravenous self-administration in rats: mediation by separate neural systems. Psychopharmacology. 1982;78:204–9.
Pocock D, Leeb K, Wise RA. Elevations and phasic fluctuations in nucleus accumbens dopamine during IV heroin self-administration. Soc Neurosci Abstr. 1994;20:1234.
Aghajanian GK, Bunney BS. Dopamine “autoreceptors”: pharmacological characterization by microiontophoretic single cell recording studies. Naunyn-Schmiedeberg’s Arch Pharmacol. 1977;297(1):1–7.
Wise RA. Intravenous drug self-administration: a special case of positive reinforcement. In: Bozarth MA, editor. Methods of assessing the reinforcing properties of abused drugs. New York: Springer-Verlag; 1987. p. 117–41.
Bozarth MA, Wise RA. Heroin reward is dependent on a dopaminergic substrate. Life Sci. 1981;29(18):1881–6.
Bechara A, Harrington F, Nader K, van der Kooy D. Neurobiology of motivation: Double dissociation of two motivational mechanisms mediating opiate reward in drug-naive versus drug-dependent rats. Behav Neurosci. 1992;106:798–807.
Grenhoff J, Aston-Jones G, Svensson TH. Nicotinic effects on the firing pattern of midbrain dopamine neurons. Acta Physiologica Scandanavica. 1986;128:351–8.
Doyon WM, Thomas AM, Ostroumov A, Dong Y, Dani JA. Potential substrates for nicotine and alcohol interactions: a focus on the mesocorticolimbic dopamine system. Biochem Pharmacol. 2013;86(8):1181–93.
Di Chiara G. Role of dopamine in the behavioural actions of nicotine related to addiction. Eur J Pharmacol. 2000;393:295–314.
Corrigall WA, Coen KM. Selective dopamine antagonists reduce nicotine self-administration. Psychopharmacology. 1991;104:171–6.
Corrigall WA, Franklin KBJ, Coen KM, Clarke P. The mesolimbic dopaminergic system is implicated in the reinforcing effects of nicotine. Psychopharmacology. 1992;107:285–9.
Picciotto MR, Zoli M, Rimondini R, Lena C, Marubio LM, Pich EM, et al. Acetylcholine receptors containing the beta2 subunit are involved in the reinforcing properties of nicotine. Nature. 1998;391(6663):173–7.
Maskos U, Molles BE, Pons S, Besson M, Guiard BP, Guilloux JP, et al. Nicotine reinforcement and cognition restored by targeted expression of nicotinic receptors. Nature. 2005;436(7047):103–7.
Tolu S, Eddine R, Marti F, David V, Graupner M, Pons S, et al. Co-activation of VTA DA and GABA neurons mediates nicotine reinforcement. Mol Psychiat. 2013;18(3):382–93.
Acquas E, Carboni E, Leone P, Di Chiara G. SCH 23390 blocks drug-conditioned place-preference and place-aversion: anhedonia (lack of reward) or apathy (lack of motivation) after dopamine-receptor blockade? Psychopharmacology. 1989;99(2):151–5.
Huang YY, Kandel DB, Kandel ER, Levine A. Nicotine primes the effect of cocaine on the induction of LTP in the amygdala. Neuropharmacology. 2013;74:126–34.
Chen YH, Hsieh TH, Kuo TT, Kao JH, Ma KH, Huang EY, et al. Release parameters during progressive degeneration of dopamine neurons in a mouse model reveal earlier impairment of spontaneous than forced behaviors. J Neurochem. 2019;150(1):56–73.
Swanson LW, Petrovich GD. What is the amygdala? Trends Neurosci. 1998;21(8):323–31.
Wise RA. Maximization of ethanol intake in the rat. Advances in Exper Med Biol. 1975;59:279–94.
Wise RA. Voluntary ethanol intake in rats following exposure to ethanol on various schedules. Psychopharmacologia. 1973;29(3):203–10.
Samson HH. Initiation of ethanol reinforcement using a sucrose-substitution procedure in food- and water-sated rats. Alcohol Clin Exper Res. 1986;10:436–42.
Sinclair JD, Hyytiä P, Nurmi M. The limited access paradigm: description of one method. Alcohol (Fayetteville, NY). 1992;9(5):441–4.
Simms JA, Steensland P, Medina B, Abernathy KE, Chandler LJ, Wise R, et al. Intermittent access to 20% ethanol induces high ethanol consumption in Long-Evans and Wistar rats. Alcohol Clin Exper Res. 2008;32(10):1816–23.
Goldstein DB, Pal N. Alcohol dependence produced in mice by inhalation of ethanol: grading the withdrawal reaction. Science. 1971;172:288–90.
Macey DJ, Schulteis G, Heinrichs SC, Koob GF. Time-dependent quantifiable withdrawal from ethanol in the rat: effect of method of dependence induction. Alcohol (Fayetteville, NY). 1996;13(2):163–70.
Hopf FW, Martin M, Chen BT, Bowers MS, Mohamedi MM, Bonci A. Withdrawal from intermittent ethanol exposure increases probability of burst firing in VTA neurons in vitro. J Neurophysiol. 2007;98(4):2297–310.
Doyon WM, Ostroumov A, Ontiveros T, Gonzales RA, Dani JA. Ethanol produces multiple electrophysiological effects on ventral tegmental area neurons in freely moving rats. Addiction Biol. 2021;26(2):e12899.
Brodie MS, Shefner SA, Dunwiddie TV. Ethanol increases the firing rate of dopamine neurons of the rat ventral tegmental area in vitro. Brain Res. 1990;508(1):65–9.
Rassnick S, Pulvirenti L, Koob GF. Oral ethanol self-administration in rats is reduced by the administration of dopamine and glutamate receptor antagonists into the nucleus accumbens. Psychopharmacology. 1992;109:92–8.
Samson HH, Hodge CW, Tolliver GA, Haraguchi M. Effect of dopamine agonists and antagonists on ethanol-reinforced behavior: the involvement of the nucleus accumbens. Brain Res Bull. 1993;30:133–41.
Ting AKR, Dockstader C, Heinmiller A, Grieder T, van der Kooy D. GABA(A) receptors mediate the opposing roles of dopamine and the tegmental pedunculopontine nucleus in the motivational effects of ethanol. Eur J Neurosci. 2009;29(6):1235–44.
Laviolette SR, van der Kooy D. GABA(A) receptors in the ventral tegmental area control bidirectional reward signalling between dopaminergic and non-dopaminergic neural motivational systems. Eur J Neurosci. 2001;13(5):1009–15.
Tanda G, Pontieri FE, Di Chiara G. Cannabinoid and heroin activataion of mesolimbic dopamine ransmission by a common µ 1 opioid receptor mechanism. Science. 1997;276:2048–50.
Justinova Z, Tanda G, Redhi GH, Goldberg SR. Self-administration of delta9-tetrahydrocannabinol (THC) by drug naive squirrel monkeys. Psychopharmacology. 2003;169(2):135–40.
Chen JP, Paredes W, Li J, Smith D, Lowinson J, Gardner EL. Delta 9-tetrahydrocannabinol produces naloxone-blockable enhancement of presynaptic basal dopamine efflux in nucleus accumbens of conscious, freely-moving rats as measured by intracerebral microdialysis. Psychopharmacology. 1990;102(2):156–62.
Lupica CR, Riegel AC. Endocannabinoid release from midbrain dopamine neurons: a potential substrate for cannabinoid receptor antagonist treatment of addiction. Neuropharmacology. 2005;48(8):1105–16.
Oleson EB, Cachope R, Fitoussi A, Tsutsui K, Wu S, Gallegos JA, et al. Cannabinoid receptor activation shifts temporally engendered patterns of dopamine release. Neuropsychopharmacology. 2014;39(6):1441–52.
Bossong MG, van Berckel BN, Boellaard R, Zuurman L, Schuit RC, Windhorst AD, et al. Delta 9-tetrahydrocannabinol induces dopamine release in the human striatum. Neuropsychopharmacology. 2009;34(3):759–66.
Mansbach RS, Nicholson KL, Martin BR, Balster RL. Failure of Delta(9)-tetrahydrocannabinol and CP 55,940 to maintain intravenous self-administration under a fixed-interval schedule in rhesus monkeys. Behav Pharmacol. 1994;5(2):219–25.
Wakeford AGP, Wetzell BB, Pomfrey RL, Clasen MM, Taylor WW, Hempel BJ, et al. The effects of cannabidiol (CBD) on ∆ 9 -tetrahydrocannabinol (THC) self-administration in male and female Long-Evans rats. Exper Clin Psychopharmacol. 2017;25(4):242–8.
Freels TG, Baxter-Potter LN, Lugo JM, Glodosky NC, Wright HR, Baglot SL, et al. Vaporized cannabis extracts have reinforcing properties and support conditioned drug-seeking behavior in rats. J Neurosci. 2020;40(9):1897–908.
Smoker MP, Mackie K, Lapish CC, Boehm SL 2nd. Self-administration of edible Δ(9)-tetrahydrocannabinol and associated behavioral effects in mice. Drug Alcohol Depend. 2019;199:106–15.
Jordan CJ, Xi ZX. Progress in brain cannabinoid CB(2) receptor research: from genes to behavior. Neurosci Biobehav Rev. 2019;98:208–20.
Mateo Y, Johnson KA, Covey DP, Atwood BK, Wang HL, Zhang S, et al. Endocannabinoid actions on cortical terminals orchestrate local modulation of dopamine release in the nucleus accumbens. Neuron. 2017;96(5):1112-26.e5.
Davis MI, Crittenden JR, Feng AY, Kupferschmidt DA, Naydenov A, Stella N, et al. The cannabinoid-1 receptor is abundantly expressed in striatal striosomes and striosome-dendron bouquets of the substantia nigra. PLoS ONE. 2018;13(2):e0191436.
Zangen A, Solinas M, Ikemoto S, Goldberg SR, Wise RA. Two brain sites for cannabinoid reward. J Neurosci. 2006;26(18):4901–7.
Deneau G, Yanagita T, Seevers MH. Self-administration of psychoactive substances by the monkey. Psychopharmacologia. 1969;16:30–48.
Pickens R, Muchow D, DeNoble V. Methohexital-reinforced responding in rats: effects of fixed ratio size and injection dose. J Pharmacol Exp Ther. 1981;216(2):205–9.
Griffiths RR, Lukas SE, Bradford LE, Brady JV, Snell JD. Self-injection of barbiturates and benzodiazepines in baboons. Psychopharmacology. 1981;75:101–9.
Szostak C, Finlay JM, Fibiger HC. Intravenous self-administration of the short-acting benzodiazepine midazolam in the rat. Neuropharmacology. 1987;26:1673–6.
Ikemoto S, Kohl RR, McBride WJ. GABA(A) receptor blockade in the anterior ventral tegmental area increases extracellular levels of dopamine in the nucleus accumbens of rats. J Neurochem. 1997;69:137–43.
Ikemoto S, Murphy JM, McBride WJ. Regional differences within the rat ventral tegmental area for muscimol self-infusions. Pharmacol Biochem Behav. 1998;61:87–92.
Heikkinen AE, Möykkynen TP, Korpi ER. Long-lasting modulation of glutamatergic transmission in VTA dopamine neurons after a single dose of benzodiazepine agonists. Neuropsychopharmacology. 2009;34(2):290–8.
Tan KR, Brown M, Labouèbe G, Yvon C, Creton C, Fritschy JM, et al. Neural bases for addictive properties of benzodiazepines. Nature. 2010;463(7282):769–74.
Soden ME, Chung AS, Cuevas B, Resnick JM, Awatramani R, Zweifel LS. Anatomic resolution of neurotransmitter-specific projections to the VTA reveals diversity of GABAergic inputs. Nat Neurosci. 2020;23:968.
Vashchinkina E, Panhelainen A, Aitta-Aho T, Korpi ER. GABAA receptor drugs and neuronal plasticity in reward and aversion: focus on the ventral tegmental area. Front Pharmacol. 2014;5:256.
Di Chiara G, Imperato A. Preferential stimulation of dopamine release in the nucleus accumbens by opiates, alcohol, and barbiturates: studies with transcerebral dialysis in freely moving rats. Ann NY Acad Sci. 1986;473:367–81.
O’Brien DP, White FJ. Inhibition of non-dopamine cells in the ventral tegmental area by benzodiazepines: relationship to A10 dopamine cell activity. Eur J Pharmacol. 1987;142:343–54.
Schelp SA, Brodnik ZD, Rakowski DR, Pultorak KJ, Sambells AT, Espana RA, et al. Diazepam concurrently increases the frequency and decreases the amplitude of transient dopamine release events in the nucleus accumbens. J Pharmacol Exp Ther. 2018;364(1):145–55.
van der Kooij MA, Hollis F, Lozano L, Zalachoras I, Abad S, Zanoletti O, et al. Diazepam actions in the VTA enhance social dominance and mitochondrial function in the nucleus accumbens by activation of dopamine D1 receptors. Mol Psychiatry. 2018;23(3):569–78.
Horger BA, Wellman PJ, Morien A, Davies BT, Schenk S. Caffeine exposure sensitizes rats to the reinforcing effects of cocaine. NeuroReport. 1991;2:53–6.
Bradley CA, Palmatier MI. Intravenous and oral caffeine self-administration in rats. Drug Alcohol Depend. 2019;203:72–82.
Myers KP, Izbicki EV. Reinforcing and aversive effects of caffeine measured by flavor preference conditioning in caffeine-naive and caffeine-acclimated rats. Physiol Behav. 2006;88(4–5):585–96.
Yee M, Maal-Bared G, Ting AKR, Chwalek M, Mackay-Clackett I, Bergamini M, et al. Segregation of caffeine reward and aversion in the rat nucleus accumbens shell versus core. Eur J Neurosci. 2020;52(3):3074–86.
Galvalisi M, Prieto JP, Martinez M, Abin-Carriquiry JA, Scorza C. Caffeine induces a stimulant effect and increases dopamine release in the nucleus accumbens shell through the pulmonary inhalation route of administration in rats. Neurotox Res. 2017;31(1):90–8.
Volkow ND, Wang GJ, Logan J, Alexoff D, Fowler JS, Thanos PK, et al. Caffeine increases striatal dopamine D2/D3 receptor availability in the human brain. Trans Psychiat. 2015;5:e549.
Zheng X, Takatsu S, Wang H, Hasegawa H. Acute intraperitoneal injection of caffeine improves endurance exercise performance in association with increasing brain dopamine release during exercise. Pharmacol Biochem Behav. 2014;122:136–43.
Holstein SE, Barkell GA, Young MR. Caffeine increases alcohol self-administration, an effect that is independent of dopamine D(2) receptor function. Alcohol (Fayetteville, NY). 2021;91:61–73.
Download references
Acknowledgements
Author information, authors and affiliations.
Intramural Research Program, National Institute on Drug Abuse, 250 Mason Lord Drive, Baltimore, MD, USA
Roy A. Wise
Behavior Genetics Laboratory, McLean Hospital, Harvard Medical School, 115 Mill Street, Belmont, MA, 02478, USA
Division of Alcohol, Drugs and Addiction, Department of Psychiatry, Harvard Medical School, McLean Hospital, 115 Mill Street, Belmont, MA, 02478, USA
Chloe J. Jordan
You can also search for this author in PubMed Google Scholar
Contributions
RAW and CJJ each read the cited articles, planned the review, wrote the review, responded to reviewer comments, and modified the references for citation style. All authors read and approved the final manuscript.
Corresponding author
Correspondence to Roy A. Wise .
Ethics declarations
Ethics approval and consent to participate, consent for publication, competing interests.
The authors declare that they have no competing interests.
Additional information
Publisher's note.
Springer Nature remains neutral with regard to jurisdictional claims in published maps and institutional affiliations.
Rights and permissions
Open Access This article is licensed under a Creative Commons Attribution 4.0 International License, which permits use, sharing, adaptation, distribution and reproduction in any medium or format, as long as you give appropriate credit to the original author(s) and the source, provide a link to the Creative Commons licence, and indicate if changes were made. The images or other third party material in this article are included in the article's Creative Commons licence, unless indicated otherwise in a credit line to the material. If material is not included in the article's Creative Commons licence and your intended use is not permitted by statutory regulation or exceeds the permitted use, you will need to obtain permission directly from the copyright holder. To view a copy of this licence, visit http://creativecommons.org/licenses/by/4.0/ . The Creative Commons Public Domain Dedication waiver ( http://creativecommons.org/publicdomain/zero/1.0/ ) applies to the data made available in this article, unless otherwise stated in a credit line to the data.
Reprints and permissions
About this article
Cite this article.
Wise, R.A., Jordan, C.J. Dopamine, behavior, and addiction. J Biomed Sci 28 , 83 (2021). https://doi.org/10.1186/s12929-021-00779-7
Download citation
Received : 11 May 2021
Accepted : 19 November 2021
Published : 02 December 2021
DOI : https://doi.org/10.1186/s12929-021-00779-7
Share this article
Anyone you share the following link with will be able to read this content:
Sorry, a shareable link is not currently available for this article.
Provided by the Springer Nature SharedIt content-sharing initiative
Journal of Biomedical Science
ISSN: 1423-0127
- Submission enquiries: Access here and click Contact Us
- General enquiries: [email protected]
HYPOTHESIS AND THEORY article
The dopamine hypothesis of drug addiction and its potential therapeutic value.

- ‘G. Minardi’ Cognitive Neuroscience Laboratory, Department of Drug Sciences, University of Sassari, Sassari, Italy
Dopamine (DA) transmission is deeply affected by drugs of abuse, and alterations in DA function are involved in the various phases of drug addiction and potentially exploitable therapeutically. In particular, basic studies have documented a reduction in the electrophysiological activity of DA neurons in alcohol, opiate, cannabinoid, and other drug-dependent rats. Further, DA release in the Nucleus accumbens (Nacc) is decreased in virtually all drug-dependent rodents. In parallel, these studies are supported by increments in intracranial self stimulation (ICSS) thresholds during withdrawal from alcohol, nicotine, opiates, and other drugs of abuse, thereby suggesting a hypofunction of the neural substrate of ICSS. Accordingly, morphological evaluations fed into realistic computational analysis of the medium spiny neuron of the Nacc, post-synaptic counterpart of DA terminals, show profound changes in structure and function of the entire mesolimbic system. In line with these findings, human imaging studies have shown a reduction of dopamine receptors accompanied by a lesser release of endogenous DA in the ventral striatum of cocaine, heroin, and alcohol-dependent subjects, thereby offering visual proof of the “ dopamine-impoverished” addicted human brain. The lasting reduction in physiological activity of the DA system leads to the idea that an increment in its activity, to restore pre-drug levels, may yield significant clinical improvements (reduction of craving, relapse, and drug-seeking/taking). In theory, it may be achieved pharmacologically and/or with novel interventions such as transcranial magnetic stimulation (TMS). Its anatomo-physiological rationale as a possible therapeutic aid in alcoholics and other addicts will be described and proposed as a theoretical framework to be subjected to experimental testing in human addicts.
Drug addiction is a brain disease that produces profound modifications in human behavior ( Hyman, 2007 ; Koob and Volkow, 2010 ), with important negative consequences at various levels, including personal health, employment, family interactions, and society in general ( Chandler et al., 2009 ). Therapeutic possibilities for this devastating illness are, with some rare exceptions, limited to pharmacologic treatments that are largely unsatisfactory ( Koob et al., 2009 ; Leggio et al., 2010 ; Swift, 2010 ). From here the necessity to develop new therapeutic hypothesis/interventions independent from those commonly employed.
Transcranial magnetic stimulation (TMS), through generation of an electromagnetic field capable of crossing painlessly through the skull and influencing the underlying brain matter, appears to be a promising candidate for treating addictive behaviors ( Barr et al., 2008 ; Feil and Zangen, 2010 ) and other brain diseases ( Kobayashi and Pascual-Leone, 2003 ). In brief, this relatively new method allows modulation of discrete brain areas of the awake and conscious subject under study. The pulsatile electromagnetic field generated around the coil crosses the skull and is capable of directly exciting/inhibiting neurons in the underlying cortices ( Padberg and George, 2009 ). Commonly employed as a research tool, TMS is recently affirming its role as a potential therapeutic means approved by the Food and Drug Administration for brain pathologies such as drug-resistant major depression, bipolar syndrome, and negative symptoms of schizophrenia. In the drug addiction field, the therapeutic potential of TMS has been tested in nicotine-dependent subjects ( Lang et al., 2008 ; Amiaz et al., 2009 ), cocaine addicts ( Boutros et al., 2001 , 2005 ; Sundaresan et al., 2007 ; Politi et al., 2008 ), and alcoholics ( Conte et al., 2008 ; Mishra et al., 2010 ). Although the results are certainly encouraging, the disparity of clinical outcomes evaluated in different studies and diversity of pattern/site/methodology of stimulation precludes direct comparisons and hampers firm conclusions. However, in those studies in which craving was measured ( Politi et al., 2008 ; Amiaz et al., 2009 ; Mishra et al., 2010 ) significant reductions have been found, thus encouraging further experimental scrutiny. At present, we are evaluating anti-craving and alcohol-intake efficacy of TMS in alcoholics (Addolorato et al., in preparation), short and long-term cocaine intake in treatment-seeking cocaine addicts (Pedetti et al., in preparation), and money/cocaine choice in a lab study of cocaine addicts non-seeking treatment (Martinez et al., in preparation). Nevertheless, the brain site(s) to be stimulated/inhibited and the stimulation parameters (i.e., frequency of stimulation, number of session etc.,) are matters of intense debate and an appropriate rationale is needed.
Dopamine as a Possible Therapeutic Target
The role of central DA systems in the acute effects of drugs of abuse was recognized long ago ( Wise, 1980 , 1987 ; Di Chiara and Imperato, 1988 ). Even before ( Ahlenius et al., 1973 ), attempts were made to prevent human alcohol-induced euphoria through administration of the DA synthesis inhibitor alpha methyl-para-tyrosine. Although theoretically ineccepibile, this approach (reduction of drug-induced DA increments to prevent abuse) is unlikely to have a practical validity as any compound with DA antagonistic (i.e., neuroleptics) properties is known to be aversive in humans. On the other hand, widely documented experimental evidence suggests that the mesolimbic dopamine system is “hypofunctional” in the addicted brain ( Melis et al., 2005 ). In brief, the hypothesis contends that decreased DA function in addicted subjects results in a decreased interest to non-drug-related stimuli and increased sensitivity to the drug of choice ( Melis et al., 2005 ), leading to propose that restoring DA function might be therapeutically advantageous.
Alcohol-dependent (in the present context the term “dependent,” when referred to a non-human experimental subject, indicates a condition in which the subject has shown unequivocally a proof of dependency, i.e., somatic signs of withdrawal) rats show a profound reduction of spontaneous firing rate and burst firing of antidromically identified Nucleus accumbens (Nacc)-projecting ventral tegmental area (VTA) DA-containing neurons in rats ( Diana et al., 1993 ) and mice ( Bailey et al., 2001 ) resulting in a concomitant reduction of microdialysate DA in the Nacc ( Rossetti et al., 1992 ; Diana et al., 1993 ; Barak et al., 2011 ). Further, the reduced dopaminergic activity outlasts somatic signs of alcohol-withdrawal ( Diana et al., 1996 , 2003 ) thereby suggesting a role for DA in the lasting consequences of alcohol dependence while excluding the possibility of a DA role in somatic aspects of withdrawal. Further, original (pre-dependence) DA levels in the Nacc are restored when ethanol is self ( Weiss et al., 1996 ) and/or passively administered ( Diana et al., 1993 , 1996 ). These observations are paralleled by intracranial self stimulation (ICSS) studies showing that ethanol-withdrawn rats are capable of maintaining the ICSS behavior provided that the stimulus current intensity is increased ( Schulteis et al., 1995 ). This important observation strongly indicates that the neural substrate responsible for maintaining the ICSS behavior is hyperpolarized, or more refractory, in the alcohol-dependent subject as compared with its control. Since the neural substrate of ICSS involves DA axons ( Yeomans, 1989 ; Yeomans et al., 1993 ) near the stimulating electrode, the results are complementary to those reported above and well support a deficitary function of DA neurons. In addition, the perseverance of the reduction in DA activity (beyond resolution of somatic signs of withdrawal) has also been documented in morphine-dependent rats ( Diana et al., 1999 ), while a dichotomy between DA function and somatic withdrawal has been observed in cannabinoid–withdrawn rats ( Diana et al., 1998 ). Similarly, conditioned heroin withdrawal decreases reward sensitivity ( Kenny et al., 2006 ) which persists well beyond the initial phase of withdrawal. These findings, observed across different addicting compounds and experimental conditions, suggest that DA hypofunction persists over time, although reverting to “normality” ( Diana et al., 1999 , 2006 ), eventually with species-specific time course.
In addition to basic literature, reports in humans are also supportive of a compromised role of DA transmission in alcoholics. While alcohol increases DA release in healthy subjects ( Boileau et al., 2003 ) with some gender differences ( Urban et al., 2010 ), a reduced number of DA receptors has been observed ( Volkow et al., 1996 ; Martinez et al., 2005 ) in alcoholics that appears to be accompanied by a blunted DA release ( Martinez et al., 2005 , 2007 ; Volkow et al., 2007 ). While the reduced number of DA receptors could be, at first sight, be viewed as suggesting an increased DA release, it should be noted that by administering the DA inhibitor alpha methyl-para-tyrosine, Martinez et al. (2009) were able to exclude this possibility. Indeed, while healthy controls do show an increased raclopride binding after acute alpha methyl-para-tyrosine administration, cocaine-dependent subjects do not (or to a significantly lesser extent; Martinez et al., 2009 ). Similar results were obtained with the dopamine releasing agent methylphenidate ( Volkow et al., 2007 ) and amphetamine ( Martinez et al., 2005 ) in alcoholics. Notably, artificially increasing the brain levels of DAD2 receptors, using a replication-deficient adenoviral vector containing the rat cDNA insert for DAD2 into the Nacc, reduces alcohol intake in spontaneously drinking rats, thereby offering the counterproof that a potentiation of DA transmission may have beneficial effects on alcohol-seeking and alcohol-taking, in experimental models ( Thanos et al., 2001 , 2004 ). In line with this conclusion, a spontaneous high number of DA D2 receptors has been shown to have a protective role in non-alcoholic members of alcoholic families ( Volkow et al., 2006 ). These findings further support the notion that the number of DA receptors (and consequently DA transmission) inversely correlates with alcohol drinking.
These observations may suggest that “ boosting” DA neurons to produce more available DA in the synaptic cleft could alleviate some of the symptoms of addiction and alcoholism, thereby acquiring a therapeutic character. In theory, this could be achieved by two different strategies: (1) DA-potentiating drugs and (2) TMS. Both possibilities are discussed below.
Dopamine-Potentiating Drugs
Although medications that increase DA activity could be effective in treating alcohol abuse disorders, conflicting results have been produced ( Swift, 2010 ). For example, it was suggested that the DA agonist bromocriptine reduced drinking in alcoholics ( Lawford et al., 1995 ), but a randomized, double-blind, placebo-controlled study using a long-acting injectable bromocriptine preparation in 366 alcoholic-dependent individuals did not find difference in alcohol relapse between medication and placebo ( Naranjo et al., 1997 ). Another example is the stimulant medication modafinil (DA indirect agonist), found to improve cognition in 40 alcoholics with organic brain syndrome, but effects on drinking could not be measured ( Saletu et al., 1990 ). However, modafinil reduced cocaine use in a placebo-controlled study with 62 cocaine-dependent individuals ( Dackis and O’Brien, 2005 ), while another trial did not find differences between modafinil and placebo tested for methamphetamine users ( Shearer et al., 2010 ). While evidence for the use of DA agonists as a treatment for alcohol and/or substance use disorders is inconclusive ( Swift, 2010 ), there has been a revived interest for these drugs, possibly because adequate neurobiological rationale ( Melis et al., 2005 ) is now available. For example, aripiprazole ( Semba et al., 1995 ; Burris et al., 2002 ; Shapiro et al., 2003 ) a partial DA agonist which in principle should antagonize DA when tone is high, whereas should increase DA transmission when basic tone is low, represents a proposed treatment for alcohol abuse disorders ( Kenna et al., 2009 ). Human laboratory alcohol studies have shown that aripiprazole reduces drinking ( Kranzler et al., 2008 ), especially in the more impulsive alcoholic ( Voronin et al., 2008 ). An fMRI study demonstrated that aripiprazole significantly attenuates neural activity in the ventral striatum in response to alcohol cues ( Myrick et al., 2010 ) thereby suggesting a therapeutic potential for cue-induced relapse. Further, a 12-week, double-blind, placebo-controlled treatment study with 295 alcohol-dependent individuals found that aripiprazole initially decreased heavy drinking days compared to placebo, but this significant effect was not present when the target dose of 30 mg was reached ( Anton et al., 2008 ). This trial also showed greater side-effects and greater study discontinuation in the aripiprazole arm, as compared to placebo ( Anton et al., 2008 ). Interestingly, an open-label study of aripiprazole ( Martinotti et al., 2009 ) and a recent human laboratory study ( Kenna et al., 2009 ) suggests that lower doses of aripiprazole (5–15 mg per day) may be better tolerated and still reduce drinking with effects on relapse comparable to those obtained with the opiate antagonist naltrexone ( Martinotti et al., 2009 ).
In summary, dopamine plays a key role in the addiction process, but significant side-effects have limited the use of medications that work directly on the dopaminergic system. The use of DA partial agonists with lower side effect profiles, and appropriate dosing represent important directions for future research in this area.
Transcranial Magnetic Stimulation
Increasing DA tone with appropriate pharmacological tools, is only one of the possible strategies. Endogenous activity of DA-containing neurons can be augmented with non-pharmacological tools such as TMS ( Strafella et al., 2001 ) thereby providing, in principle, an adjunct to the “therapeutic arsenal” against addiction, endowed with lesser systemic side-effects and limited contraindications. However, while the rationale is “neurochemical” for pharmacological agents (neurotransmitter receptors, brain area etc.,) , it must be anatomically based for TMS. Being that DA-containing neurons are located deeply in the brainstem (thereby making the neurons inaccessible to direct TMS stimuli) it becomes unavoidable to reach them indirectly through neurons located elsewhere in the brain. The dorsolateral prefrontal cortex (DLPfcx) by projecting monosynaptically to the rat ( Carr and Sesack, 2000 ) and primate ( Frankle et al., 2006 ) VTA may serve this function. These studies show a projection from the PFC to midbrain DA neurons, terminating both within the SN proper as well as in the VTA. They arise from a broad region of the PFC, including the DLPfcx, cingulate, and orbital cortices. Indeed, these pyramidal neurons (Figure 1 ) could be exploited as the primary target of the TMS stimulus and their increased activity to produce, ultimately, an enhancement in DA availability in the synaptic cleft in the Nacc. Schematically, the hypothesized circuit (Figure 2 ) would be the following: TMS → DLPfcx → VTA → DA increase in forebrain projection site (i.e., Nacc). In this context, it is imperative to employ stimulation parameters consonant with the physiological activity of the system under study to restore pre-drug DA levels. For instance, it has been shown that DLPfcx stimulation produces bursts in rat DA neurons ( Gariano and Groves, 1988 ; Murase et al., 1993 ), highlighting the importance of stimulation parameters. Indeed, burst firing is more efficacious than single spiking (of identical frequency but evenly spaced action potentials) in inducing DA release in terminal areas ( Gonon, 1988 ; Manley et al., 1992 ). Consistently, the role of DLPfcx in regulating basal DA activity through the VTA has been reported ( Taber et al., 1995 ; Karreman and Moghaddam, 1996 ).
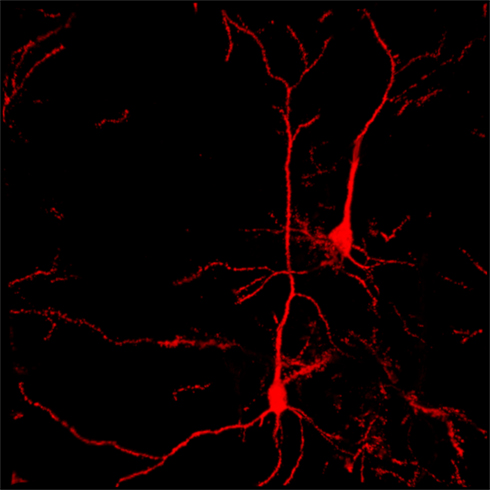
Figure 1. Confocal reconstruction of Golgi-stained pyramidal neurons from DLPfcx obtained by a projection of 55 scans for a depth of 27.5 μm in the z -axis . DLPfxc may represent a useful target for rTMS stimulation.
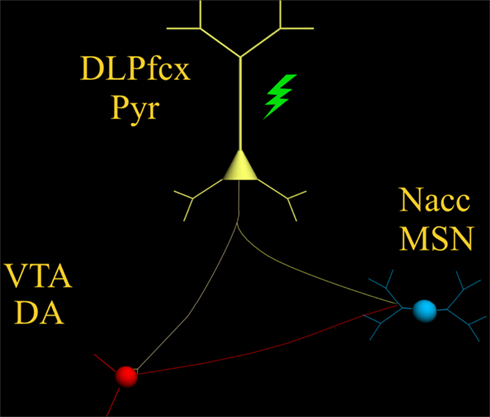
Figure 2. The scheme illustrates the proposed circuit to be activated by the TMS stimulus (green) which, by activating the pyramidal neuron (yellow) with its neurotransmitter glutamate, would excite: (1) DA-containing neurons of the VTA (red) and (2) MSN of the Nacc (blue) .
Among the various factors that are likely to influence its efficacy, the importance of the baseline cortical activation state on the impact of TMS is fundamental ( Silvanto and Pascual-Leone, 2008 ). This state-dependency is key as the neural impact of any external stimulus represents an interaction with the ongoing brain activity at the time of stimulation. The effects of any external stimulus are therefore not only determined by the properties of that stimulus but also by the activation state of the brain. Accordingly, it has been shown that baseline cortical activity determines whether TMS hampers or hastens behavior ( Silvanto et al., 2008 ). The state-dependency principle described above would also apply to the state of the DA system. The hypodopaminergic state ( Melis et al., 2005 ) should then “amplify” the effect of TMS as compared with that expected in a normo-functioning DA system.
The responsivity of the neuron(s) to electrical and synaptic stimuli is strictly dependent on its morphological features, which in turn, are deeply modified by drugs of abuse ( Robinson and Kolb, 2004 ) and withdrawal from chronic treatment with opiates ( Sklair-Tavron et al., 1996 ; Spiga et al., 2003 , 2005 ), cannabis derivatives/analogs ( Spiga et al., 2010 ), and psychostimulants ( Robinson and Kolb, 1997 ) have been shown to produce reductions in DA cells size ( Sklair-Tavron et al., 1996 ; Spiga et al., 2003 ), paralleled by persistently ( Diana et al., 2006 ) altered patterns of synaptic connectivity, and spines density in the Nacc and Pfcx ( Robinson and Kolb, 1997 ). These architectural changes would be expected to modify intrinsic spontaneous action potential generating capacity and responsiveness of the system to the TMS stimuli. Accordingly, realistic computational analysis ( Spiga et al., 2010 ) of cannabis-dependent rats, generated by input of experimentally verified morphometrical and electrophysiological properties, predicts a lower action potential generation of Nacc medium spiny neuron (MSN). These results suggest that MSN, of cannabis-dependent rats are likewise hypofunctional. Considering that the main drive of these neurons is cortical glutamate (Glu; see discussion in Spiga et al., 2010 , and references therein; Kalivas and Hu, 2006 ) it raises the possibility of a reduction of Glu as a causal factor. This finding, thus offers the additional possibility that stimulation of these units through TMS may be advantageous in restoring pre-drug physiological activity. Indeed, TMS cortical application should increase the activity of glutamate-containing cortico-fugal fibers monosynaptically impinging upon the spine’s heads of Nacc MSN ( Groenewegen et al., 1991 ). Considering the fundamental role Glu plays in synaptic plasticity ( Russo et al., 2010 ), its role could also be exploited in LTP-like stimulation parameters, ultimately aimed at producing lasting and enduring restoration of original physiological activity. These characteristics must be considered and coherently inserted into a framework to obtain optimal stimulation parameters. In vivo recordings of VTA-projecting DLPfcx neurons do fire spontaneously around 4–6 Hz ( Pistis et al., 2001 ) and a TMS stimulus frequency of 10 Hz could be a reasonable frequency to obtain a significant increase in VTA-projecting neurons aimed at stimulating the “ deficient” dopamine system and its post-synaptic counterpart (i.e., MSN of the Nacc).
Another factor to be considered is that all previous studies (see above) applied the TMS stimulus monolaterally, yet obtaining a reduction of alcohol craving ( Mishra et al., 2010 ). While alcohol intake was not measured, and contralateral effects cannot be excluded a priori , it is possible that application of TMS bilaterally, as in the case of the H-coil ( Feil and Zangen, 2010 ), would yield stronger cortical activation (larger number of fibers activated) with an increased probability of a significant increment of bilateral DA release. It should be noted that unilateral TMS application has already been reported to increase DA release ( Strafella et al., 2001 ) omolaterally in the human striatum, as well as in rodents ( Keck et al., 2002 ; Zangen and Hyodo, 2002 ), and even in morphine-withdrawn rats ( Erhardt et al., 2004 ), thereby supporting the rationale outlined above. Although Strafella et al. (2001) proposed activation of (Glu-containing) cortico-fugal fibers making synaptic contact with DA-containing terminals in the ventral striatum, to explain their results, it should be noted that the existence of axo-axonic contacts has always being questioned based on the lack of appropriate anatomical observations ( Groenewegen et al., 1991 ; Meredith et al., 2008 ).
While many technical details for optimal stimulation parameters need further investigation and optimization, the TMS appears to deserve careful experimental scrutiny as a potential therapeutic tool in alcoholics and other addicts. Indeed, with its nearly absent systemic effects, minimal side-effects, and a low degree of invasiveness, TMS may offer the first opportunity for an efficacious, non-pharmacological, therapeutic tool in alcoholism and other chemical dependencies. If appropriately combined with a solid neurobiological rationale (DA system), it may offer a unique opportunity for developing further the first “ electrophysiological” approach in studying and eventually treating the devastating and widespread brain disease of addiction.
Conflict of Interest Statement
The author declares that the research was conducted in the absence of any commercial or financial relationships that could be construed as a potential conflict of interest.
Acknowledgments
This work was supported, in part, by grants from MIUR (PRIN. N°2004052392) and Dipartimento Politiche Antidroga. The author wish to thank S. Spiga for elaborating iconographic material presented.
Ahlenius, S., Carlsson, A., Engel, J., Svensson, T., and Södersten, P. (1973). Antagonism by alpha methyltyrosine of the ethanol-induced stimulation and euphoria in man. Clin. Pharmacol. Ther. 14, 586–591.
Pubmed Abstract | Pubmed Full Text
Amiaz, R., Levy, D., Vainiger, D., Grunhaus, L., and Zangen, A. (2009). Repeated high-frequency transcranial magnetic stimulation over the dorsolateral prefrontal cortex reduces cigarette craving and consumption. Addiction 104, 653–660.
Pubmed Abstract | Pubmed Full Text | CrossRef Full Text
Anton, R. F., Kranzler, H., Breder, C., Marcus, R. N., Carson, W. H., and Han, J. (2008). A randomized, multicenter, double-blind, placebo-controlled study of the efficacy and safety of aripiprazole for the treatment of alcohol dependence. J. Clin. Psychopharmacol. 28, 5–12.
Bailey, C. P., O’Callaghan, M. J., Croft, A. P., Manley, S. J., and Little, H. J. (2001). Alterations in mesolimbic dopamine function during the abstinence period following chronic ethanol consumption. Neuropharmacology 41, 989–999.
Barak, S., Carnicella, S., Yowell, Q. W., and Ron, D. (2011). Glial cell line-derived neurotrophic factor reverses alcoho-induced allostasis of the mesolimbic dopaminergic system: implications for alcohol reward and seeking. J. Neurosci. 31, 9885–9894.
Barr, M. S., Fitzgerald, P. B., Farzan, F., George, T. P., and Daskalakis, Z. J. (2008). Transcranial magnetic stimulation to understand the pathophysiology and treatment of substance use disorders. Curr. Drug Abuse Rev. 1, 328–339.
Boileau, I., Assaad, J. M., Pihl, R. O., Benkelfat, C., Leyton, M., Diksic, M., Tremblay, R. E., and Dagher, A. (2003). Alcohol promotes dopamine release in the human nucleus accumbens. Synapse 15, 226–231.
CrossRef Full Text
Boutros, N. N., Lisanby, S. H., McClain-Furmanski, D., Oliwa, G., Gooding, D., and Kosten, T. R. (2005). Cortical excitability in cocaine-dependent patients: a replication and extension of TMS findings. J. Psychiatr. Res. 39, 295–302.
Boutros, N. N., Lisanby, S. H., Tokuno, H., Torello, M. W., Campbell, D., Berman, R., Malison, R., Krystal, J. H., and Kosten, T. (2001). Elevated motor threshold in drug-free, cocaine-dependent patients assessed with transcranial magnetic stimulation. Biol. Psychiatry 49, 369–373.
Burris, K. D., Molski, T. F., Xu, C., Ryan, E., Tottori, K., Kikuchi, T., Yocca, F. D., and Molinoff, P. B. (2002). Aripiprazole, a novel antipsychotic, is a high-affinity partial agonist at human dopamine D2 receptors. J. Pharmacol. Exp. Ther. 302, 381–389.
Carr, D. B., and Sesack, S. R. (2000). Projections from the rat prefrontal cortex to the ventral tegmental area: target specificity in the synaptic associations with mesoaccumbens and mesocortical neurons. J. Neurosci. 20, 3864–3873.
Chandler, R. K., Fletcher, B. W., and Volkow, N. D. (2009). Treating drug abuse and addiction in the criminal justice system: improving public health and safety. JAMA 301, 183–190.
Conte, A., Attilia, M. L., Gilio, F., Iacovelli, E., Frasca, V., Bettolo, C. M., Gabriele, M., Giacomelli, E., Prencipe, M., Berardelli, A., Ceccanti, M., and Inghilleri, M. (2008). Acute and chronic effects of ethanol on cortical excitability. Clin. Neurophysiol. 119, 667–674.
Dackis, C., and O’Brien, C. (2005). Neurobiology of addiction: treatment and public policy ramifications. Nat. Neurosci. 8, 1431–1436.
Di Chiara, G., and Imperato, A. (1988). Drugs abused by humans preferentially increase synaptic dopamine concentrations in the mesolimbic system of freely moving rats. Proc. Natl. Acad. Sci. U.S.A. 85, 5274–5278.
Diana, M., Brodie, M., Muntoni, A., Puddu, M. C., Pillolla, G., Steffensen, S., Spiga, S., and Little, H. J. (2003). Enduring effects of chronic ethanol in the CNS: basis for alcoholism. Alcohol. Clin. Exp. Res. 27, 354–361.
Diana, M., Melis, M., Muntoni, A. L., and Gessa, G. L. (1998). Mesolimbic dopaminergic decline after cannabinoid withdrawal. Proc. Natl. Acad. Sci. U.S.A. 18, 10269–10273.
Diana, M., Muntoni, A. L., Pistis, M., Melis, M., and Gessa, G. L. (1999). Lasting reduction in mesolimbic dopamine neuronal activity after morphine withdrawal. Eur. J. Neurosci. 11, 1037–1041.
Diana, M., Pistis, M., Carboni, S., Gessa, G. L., and Rossetti, Z. L. (1993). Profound decrement of mesolimbic dopaminergic neuronal activity during ethanol withdrawal syndrome in rats: electrophysiological and biochemical evidence. Proc. Natl. Acad. Sci. U.S.A. 90, 7966–7969.
Diana, M., Pistis, M., Muntoni, A., and Gessa, G. L. (1996). Mesolimbic dopaminergic reduction outlasts ethanol withdrawal syndrome: Evidence of protracted abstinence. Neuroscience 71, 411–415.
Diana, M., Spiga, S., and Acquas, E. (2006). Persistent and reversible morphine withdrawal-induced morphological changes in the nucleus accumbens. Ann. N. Y. Acad. Sci. 1074, 446–457.
Erhardt, A., Sillaber, I., Welt, T., Müller, M. B., Singewald, N., and Keck, M. E. (2004). Repetitive transcranial magnetic stimulation increases the release of dopamine in the nucleus accumbens shell of morphine-sensitized rats during abstinence. Neuropsychopharmacology 29, 2074–2080.
Feil, J., and Zangen, A. (2010). Brain stimulation in the study and treatment of addiction. Neurosci. Biobehav. Rev. 34, 559–574.
Frankle, W. G., Laruelle, M., and Haber, S. N. (2006). Prefrontal cortical projections to the midbrain in primates: evidence for a sparse connection. Neuropsychopharmacology 31, 1627–1636.
Gariano, R. F., and Groves, P. M. (1988). Burst firing induced in midbrain dopamine neurons by stimulation of the medial prefrontal and anterior cingulate cortices. Brain Res. 462, 194–198.
Gonon, F. (1988). Nonlinear relationship between impulse flow and dopamine released by rat midbrain dopaminergic neurons as studied by in vivo electrochemistry. Neuroscience 24, 19–28.
Groenewegen, H. J., Berendse, H. W., Meredith, G. E., Haber, S. N., Voorn, P., Wolters, J. G., and Lohman, A. H. M. (1991). “Functional anatomy of the ventral, limbic system-innervated striatum,” in The Mesolimbic Dopamine System: From Motivation to Action , eds P. Willner and J. Scheel-Krüger (New York: Wiley), 19–59.
Hyman, S. E. (2007). The neurobiology of addiction: implications for voluntary control of behavior. Am. J. Bioeth. 7, 8–11.
Kalivas, P. W., and Hu, X. T. (2006). Exciting inhibition in psychostimulation addiction. Trends Neurosci. 29, 610–616.
Karreman, M., and Moghaddam, B. (1996). The prefrontal cortex regulates the basal release of dopamine in the limbic striatum: an effect mediated by ventral tegmental area. J. Neurochem. 66, 589–598.
Keck, M. E., Welt, T., Müller, M. B., Erhardt, A., Ohl, F., Toschi, N., Holsboer, F., and Sillaber, I. (2002). Repetitive transcranial magnetic stimulation increases the release of dopamine in the mesolimbic and mesostriatal system. Neuropharmacology 43, 101–109.
Kenna, G. A., Leggio, L., and Swift, R. M. (2009). A safety and tolerability laboratory study of the combination of aripiprazole and topiramate in volunteers who drink alcohol. Hum. Psychopharmacol. 24, 465–472.
Kenny, P. J., Chen, S. A., Kitamura, O., Markou, A., and Koob, G. F. (2006). Conditioned withdrawal drives heroin consumption and decreases reward sensitivity. J. Neurosci. 26, 5894–5900.
Kobayashi, M., and Pascual-Leone, A. (2003). Transcranial magnetic stimulation in neurology. Lancet Neurol. 2, 145–156.
Koob, G. F., Kenneth Lloyd, G., and Mason, B. J. (2009). Development of pharmacotherapies for drug addiction: a Rosetta stone approach. Nat. Rev. Drug Discov. 8, 500–515.
Koob, G. F., and Volkow, N. D. (2010). Neurocircuitry of addiction. Neuropsychopharmacology 35, 217–238.
Kranzler, H. R., Covault, J., Pierucci-Lagha, A., Chan, G., Douglas, K., Arias, A. J., and Oncken, C. (2008). Effects of aripiprazole on subjective and physiological responses to alcohol. Alcohol. Clin. Exp. Res. 32, 573–579.
Lang, N., Hasan, A., Sueske, E., Paulus, W., and Nitsche, M. A. (2008). Cortical hypoexcitability in chronic smokers? A transcranial magnetic stimulation study. Neuropsychopharmacology 33, 2517–2523.
Lawford, B. R., Young, R. M., Rowell, J. A., Qualichefski, J., Fletcher, B. H., Syndulko, K., Ritchie, T., and Noble, E. P. (1995). Bromocriptine in the treatment of alcoholics with the D2 dopamine receptor A1 allele. Nat. Med. 1, 337–341.
Leggio, L., Cardone, S., Ferrulli, A., Kenna, G. A., Diana, M., Swift, R. M., and Addolorato, G. (2010). Turning the clock ahead: potential preclinical and clinical neuropharmacological targets for alcohol dependence. Curr. Pharm. Des. 16, 2159–2181.
Manley, L. D., Kuczenski, R., Segal, D. S., Young, S. J., and Groves, P. M. (1992). Effects of frequency and pattern of medial forebrain bundle stimulation on caudate dialysate dopamine and serotonin. J. Neurochem. 58, 1491–1498.
Martinez, D., Gil, R., Slifstein, M., Hwang, D. R., Huang, Y., Perez, A., Kegeles, L., Talbot, P., Evans, S., Krystal, J., Laruelle, M., and Abi-Dargham, A. (2005). Alcohol dependence is associated with blunted dopamine transmission in the ventral striatum. Biol. Psychiatry 58, 779–786.
Martinez, D., Greene, K., Broft, A., Kumar, D., Liu, F., Narendran, R., Slifstein, M., Van Heertum, R., and Kleber, H. D. (2009). Lower level of endogenous dopamine in patients with cocaine dependence: findings from PET imaging of D(2)/D(3) receptors following acute dopamine depletion. Am. J. Psychiatry 166, 1170–1177.
Martinez, D., Kim, J. H., Krystal, J., and Abi-Dargham, A. (2007). Imaging the neurochemistry of alcohol and substance abuse. Neuroimaging Clin. N. Am. 17, 539–555.
Martinotti, G., Di Nicola, M., Di Giannantonio, M., and Janiri, L. (2009). Aripiprazole in the treatment of patients with alcohol dependence: a double-blind, comparison trial vs. naltrexone. J. Psychopharmacol. 23, 123–129.
Melis, M., Spiga, S., and Diana, M. (2005). The dopamine hypothesis of drug addiction: hypodopaminergic state. Int. Rev. Neurobiol. 63, 101–154.
Meredith, G. E., Baldo, B. A., Andrezjewsky, M. E., and Kelley, A. E. (2008). The structural basis for mapping behavior onto the ventral striatum and its subdivisions. Brain Struct. Funct. 213, 17–27.
Mishra, B. R., Nizamie, S. H., Das, B., and Praharaj, S. K. (2010). Efficacy of repetitive transcranial magnetic stimulation in alcohol dependence: a sham-controlled study. Addiction 105, 49–55.
Murase, S., Grenhoff, J., Chouvet, G., Gonon, F. G., and Svensson, T. H. (1993). Prefrontal cortex regulates burst firing and transmitter release in rat mesolimbic dopamine neurons studied in vivo. Neurosci. Lett. 157, 53–56.
Myrick, H., Li, X., Randall, P. K., Henderson, S., Voronin, K., and Anton, R. F. (2010). The effect of aripiprazole on cue-induced brain activation and drinking parameters in alcoholics. J. Clin. Psychopharmacol. 30, 365–372.
Naranjo, C. A., Dongier, M., and Bremner, K. E. (1997). Long-acting injectable bromocriptine does not reduce relapse in alcoholics. Addiction 92, 969–978.
Padberg, F., and George, M. S. (2009). Repetitive transcranial magnetic stimulation of the prefrontal cortex in depression. Exp. Neurol. 219, 2–13.
Pistis, M., Porcu, G., Melis, M., Diana, M., and Gessa, G. L. (2001). Effects of cannabinoids on prefrontal neuronal responses to ventral tegmental area stimulation. Eur. J. Neurosci. 14, 96–102.
Politi, E., Fauci, E., Santoro, A., and Smeraldi, E. (2008). Daily sessions of transcranial magnetic stimulation to the left prefrontal cortex gradually reduce cocaine craving. Am. J. Addict. 17, 345–346.
Robinson, T. E., and Kolb, B. (1997). Persistent structural modifications in nucleus accumbens and prefrontal cortex neurons produced by previous experience with amphetamine. J. Neurosci. 17, 8491–8497.
Robinson, T. E., and Kolb, B. (2004). Structural plasticity associated with exposure to drugs of abuse. Neuropharmacology 47(Suppl. 1), 33–46.
Rossetti, Z. L., Melis, F., Carboni, S., Diana, M., and Gessa, G. L. (1992). Alcohol withdrawal in rats is associated with a marked fall in extraneuronal dopamine. Alcohol. Clin. Exp. Res. 16, 529–532.
Russo, S. J., Dietz, D. M., Dumitriu, D., Morrison, J. H., Malenka, R. C., and Nestler, E. J. (2010). The addicted synapse: mechanisms of synaptic and structural plasticity in nucleus accumbens. Trends Neurosci. 33, 267–276.
Saletu, B., Saletu, M., Grünberger, J., Frey, R., Zatschek, I., and Mader, R. (1990). On the treatment of the alcoholic organic brain syndrome with an alpha-adrenergic agonist modafinil: double-blind, placebo-controlled clinical, psychometric and neurophysiological studies. Prog. Neuropsychopharmacol. Biol. Psychiatry 14, 195–214.
Schulteis, G., Markou, A., Cole, M., and Koob, G. F. (1995). Decreased brain reward produced by ethanol withdrawal. Proc. Natl. Acad. Sci. U.S.A. 20, 5880–5884.
Semba, J., Watanabe, A., Kito, S., and Toru, M. (1995). Behavioural and neurochemical effects of OPC-14597, a novel antipsychotic drug, on dopaminergic mechanisms in rat brain. Neuropharmacology 34, 785–791.
Shapiro, D. A., Renock, S., Arrington, E., Chiodo, L. A., Liu, L. X., Sibley, D. R., Roth, B. L., and Mailman, R. (2003). Aripiprazole, a novel atypical antipsychotic drug with a unique and robust pharmacology. Neuropsychopharmacology 28, 1400–1411.
Shearer, J., Shanahan, M., Darke, S., Rodgers, C., van Beek, I., McKetin, R., and Mattick, R. P. (2010). A cost-effectiveness analysis of modafinil therapy for psychostimulant dependence. Drug Alcohol Rev. 29, 235–242.
Silvanto, J., Cattaneo, Z., Battelli, L., and Pascual-Leone, A. (2008). Baseline cortical excitability determines whether TMS disrupts or facilitates behavior. J. Neurophysiol. 99, 2725–2730.
Silvanto, J., and Pascual-Leone, A. (2008). State-dependency of transcranial magnetic stimulation. Brain Topogr. 21, 1–10.
Sklair-Tavron, L., Shi, W. X., Lane, S. B., Harris, H. W., Bunney, B. S., and Nestler, E. J. (1996). Chronic morphine induces visible changes in the morphology of mesolimbic dopamine neurons. Proc. Natl. Acad. Sci. U.S.A. 1, 11202–11207.
Spiga, S., Lintas, A., Migliore, M., and Diana, M. (2010). Altered architecture and functional consequences of the mesolimbic dopamine system in cannabis dependence. Addict Biol. 15, 266–276.
Spiga, S., Puddu, M. C., Pisano, M., and Diana, M. (2005). Morphine withdrawal-induced morphological changes in the nucleus accumbens. Eur. J. Neurosci. 22, 2332–2340.
Spiga, S., Serra, G. P., Puddu, M. C., Foddai, M., and Diana, M. (2003). Morphine withdrawal-induced abnormalities in the VTA: confocal laser scanning microscopy. Eur. J. Neurosci. 17, 605–612.
Strafella, A. P., Paus, T., Barrett, J., and Dagher, A. (2001). Repetitive transcranial magnetic stimulation of the human prefrontal cortex induces dopamine release in the caudate nucleus. J. Neurosci. 21, RC157.
Sundaresan, K., Ziemann, U., Stanley, J., and Boutros, N. (2007). Cortical inhibition and excitation in abstinent cocaine-dependent patients: a transcranial magnetic stimulation study. Neuroreport 18, 289–292.
Swift, R. M. (2010). Medications acting on the dopaminergic system in the treatment of alcoholic patients. Curr. Pharm. Des. 16, 2136–2140.
Taber, M. T., Das, S., and Fibiger, H. C. (1995). Cortical regulation of subcortical dopamine release: mediation via the ventral tegmental area. J. Neurochem. 65, 1407–1410.
Thanos, P. K., Taintor, N. B., Rivera, S. N., Umegaki, H., Ikari, H., Roth, G., Ingram, D. K., Hitzemann, R., Fowler, J. S., Gatley, S. J., Wang, G. J., and Volkow, N. D. (2004). DRD2 gene transfer into the nucleus accumbens core of the alcohol preferring and nonpreferring rats attenuates alcohol drinking. Alcohol. Clin. Exp. Res. 28, 720–728.
Thanos, P. K., Volkow, N. D., Freimuth, P., Umegaki, H., Ikari, H., Roth, G., Ingram, D. K., and Hitzemann, R. (2001). Overexpression of dopamine D2 receptors reduces alcohol self-administration. J. Neurochem. 78, 1094–1103.
Urban, N. B., Kegeles, L. S., Slifstein, M., Xu, X., Martinez, D., Sakr, E., Castillo, F., Moadel, T., O’Malley, S. S., Krystal, J. H., and Abi-Dargham, A. (2010). Sex differences in striatal dopamine release in young adults after oral alcohol challenge: a positron emission tomography imaging study with [11C] raclopride. Biol. Psychiatry 68, 689–696.
Volkow, N. D., Wang, G. J., Begleiter, H., Porjesz, B., Fowler, J. S., Telang, F., Wong, C., Ma, Y., Logan, J., Goldstein, R., Alexoff, D., and Thanos, P. K. (2006). High levels of dopamine D2 receptors in unaffected members of alcoholic families: possible protective factors. Arch. Gen. Psychiatry 63, 999–1008.
Volkow, N. D., Wang, G. J., Fowler, J. S., Logan, J., Hitzemann, R., Ding, Y. S., Pappas, N., Shea, C., and Piscani, K. (1996). Decreases in dopamine receptors but not in dopamine transporters in alcoholics. Alcohol. Clin. Exp. Res. 20, 1594–1598.
Volkow, N. D., Wang, G. J., Telang, F., Fowler, J. S., Logan, J., Jayne, M., Ma, Y., Pradhan, K., and Wong, C. (2007). Profound decreases in dopamine release in striatum in detoxified alcoholics: possible orbitofrontal involvement. J. Neurosci. 27, 12700–12706.
Voronin, K., Randall, P., Myrick, H., and Anton, R. (2008). Aripiprazole effects on alcohol consumption and subjective reports in a clinical laboratory paradigm-possible influence of self-control. Alcohol. Clin. Exp. Res. 32, 1954–1961.
Weiss, F., Parsons, L. H., Schulteis, G., Hyytia, P., Lorang, M. T., Bloom, F. E., and Koob, G. F. (1996). Ethanol self-administration restores withdrawal-associated deficiencies in accumbal dopamine and 5-hydroxytryptamine release in dependent rats. J. Neurosci. 16, 3474–3485.
Wise, R. A. (1980). Action of drugs of abuse on brain reward systems. Pharmacol. Biochem. Behav. 13(Suppl. 1), 213–223.
Wise, R. A. (1987). The role of reward pathways in the development of drug dependence. Pharmacol. Ther. 35, 227–263.
Yeomans, J. S. (1989). Two substrates for medial forebrain bundle self-stimulation: myelinated axons and dopamine axons. Neurosci. Biobehav. Rev. 13, 91–98.
Yeomans, J. S., Mathur, A., and Tampakeras, M. (1993). Rewarding brain stimulation: role of tegmental cholinergic neurons that activate dopamine neurons. Behav. Neurosci. 107, 1077–1087.
Zangen, A., and Hyodo, K. (2002). Transcranial magnetic stimulation induces increases in extracellular levels of dopamine and glutamate in the nucleus accumbens. Neuroreport 13, 2401–2405.
Keywords: addiction, dopamine, rTMS, dopamine agents, VTA, prefrontal cortex
Citation: Diana M (2011) The dopamine hypothesis of drug addiction and its potential therapeutic value. Front. Psychiatry 2 :64. doi: 10.3389/fpsyt.2011.00064
Received: 14 September 2011; Paper pending published: 07 October 2011; Accepted: 02 November 2011; Published online: 29 November 2011.
Reviewed by:
Copyright: © 2011 Diana. This is an open-access article subject to a non-exclusive license between the authors and Frontiers Media SA, which permits use, distribution and reproduction in other forums, provided the original authors and source are credited and other Frontiers conditions are complied with.
*Correspondence: Marco Diana, ‘G. Minardi’ Cognitive Neuroscience Laboratory, Department of Drug Sciences, University of Sassari, Via Muroni n. 23, Sassari, Italy. e-mail: dsfdiana@uniss.it
Disclaimer: All claims expressed in this article are solely those of the authors and do not necessarily represent those of their affiliated organizations, or those of the publisher, the editors and the reviewers. Any product that may be evaluated in this article or claim that may be made by its manufacturer is not guaranteed or endorsed by the publisher.
Substance Use Disorders and Addiction: Mechanisms, Trends, and Treatment Implications
Information & authors, metrics & citations, view options, insights into mechanisms related to cocaine addiction using a novel imaging method for dopamine neurons, treatment implications of understanding brain function during early abstinence in patients with alcohol use disorder, relatively low amounts of alcohol intake during pregnancy are associated with subtle neurodevelopmental effects in preadolescent offspring, increased comorbidity between substance use and psychiatric disorders in sexual identity minorities, trends in nicotine use and dependence from 2001–2002 to 2012–2013, conclusions, information, published in.

- Substance-Related and Addictive Disorders
- Addiction Psychiatry
- Transgender (LGBT) Issues
Competing Interests
Export citations.
If you have the appropriate software installed, you can download article citation data to the citation manager of your choice. Simply select your manager software from the list below and click Download. For more information or tips please see 'Downloading to a citation manager' in the Help menu .
Format | |
---|---|
Citation style | |
Style | |
To download the citation to this article, select your reference manager software.
There are no citations for this item
View options
Login options.
Already a subscriber? Access your subscription through your login credentials or your institution for full access to this article.
Not a subscriber?
Subscribe Now / Learn More
PsychiatryOnline subscription options offer access to the DSM-5-TR ® library, books, journals, CME, and patient resources. This all-in-one virtual library provides psychiatrists and mental health professionals with key resources for diagnosis, treatment, research, and professional development.
Need more help? PsychiatryOnline Customer Service may be reached by emailing [email protected] or by calling 800-368-5777 (in the U.S.) or 703-907-7322 (outside the U.S.).
Share article link
Copying failed.
NEXT ARTICLE
Request username.
Can't sign in? Forgot your username? Enter your email address below and we will send you your username
If the address matches an existing account you will receive an email with instructions to retrieve your username
Create a new account
Change password, password changed successfully.
Your password has been changed
Reset password
Can't sign in? Forgot your password?
Enter your email address below and we will send you the reset instructions
If the address matches an existing account you will receive an email with instructions to reset your password.
Your Phone has been verified
As described within the American Psychiatric Association (APA)'s Privacy Policy and Terms of Use , this website utilizes cookies, including for the purpose of offering an optimal online experience and services tailored to your preferences. Please read the entire Privacy Policy and Terms of Use. By closing this message, browsing this website, continuing the navigation, or otherwise continuing to use the APA's websites, you confirm that you understand and accept the terms of the Privacy Policy and Terms of Use, including the utilization of cookies.

An official website of the United States government
The .gov means it’s official. Federal government websites often end in .gov or .mil. Before sharing sensitive information, make sure you’re on a federal government site.
The site is secure. The https:// ensures that you are connecting to the official website and that any information you provide is encrypted and transmitted securely.
- Publications
- Account settings
- My Bibliography
- Collections
- Citation manager
Save citation to file
Email citation, add to collections.
- Create a new collection
- Add to an existing collection
Add to My Bibliography
Your saved search, create a file for external citation management software, your rss feed.
- Search in PubMed
- Search in NLM Catalog
- Add to Search
The dopamine hypothesis of drug addiction: hypodopaminergic state
Affiliation.
- 1 B.B. Brodie Department of Neuroscience, University of Cagliari, 09042 Monserrato, Italy.
- PMID: 15797467
- DOI: 10.1016/S0074-7742(05)63005-X
PubMed Disclaimer
Similar articles
- Dopamine, reinforcement learning, and addiction. Dayan P. Dayan P. Pharmacopsychiatry. 2009 May;42 Suppl 1:S56-65. doi: 10.1055/s-0028-1124107. Epub 2009 May 11. Pharmacopsychiatry. 2009. PMID: 19434556 Review.
- How can drug addiction help us understand obesity? Volkow ND, Wise RA. Volkow ND, et al. Nat Neurosci. 2005 May;8(5):555-60. doi: 10.1038/nn1452. Nat Neurosci. 2005. PMID: 15856062 Review. No abstract available.
- Drug abuse: vulnerability and transition to addiction. Le Moal M. Le Moal M. Pharmacopsychiatry. 2009 May;42 Suppl 1:S42-55. doi: 10.1055/s-0029-1216355. Epub 2009 May 11. Pharmacopsychiatry. 2009. PMID: 19434555 Review.
- [Neurobiology of substance-related addiction: findings of neuroimaging]. Kienast T, Wrase J, Heinz A. Kienast T, et al. Fortschr Neurol Psychiatr. 2008 May;76 Suppl 1:S68-76. doi: 10.1055/s-2008-1038141. Fortschr Neurol Psychiatr. 2008. PMID: 18461548 Review. German.
- Divergent anatomical pattern of D1 and D3 binding and dopamine- and cyclic AMP-regulated phosphoprotein of 32 kDa mRNA expression in the Roman rat strains: Implications for drug addiction. Guitart-Masip M, Johansson B, Fernández-Teruel A, Cañete T, Tobeña A, Terenius L, Giménez-Llort L. Guitart-Masip M, et al. Neuroscience. 2006 Nov 3;142(4):1231-43. doi: 10.1016/j.neuroscience.2006.07.041. Epub 2006 Sep 26. Neuroscience. 2006. PMID: 17008016
- Transcranial Magnetic Stimulation as a Tool to Promote Smoking Cessation and Decrease Drug and Alcohol Use. Harmelech T, Hanlon CA, Tendler A. Harmelech T, et al. Brain Sci. 2023 Jul 14;13(7):1072. doi: 10.3390/brainsci13071072. Brain Sci. 2023. PMID: 37509004 Free PMC article. Review.
- Oral and fecal microbiota perturbance in cocaine users: Can rTMS-induced cocaine abstinence support eubiosis restoration? Gerace E, Baldi S, Salimova M, Di Gloria L, Curini L, Cimino V, Nannini G, Russo E, Pallecchi M, Ramazzotti M, Bartolucci G, Occupati B, Lanzi C, Scarpino M, Lanzo G, Grippo A, Lolli F, Mannaioni G, Amedei A. Gerace E, et al. iScience. 2023 Apr 20;26(5):106627. doi: 10.1016/j.isci.2023.106627. eCollection 2023 May 19. iScience. 2023. PMID: 37250301 Free PMC article.
- Symptoms, Mechanisms, and Treatments of Video Game Addiction. Mohammad S, Jan RA, Alsaedi SL. Mohammad S, et al. Cureus. 2023 Mar 31;15(3):e36957. doi: 10.7759/cureus.36957. eCollection 2023 Mar. Cureus. 2023. PMID: 37009362 Free PMC article. Review.
- Behavioral and biochemical effects of alcohol withdrawal in female C3H/HeNRj and C57BL/6JRj mice. Tonetto S, Weikop P, Brudek T, Thomsen M. Tonetto S, et al. Front Behav Neurosci. 2023 Feb 23;17:1143720. doi: 10.3389/fnbeh.2023.1143720. eCollection 2023. Front Behav Neurosci. 2023. PMID: 36910126 Free PMC article.
- Negative symptoms in alcohol use disorder: A pilot study applying the two-factor model of negative symptoms to patients with alcohol use disorder. Buschner M, Dürsteler KM, Fischli G, Hess J, Kirschner M, Kaiser S, Herdener M. Buschner M, et al. Front Psychiatry. 2022 Nov 18;13:957924. doi: 10.3389/fpsyt.2022.957924. eCollection 2022. Front Psychiatry. 2022. PMID: 36479554 Free PMC article.
Publication types
- Search in MeSH
Related information
- PubChem Compound
- PubChem Compound (MeSH Keyword)
- PubChem Substance
LinkOut - more resources
Full text sources.
- Elsevier Science
Other Literature Sources
- The Lens - Patent Citations
- MedlinePlus Consumer Health Information
- MedlinePlus Health Information
- Citation Manager
NCBI Literature Resources
MeSH PMC Bookshelf Disclaimer
The PubMed wordmark and PubMed logo are registered trademarks of the U.S. Department of Health and Human Services (HHS). Unauthorized use of these marks is strictly prohibited.
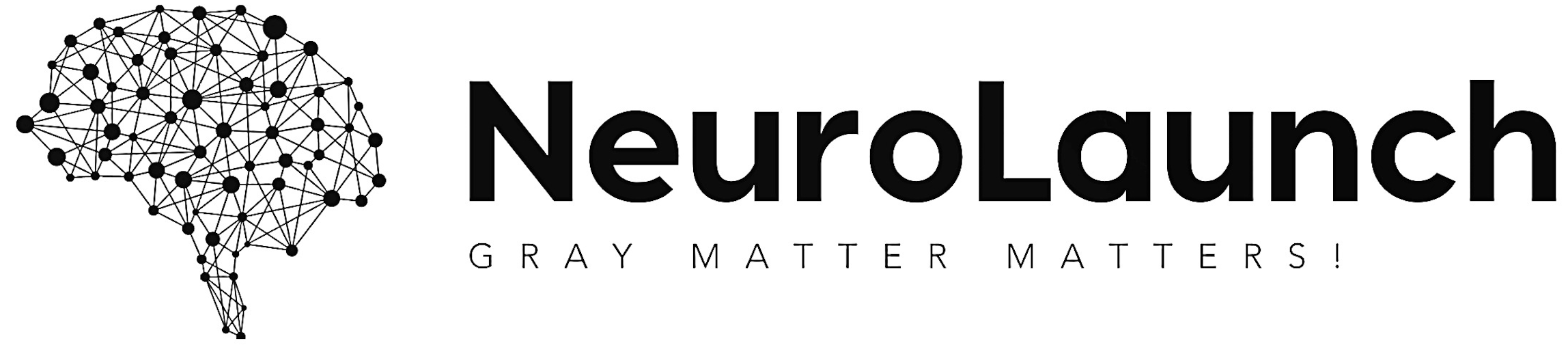
Drug Addiction Research: Latest Findings and Future Directions
A silent war rages within the minds and bodies of millions, as drug addiction continues to claim lives and tear families apart, driving researchers to delve deeper into the intricate web of biological, psychological, and social factors that fuel this devastating disorder. The battle against addiction is far from over, but scientists and healthcare professionals are making significant strides in understanding and treating this complex condition.
As we peel back the layers of addiction biology , we uncover a fascinating tapestry of neural pathways, genetic predispositions, and environmental influences that shape an individual’s susceptibility to substance abuse. This ever-evolving field of study has become a cornerstone in our quest to develop more effective treatments and prevention strategies.
The importance of drug addiction research cannot be overstated. With millions of lives hanging in the balance, scientists are working tirelessly to unravel the mysteries of addiction and find new ways to combat its devastating effects. From neuroscientists probing the depths of the brain’s reward systems to psychologists exploring the intricate interplay between emotions and substance use, the field of addiction studies is a vibrant and dynamic arena of scientific inquiry.
Neurobiology of Drug Addiction: Unveiling the Brain’s Hidden Secrets
At the heart of addiction lies the brain’s reward system, a complex network of neural circuits that evolved to reinforce behaviors essential for survival. However, drugs of abuse hijack this system, creating a false sense of reward that can lead to compulsive drug-seeking behavior.
Recent advances in neuroimaging techniques have allowed researchers to peer into the brains of individuals struggling with addiction, revealing startling changes in structure and function. These studies have shown that chronic drug use can lead to long-lasting alterations in brain chemistry, affecting everything from decision-making to impulse control.
One particularly intriguing area of research focuses on neuroplasticity – the brain’s ability to form new neural connections and adapt to changing circumstances. While this remarkable feature of our brains allows us to learn and grow throughout our lives, it can also work against us in the context of addiction. As drug use becomes habitual, the brain adapts to the constant presence of these substances, leading to tolerance and dependence.
But it’s not just our experiences that shape our susceptibility to addiction. Genetic factors play a significant role as well. Scientists have identified numerous genes that may increase an individual’s risk of developing a substance use disorder. These genetic variations can affect everything from how quickly a person metabolizes drugs to how strongly they respond to their effects.
The Psychology of Addiction: Unraveling the Mind’s Complex Tapestry
While the biological underpinnings of addiction are crucial to understanding the disorder, we cannot ignore the psychological factors that contribute to its development and persistence. The science of addiction extends far beyond the realm of neurobiology, encompassing a wide range of cognitive and emotional processes.
One of the most challenging aspects of addiction is its impact on decision-making and impulse control. Individuals struggling with substance use disorders often find themselves caught in a cycle of craving and use, even when they’re acutely aware of the negative consequences. This phenomenon has led researchers to explore the cognitive processes underlying addiction, including attention bias, working memory deficits, and impaired executive function.
Emotional regulation also plays a crucial role in the development and maintenance of addiction. Many individuals turn to drugs as a way of coping with difficult emotions or traumatic experiences. This self-medication hypothesis has gained significant traction in recent years, leading to a greater focus on addressing underlying emotional issues in addiction treatment.
Behavioral theories of addiction have also contributed greatly to our understanding of the disorder. From classical conditioning to operant reinforcement, these models help explain how drug use becomes ingrained in an individual’s behavioral repertoire over time.
Perhaps one of the most significant challenges in addiction treatment is the high prevalence of comorbid mental health disorders. Conditions such as depression, anxiety, and PTSD often go hand-in-hand with substance use disorders, creating a complex web of interrelated symptoms that can be difficult to untangle.
Social and Environmental Factors: The External Forces Shaping Addiction
While biology and psychology play crucial roles in addiction, we cannot ignore the profound impact of social and environmental factors. The causes of drug addiction are multifaceted, extending far beyond individual choices or genetic predispositions.
Family and peer influences can significantly shape an individual’s relationship with drugs and alcohol. Growing up in a household where substance use is normalized or being surrounded by peers who engage in drug use can increase the likelihood of developing an addiction. Conversely, strong family bonds and positive peer relationships can serve as protective factors against substance abuse.
Socioeconomic factors also play a crucial role in the development and persistence of addiction. Poverty, unemployment, and lack of access to healthcare and education can all contribute to increased rates of substance use disorders. These systemic issues highlight the need for comprehensive, community-based approaches to addiction prevention and treatment.
Cultural perspectives on addiction vary widely across different societies and can significantly impact how individuals view substance use and seek help for addiction. In some cultures, addiction may be stigmatized as a moral failing, while others may view it as a medical condition requiring compassionate care and treatment.
Stress, whether acute or chronic, is another critical factor in the development and maintenance of addiction. The relationship between stress and substance use is complex and bidirectional – stress can lead to increased drug use, while drug use can exacerbate stress levels, creating a vicious cycle that can be difficult to break.
Treatment and Intervention Research: Paving the Way for Recovery
As our understanding of addiction grows, so too does our arsenal of treatment options. From pharmacological interventions to innovative behavioral therapies, researchers are constantly working to develop more effective ways to help individuals overcome substance use disorders.
Pharmacological treatments have come a long way in recent years, with new drugs for addiction offering hope to those struggling with various substance use disorders. These medications can help manage withdrawal symptoms, reduce cravings, and even block the rewarding effects of drugs, making it easier for individuals to maintain sobriety.
Behavioral therapies remain a cornerstone of addiction treatment, with approaches like cognitive-behavioral therapy (CBT), motivational interviewing, and contingency management showing promising results. These interventions help individuals develop coping skills, address underlying psychological issues, and build motivation for change.
Novel approaches in addiction treatment are also emerging, drawing on cutting-edge research in neuroscience and psychology. From neurofeedback techniques to virtual reality-based therapies, these innovative interventions offer new avenues for treating addiction and preventing relapse.
The field of personalized medicine is also making waves in addiction care. By tailoring treatments to an individual’s unique genetic makeup, personal history, and specific needs, healthcare providers can offer more targeted and effective interventions.
Prevention Strategies and Public Health Approaches: Stemming the Tide of Addiction
While treatment is crucial, prevention remains the most effective way to combat the addiction epidemic. Researchers and public health officials are working tirelessly to develop and implement strategies to prevent substance use disorders before they take hold.
Early intervention programs aim to identify and address risk factors for addiction in children and adolescents. By providing support and education during these crucial developmental years, we can help young people build resilience and make informed decisions about substance use.
Community-based prevention initiatives take a holistic approach to addiction prevention, addressing not just individual behaviors but also the social and environmental factors that contribute to substance use. These programs often involve collaboration between schools, healthcare providers, law enforcement, and community organizations.
Policy and legislative approaches also play a crucial role in addressing the addiction crisis. From regulating the marketing and availability of addictive substances to implementing harm reduction strategies, policymakers have a range of tools at their disposal to combat addiction at a societal level.
Education and awareness campaigns remain a vital component of addiction prevention efforts. By providing accurate information about the risks of substance use and challenging harmful stereotypes about addiction, these initiatives can help reduce stigma and encourage individuals to seek help when needed.
The Road Ahead: Challenges and Opportunities in Addiction Research
As we look to the future of addiction research and theory , it’s clear that we’ve made significant strides in understanding this complex disorder. From unraveling the intricate workings of the brain’s reward system to developing innovative treatment approaches, researchers have laid a solid foundation for future advances in the field.
However, significant challenges remain. The opioid epidemic continues to claim lives at an alarming rate, and new synthetic drugs are constantly emerging, presenting novel threats to public health. Moreover, the COVID-19 pandemic has exacerbated many of the risk factors for addiction, including social isolation, economic instability, and mental health issues.
Despite these challenges, there is reason for hope. Emerging trends in addiction research, such as the exploration of psychedelic-assisted therapies and the development of more targeted pharmacological interventions, offer exciting possibilities for the future of addiction treatment.
As we continue to grapple with the complexities of drug use and addiction , it’s clear that a multidisciplinary approach is essential. By bringing together experts from fields as diverse as neuroscience, psychology, sociology, and public health, we can develop more comprehensive and effective strategies for preventing and treating addiction.
Ultimately, the key to progress lies in continued investment in addiction research. By supporting the tireless efforts of scientists, healthcare professionals, and policymakers, we can hope to turn the tide in the battle against addiction and bring hope to the millions of individuals and families affected by this devastating disorder.
As we stand on the cusp of new breakthroughs in addiction science, one thing is clear: the fight against addiction is far from over, but with each new discovery, we move one step closer to a world where substance use disorders are preventable, treatable, and ultimately, beatable.
References:
1. Volkow, N. D., Koob, G. F., & McLellan, A. T. (2016). Neurobiologic advances from the brain disease model of addiction. New England Journal of Medicine, 374(4), 363-371.
2. Nestler, E. J. (2014). Epigenetic mechanisms of drug addiction. Neuropharmacology, 76, 259-268.
3. Hendershot, C. S., Witkiewitz, K., George, W. H., & Marlatt, G. A. (2011). Relapse prevention for addictive behaviors. Substance Abuse Treatment, Prevention, and Policy, 6(1), 17.
4. Substance Abuse and Mental Health Services Administration. (2020). Key substance use and mental health indicators in the United States: Results from the 2019 National Survey on Drug Use and Health. https://www.samhsa.gov/data/sites/default/files/reports/rpt29393/2019NSDUHFFRPDFWHTML/2019NSDUHFFR1PDFW090120.pdf
5. National Institute on Drug Abuse. (2020). Drugs, Brains, and Behavior: The Science of Addiction. https://nida.nih.gov/publications/drugs-brains-behavior-science-addiction
6. World Health Organization. (2018). Global status report on alcohol and health 2018. https://www.who.int/publications/i/item/9789241565639
7. Volkow, N. D., & Boyle, M. (2018). Neuroscience of addiction: Relevance to prevention and treatment. American Journal of Psychiatry, 175(8), 729-740.
8. Koob, G. F., & Volkow, N. D. (2016). Neurobiology of addiction: a neurocircuitry analysis. The Lancet Psychiatry, 3(8), 760-773.
9. Sinha, R. (2008). Chronic stress, drug use, and vulnerability to addiction. Annals of the New York Academy of Sciences, 1141, 105-130.
10. Blanco, C., Iza, M., Rodríguez-Fernández, J. M., Baca-García, E., Wang, S., & Olfson, M. (2015). Probability and predictors of treatment-seeking for substance use disorders in the U.S. Drug and Alcohol Dependence, 149, 136-144.
Similar Posts
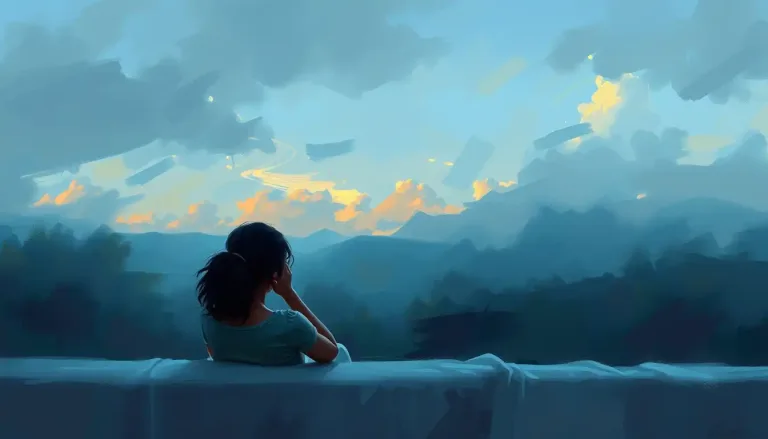
Addiction Terminology: Exploring Synonyms, Antonyms, and Alternative Expressions
A tangled web of words, addiction’s lexicon is a minefield of nuance, where synonyms and antonyms intertwine to shape our perceptions and responses to this complex issue. As we navigate this linguistic labyrinth, we find ourselves confronted with a kaleidoscope of terms, each carrying its own weight and implications. It’s a bit like trying to…
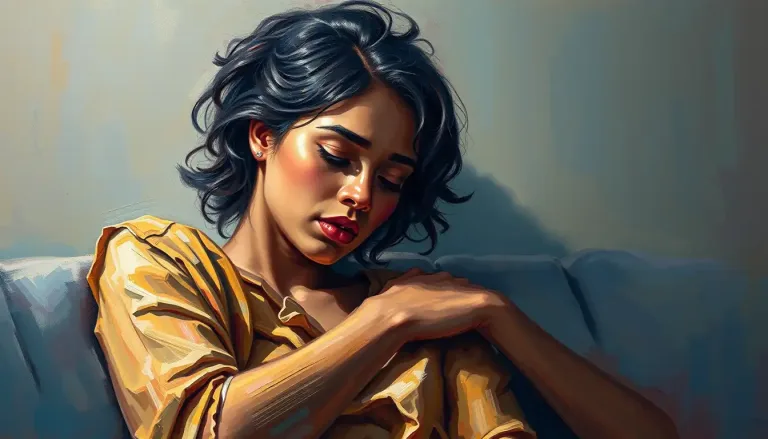
Addiction Treatment Market: Global Trends, Challenges, and Future Outlook
As the global battle against addiction rages on, the addiction treatment market finds itself at a critical juncture, poised to revolutionize the way we approach and overcome this pervasive public health crisis. The landscape of addiction treatment is evolving rapidly, with new technologies, therapies, and approaches emerging to tackle this complex and multifaceted issue. It’s…
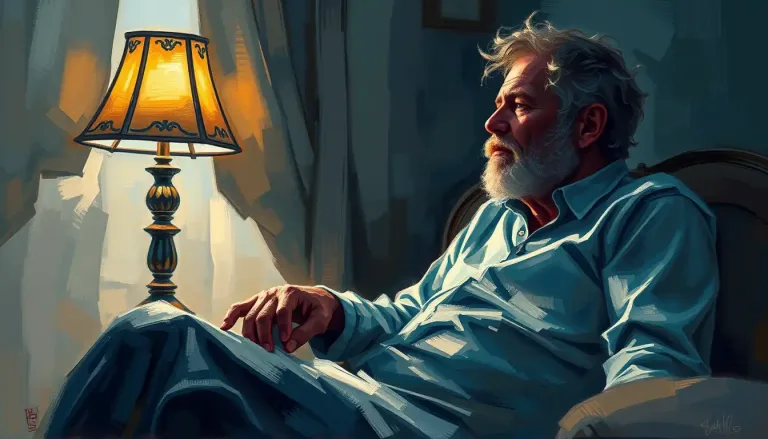
Opiate Addiction: Understanding the Opioid Crisis and Finding Help
A silent killer, opiate addiction has swept across the nation, leaving a trail of shattered lives and broken communities in its wake. This insidious epidemic has crept into every corner of society, affecting people from all walks of life, regardless of age, race, or socioeconomic status. It’s a crisis that demands our attention, understanding, and…
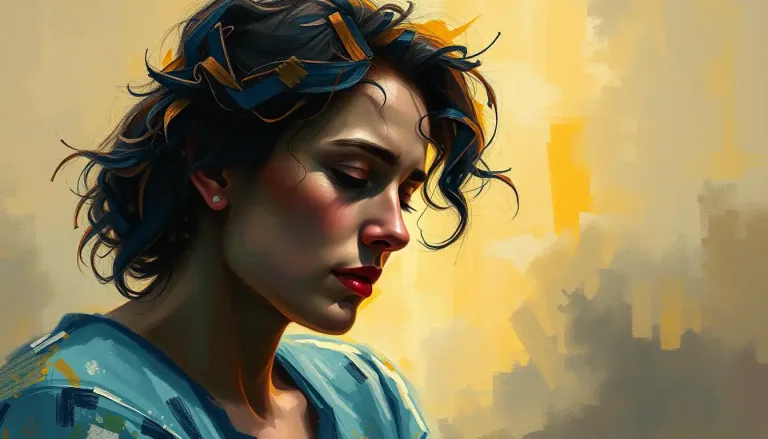
Dislocation Theory of Addiction: A New Perspective on Substance Abuse
A groundbreaking theory is challenging the way we think about addiction, offering a fresh perspective that could revolutionize treatment and recovery for millions struggling with substance abuse. This new approach, known as the dislocation theory of addiction, is turning heads in the scientific community and sparking heated debates among addiction specialists worldwide. For decades, we’ve…
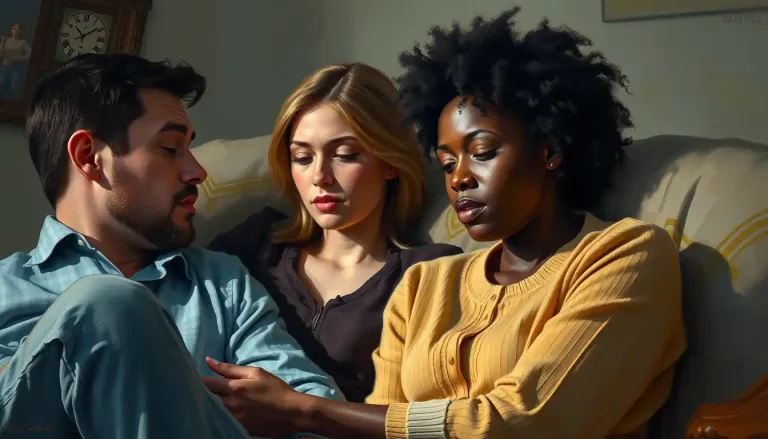
Addiction and Genetics: Exploring the Family Connection
Addiction’s sinister threads weave through generations, ensnaring families in a complex tapestry of genetic predispositions and environmental influences. The specter of addiction looms large in many households, casting long shadows that stretch across time and bloodlines. But what exactly is addiction, and why does it seem to cling so tenaciously to certain family trees? At…
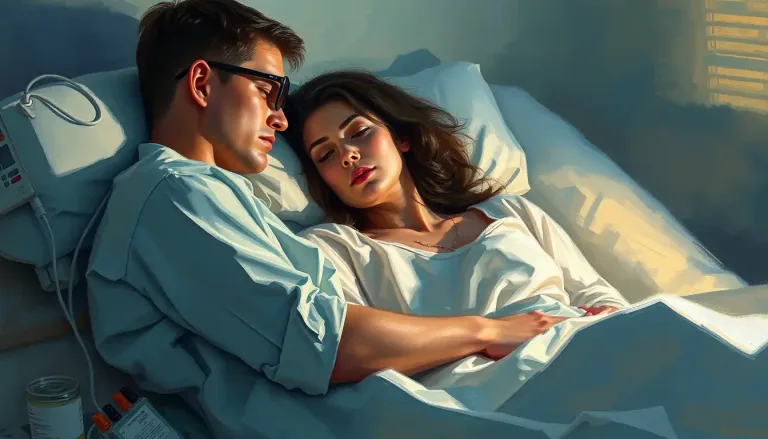
Morphine Addiction: Recognizing Signs, Understanding Risks, and Finding Recovery
Morphine’s seductive embrace lures unsuspecting souls into a treacherous dance, where the line between relief and ruin blurs with each dose. This potent opioid, derived from the opium poppy, has been both a blessing and a curse in the medical world. Its pain-relieving properties have brought comfort to countless patients, but its potential for addiction…
Leave a Reply Cancel reply
Your email address will not be published. Required fields are marked *
Save my name, email, and website in this browser for the next time I comment.
- Alzheimer's disease & dementia
- Arthritis & Rheumatism
- Attention deficit disorders
- Autism spectrum disorders
- Biomedical technology
- Diseases, Conditions, Syndromes
- Endocrinology & Metabolism
- Gastroenterology
- Gerontology & Geriatrics
- Health informatics
- Inflammatory disorders
- Medical economics
- Medical research
- Medications
- Neuroscience
- Obstetrics & gynaecology
- Oncology & Cancer
- Ophthalmology
- Overweight & Obesity
- Parkinson's & Movement disorders
- Psychology & Psychiatry
- Radiology & Imaging
- Sleep disorders
- Sports medicine & Kinesiology
- Vaccination
- Breast cancer
- Cardiovascular disease
- Chronic obstructive pulmonary disease
- Colon cancer
- Coronary artery disease
- Heart attack
- Heart disease
- High blood pressure
- Kidney disease
- Lung cancer
- Multiple sclerosis
- Myocardial infarction
- Ovarian cancer
- Post traumatic stress disorder
- Rheumatoid arthritis
- Schizophrenia
- Skin cancer
- Type 2 diabetes
- Full List »
share this!
September 11, 2024
This article has been reviewed according to Science X's editorial process and policies . Editors have highlighted the following attributes while ensuring the content's credibility:
fact-checked
peer-reviewed publication
trusted source
How the Parkinson's drug Levodopa causes psychiatric complications similar to drug abuse
by Karolinska Institutet

Researchers at Karolinska Institutet have identified a mechanism responsible for a serious psychiatric complication caused by dopamine replacement therapy in Parkinson's disease. The results of these findings were recently published in Molecular Psychiatry .
Treatment of Parkinson's disease is in large part based on the administration of levodopa. The use of this drug is limited by the development of psychiatric complications, including a disorder similar to drug abuse and referred to as dopamine dysregulation syndrome (DDS).
"We have identified a mechanism at the basis of DDS. We found that, in an experimental model of Parkinson's disease, treatment with levodopa leads to abnormal activation of a specific group of neurons located in the brain rewarding system and involved in the effects of addictive substances," explains Gilberto Fisone, Professor at the Department of Neuroscience and main author of the study.
The resulting change in transmission leads to dysregulation of specific proteins involved in neuronal function, which represent a potential target for interventions counteracting psychiatric disorders in Parkinson's disease patients.
DDS affects approximately five percent of Parkinson's patients treated with levodopa and involves a pathological overconsumption of medication, far beyond that necessary to correct the motor symptoms of the disease.
"Patients with DDS feel under-medicated, ignore recommended dosing schedules and self-medicate to a level that often leads to further complications, such as dyskinesia," says Fisone.
DDS is a major concern for patients and their families. However, little is known about the mechanisms involved in this disorder. The identification of a specific set of neurons pathologically affected by levodopa and causally linked to addictive behavior provides this information, paving the way for the future identification of measures to improve the treatment of Parkinson's disease.
The study was based on the use of a rodent model of Parkinson's disease that recreates its complex pathology. The researchers found that the addictive-like effect of levodopa was due to its ability to hyperactivate a group of neurons expressing the dopamine D1 receptor (D1R) in the basal ganglia.
The next step is to investigate the possible involvement in DDS of Delta-FosB, an important regulator of gene expression implicated in drug addiction. Delta-Fos B levels are enhanced in D1R-expressing neurons following administration of levodopa .
"Our hypothesis is that by controlling the overexpression of Delta-FosB via genetic intervention we can also counteract DDS," says Fisone.
Explore further
Feedback to editors

Team discovers role of ferroptosis in combating breast cancer resistance
Sep 13, 2024

Metformin found to reduce organ aging in male monkeys

Researchers discover new target for treating heart failure: Protein kinase N

RNA-sequencing study provides novel insights into chronic lymphocytic leukemia

Scientists discover potential cause of an enigmatic vascular disease primarily impacting women

Key factors identified that can impact long-term weight loss in patients with obesity prescribed GLP-1 RA medications

Study finds 'supercharging' T cells with mitochondria enhances their antitumor activity

Using AI, researchers find e-cigarette brands are skirting the rules about health warning labels on Instagram

New therapy that targets and destroys tau tangles: A promising Alzheimer's disease treatment

Neoself-antigens found to induce autoimmune response in lupus
Related stories.

Finding right drug balance for Parkinson's patients
Sep 29, 2020

Clinical trial shows subcutaneous infusion pump safe, effective for Parkinson's treatment
Mar 18, 2024
Resting tremor in focus in new Parkinson's study
Jun 10, 2024

Subcutaneous infusion of levodopa-carbidopa beneficial for Parkinson's disease
Apr 13, 2024

New form of levodopa might improve parkinson's care
Apr 26, 2023

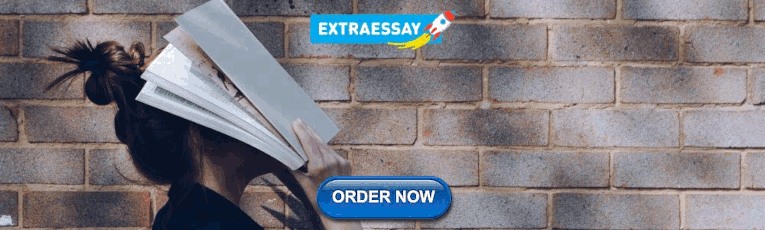
Study finds that dopaminergic medication improves sleep quality in Parkinson's disease patients
Aug 13, 2024
Recommended for you

Using machine learning to uncover predictors of well-being

How genes shape personality traits: New links discovered
Sep 12, 2024

A novel neural explanation for choking under pressure

Rapid blood diagnostic test developed for amyotrophic lateral sclerosis

Exposure to air pollution during pregnancy increases postpartum depression risk for at least three years, study finds

Supported youth become supportive adults, researchers find
Let us know if there is a problem with our content.
Use this form if you have come across a typo, inaccuracy or would like to send an edit request for the content on this page. For general inquiries, please use our contact form . For general feedback, use the public comments section below (please adhere to guidelines ).
Please select the most appropriate category to facilitate processing of your request
Thank you for taking time to provide your feedback to the editors.
Your feedback is important to us. However, we do not guarantee individual replies due to the high volume of messages.
E-mail the story
Your email address is used only to let the recipient know who sent the email. Neither your address nor the recipient's address will be used for any other purpose. The information you enter will appear in your e-mail message and is not retained by Medical Xpress in any form.
Newsletter sign up
Get weekly and/or daily updates delivered to your inbox. You can unsubscribe at any time and we'll never share your details to third parties.
More information Privacy policy
Donate and enjoy an ad-free experience
We keep our content available to everyone. Consider supporting Science X's mission by getting a premium account.
E-mail newsletter

An official website of the United States government
Here’s how you know
Official websites use .gov A .gov website belongs to an official government organization in the United States.
Secure .gov websites use HTTPS A lock ( Lock Locked padlock icon ) or https:// means you’ve safely connected to the .gov website. Share sensitive information only on official, secure websites.
2024 NIDA International Forum Executive Summary
June 14-15, 2024 Montreal, Canada
After three years of virtual meetings, the 2024 NIDA International Forum returned to an in-person format. The meeting, which was held on June 14 and 15 in Montreal in conjunction with the College on Problems of Drug Dependence (CPDD) Annual Scientific Meeting, was cochaired by NIDA International Program’s leadership, Dr. Tom Clarke and Dr. Lindsey Friend. It was attended by over 200 individuals from 73 countries. The meeting included several plenary sessions, four breakout sessions, and an international research poster session featuring 90 posters presented by substance use and addiction researchers from 26 countries.
The Forum began on June 14 with two sessions on advancing quality of addiction study programs and on addiction neuroscience research in Bulgaria, as well as a workshop on how to get addiction research published in peer-reviewed journals.
Day 2 started with the official welcome and updates on NIDA’s programs. This was followed by four breakout sessions on addiction treatment and criminal justice systems, professional healthcare education regarding medical cannabis, addressing opioid stigma in pharmacies, and effective interventions to increase treatment access and intake in real-world settings across the globe. The meeting concluded with two plenary sessions that discussed substance use and associated health problems in humanitarian settings and addressed two current global topics, namely, emerging synthetic drugs in Latin America and the Caribbean and the treatment of children with substance use problems. Rounding out the program were a joint workshop conducted by NIDA staff and staff from the CPDD, as well as a poster session.
Following are brief summaries of the topic sessions presented at this year’s International Forum.
Welcome and NIDA Update
Dr. Clarke provided a high-level overview of NIDA’s research priorities. In the United States, the most pressing problem continues to be the opioid overdose crisis, which is currently driven by co-use of fentanyl and stimulants and disproportionately affects American Indian/Alaska Native and Black communities. Also highly concerning are continued high rates of unintentional fentanyl-related overdose deaths in youths ages 15-19. In response, NIDA’s 2022 to 2026 strategic plan has identified five core research priorities, including (1) understanding drugs, the brain, and behavior; (2) prevention, treatment, and recovery; (3) the intersection of substance use and HIV; (4) implementation science; and (5) innovative heath applications.
Dr. Clarke also introduced several large programs that NIDA supports — partly together with other NIH Institutes — to address the opioid public health crisis. One of these efforts is the Helping to End Addiction Long-term® Initiative, or NIH HEAL Initiative® — an NIH-wide effort to speed scientific solutions to the opioid health crisis that is jointly led by NIDA and the National Institute on Neurological Disorders and Stroke. Other major NIDA-supported research efforts include the longitudinal HEALthy Brain and Child Development (HBCD) and Adolescent Brain Cognitive Development (ABCD) studies, the HEALing Communities Study, the Justice Community Opioid Innovation Network (JCOIN), the Harm Reduction Research Network, and the NIDA Clinical Trials Network. Together, these efforts address challenges, such as emerging drugs (e.g., xylazine), increasing fentanyl and polysubstance use, persistent stigma, better care for hard-to-reach and underserved populations, continuity of care for substance use disorders (SUDs), and novel harm reduction approaches.
Dr. Friend summarized the activities of the NIDA International Program and its role in international policy development, information, exchange, research, and training. In particular, NIDA supports international research through grants awarded to U.S. researchers partnering with foreign investigators. NIDA’s international training and research programs include INVEST postdoctoral fellowships and Hubert H. Humphrey Fellowships. INVEST fellowships offer a 12-month postdoctoral research training with a NIDA grantee that includes professional development activities to establish personal relationships between the fellows and NIDA grantees and NIDA officials. They are for international researchers with a doctoral degree and a minimum of 2 years postdoctoral experience. Hubert H. Humphrey Fellowships are mid-career fellowships to approximately 12 individuals per year that include a 10-month research fellowship at Virginia Commonwealth University as well as 6-week professional affiliations, allowing fellows to make connections to advance their ongoing work. Additionally, NIDA offers the Distinguished International Scientist Collaboration Awards (DISCA) that fund innovative collaborations between international and NIDA-funded senior researchers. Dr. Friend also highlighted NIH’s Research Portfolio Online Reporting Tool, NIH RePORTER ( https://reporter.nih.gov ), as an excellent tool for learning about NIDA-funded projects or investigators funded in other countries.
Addiction Treatment and Criminal Justice Systems: Drug Policy Reforms and Implications
This breakout session was chaired by Dr. Gregory Bunt from the International Society of Addiction Medicine (ISAM), who introduced the International Consortium for Alternatives to Incarceration (ICATI). ICATI works with countries, organizations, professionals, and program sites to support the global development of treatment, care, and accountability as alternatives to incarceration for individuals who use drugs and become involved with the justice system. At various stages in the judicial process, the justice system can divert people to treatment and services in the community instead of incarcerating them. This has been shown to reduce recidivism. To help adopt and implement such measures, ICATI promotes and supports all phases of national and regional planning, training, technical assistance, evaluation, and implementation; identifies, develops, and provides resources; and helps establish international networks.
In lieu of Dr. Carl Erik Fisher from Columbia University in New York, who was not able to attend the meeting, Dr. Bunt also gave Dr. Fisher’s presentation on the “Portuguese model” of decriminalization as an alternative harm reduction approach for treating SUDs in minoritized communities. Decriminalization means elimination of criminal penalties for drug possession for personal use; it differs from depenalization, which generally means that criminal penalties are reduced or not enforced but are not legally eliminated, and from legalization of drug use. This approach was first implemented in Portugal, where drug possession was decriminalized in 2001 but people carrying a personal drug supply had to get mandatory treatment. This was accompanied by several other socio-medical and harm-reduction interventions for a holistic approach with wrap-around services. However, because existing laws against personal drug use had already been only loosely enforced, decriminalization may ultimately have formalized existing practices rather than drive new norms and attitudes. In the United States, a similar approach has been implemented in Oregon but has been less successful because people were not diverted to effective treatment. This suggests that even the best reform programs do not guarantee success because drug-related problems are shaped by additional factors, such as cultural attitudes, social support networks, or mental health resources.
Dr. David Martell from the Canadian Society of Addiction Medicine reported on Canada’s drug decriminalization efforts. Canada has a history of targeted exemptions from penalizing drug use, from prescription maintenance programs and wide availability of opioid agonist therapy to supervised consumption sites and drug checking programs. Dr. Martell also listed various legislative approaches to drug decriminalization, such as the 2017 “Good Samaritan Drug Overdose Act” or cannabis legalization in 2018, which legalized personal use but maintained criminal penalties for certain offenses. This legalization resulted in increased cannabis use for most age groups and a temporary increase in the cannabis industry, but more Canadians now get cannabis legally. A newer decriminalization pilot program started in 2023 decriminalizes use of small amounts of opioids, crack, cocaine, methamphetamine, or MDMA at certain sites (e.g., private residences, but also overdose prevention, drug checking, or supervised consumption sites). The aim is to eliminate the harms associated with drug seizure, help connect people who use drugs to services and support, and enhance public awareness and understanding. However, over the first 12 months, the project has not yielded benefits, and many issues are also still unaddressed. Dr. Martell concluded that Canada’s national drug policy landscape is still fragmented and the search for the best approach continues.
Dr. Hamed Ekhtiari from the University of Minnesota and ISAM Regional Council chair, discussed how the ISAM Global Expert Network (ISAM GEN) can contribute to global drug policy reforms. ISAM’s organizational hierarchy allows for representation and policy involvement across the globe through regional representatives, national ambassadors, addiction medicine societies, and 10 country experts for each country who represent the ISAM GEN. By eliciting information from these experts and conducting a range of global surveys, ISAM GEN can help map and reshape global policies. ISAM GEN has established a well-honed road map for conducting their surveys, from idea development and steering committee formation all the way to manuscript submission. ISAM GEN’s first global survey assessed treatment service provision for opioid use disorder by querying national addiction societies and organizations in 39 countries from six continents. It gathered valuable information on availability of different types of opioids and availability of different types of treatment services for opioid use disorder, harm reduction facilities, and 12-step programs. Researchers interested in establishing their own international working groups or developing surveys can contact ISAM for further information.
Medical Cannabis in Professional Healthcare Education: Multinational Policies, Perspectives, and Competencies
In this breakout session, which was moderated by Dr. Richard Isralowitz from the Ben-Gurion University of the Negev—RADAR Center in Israel, Dr. Richard Rawson, professor emeritus from the University of California, Los Angeles, first provided a historical overview of substance use training in professional curricula. He noted that information on substance use and its impact has only recently been integrated into professional healthcare training rather than being covered only in ad-hoc separate training events. Since 2000, training in substance use and its treatment has become more formalized, with exams and certification processes offered by national and international certification organizations. International training activities are coordinated by the International Consortium of Universities for Drug Demand Reduction (ICUDDR), which facilitates networking among universities to promote high-quality education and training for prevention, treatment, and public health intervention purposes. As a result, many universities now integrate alcohol, tobacco, and other drug content into their curricula, often within many specialty areas; however, cannabis and medical marijuana remain a new curriculum topic.
Dr. Yuval Zolotov from the Albert Einstein College of Medicine in the Bronx, New York, summarized the results of an international survey among healthcare professions’ students in Israel, Malta, Greece, Cyprus, Russia, Belarus, Thailand, and the United States regarding their attitudes, beliefs, and knowledge about medical cannabis. Participants were 4,427 students (70% female) of medicine, nursing, social work, and psychology; 74% came from countries where medical cannabis was legal. Dr. Zolotov reported that, overall, respondents expressed relatively high levels of support for medical cannabis, but also concerns about potential risks and harms. Almost all respondents believed that medical cannabis should be included in their education and practical experiences, but almost 87% reported receiving no formal education in this area. As a result, about 70% of respondents felt unprepared to answer patient questions about medical cannabis. Dr. Zolotov concluded that a great need for formal education in medical cannabis exists and that standardized curricula can help bridge the existing gaps.
Dr. Mazen Sakka from the Substance Abuse Research Center, Palestine, summarized a similar survey among medical staff at two hospitals and students at four universities in the Palestinian territories (West Bank, Gaza Strip) about their knowledge, beliefs, and attitudes regarding medical cannabis. The survey also explored the roles of gender, religiosity, and legal status of medical cannabis on these domains. In the Palestinian territories, cannabis is considered a schedule I substance, together with other addictive drugs. The survey found that while most medical staff and many students believed that medical cannabis can help certain patients, they also saw a high risk of mental and physical health problems. However, many respondents had only limited knowledge on the subject. Additionally, attitudes and beliefs differed somewhat between males and females and were affected by religious views and the fact that cannabis is considered an illegal drug. Nevertheless, Dr. Sakka noted that most respondents seemed open to more information or training on medical marijuana, supporting efforts to enhance such activities.
Given the apparent need for and interest in professional healthcare education on medical cannabis, Dr. Mikhail Kogan from George Washington University and Dr. Leslie Mendoza Temple from the University of Chicago described a Delphi process to identify teaching standards and essential medical cannabis education competencies. The Drug Enforcement Agency in the United States is set to reschedule cannabis to a lower status, which would require all pharmacies in the United States to carry cannabis products. After a 2021 scoping review found that healthcare trainees lack proper education and knowledge about medical cannabis, implementation of expanded competencies-based curricula is essential. Drs. Kogan and Temple described the Delphi process methodology, which relies on synthesizing opinions among a group of experts via repeated rounds of discussion until a consensus is reached. In this case, the process involved 23 experts with different areas of expertise, who identified six core competencies to guide development of medical school curricula in the United States and Canada. These included (1) understanding the basics of the endocannabinoid system, (2) describing the main components of the cannabis plant and their effects, (3) reviewing the legal and regulatory landscape on cannabis in the United States, (4) describing the evidence base for health conditions that are commonly managed with cannabis, (5) understanding the potential risks of medical cannabis use, and (6) understanding basic clinical management with medical cannabis.
Addressing Opioid Stigma in Pharmacies — Developing Strategies for Pharmacy Professionals
This breakout session with Dr. Beth Sproule and Dr. Braiden Cutmore from the Canadian Centre for Addiction and Mental Health and Dr. Sarah Bhatti from the Public Health Agency of Canada (PHAC) centered on the stigma that people who use drugs and people with chronic pain frequently face. The session focused specifically on stigma from pharmacy professionals. As Dr. Bhatti noted, experiences of stigma may decrease use of treatment and social services as well as quality of care. A 2019 report by the Canadian Chief Public Health Officer concluded that responding to stigma in the health system requires a comprehensive approach through education, training, practice, and policy. PHAC conducted a literature review to clarify the nature and manifestations of stigma; assess stigma related to treatment with naloxone, opioid agonist therapy, harm reduction approaches, and opioid treatment for pain in pharmacy professionals; as well as anti-stigma approaches. The review identified several key ingredients for anti-stigma programs, such as repeated social contact, demonstration of recovery, myth busting, and enthusiastic facilitation. Dr. Bhatti also indicated that targeted education is more successful than general education.
Dr. Cutmore described several stakeholder roundtables organized by PHAC that discussed definitions of stigma, experiences of stigma, positive experiences with pharmacies, and potential messages for pharmacy professionals. The roundtables included people with opioid use disorder, people with chronic pain, and pharmacy professionals, respectively. Based on the feedback from the three stakeholder groups, six messages for pharmacists were drafted that addressed the main themes raised — for example, that pharmacy professionals need to see patients as a whole person and treat them with dignity and respect, that they need to understand the patient’s opioid medication experience and act as allies to support patients, or that they need to proactively engage and empower patients through education. Agreement with these messages was then assessed in an anonymous survey among roundtable participants. According to Dr. Cutmore, the roundtables and resulting messages highlighted that it is crucial for pharmacy professionals to challenge negative attitudes and beliefs by reflecting on their assumptions toward people taking opioids and recognize their impact. Additionally, they need to help empower through communication their patients who are prescribed opioids.
Dr. Sproule then presented a newly developed tool kit delineating strategies for pharmacy professionals to address opioid stigma in pharmacies. It highlights eight key strategies to support people who use opioids, such as respecting all customers equally, getting to know customers, offering support through education, explaining and reviewing pharmacy process, providing harm reduction services, delivering compassionate healthcare, respecting the right to privacy, and raising public awareness. The toolkit also provides tips on how to put those strategies into practice. One concept Dr. Sproule highlighted is the idea that “words matter” — that how one talks to or about people who use substances can either reinforce or, in contrast, counter stigmatizing attitudes, views, and actions. The toolkit also includes social media assets and posters to raise awareness of and address opioid stigma in pharmacies. Infographics, videos, courses, and other resources are also available. The toolkit was launched in February 2024 with a webinar attended by more than 250 pharmacy professionals and other stakeholders.
Successful Real-World Evidence-Based Interventions That Have Increased Treatment Access and Intake in Different Countries
This breakout session was chaired by Dr. Adrian Abagiu from the Romanian National Institute for Infectious Diseases and Dr. Matei Bals from the ARENA OST Center in Romania. Dr. Abagiu reported on a program in Romania to increase adherence to antiretroviral therapy (ART) among people living with HIV (PLWH) who were incarcerated. He described that some of these individuals refused ART due to restrictions according to detention regulations. To improve ART adherence, they developed an information program involving a 20-minute PowerPoint presentation, a 10-minute Q&A session, and information leaflets for small groups of PLWH at one of the prisons. The intervention improved adherence, but the effect declined after 4 months. As a result, the program was refined further and assessed in a study comparing 100 PLWH who received the intervention with 50 PLWH who did not in one jail. Before the intervention, ART refusal rates were the same in both groups. After the intervention, refusal rates declined in the intervention group, but remained relatively stable in the control group; again, the effect was lost at 4 months after the intervention. Based on these findings, the intervention was delivered every 2 months to all new PLWH arriving at the prison, and while it had to be halted during the COVID-19 pandemic, it was resumed after the end of the pandemic.
Next, Dr. Dace Svikis from Virginia Commonwealth University described the Expanding Medical Prevention and Outreach While Enhancing Recovery and Retention (EMPOWER) project that seeks to increase engagement and retention in SUD and HIV treatment for underserved Black people in Baltimore, Maryland. Dr. Svikis explained that Baltimore has one of the highest rates of new HIV cases and also has the highest fatal overdose rates among large cities in the United States, but is also a leader in SUD treatment and harm reduction approaches. EMPOWER was assessed within the Recovery Enhanced by Access to Comprehensive Healthcare (REACH) Health Services Program that offers comprehensive outpatient SUD treatment as well as mental health counseling. However, Black individuals in the program were less likely to engage in SUD treatment, more likely to drop out of care, and thus less access to HIV-related testing and care. The EMPOWER program seeks to address these disparities using tailored, patient-focused, evidence-based interventions to engage and retain Black individuals in treatment. Importantly, the EMPOWER team includes people who have lived experience and represent the population of focus, such as peer recovery specialists and nurse care managers. EMPOWER uses evidence-based interventions, such as motivational interviewing, continency management, safer sex skill building, and Seeking Safety, a cognitive behavioral therapy for post-traumatic stress disorder and SUD. The EMPOWER program started recruitment in February 2024 and will last 6 months, after which regular REACH services will continue. Preliminary findings indicate that EMPOWER can increase treatment retention at 3 months compared with the normal REACH program; additional data will be gathered in the coming months.
The third presenter was current Hubert H. Humphrey Fellow Thinzar Tun from Myanmar, director of the Asian Harm Reduction Network (AHRN) and Best Shelter Myanmar, who reported on a program she is spearheading to increase treatment access and intake for people who use drugs (PWUD) or inject drugs (PWID). She explained that Myanmar has about 93,000 PWID, about 35% of whom have HIV. AHRN provides facility-based harm reduction, treatment, and care, whereas Best Shelter offers community-based harm reduction, prevention, referral, and care. Specific services include outreach, health education, self-help groups, job creation efforts, peer education, counseling, and treatment. Some services are specifically aimed at women as a particularly vulnerable population, such as services to improve sexual and reproductive health, as well as pre- and postnatal care, and services to curb gender-based violence. Ms. Tun specifically described their needle and syringe programs, including efforts to return and safely dispose of used needles and syringes, as well as HIV testing and treatment. These are implemented either during outreach with mobile units or at fixed locations in clinics or in the community. Ms. Thun reported that for 2022, about 18 million needles and syringes were distributed, with a return rate of 86%. For HIV testing, the program brought testing closer to the PWID and their families, allowed for effective linkage to care and rapid ART initiation, as well as testing and treatment in hard-to-reach locations. The program reduced the proportion of PWID who were HIV positive and achieved high linkage to treatment and ART initiation for both PWUD and PWID and their sexual partners. Ms. Tun concluded that to address drug use and HIV in countries such as Myanmar, understanding the context and needs of clients is essential, and that community inclusion and harm reduction approaches work.
Substance Use and Associated Health Problems in Humanitarian Settings — Responding to the Needs of Affected Populations
This plenary session was started by Dr. Anja Busse from the United Nations Office on Drugs and Crime (UNODC), who provided an update on addressing substance use in humanitarian emergencies. Dr. Busse explained that humanitarian emergencies (e.g., disasters, armed conflicts, epidemics) threaten the lives and well-being of very large numbers of people or a very large percentage of a population, often result in displacement, and require assistance from multiple sectors. There is only limited evidence on substance use among displaced persons; however, there seem to be regional differences regarding the most commonly used substances. Displaced populations or people in humanitarian emergencies may face particular challenges related to drug use, such as sudden interruptions of patterns of drug use, disruption of treatment services, and resumption of drug use with increased risk of overdose; these compound social and mental health problems associated with humanitarian crises. Dr. Busse noted that substance use is included in humanitarian health guidance as part of mental health interventions. A Desk Review by the United Nations High Commissioner for Refugees (UNHCR) found that community-based, peer-led programs and training of healthcare workers in SUD treatment are feasible in low-resource and refugee settings and that brief interventions have significant potential. Opioid agonist therapy may also be possible in humanitarian settings with support from international donors and organizations. Several international policy documents have provided policy direction for addressing SUDs in humanitarian emergencies. UNODC and the World Health Organization (WHO) have a joint program for such situations, and the UNODC/WHO International Standards for Treatment of Drug Use Disorders can be adapted for humanitarian emergencies. A handbook by UNODC, UNHCR, and WHO will soon be published, disseminated, and field-tested. It creates an implementation framework for both the acute phase and the protracted phase of the emergency. It has identified two main principles — saving lives first (i.e., addressing life-threatening concerns such as overdose and withdrawal first), and full inclusion of people with SUD in other support available.
Dr. Dzmitry Krupchanka from the WHO described the organization’s approach to addressing substance use during humanitarian emergency responses. He noted that people in humanitarian settings have much higher rates of mental health problems than other populations; moreover, mental and physical health conditions are often comorbid with SUDs. Dr. Krupchanka mentioned a 2024 WHO draft resolution on strengthening mental health and psychosocial support (MHPSS) before, during, and after humanitarian emergencies. The resolution includes MHPSS as an integral component of preparedness, response, and recovery activities in all emergencies. He then reviewed the Inter-Agency Standing Committee (IASC) Guidelines on Mental Health and Psychosocial Support in Emergency Settings, which delineate key actions to minimize harm related to alcohol and other substance use. These measures aim to conduct rapid assessments, prevent harmful alcohol and other substance use and dependence, facilitate harm reduction interventions in the community, and manage withdrawal and other acute problems. An open, free course is available to learn about this, as well as some cultural adaptations. Additionally, several WHO resources to address substance use and its consequences in humanitarian settings will be forthcoming.
Dr. Karen Paul from the IASC Reference Group for Mental Health and Psychosocial Support in Emergencies talked about interagency collaboration regarding substance use and SUD in humanitarian emergencies. She emphasized that a multi-sector response is needed in such situations, and some of these sectors are also addressed in the IASC Guidelines mentioned by Dr. Krupchanka. Dr. Paul also described the IASC MHPSS Reference Group, which is cochaired by WHO, UNODC, and UNHCR and involves over 11 member agencies as well as five observers. Their current priority is developing materials for training and orienting various groups of humanitarian workers on substance use, from materials for basic communication to materials on advanced interventions. For example, orientation materials are being developed on SUDs as health conditions, how to provide support, life-threatening conditions related to substance use, and similar topics. These materials are currently being field tested, with an eye to adapting them to various cultures and contexts. A substance use module for integrating MHPSS into disaster risk management and preparedness training is also being developed, including a framework for assessment and action.
Finally, Dr. Mustafa al’Absi from the Duluth Global Health Research Institute and the University of Minnesota discussed the implications of traumatic stress and substance use in the context of humanitarian emergencies. He noted that trauma exposure, particularly in children and adolescents, is associated with an increased risk for SUD; moreover, substance use can impact the course and severity of traumatic stress symptoms. Increases in political conflicts as well as natural disasters have led to rising mental health and substance use problems. Consequently, it is important to integrate mental health and substance use services in emergency responses and increase capacity for trauma-informed care among humanitarian workers. Dr. al’Absi presented case studies from recent conflicts and natural disasters, such as the conflicts in Syria, Yemen, and Afghanistan, or the an earthquake in Turkey. Each of these has unique features regarding substance use that need to be considered. The crisis in Afghanistan also provided an example of partnerships between WHO, UNODC, and others for treatment of SUDs to improve the health and well-being of vulnerable populations. Dr. al’Absi concluded that guidelines informed by epidemiological, intervention, and implementation knowledge are needed to address the challenge of SUD in humanitarian settings. He also issued a call to action for improving substance use services capacity and integration in humanitarian emergencies in order to help prioritize the well-being of affected populations in emergency response efforts. These efforts also must address the issue of stigma in order to be effective.
Current Global Topics: Synthetic Drugs in Latin America and the Caribbean and Treating Children for Substance Use Problems
In this session moderated by Dr. Andrew Thompson from the Bureau of International Narcotics and Law Enforcement Affairs (INL), Dr. Marya Hynes from the Inter-American Observatory on Drugs reported on emerging synthetics and new psychoactive substances (NPS) in Latin America and the Caribbean. She noted that synthetic drugs have proliferated in those drug markets, particularly since 2013, and that NPS also have become more common. Of concern are amphetamine-type stimulants (ATS), such as amphetamines and prescription stimulants, methamphetamine, synthetic hallucinogens, and synthetic opioids. Information on NPS is coming from drug seizures and early warning data that are reported to the Early Warning System for the Americas. These notifications have highlighted several trends, such as appearance of “pink cocaine” (a mixture of various synthetic compounds); increasing prevalence of benzodiazepines in females in every population studied; greater presence of stimulants, particularly among females; synthetic cannabinoids; and a variety of synthetics sold as other drugs. As Dr. Hynes emphasized, even at low prevalences, these trends are concerning because they indicate that people are using more and more mixed and adulterated substances that have unknown composition, unknown effects, often high potency, and often rapid onset and long duration of effects. The most severe implications are for countries with fragile health care systems. These developments affect particularly teenagers and young adults, including health risks and mental health issues that drive drug use and are themselves driven by drug use. These developments need to inform efforts for detection, treatment, and prevention.
Dr. Antonio Pascale from the University of Montevideo further described the health impacts of NPS and increasing availability of adulterated drugs among younger users. For example, adulterants may potentiate the toxicity of the original drugs or induce unintended toxic effects. ATS are associated with harmful effects, such as hyperthermia, which can be exacerbated by the settings where these drugs are often consumed (e.g., clubs with high temperatures, poor ventilation, and intense physical activity); serotonergic syndrome; and hyponatremia. Synthetic cannabinoids, which are increasingly used by young people as well as other vulnerable populations, can be associated with acute toxicity as well as greater morbidity and mortality in the context of polydrug use. Synthetic opioids (e.g., fentanyl and its analogues, nitazenes) are an increasing problem in Latin America with the associated acute toxicity and risk of fatal overdoses. An emerging concern is illicit use of benzodiazepines (often as adulterants of other drugs), which is associated with acute intoxication and altered consciousness that increases vulnerability to sexual crimes and other harms. Dr. Pascale concluded that to address this public health problem effectively, it is essential to strengthen networks involving government agencies, clinical and forensic laboratories, universities, toxicology centers, and nongovernment agencies with access to PWUD.
Dr. Hendrée Jones from the University of North Carolina at Chapel Hill provided an overview of the Child Intervention for Living Drug-free (CHILD) program to treat children under age 12 for substance use problems. Dr. Jones explained that children are increasingly victims of drug trafficking and drug use within their families and communities, leading to children as young as 5-12 years having an SUD. The CHILD intervention was developed to respond to this growing threat. It is a global, evidence-based program with an integrated health approach, full spectrum of services, and family involvement. It includes professional development and a tailored program for each country that uses techniques (e.g., motivational interviewing, mindfulness, dialectic behavior therapy, art therapy) that can be translated into language and activities appealing to children. The program has been disseminated to numerous countries through “training of trainers,” webinars, and “Echo” training. Dr. Jones also shared information on a randomized clinical trial of the CHILD intervention in children ages 7-12 in India. Preliminary results indicate that it reduces substance use in these children at 12 months after treatment better than usual care.
Advancing Quality in Addiction Studies Programs
In this session chaired by Dr. Carly Searcy from ICUDDR, Mr. Jordan Turner, also from ICUDDR, provided an overview of the current state of quality assurance in specialty addiction studies programs. He shared results from a survey of 322 ICUDDR members that assessed program quality along six dimensions of care in members’ curricula. Among the 88 respondents, 63% addressed quality improvement through their curriculum plans. However, qualitative data indicated a minimal focus on quality of care among institutions; barriers within member institutions that prevented quality of care to be addressed, and a need for ICUDDR’s assistance in curriculum building. Mr. Turner concluded that the study positioned ICUDDR to work with members to create frameworks for addressing quality and quality improvement.
Dr. Amelie Lososová from the Charles University in Prague presented previous research on establishing quality assurance at the university level. Several organizations, such as the Substance Abuse and Mental Health Services Administration, American Society of Addiction Medicine, and American Psychiatric Association in the United States, as well as the WHO have addressed quality standards in education programs. Similarly, two projects (WAVE project and FENIQS-EU project) have been conducted in Europe, and for the WAVE project, several project papers will be published soon. Dr. Lososová noted the importance of making education a research focus and highlighted several future research questions and challenges—for example, whether international standards are really needed, what areas of addiction research should be included; which educational programs should apply the standards; and how regional, cultural, and communication differences can be accommodated.
Dr. Dennis McCarty from the Oregon Health & Science University discussed what kind of research is needed. Examples included integration of research into educational standard development, integration of outcome measures into training processes and systems of care, assessments of the impact of standards on care delivery and outcomes, and cross-national comparisons of training and training standards. Dr. McCarty pointed out that each country is unique, and it is therefore important to determine which facets of addiction studies programs are replicable and generalizable. The relatively new areas of dissemination and implementation research are also crucial to determine how research findings can be translated into standards of practice on an international scale.
In the final presentation, Dr. Victor Capoccia from the Technical Assistance Collaborative discussed the development a certification framework for addiction studies programs as a documentation of program quality. He also highlighted questions that would need to be addressed in this process. For example, what should be included in a certification according to international standards (e.g., minimum content for the curriculum, minimum hours of exposure to content, number and qualifications of faculty). Equally important is an understanding of factors that drive differences in standards across the globe. Levels of education and credentialing desired (e.g., nondegree certification, associate, baccalaureate, master’s), cultural and historical contexts of drug use, governmental/legal context, health system infrastructure, and medical traditions all need to be considered. In fact, one set of standards may not be appropriate for all international scenarios. Thus, development of any certification or accreditation process must first determine what components are nonnegotiable and must be uniform and what country or regional characteristics might be accommodated in variations from the standards.
Twenty Years of Addiction Neuroscience Research in Bulgaria: Lessons Learned and Future Directions
This session presented the results of three sets of studies conducted by a large multinational team of researchers in Bulgaria over the past 20 years. As the first presenter, Dr. Jasmin Vassileva from Virginia Commonwealth University, explained, Bulgaria is well suited for their studies of people who use opiates and stimulants because it is located on one of the main heroin trafficking routes into Europe and is a major center for amphetamine production. Dr. Vassileva is investigating which dimensions of impulsivity are associated with addiction in general or specific drug classes. Her team used computational approaches to address this question, including data-driven, machine learning processes for classification and prediction, as well as theory-driven cognitive modeling to increase precision of neurocognitive phenotyping. Dr. Vassileva presented results of several of their studies that have been published in recent years. The findings indicated that some dimensions of impulsivity are common across addictions, whereas others are unique to specific drug classes. The studies also demonstrated that computational approaches have significant potential as phenotyping tools, for identifying markers for addictions, for increasing precision of neurocognitive assessments, and for prognosis and diagnosis. They may also help refine neurocognitive phenotypes and identify different biotypes of addictions with different underlying mechanisms, which may have implications for prevention and intervention.
Dr. Elena Psederska from the New Bulgarian University presented on studies assessing the interplay of personality and neurocognitive function in stimulant and opioid use disorders. Specifically, the team studied the effects of psychopathy on neurocognitive domains of impulsivity in people who previously had used heroin and amphetamine. They assessed the effects of the interpersonal/affective domain and the impulsive/antisocial domain of psychopathy on impulsive choice and impulsive action in both groups. The analyses identified an interaction between the two dimensions of psychopathy and dependence on specific classes of drugs. Some profiles were common across SUDs, whereas others were unique for specific drugs. Another investigation assessed neurocognitive impulsivity in people who used heroin at different times of abstinence. These analyses demonstrated that while some deficits in impulsive choice and impulsive action may only occur in early abstinence, others may persist even with sustained periods of abstinence. Overall, Dr. Psederska concluded that personality influences both common and unique profiles of impairments in neurocognitive impulsivity in people who use opioids or stimulants, and that these impairments may persist even with sustained abstinence. Thus, extended treatment and rehabilitation approaches that are tailored to individual personality profiles are needed.
The final presentation by Dr. James Bjork from Virginia Commonwealth University addressed similarities and differences in brain recruitment by reward, inhibition, and memory in people with different SUDs. The study included individuals with opioid, stimulant, or polysubstance use disorder as well as controls and compared individual differences in brain activation as measured by MRI while participants were conducting three different tasks. The study is still ongoing, but there is preliminary evidence that brain areas activated by specific tasks can be correlated with characteristics determined in addictions neuroclinical assessments, such as emotion-related impulsivity, cognitive difficulties in daily life, or inattention.
Publishing Workshop: How to Get Your Addiction Research Published in Peer-Reviewed Journals
In this workshop, Adam Gordon and Casy Calver from the International Society of Addiction Journal Editors (ISAJE) highlighted three essential aspects to publishing addiction research: choosing a journal, submission and peer review, and authorship. They noted that there are over 100 peer-reviewed addiction journals, and journals from other disciplines also publish addiction articles. They listed 15 factors to consider when choosing a journal as well as questions to ask of a journal (e.g., whether it reaches the specific audience authors want to target, the journal’s mission and content area). Practical aspects, such as editorial support provided or cost of publication/open access, are also important, as are various metrics associated with the journal. These include journal metrics (e.g., impact factor), public impact metrics (e.g., Altmetric attention score), editorial efficiency metrics (e.g., time to first decision, acceptance rate), and author metrics.
For submitting manuscripts, authors first need to check author guidelines, select an appropriate article type, and obtain approval from all authors to submit. Once a manuscript is submitted, a multistep peer-review process follows that may include one or more author revision steps. Peer review is essential to advise the editorial decision-making process, justify rejections, improve the quality of acceptable manuscripts, and identify instances of ethical or scientific misconduct. While the reviewers make recommendations, the editors make the final decision and may even disagree with reviewer recommendations. If the decision is to revise and resubmit the paper, authors should decide if they want to resubmit the paper and, if so, respond to each criticism by either modifying the manuscript or debating/refuting the criticism.
The issue of authorship is increasingly important. The authors on a manuscript certify a public responsibility for the truth of the publication. Authorship also is an indicator of productivity, promotion, and prestige, and credit should be assigned equitably. Especially in addiction research, inclusion of people with lived experience (e.g., patient coauthors in case studies) is important but authors also need to be aware of and sensitive to ethical considerations. Several measures can be taken to avoid authorship problems (e.g., early agreement on the precise roles of all contributors; periodic review of authorship credit status, and adherence to authorship guidelines). Overall, authors must avoid the “seven deadly sins” of carelessness, redundant publication, unfair authorship, undeclared conflict of interest, human subjects’ violations, plagiarism, and other fraud. To avoid plagiarism, all sources must be appropriately acknowledged and permission for use of large amounts of others’ written or illustrative materials obtained. This also applies to self-plagiarism, as authors are not allowed to reuse previously published materials when rights have been assigned to the publisher. The ISAJE provides author resources on their website ( https://www.isaje.net/ ); additional information is available in the JAMA Users’ Guide to Medical Literature and from the Committee on Publication Ethics (COPE) ( https://publicationethics.org ).
Thank you for visiting nature.com. You are using a browser version with limited support for CSS. To obtain the best experience, we recommend you use a more up to date browser (or turn off compatibility mode in Internet Explorer). In the meantime, to ensure continued support, we are displaying the site without styles and JavaScript.
- View all journals
- Explore content
- About the journal
- Publish with us
- Sign up for alerts
- Perspective
- Published: 01 February 2001
Drug Addiction, Dysregulation of Reward, and Allostasis
- George F Koob Ph.D 1 &
- Michel Le Moal MD, Ph.D 2
Neuropsychopharmacology volume 24 , pages 97–129 ( 2001 ) Cite this article
78k Accesses
2047 Citations
204 Altmetric
Metrics details
This paper reviews recent developments in the neurocircuitry and neurobiology of addiction from a perspective of allostasis. A model is proposed for brain changes that occur during the development of addiction that explain the persistent vulnerability to relapse long after drug-taking has ceased. Addiction is presented as a cycle of spiralling dysregulation of brain reward systems that progressively increases, resulting in the compulsive use and loss of control over drug-taking. The development of addiction recruits different sources of reinforcement, different neuroadaptive mechanisms, and different neurochemical changes to dysregulate the brain reward system. Counteradaptive processes such as opponent-process that are part of normal homeostatic limitation of reward function fail to return within the normal homeostatic range and are hypothesized to form an allostatic state. Allostasis from the addiction perspective is defined as the process of maintaining apparent reward function stability by changes in brain reward mechanisms. The allostatic state represents a chronic deviation of reward set point and is fueled not only by dysregulation of reward circuits per se, but also by the activation of brain and hormonal stress responses. The manifestation of this allostatic state as compulsive drug-taking and loss of control over drug-taking is hypothesized to be expressed through activation of brain circuits involved in compulsive behavior such as the cortico-striatal-thalamic loop. The view that addiction is the pathology that results from an allostatic mechanism using the circuits established for natural rewards provides a realistic approach to identifying the neurobiological factors that produce vulnerability to addiction and relapse.
Similar content being viewed by others
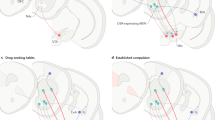
The transition to compulsion in addiction
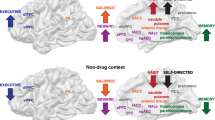
The neurobiology of drug addiction: cross-species insights into the dysfunction and recovery of the prefrontal cortex
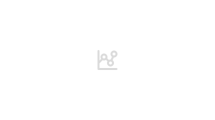
Addiction is driven by excessive goal-directed drug choice under negative affect: translational critique of habit and compulsion theory
Drug addiction is a chronically relapsing disorder that is defined by two major characteristics: a compulsion to take the drug with a narrowing of the behavioral repertoire toward excessive drug intake, and a loss of control in limiting intake ( American Psychiatric Association 1994 ; World Health Organization 1992 ). An important challenge for neurobiological research is to understand the neuroadaptive differences between controlled drug use and loss of control, and by extension, the molecular, cellular and system processes that lead to addiction ( Koob and Le Moal 1997 ).
Animal models are critical for understanding the neuropharmacological mechanisms involved in the development of addiction. While there are no complete animal models of addiction, animal models do exist for many elements of the syndrome. These elements can be derived from symptoms or diagnostic criteria for addiction or conceptual frameworks such as different sources of reinforcement ( American Psychiatric Association 1994 ; World Health Organization 1992 ). Linking neuropharmacological events to animal models can occur at multiple levels of analysis — molecular, cellular and system — and it will only be the integration of these changes across dimensions of analysis that will allow a full understanding of the neurobiology of drug addiction.
Advances in neuroscience research are rapidly unraveling the complexity of processes underlying addiction in general and of drug abuse in particular. Assuming that the reductionist approach is useful in bringing about precise knowledge concerning biologic aspects of addiction, new conceptual frameworks will be required to understand what has been labeled as “the quintessential biobehavioral disorder” ( Leshner 1999 ), a disorder deeply imbedded with psychosocial vulnerability as well as genetic vulnerability ( Koob and Le Moal 1997 ). Such an integrated pathogenic view of addiction has profound implications for the treatment of drug abuse, and the best therapeutic approaches include social, behavioral and biological aspects, in combination with pharmacotherapies (Baxter et al. 1992 , 1996 ; McLellan et al. 1993 ; O'Brien 1997 ; Project MATCH Research Group 1997 ; Schwartz 1999 ).
Drugs of abuse act at local cellular-membrane sites, within neurochemical systems that are part of a reward system neurocircuitry. These systems include the dopamine and opioid peptide networks which have many different projection sites. The midbrain dopamine systems have critical roles not only in the reward and motor systems but also in higher-order functions, including cognition and memory ( Grant et al. 1996 ). Opioid peptides have been implicated in pain and emotional processing throughout the neuraxis.
The mechanisms and processes leading from an initial cellular action of drugs of abuse to an action within reward circuits and beyond require a psychobiological integration that parallels the processes leading from use, to misuse, to abuse and dependence and vulnerability to relapse. Addicted patients are gripped by unusual emotional states, they compulsively have urges to abuse and they are remarkably unencumbered by the memory of negative consequences of drug-taking ( Childress et al. 1999 ). These clinical states are hypothesized to be mirrored by a “brain signature” ( Nestler and Aghajanian 1997 ), and modern imaging technologies help to see how such complex constructs, as compulsion to use drugs or compulsion to engage in repetitive dysfunctional behaviors, are implemented into brain networks including large and complex circuitries of the brain reward and emotional systems ( Childress et al. 1999 ; Volkow et al. 1999 ). Other areas of psychobiological integration include the translation of animal research advances in the neurobiology of addiction to useful clinical studies and the development of conceptual frameworks that integrate both domains. The present review will examine the neurobiological mechanisms of addiction with a focus on dysregulation of the brain reward system and allostasis ( Koob and Le Moal 1997 ). Emphasis will be placed on integrating across the different levels of analysis in an attempt to identify what is known and what remains to be elucidated.
ADDICTION CYCLE
Drug addiction is not a static phenomenon, and as with other biobehavioral dysregulation, such as compulsive gambling and binge eating, there are different components that constitute a cycle or circle of ever-growing pathology ( Baumeister et al. 1994 ). Derived from social psychology and conceptualized as sources of self-regulation failures, the addiction cycle has been described as having three components: preoccupation-anticipation, binge-intoxication, and withdrawal-negative affect ( Koob and Le Moal 1997 ). Spiralling distress describes how, in some cases, the first self-regulation failure can lead to emotional distress, which sets up a cycle of repeated failures to self-regulate, and where each violation brings additional negative affect ( Baumeister et al. 1994 ). Spiralling distress also has been described as the progressive dysregulation of the brain reward system within the context of repeated addiction cycles ( Figure 1 ). Psychiatric and experimental psychological constructs address the same addiction cycle, and animal models have been established and validated for different symptoms or constructs associated with elements of the addiction cycle, addiction criteria, and sources of reinforcement associated with addiction ( American Psychiatric Association 1994 ; Koob 1995 ; Koob et al. 1998a ; Markou et al. 1993 ).
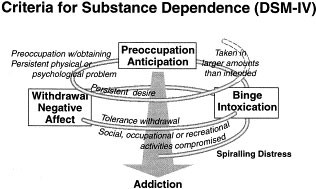
Diagram describing the spiralling distress/addiction cycle from a psychiatric perspective with the different criteria for substance dependence from the Diagnostic and Statistical Manual of Mental Disorders , 4th edition, incorporated at different stages. There are three major components of the addiction cycle: preoccupation-anticipation, binge-intoxication, and withdrawal-negative affect. From an experimental psychology perspective, different sources of reinforcement correspond to different components of the addiction cycle with the binge-intoxication component largely associated with positive reinforcement and the withdrawal-negative affect component largely associated with negative reinforcement. Conditioned positive and conditioned negative reinforcement would be hypothetically associated with the preoccupation-anticipation component. The theoretical constructs of psychomotor sensitization and counteradaptation also vary with the stage of the cycle, with psychomotor sensitization probably having a greater role in the preoccupation-anticipation stage and counteradaptation having a greater role in the withdrawal-negative affect component ( Koob and Le Moal 1997 ). Similarly, the hypothetical role of different neurochemical/endocrine systems in the addiction cycle would fall into different components. The binge-intoxication component is reflected by activation of dopaminergic and opioid peptide systems, and the withdrawal-negative affect component is dominated by decreases in dopamine and opioid peptide function and increases in brain stress systems such as CRF. Note that the addiction cycle is conceptualized as a spiral that increases in amplitude with repeated experience, ultimately resulting in the pathological state known as addiction. (Taken with permission from Koob and Le Moal 1997 .)
Superimposed on the addiction cycle are multiple sources of reinforcement that can contribute to compulsive use of drugs of abuse during the course of drug addiction. Positive reinforcement occurs when presentation of the drug increases the probability of a response to obtain the drug again. The positive reinforcing effects of drugs when described in the context of reward often are equated with the pleasurable effects of drugs in the absence of a deficit state. Negative reinforcement, in contrast, occurs with alleviation of an existing aversive state or alleviation of a drug-generated aversive state (e.g., withdrawal) ( Wikler 1973 ). Secondary positive reinforcing effects can be obtained through conditioned positive reinforcement (e.g., pairing of previously neutral stimuli with acute positive reinforcing effects of drugs). Secondary negative reinforcing effects can be obtained through removal of the conditioned negative reinforcing effects of conditioned abstinence. Positive reinforcement as a construct is associated largely with the binge intoxication stage outlined in Figure 1 , and the construct of negative reinforcement is associated largely with the negative affect/withdrawal stage. Conditioned positive reinforcement and conditioned negative reinforcement can be conceptualized to contribute to the preoccupation/anticipation stage.
The neurobiological bases for the acute positive reinforcing effects of drugs of abuse, the negative reinforcing effects imparted by the dependent state, and the conditioned reinforcing effects associated with protracted abstinence (defined as a state of residual reward dysregulation after acute withdrawal) and relapse have provided significant insight into the building blocks of addiction ( Koob et al. 1993 ). However, linking the animal models to the different components of the criteria used to diagnose substance dependence in humans requires a conceptual framework that will have heuristic value for explaining compulsive use and loss of control that are not inherent components of any one source of reinforcement. A transition occurs between limited access, controlled drug use (e.g., social drinking), and the loss of control and compulsive use of alcohol that constitutes alcoholism and to date few conceptual frameworks have bridged that gap. The hypothesis under exploration here is that drug addiction not only involves changes in the neural substrates of positive reinforcement but that other neuroadaptive systems are recruited, notably in the domains of reward and stress to produce changes in motivational systems. Such changes are hypothesized to contribute to an allostatic state that renders the organism vulnerable to the spiralling distress and pathology of addiction (see below).
Stress has been defined historically as responses to demands (usually noxious) upon the body ( Selye 1936 ), or in a definition more focused on the central nervous system, as alterations in psychological homeostatic processes ( Burchfield 1979 ). A state of stress is associated with various external and internal challenges to the body and brain, usually termed stressors, and the construct of stress may represent the extreme pathological continuum of overactivation of the normal activational (arousal) or emotional systems of the body ( Hennessy and Levine 1979 ). Such arousal-activational mechanisms trigger biological and behavioral strategies of coping and control that mobilize many organismic and central nervous system mechanisms, whose failure leads to illness ( McEwen 1998a ; Schulkin et al. 1994 ; Sterling and Eyer 1981 ). The state of stress is reflected biologically by various physiological changes that include an activation of the pituitary-adrenal axis and release of glucocorticoids into the bloodstream ( Stanford and Salmon 1993 ), activation of the sympathetic nervous system, and activation of brain emotional systems.
Pituitary-adrenal axis activation is triggered by the release of adrenocorticotropic hormone (ACTH) from the pituitary. ACTH release is in turn controlled by the liberation of hypothalamic corticotropin-releasing factor (CRF) into the pituitary portal system of the median eminence. Extrahypothalamic CRF systems are involved in the adaptive autonomic nervous system, and behavioral changes are another major component of the response to bodily demands or challenges to homeostasis ( Koob et al. 1993 ). Evidence demonstrating a neurotropic role for CRF in the central nervous system outside the pituitary-adrenal axis suggests a parallel means for mediating autonomic and behavioral responses to stressors and a contribution to the behavioral state of stress in addition to the classic activation of adrenal steroids (Figures 2A , and 2B ; for a more integrated review, see below and Figure 3 ).
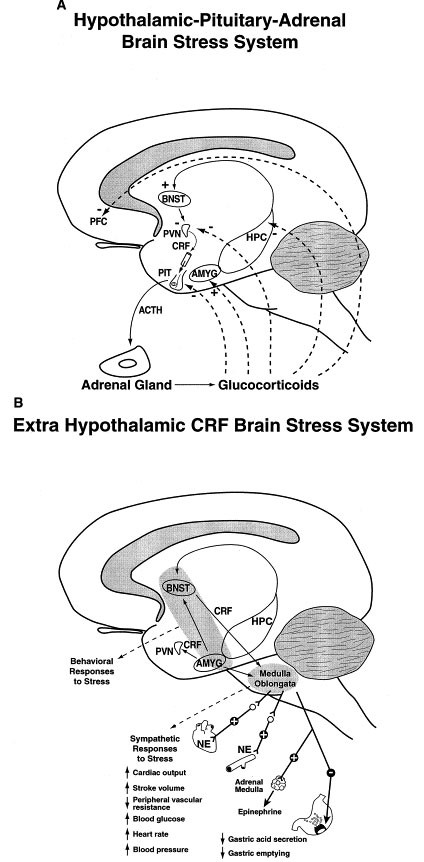
Diagrams illustrating the central role of corticotropin-releasing factor in the (A) hypothalamic-pituitary-adrenal stress axis, and (B) the brain stress systems. The functional interactions between glucocorticoids, CRF and stress represent an exquisitely balanced stress system. (A) Hypothalamic-pituitary-adrenal stress system. Stressful stimuli increase CRF which in turn stimulates ACTH release from the pituitary which results in enhanced release of glucocorticoids from the adrenal gland. High levels of glucocorticoids, through negative feedback, decrease CRF synthesis at the level of the PVN but activate CRF activity at the level of the central nucleus of the amygdala. (B) Extrahypothalamic CRF stress system. Stressful stimuli also activate CRF systems in the basal forebrain, notably the bed nucleus of the stria terminalis and the central nucleus of the amygdala to help mediate behavioral responses to stressors and to mediate sympathetic activation associated with stressors. Glucocorticoids, instead of exerting a feedback suppression of CRF synthesis in the central nucleus of the amygdala, actually increase synthesis of CRF providing a means for extending the contribution of the brain stress systems to allostasis (see text). ACTH, adrenocorticotropic hormone; AMYG, amygdala; BNST, bed nucleus of the stria terminalis; CRF, corticotropin-releasing factor; HPC, hippocampus; NE, norepinephrine; PIT, pituitary gland; PFC, prefrontal cortex; PVN, paraventricular nucleus.
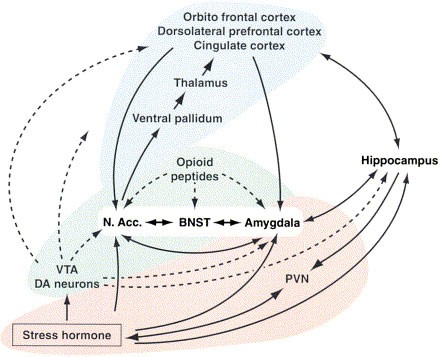
Circuit diagram illustrating the hypothesized extended amygdala reward system as a focal point for allostasis in addiction and the interaction of two major brain circuits that help perpetuate the allostatic states in the brain reward system. Elements of the nucleus accumbens, bed nucleus of the stria terminalis and central nucleus of the amygdala have cytoarchitectual, circuit and functional similarities that have been hypothesized to form a key component of what has been conceptualized as a brain reward circuit. Critical, but not exclusive, modulatory neurochemical components of the brain reward circuit include the mesolimbic dopamine system and opioid peptides. Fueling dysregulation within the brain reward circuit are the HPA stress axis and brain stress systems, and expressing and perpetuating the dysregulation of reward in the form of compulsive behavior is the cortico-thalamic-striatal loop circuit ( Swerdlow and Koob 1987 ). BNST, bed nucleus of the stria terminalis; DA, dopamine; N. Acc., nucleus accumbens; PVN, paraventricular nucleus; VTA, ventral tegmental area
As noticed since the beginning of the stress concept ( Selye 1976 ), as the process goes along (as coping, adjustment and successful adaptations fail), more and more neuroendocrine and neurobiological systems are recruited. The hormones that are beneficial when contained within the homeostatic range are a source of pathophysiological cascades when they remain persistently elevated due to a breakdown of negative feedback or a failure of feedback regulation of CRF and ACTH secretion. Ultimately, various peripheral and central pathological effects are hypothesized to ensue, such as an imbalance in noradrenergic systems which may enhance anxiety ( Chrousos and Gold 1992 ), neural atrophy in the hippocampal CA3 region with cognitive consequences, and various endocrine and systemic disorders (e.g., elevated insulin, increased blood pressure, and so on) (for review see McEwen 1995 ).
Homeostasis , in principle, corresponds to the mechanisms that maintain stability within the physiological systems and hold all the parameters of the organisms internal milieu within limits that allow an organism to survive ( Bernard 1865 ; Cannon and Rosenblueth 1933 ; Sterling and Eyer 1988 ). It implied originally that i) deviations from normal set points are automatically corrected by local negative feedbacks, and ii) bodily organs are considered as functioning autonomously. Subsequently, homeostasis has been described as a self-regulating process for maintaining body parameters around a set point critical for survival ( McEwen 2000 ). This includes multi-system coordination of the organism's response to an acute challenge, including the brain, pituitary, autonomic system, and skeleto-motor systems. However, while some of the parameters of the internal milieu are held constant (like body temperature), other parameters like stress hormones are varied within a wide range in an attempt to maintain homeostasis.
In contrast, the principle of allostasis proposes maintenance of stability outside of the normal homeostatic range, where an organism must vary all the parameters of its physiological systems to match them appropriately to chronic demands (e.g., reset the system parameters at a new set point) ( Sterling and Eyer 1988 ). Allostasis refers to the integrative adaptive processes maintaining stability through change, a stability that is not within the normal homeostatic range. It implies that many, if not all, physiological functions are mobilized or suppressed, as reflected in a cascade of brain-organism interactions overriding local regulation. By controlling all the mechanisms simultaneously, the brain can enforce its command and introduce experience, memories, anticipation and re-evaluation of needs in anticipation of physiological requirements.
The allostatic model, because it involves the whole brain and body instead of simply local feedbacks, is far more complex than homeostasis. All parameters of a given domain (e.g., blood pressure, or in the central nervous system reward function) are controlled by numerous mutually interacting signals. When demands become chronic, the brain-body system tonically adapts at essentially all levels of organization implying widespread changes in set points, and entry into a relaxed condition may create an unpleasant state of withdrawal from one's physiological regulation. Such changes in hormones, opioids, transmitters, and so on, provide a physiological basis for the individual to continue to seek a condition of high demand ( Sterling and Eyer 1988 ), and a stabilized new level of activity far from homeostatic equilibrium. However, when chronic arousal, repeated stress and negative affective states impose prolonged regulations far from normality, there is no margin left for responding to additional challenges, no opportunity for relaxation, and no capacity for more responsiveness. This stabilized new level of activity far from homeostatic equilibrium forms an allostatic state. An allostatic state can be defined as a state of chronic deviation of the regulatory systems from their normal state of operation with establishment of a new set point.
This chronic stress and chronic arousal has been hypothesized to lead to breakdown, damaging consequences, and illness ( Sterling and Eyer 1981 ). Allostatic load as defined by McEwen and Stellar (1993) refers to the cost or the price the body may have to pay for being forced to adapt to an adverse or deleterious psychological or physical situation, and it represents the presence of too much demand on the operation of the regulatory systems — mainly the primary mediators of the physiological response — or their failure to relax when the demand is over. Different types of allostatic load have been considered that may explain different types or gradients of morbidity ( McEwen 1998a ). Whatever the modality, the process is translated into a different structural-functional state (i.e., a vulnerable phenotype).
Thus, a simple definition of allostasis is a process of maintaining stability, or “apparent stability,” through change but at a price ( Sterling and Eyer 1988 ). With continuing demand, the flexibility to maintain stability through allostatic maladaptations decreases, leading to breakdown and illness. Here, drug addiction is hypothesized to involve a change in drug reward set point and reflects an allostatic, rather than a homeostatic, adaptation (i.e., outside the normal set point). Thus, in the addiction domain to be elucidated here, the stability to be maintained is stability in reward function and the change is the mobilization of multiple neurotransmitter and hormonal systems needed to maintain normal reward function. Reward neurotransmitters such as dopamine and opioid peptides are hypothesized to have a limited capacity to maintain reward function within homeostatic range.
The acute effects of initial drug intake and consequent glucocorticoid activation can extend this capacity but also can trigger the beginnings of counteradaptive mechanisms such as neuroadaptation within the dopamine and opioid peptide systems (presynaptic to postsynaptic to transduction mechanisms), and the recruitment of opposing systems such as activation of brain stress systems such as corticotropin-releasing factor and norepinephrine. Opponent-process counteradaptation is defined here as the opposing of the initial hedonic effects of a drug or stimulus by a slowly developing counteracting process that becomes larger over time and masks the initial hedonic effects of the drug or stimulus ( Solomon and Corbit 1974 ). These counteradaptive mechanisms are hypothesized to form the driving force of a reward system allostatic state (increase in reward set point; see below). Carrying such a state of allostasis would be hypothesized to reflect both genetic and environmental factors that, when combined, render the individual vulnerable to entry into any one of a number of components of the addiction cycle. Thus, multiple brain and hormonal mechanisms can combine to produce the allostatic state that underlies the severe pathology of the “addicted” state.
Drawing from recent conceptualizations about allostasis, anxiety and negative affective states do not have a simple set point that is maintained and regulated ( Schulkin et al. 1998 ). Chronic elevation of stress axis reactivity and central CRF, its gene expression in the amygdala, and loss of gene inhibition in the paraventricular nucleus by glucocorticoids beyond homeostatic regulation, may represent a condition of allostasis within the brain/hormonal stress system. Similar stress-like conditions also are found in drug dependence, withdrawal and craving for psychoactive agents where both CRF expression and cortisol levels are increased and may contribute to the allostatic state hypothesized to drive drug addiction. Such a chronic stress-like contribution to allostasis can extend also to other systems in the form of pathology to compromise sleep, feeding, immune function, and so on. Thus, for the purposes of this review, the following definitions apply: allostasis , the process of achieving stability through change ( Sterling and Eyer 1981 ); allostatic state , a state of chronic deviation of the regulatory system from its normal (homeostatic) operating level; allostatic load , the cost to the brain and body of the deviation, accumulating over time, and reflecting in many cases pathological states and accumulation of damage ( McEwen and Stellar 1993 ).
NEUROBIOLOGICAL MECHANISMS FOR THE POSITIVE REINFORCING EFFECTS OF DRUGS
To understand how the brain reward systems are changed with the development of addiction, one must understand the neurobiological bases for drug reward, and there have been major advances in this domain of research. One of the principle focuses of research on the neurobiology of the positive reinforcing effects of drugs of abuse has been the origins and terminal areas of the mesocorticolimbic dopamine system, and there is now compelling evidence for the importance of this system in drug reward ( Le Moal and Simon 1991 ). The major components of this drug reward circuit are the ventral tegmental area (the site of dopaminergic cell bodies), the basal forebrain (the nucleus accumbens, olfactory tubercle, amygdala, and frontal and limbic cortices), the dopaminergic connection between the ventral tegmental area and the basal forebrain, and opioid peptide neurons within these circuits. Other components are the many neural inputs and outputs that interact with the ventral tegmental area and the basal forebrain utilizing GABA, glutamate, and serotonin as neurotransmitters ( Koob 1992 ).
The dopamine projections that modulate forebrain and cortical regions enable in normal conditions the integration of the functioning of these areas, some of these coordinated in parallel modular organizations ( Le Moal 1995 ). At the core of these interrelations and imbalances ( Figure 3 ), two related sets of structures are considered: the mesolimbic-accumbens-amygdaloid complex, with an emphasis on the extended amygdala and accumbens shell ( Alheid and Heimer 1988 ; Heimer et al. 1991 ; Koob 1999b ), and the cortico-frontal-cingulate complex related to a striatal-pallidal-thalamic circuitry ( Figure 3 ). Dopamine projections to the first set of structures are functionally involved in the acute reinforcing effects of psychostimulants, and neurochemical changes associated with the motivational aspects of drug withdrawal may involve neuroadaptations within the extended amygdala ( Koob 1999b ; Di Chiara 1999 ).
Drug-seeking behavior under the control of reinforcing processes that result from conditioned or second-order schedules of reinforcement may depend ultimately on this extended amygdala circuit and involve basolateral cortical projections to the core of the nucleus accumbens which is anatomically more related to striatal-pallidal circuitry ( Everitt et al. 1999 ). Note that the central nucleus of the amygdala is connected with hypothalamic and brain-stem regions included in autonomic and consummatory responses, while the cortico-striatal-thalamic circuitry is comprised of regions implicated in motor learning, reinforcer evaluation (nucleus accumbens) or stimulus-reward associations ( Jentsch and Taylor 1999 ). The cortico-striatal-thalamic circuitry is related more to cognitive functioning, to active inhibitory central mechanisms, drive and compulsive repetitive behaviors. Activation of these regions as visualized by imaging techniques in drug abuse during intense craving ( Volkow and Fowler 2000 ) is the same as those visualized in obsessive-compulsive disorder (Baxter et al. 1992 , 1996 ; Graybiel et al. 1994 ; Modell et al. 1989 ; Schwartz 1997 ).
Compulsive disorders and anxiety may in some cases point to a similar phenotype but via different origins. In anxiety associated with drug dependence, anticipatory angst and negative affect may trigger compulsive searching for the drug but the sequence might be different in other pathological conditions, such as obsessive compulsive behavior. Important points to consider are that these two structural-functional sets are connected and second that they are both modulated by dopamine ( Le Moal 1995 ). Moreover, it is well documented that mesocortical dopamine and mesolimbic dopamine neurons have different physiological regulation, dopamine utilization in the nucleus accumbens being under the control of a cortical-accumbens excitatory pathway (for review see Le Moal and Simon 1991 ). Specific drugs may enter into this neurocircuitry at different points and via different molecular/cellular mechanisms ( Table 1 ).
Psychomotor Stimulants
Psychomotor stimulants of high abuse potential interact initially to block monoamine transporter proteins which are located on monoaminergic nerve terminals. Cocaine inhibits all three monoamine transporters — dopamine, serotonin, and norepinephrine — thereby, potentiating monoaminergic transmission. Amphetamine and its derivatives also potentiate monoaminergic transmission by blocking reuptake and also by increasing monoamine release. Amphetamine itself is transported into monoaminergic nerve terminals by all three transporters, where it produces a reverse transport of the monoamine into the synaptic cleft via the monoamine transporters ( Rudnick and Clark 1993 ).
The dopamine system appears to be the critical substrate for both the psychomotor stimulant effects of amphetamine and cocaine and their reinforcing actions since in studies of intravenous self-administration and studies of conditioned place preference dopamine receptor antagonists, when injected systemically, reliably decrease the reinforcing effects of cocaine and amphetamine self-administration in rats and block conditioned place preferences for these drugs ( Beninger and Hahn 1983 ; Beninger and Herz 1986 ; Ettenberg et al. 1982 ; Morency and Beninger 1986 ; Phillips and Fibiger 1987 ; Yokel and Wise 1975 ). All three dopamine receptor subtypes have been implicated in the reinforcing actions of cocaine as measured by intravenous self-administration including the D1 ( Koob et al. 1987 ), D2 ( Bergman et al. 1990 ; Woolverton and Virus 1989 ), and D3 receptors ( Caine and Koob 1993 ). Dopamine D1 and D2 antagonists also block the place conditioning produced by amphetamine ( Beninger et al. 1989 ; Leone and Di Chiara 1987 ). The specific anatomical site for the rewarding action of cocaine and amphetamine appears to be the mesocorticolimbic dopamine system since dopamine lesions and microinjection of dopamine antagonists into the terminal regions of the mesocorticolimbic, but not nigrostriatal, dopamine system block cocaine and amphetamine reinforcement ( Maldonado et al. 1993 ; Roberts et al. 1977 , 1980 ).
The acute reinforcing properties of opiates in nondependent animals are blocked by systemic and central administration of competitive opiate antagonists ( Ettenberg et al. 1982 ; Goldberg et al. 1971 ; Koob et al. 1984 ; Vaccarino et al. 1985 ; Weeks and Collins 1976 ). Pharmacological studies have shown that the mu opioid receptor subtype appears to be particularly important for the reinforcing actions of heroin and morphine ( Negus et al. 1993 ), and knockout mice without the mu receptor fail to show morphine-induced analgesia or morphine-induced conditioned place preference ( Matthes et al. 1996 ). The sites of action for opioid antagonists to block the reinforcing effects of opiates appears to be associated with the same neural circuitry associated with psychomotor stimulant reward ( Table 1 ). Much data suggest that neural elements in the region of the ventral tegmental area and the nucleus accumbens are responsible for the reinforcing properties of opiates and that there are both dopamine-dependent and dopamine-independent mechanisms of opiate action ( Pettit et al. 1984 ; Shippenberg et al. 1992 ; Spyraki et al. 1983 ; Stinus et al. 1989 ).
Alcohol and Sedative Hypnotics
Alcohol and other sedative-hypnotics, such as barbiturates and benzodiazepines, all produce a characteristic euphoria, disinhibition, anxiety reduction, sedation, and hypnosis. There is much evidence implicating dopamine in the reinforcing actions of low, nondependence-inducing doses of alcohol. Dopamine receptor antagonists have been shown to reduce lever-pressing for alcohol in nondeprived rats ( Pfeffer and Samson 1988 ), and extracellular dopamine levels also have been shown to increase in nondependent rats orally self-administering low doses of alcohol ( Weiss et al. 1992a ). However, virtually complete 6-hydroxydopamine denervation of the nucleus accumbens failed to alter voluntary responding for alcohol ( Rassnick et al. 1993c ) suggesting that dopamine-independent, neurochemical systems likely contribute critically to the mediation of alcohol's reinforcing actions.
The sedative and anti-punishment (anxiolytic) effects of sedative-hypnotics are associated with facilitation of the GABA A receptor and/or inhibition of the NMDA glutamate receptor ( Hoffman et al. 1989 ; Lovinger et al. 1989 ; Richards et al. 1991 ). GABAergic antagonists also reverse many of the behavioral effects of alcohol that are associated with intoxication ( Frye and Breese 1982 ; Liljequist and Engel 1982 ). The partial inverse benzodiazepine agonist RO 15-4513, which has been shown to reverse some of the behavioral effects of alcohol ( Suzdak et al. 1986 ), produces a dose-dependent reduction of oral alcohol (10%) self-administration in rats ( June et al. 1992 ; Rassnick et al. 1993a ; Samson et al. 1987 ).
With central microinjection studies, potent GABA antagonists microinjected into the brain produced their most effective blockade when microinjected into the central nucleus of the amygdala ( Hyytia and Koob 1995 ). Alcohol also appears to interact with specific serotonergic receptor systems, notably serotonin-1A, serotonin-2 and serotonin-3 ( Eckardt et al. 1998 ; Roberts et al. 1998 ). Opioid receptor antagonists also decrease alcohol self-administration, and as a result alcohol long has been hypothesized to activate opioid peptide systems. Mice bearing knockout of the mu opioid receptor do not drink alcohol ( Roberts et al. 2000b ). The brain sites for such interactions are likely to be in the ventral tegmental area and basal forebrain ( Gatto et al. 1994 ; Heyser et al. 1999 ). Thus, multiple neurotransmitters combine to contribute to the reward profile of alcohol ( Engel et al. 1992 ).
Nicotine is a direct agonist at nicotinic acetylcholine receptors which are widely distributed throughout the brain. The nicotinic receptors mainly implicated in the reinforcing actions of nicotine again are localized to the brain mesocorticolimbic dopamine system (Corrigall et al. 1992 , 1994 ; Malin et al. 1993 ; Pontieri et al. 1996 ). However, peptide antagonists can interact with the nicotine withdrawal syndrome in rats ( Malin et al. 1993 , 1994 ; Watkins et al. 2000 ). Thus, nicotine may alter function in both the mesocorticolimbic dopamine system and opioid peptide systems in the same neural circuitry associated with other drugs of abuse ( Corrigall et al. 1992 ).
Tetrahydrocannabinol
The major psychoactive ingredient in marijuana, tetrahydrocannabinol (THC), is a drug of abuse and dependence ( Anthony et al. 1994 ). A major initial site of THC binding is the cannabinoid-1 receptor which is widely distributed throughout the brain, but it is particularly concentrated in the extrapyramidal motor system of the rat ( Herkenham et al. 1990 ). Evidence for acute reinforcing effects of THC comes from studies of brain stimulation reward, place preference and intravenous self-administration. Reward thresholds are decreased by THC administration in rats upon acute administration ( Gardner et al. 1988 ; Lepore et al. 1996 ), and THC also produces a place preference ( Lepore et al. 1995 ). THC increases dopamine in the shell of the nucleus accumbens similar to that observed with other major drugs of abuse ( Tanda et al. 1997 ). In addition, a recent study in mice has shown intravenous self-administration of a synthetic THC analog ( Fratta et al. 1997 ), and intravenous self-administration of THC in monkeys ( Tanda et al. 2000 ). One substrate hypothesized for the acute reinforcing effects of THC is activation of the mesocorticolimbic dopamine system ( Chen et al. 1991 ), and recent data suggest that THC can selectively increase the release of dopamine in the shell of the nucleus accumbens as do other drugs of abuse ( Tanda et al. 1997 ).
DRUG REWARD AND ALLOSTASIS
Individual differences and reward.
The nature of impact of the acute effects of drugs of abuse on neuronal circuits of reinforcement can contribute to the subsequent neuroadaptations that form an allostatic state in the brain reward systems. Individual differences, either via genetic or environmental factors, at critical periods may cause a predisposition to initially self-administer drugs of abuse, and a large body of evidence exists from an experimental approach to the study of individual vulnerability to drug reward and by extrapolation to addiction (for review, see Piazza and Le Moal 1996 ; Piazza et al. 1998 ). The fulfillment of two conditions is needed: the subjects should have equal access to the drug under identical environmental circumstances, and their biobehavioral features should be characterized before exposure to the drug. Individual differences in the propensity to develop drug intake have been demonstrated readily in the laboratory rat ( Deminiere et al. 1989 ; Piazza et al. 1989 ) as well as in the propensity to manifest many other adaptive biological responses ( Hooks et al. 1994a ; Piazza et al. 1989 , 1993 ).
A now classic operational design to identify individual differences is to differentiate animals on the basis of their reactivity to a stressful event (for instance their locomotor reactivity to novelty) and divide them into high reactive (HR) and low reactive (LR). The propensity of HRs to develop drug intake, compared to LRs, has been correlated with other drug-dependent responses, administered either peripherally or centrally within the mesolimbic region ( Exner and Clark 1993 ; Hooks et al. 1991 , 1992a , b , c ; Piazza et al. 1989 , 1991a ). HR rats, independent of drug administration, show an increase of dopamine utilization in the nucleus accumbens and a decrease in the prefrontal cortex ( Piazza et al. 1991b ), a lower number of dopamine D2 receptors and an opposite change in D1 receptor binding ( Hooks et al. 1994b ). HR animals also have an increased reactivity of the stress axis.
Levels of corticosterone two hours after exposure to a stressor are positively correlated with the amount of drug consumed when it is presented for the first time to the subject (acquisition). In addition, the hormone secretion lasts longer in HRs. Finally, the levels of the stress hormone before drug administration are correlated positively with the extent of self-administration ( Goeders and Guerin 1994 ; Piazza et al. 1991a ). In summary, a vulnerable phenotype, whatever the origin, inherent and/or acquired through life experience, implies intersystemic and interrelated changes at central and peripheral levels with new drug reward set points. Recent systematic studies from different models, including history of drug intake, response to novelty, and response to stressors, have lead to the demonstration of increased drug intake across the full dose-effect function ( Ahmed and Koob 1998 ; Deroche et al. 1997 ; Piazza et al. 2000 ; Rouge-Pont et al. 1993 , 1995 ).
Much research has been devoted to the interactions between stress and drug intake, and there are clear interactions between stress, glucocorticoids and mesocorticolimbic dopaminergic neurons, and between dopaminergic neurons and vulnerability to drugs of abuse. Glucocorticoid receptors are localized in brain monoaminergic neurons, particularly in the ventral tegmental area ( Härfstrand et al. 1986 ), and these receptors have pivotal regulatory roles in many regions of the brain ( De Kloet 1991 ; Joels and de Kloet 1992 , 1994 ). Direct cellular interactions between stress hormones and dopamine neurons have been difficult to document; however, glucocorticoids can interact with dopamine reward circuitry in the basal forebrain that may be independent of direct glucocorticoid/dopamine interactions. More specifically, glucocorticoids modulate the transmission of the neuropeptides dynorphin, enkephalin, tachykinin, and neurotensin, especially in the basal ganglia and nucleus accumbens ( Ahima et al. 1992 ; Chao and McEwen 1990 ; Schoffelmeer et al. 1996 ; for review, see Angulo and McEwen 1994 ).
Increased corticosterone secretion or a higher sensitivity to the central effects of the hormone, either genetically present in certain individuals or induced by stress, increases the vulnerability to develop intake of drugs of abuse, and may have a role in dependence and relapse via an enhancement of the activity of mesencephalic dopaminergic neurons. Dopaminergic hyperresponsiveness in forebrain structures involved in regulation of motivation, such as the accumbens shell/ extended amygdala, is glucocorticoid-dependent ( Barrot et al. 2000 ), but this effect is state dependent in that it varies with nutritional status and arousal status ( Piazza et al. 1996b ). In addition, high circulating levels of glucocorticoids can feedback to shut off the hypothalamic pituitary adrenal axis and can “sensitize” the CRF systems in the central nucleus of the amygdala known to be involved in behavioral responses to stressors ( Lee et al. 1994 ; Schulkin et al. 1994 ; Shepard et al. 2000 ). These central CRF systems are well documented to contribute to behavioral responses to stressors (see above, Background, and Figure 2B ).
CRF, when injected intracerebroventricularly, is aversive and produces place aversions ( Cador et al. 1992 ) and taste aversions ( Heinrichs et al. 1991 ) and raises brain stimulation reward thresholds ( Macey et al. 2000 ). Thus, activation of brain reward systems with concomitant activation of the HPA axis ultimately can lead to activation of brain stress systems. Such an activation may contribute to a negative mood or state that dissipates with time, but with repeated administration of drug grows larger with time (or fails to return to normal homeostatic baseline), setting up a negative reinforcement mechanism (see also Drug Withdrawal and Allostasis) ( Figure 4 ). Thus, glucocorticoids are involved in adaptations from homeostasis to pathophysiology in many parts of the neuraxis including the basal ganglia/extended amygdala systems, the stress axis hypophyseal systems, and cortical systems, and as such can be considered as one of the biological substrates modulating reward ( Figure 2 ).
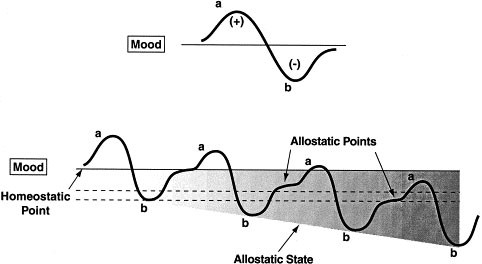
Diagram illustrating an extension of Solomon and Corbit's (1974) opponent-process model of motivation to incorporate the conceptual framework of this paper. Both panels represent the affective response to the presentation of a drug. (Top) This diagram represents the initial experience of a drug with no prior drug history, and the a-process represents a positive hedonic or positive mood state and the b-process represents the negative hedonic of negative mood state. The affective stimulus (state) has been argued to be a sum of both an a-process and a b-process. An individual whom experiences a positive hedonic mood state from a drug of abuse with sufficient time between re-administering the drug is hypothesized to retain the a-process. In other words, an appropriate counteradaptive opponent-process (b-process) that balances the activational process (a-process) does not lead to an allostatic state. (Bottom) The changes in the affective stimulus (state) in an individual with repeated frequent drug use that may represent a transition to an allostatic state in the brain reward systems and, by extrapolation, a transition to addiction (see text). Note that the apparent b-process never returns to the original homeostatic level before drug-taking begins again, thus creating a greater and greater allostatic state in the brain reward system. In other words, here the counteradaptive opponent-process (b-process) does not balance the activational process (a-process) but in fact shows a residual hysteresis. While these changes are exaggerated and condensed over time in the present conceptualization, the hypothesis here is that even during post-detoxification, a period of “protracted abstinence,” the reward system is still bearing allostatic changes (see text). The following definitions apply: allostasis , the process of achieving stability through change; allostatic state , a state of chronic deviation of the regulatory system from its normal (homeostatic) operating level; allostatic load , the cost to the brain and body of the deviation, accumulating over time, and reflecting in many cases pathological states and accumulation of damage
Environmental Factors and Reward
Environmental factors in adults, both intrinsic and extrinsic, also are important determinants of self-administration behavior, particularly during acquisition of the behavior and during reinstatement of drug-taking following extinction ( Le Sage et al. 1999 ). Food deprivation increases drug-maintained behavior, and this generalizes to different species, routes of administration, and reinforcement schedules ( De la Garza and Johanson 1987 ). Food restriction increases cocaine self-administration during unlimited access to cocaine during acquisition and reinstatement ( Carroll 1985 ; Carroll et al. 1979 ). Nondrug reinforcers, such as those concurrently available during acquisition and maintenance of cocaine self-administration, prevent acquisition and decrease maintenance ( Carroll and Lac 1993 ; Carroll and Rodefer 1993 ; Carroll et al. 1989 ). Nondrug reinforcers in a clinical setting also reduce cocaine intake ( Higgins et al. 1994 ). How these intrinsic and extrinsic factors interact with the allostatic model of addiction proposed here remains a challenge for future work.
Developmental Factors and Reward
Environmental stressful events during critical periods of development produce enduring neuroendocrinological and neurodevelopmental changes that could influence drug reward responsivity and propensity to addiction ( Fride and Weinstock 1989 ; Henry et al. 1994 ; Moyer et al. 1978 ). Prenatal stress has been found to have long-term effects on the activity of the DA system and on DA-related behaviors ( Fride and Weinstock 1989 ; Moyer et al. 1978 ). Moreover, there is evidence that prenatal stress increases and prolongs corticosterone secretion in response to stress ( Henry et al. 1994 ).
Self-administration of stimulants has been studied in the offspring of mothers submitted to a restraining procedure during the last week of pregnancy ( Deminiere et al. 1992 ; Maccari et al. 1991 ). These animals also were tested for locomotor reactivity to novelty and to stimulants, since these behaviors are characteristically enhanced in animals spontaneously predisposed to self-administration of drugs of abuse ( Piazza et al. 1989 ). Prenatal stress animals had increased locomotor reactivity to amphetamine, particularly over the first hour of testing, and a more rapid response to amphetamine. Furthermore, prenatal stress influenced the propensity to develop amphetamine self-administration. While control and stressed animals did not differ during the first day of testing, animals in the prenatal stress group showed a higher intake of amphetamine on subsequent days.
Although the development of an organism presumably carries a strong genetic component, the organism's early environment has long-lasting influence. Both components shape psychobiological temperaments and are at the origin of individual differences. Moreover, both components can contribute equally to vulnerabilities for neurodegenerative processes and ultimately deleterious life events and can interact. Prenatal and postnatal events also modify the activity of the HPA axis ( Caldji et al. 1998 ; Ladd et al. 2000 ), and maternal glucocorticoids have a major role on the development of endocrine function in the offspring. In fact, high levels of maternal glucocorticoids during prenatal stress have marked long-term repercussions on the efficiency of the offspring's HPA negative feedback mechanisms. Thus, a modification of corticosterone secretion via changes in HPA axis activity could be a biological substrate of the long-term behavioral effects of prenatal and postnatal events that could contribute to individual differences in vulnerability to allostasis in the brain reward system (see above).
DRUG WITHDRAWAL AND ALLOSTASIS
Motivational components of withdrawal.
Motivational changes associated with acute withdrawal reflect opponent-process-like changes in the reward circuitry activated by the acute reinforcing effects of drugs of abuse. Brain systems associated with the development of motivational aspects of withdrawal are hypothesized to be a major source of potential allostatic changes that drive and maintain addiction. Acute withdrawal from the chronic use of drugs of abuse long has been associated with physical signs, and the manifestation of these physical signs varies with each drug of abuse. However, the manifestation of physical signs of withdrawal are only one of a constellation of physical and motivational symptoms associated with addiction ( American Psychiatric Association 1994 ) and the physical symptoms of withdrawal may be largely irrelevant to the motivation to take drugs. For example, patients relapse long after physical signs of withdrawal have dissipated.
Acute withdrawal also is associated with a negative affective state including various negative emotions such as dysphoria, depression, irritability and anxiety. These emotional states appear to be common to withdrawal from chronic use of all major drugs of abuse and may have major motivational significance in contributing to the maintenance of drug addiction. For example, cocaine withdrawal in humans in the outpatient setting is characterized by severe depressive symptoms combined with irritability, anxiety, and anhedonia lasting several hours to several days (i.e., the “crash”) and may be one of the motivating factors in the maintenance of the cocaine-dependence cycle ( Gawin and Kleber 1986 ). Inpatient studies of cocaine withdrawal in cocaine-dependent subjects have shown similar changes in mood and anxiety states, but they generally are much less severe ( Weddington et al. 1991 ). Opiate withdrawal is characterized by severe dysphoria, and alcohol withdrawal produces pronounced dysphoria and anxiety.
Recent studies using animal models of reward and anxiety have provided measures of behavioral changes that can be linked to emotional states associated with withdrawal from all major drugs of abuse, including opiates, psychostimulants, alcohol and nicotine. A validated measure of brain reward function is the technique of intracranial self-stimulation ( Kornetsky and Bain 1990 ) where small amounts of electrical current to the medial forebrain bundle through an electrode will sustain vigorous behavior to obtain the stimulation. Using the technique of intracranial self-stimulation to measure reward thresholds throughout the course of drug dependence, reward thresholds are increased during withdrawal from chronic administration of all major drugs of abuse (reflecting a decrease in reward), and some of these changes can persist for up to a week post-drug ( Table 2 ).
Neural Substrates of Motivational Withdrawal
The neural substrates and neuropharmacological mechanisms for the negative emotional-motivational effects of drug withdrawal may involve disruption of the same neural systems implicated in the positive reinforcing effects of drugs of abuse. As such, these effects may reflect changes in the activity of the same mesocorticolimbic system (midbrain-forebrain system) implicated in the positive reinforcing effects of drugs and can last up to 72 hours depending on the drug and dose administered ( Legault and Wise 1994 ; Leith and Barrett 1976 ; Markou and Koob 1991 , 1992 ; Parsons et al. 1995 ; Schulteis et al. 1994 ) ( Table 2 ). Examples of such changes at the neurochemical level include decreases in dopaminergic and serotonergic transmission in the nucleus accumbens during drug withdrawal as measured by in vivo microdialysis ( Parsons et al. 1995 ; Weiss et al. 1992b ), increased sensitivity of opioid receptor transduction mechanisms in the nucleus accumbens during opiate withdrawal ( Stinus et al. 1990 ), decreased GABAergic and increased NMDA glutamatergic transmission during alcohol withdrawal ( Fitzgerald and Nestler 1995 ; Roberts et al. 1996 ; Weiss et al. 1996 ), and differential regional changes in nicotine receptor function ( Collins et al. 1990 ; Dani and Heinemann 1996 ) ( Table 3 ).
Other neurotransmitter systems not linked to the acute reinforcing effects of drugs of abuse also may be recruited to contribute to the negative emotional-motivational state associated with acute withdrawal ( Koob and Bloom 1988 ; Koob and Le Moal 1997 ) ( Table 3 ). Activation of brain and pituitary stress systems may be another common response to repeated administration of drugs of abuse that may be involved in the negative emotional state associated with acute withdrawal ( Table 3 ). Pituitary adrenal function is activated during drug dependence and during acute withdrawal from drugs of abuse in humans. Dysregulation of the HPA axis also can persist even past acute withdrawal ( Kreek 1987 ; Kreek et al. 1984 ). Corticotropin-releasing factor (CRF) function, outside of the pituitary adrenal axis, also is activated during acute withdrawal from cocaine, alcohol, opiates, and THC and thus may mediate some of the behavioral responses to stress associated with acute abstinence ( Heinrichs et al. 1995 ; Koob et al. 1994 ; Richter and Weiss 1999 ; Rodriguez de Fonseca et al. 1997 ).
Rats treated repeatedly with cocaine, nicotine, THC and alcohol show significant anxiety-like responses following cessation of chronic drug administration which are reversed with intracerebroventricular administration of a CRF antagonist ( Rassnick et al. 1993b ; Sarnyai et al. 1995 ). Microinjections into the central nucleus of the amygdala of lower doses of the CRF antagonist also reversed the anxiogenic-like effects of alcohol withdrawal ( Rassnick et al. 1993b ), and similar doses of the CRF antagonist injected into the amygdala were active in reversing the aversive effects of opiate withdrawal ( Heinrichs et al. 1995 ).
Studies using in vivo microdialysis have shown that rats withdrawn from chronic alcohol, withdrawn from chronic cocaine, and precipitously withdrawn from chronic cannabinoids show increases in the release of CRF from the central nucleus of the amygdala ( Cummings et al. 1983 ; Merlo-Pich et al. 1995 ; Rodriguez de Fonseca et al. 1997 ). Indeed, one could speculate that the profound activation of both the HPA axis and central CRF systems during drug withdrawal, particularly after a binge, represents the ultimate activation of the HPA axis and subsequent sensitization of central CRF that cannot return to homeostatic levels (see above and Figure 3 ). Thus, a cascade of events could be hypothesized to develop in the following manner: 1) reward system activation in a binge; 2) downregulation of dopamine/opioid peptide systems at the end of a binge; 3) continued dysregulation of reward systems during acute withdrawal; and 4) HPA activation and central CRF activation during acute withdrawal that may persist into protracted abstinence. Thus, chronic drug self-administration sets up two major components of allostasis to the brain reward systems during a binge withdrawal cycle. There is a hypofunctioning of neurotransmitter systems involved in positive reinforcement and a recruitment of neurotransmitter systems involved in negative emotional states that provide the motivation for negative reinforcement.
Neuropeptide Y (NPY), a 36 amino acid member of the pancreatic polypeptide family, also has been implicated in the neuroadaptations associated with the development of drug addiction. NPY is abundantly present in brain areas implicated in alcohol- and drug dependence, such as the ventral striatum and amygdala ( de Quidt and Emson 1986 ). Acute effects of NPY are remarkably similar to those of alcohol in producing a suppression of anxiety-like responses, sedation ( Heilig et al. 1994 ), and anticonvulsant actions ( Vezzani et al. 1999 ). A quantitative trait locus contributing to the phenotype of alcohol-preferring P rats has been found within a chromosomal region containing the NPY gene ( Carr et al. 1998 ). Furthermore, in several brain areas, central expression of NPY differs between alcohol-preferring P and non-preferring NP rats. Among these differences are suppressed levels of NPY in the central amygdala, also seen in high alcohol drinking (HAD) rats, suggesting that NPY within this structure might play a role in the regulation of alcohol intake ( Hwang et al. 1999 ). Furthermore, the electrophysiological response to intracerebroventricular NPY differs between P and NP rats ( Ehlers et al. 1999 ). A causal relation between NPY expression and alcohol intake has been suggested by the inverse relationship between NPY-expression and alcohol intake in NPY-transgenic and NPY-mutant mice, respectively ( Thiele et al. 1998 ).
NPY also may be involved in dependence on drugs other than alcohol. NPY expression in the ventral striatum is suppressed following prolonged treatment with cocaine, possibly related to the anhedonic state present during cocaine withdrawal ( Wahlestedt et al. 1991 ). Conversely, withdrawal from opiates is antagonized by central NPY ( Woldbye et al. 1998 ). Overall, the role of NPY in dependence perhaps may be best viewed as an inhibitor of neuronal excitability ( Palmiter et al. 1998 ), and it is important to note that NPY and CRF have been hypothesized to have reciprocal actions in mediating behavioral responses to stressors ( Heilig et al. 1994 ). Thus, one may speculate that during acute withdrawal, and perhaps extended to protracted abstinence, decreases in NPY activity may accompany increases in CRF activity further potentiating the neurochemical brain stress system contribution to allostasis in the reward system.
Recruitment of nonstress, anti-reward systems involving other neurotransmitter systems also has been hypothesized to contribute to the motivational changes associated with chronic administration of drugs of abuse and may represent another contribution to the allostatic state. These include the neuropeptides dynorphin, neuropeptide FF (NPFF), and more recently orphanin FQ, and these anti-reward neurotransmitters may be natural counteradaptive mechanisms that are activated to limit impulsive behavior to limit impulsive reward-seeking behavior. Dynorphin peptides appear to decrease the dopamine activity via a presynaptic action on kappa opioid receptors in the nucleus accumbens, and kappa agonists produce aversive effects in rodents and humans ( Hyman 1996 ). Moreover, dynorphin transmission also is modulated by glucocorticoids, and this modulation could represent an indirect action upon dopamine neurons at the terminal projection level (for review, see Angulo and McEwen 1994 ). Anti-opiate activities also have been hypothesized for NPFF, previously called F8Fa, based on the effects of intracerebroventricular injection of NPFF-related peptides. Administration of NPFF attenuates morphine- and stress-induced analgesia ( Kavaliers 1990 ), and precipitates morphine withdrawal ( Malin et al. 1990 ). More compelling, NPFF antagonists can increase both morphine- and stress-induced analgesia, reverse morphine tolerance ( Lake et al. 1992 ), and attenuate the naloxone-precipitated withdrawal syndrome in morphine-dependent rats. An NPFF antagonist also blocks some aspects of nicotine withdrawal ( Malin et al. 1996 ). Anti-opiate like effects also have also been reported with administration of the orphan receptor binding peptide orphanin FQ (nociceptin) ( Mogil et al. 1996 ), although more recent evidence suggests that orphanin FQ produces more anxiolytic-like effects ( Jenck et al. 1997 ).
Counter-regulatory processes within the reward domain could acutely limit drug intake. In contrast, if the initial counter-regulatory response is inappropriate and cannot balance the activational forces involved in an allostatic response, it could lead to further drug intake and the development of allostatic-like changes in these counter-regulatory processes where the anti-reward effects develop slowly (see neuroadaptation sections and Figure 4 ). One could envision genetic- and environmentally based vulnerability at both ends of the counteradaptive process. Thus, these anti-reward systems are hypothesized to be abnormally activated during the development of dependence, and thus contribute to the allostatic state of reward dysfunction in addiction.
EXTENDED AMYGDALA: A COMMON SUBSTRATE FOR ALLOSTATIC CHANGES IN REWARD FUNCTION
Specific components of the basal forebrain have been identified as potential substrates for the positive reinforcing effects of drugs of abuse and the negative reinforcement associated with a state of allostasis associated with addiction. Recent neuroanatomical data and new functional observations have identified a separate entity within the basal forebrain, termed the “extended amygdala,” that has been hypothesized to be a common neural circuitry for the reinforcing actions of drugs ( Alheid and Heimer 1988 ). The term “extended amygdala” originally was described by Johnston (1923) and represents a macrostructure that is composed of several basal forebrain structures: the bed nucleus of the stria terminalis, the central medial amygdala, the area termed the sublenticular substantia innominata, and a transition zone that forms the medial posterior part of the nucleus accumbens (e.g., shell) ( Heimer and Alheid 1991 ). These structures have similarities in morphology, immunohistochemistry and connectivity ( Alheid and Heimer 1988 ) ( Figure 3 ), and they receive afferent connections from limbic cortices, hippocampus, basolateral amygdala, midbrain, and lateral hypothalamus. The efferent connections from the extended amygdala include the posterior medial (sublenticular) ventral pallidum, medial ventral tegmental area, various brainstem projections, and a considerable projection to the lateral hypothalamus ( Heimer et al. 1991 ). The observation of a major connection to the lateral hypothalamus provides an important functional link between the neurochemical/neurobiological substrates of drug reward with the long-hypothesized neurobiological substrates of brain stimulation reward.
Specific sites within the extended amygdala and selective neurochemical and neuropharmacological actions have been identified for both the acute positive reinforcing effects of drugs of abuse and in the negative reinforcement associated with drug abstinence. Microinjections of dopamine D1 antagonists directly into the shell of the nucleus accumbens, the central nucleus of the amygdala ( Caine et al. 1995 ) and the bed nucleus of the stria terminalis ( Epping-Jordan et al. 1998 ) are particularly effective in blocking cocaine self-administration. In vivo microdialysis studies show a selective activation of dopaminergic transmission in the shell of the nucleus accumbens in response to acute administration of virtually all major drugs of abuse (Pontieri et al. 1995 , 1996 ; Tanda et al. 1997 ). In addition, the acute reinforcing effects of alcohol are blocked by the administration of GABAergic and opioidergic competitive antagonists into the central nucleus of the amygdala ( Heyser et al. 1999 ; Hyytia and Koob 1995 ), while lesions of the cell bodies within this structure markedly suppress ethanol self-administration ( Moller et al. 1997 ).
A role for the involvement of the extended amygdala in the aversive stimulus effects of drug withdrawal includes changes in opioidergic, GABAergic and CRF neurotransmission during acute withdrawal. There is enhanced sensitivity of alcohol-dependent rats to GABA agonists during acute withdrawal ( Roberts et al. 1996 ), and the CRF systems in the central nucleus of the amygdala are activated during acute alcohol, opioid, THC and cocaine withdrawal ( Merlo-Pich et al. 1995 ).
These recent developments in the neurobiology of drug reinforcement combined with existing knowledge of the substrates for emotional behavior ( Davis 1997 ) may provide critical insights to the neurobiology of the addiction process and ultimately may bridge what have been largely independent research pursuits. Perhaps more importantly, the neuronal circuit comprising the extended amygdala interacting with the brain stress axis circuit and the compulsive behavior circuit ( Figure 3 ) are well situated to form a heuristic model for exploring the mechanisms associated with allostasis in the brain reward systems that may explain such concepts as craving and vulnerability to relapse (see above). The normal role for these counteradaptive changes observed during acute withdrawal produced by repeated drug administration may be to limit impulsive reward-seeking behavior and thus would represent homeostatic changes. However, these homeostatic changes enter the allostatic domain when they persist and are maintained and keep building during the development of dependence (see next section).
VULNERABILITY TO ADDICTION AND ALLOSTASIS: NEUROADAPTATION
Sensitization and counteradaptation.
Two neuroadaptive models (sensitization and counteradaptation) have been formulated to explain the change in reward function associated with the development of addiction or substance dependence and how this change contributes to compulsive use. Sensitization has been defined as the increased response to a drug that follows its repeated presentation but can take on a broader meaning when considered in the context of increased responsiveness of any drug-related phenotype (see below). Psychomotor sensitization, as defined by increased locomotor activation produced by repeated administration of a drug, is more likely to occur with intermittent exposure of drugs, whereas tolerance is more likely to occur with continuous exposure. A conceptualization of the role of psychomotor sensitization in drug dependence has been proposed where a shift in an incentive-salience state described as “wanting” was hypothesized to be progressively increased by repeated exposure to drugs of abuse ( Robinson and Berridge 1993 ). The transition to pathologically strong wanting or craving has been proposed to define compulsive use.
An alternative counteradaptation hypothesis long has been proposed where the initial acute effect of the drug is opposed or counteracted by homeostatic changes in systems that mediate primary drug effects ( Poulos and Cappell 1991 ; Siegel 1975 ; Solomon and Corbit 1974 ). In this opponent-process theory, tolerance and dependence are inextricably linked ( Solomon and Corbit 1974 ), and affective states, pleasant or aversive, were hypothesized to be automatically opposed by centrally mediated mechanisms that reduce the intensity of these affective states. Opponent-process counteradaptation can be defined as a-processes (positive hedonic effects) occurring shortly after presentation of the reinforcer and showing tolerance ( Figure 4 ). In contrast, the b-processes (negative hedonic effects) appear after the a-process has terminated, are slow to decay, and get larger with repeated exposure.
Allostasis and Neuroadaptation
The present conceptualization is an attempt to extend neuroadaptive hypotheses to the framework of allostasis. In addition, an attempt is made to integrate the psychomotor sensitization hypothesis ( Robinson and Berridge 1993 ) and the counteradaptation hypothesis. The first important conceptualization is that the allostasis of importance to addiction is that of dysregulation of the brain reward system(s). Under such a framework of change to maintain stability, both enhanced reward and compromised reward function could contribute to an allostatic state and ultimately could potentiate the overall vulnerability to a major breakdown or dysregulation that would lead to the spiralling distress of addiction. With sensitization, empirical data support the hypothesis that the locomotor-activating effects (psychomotor sensitization) of many drugs of abuse show sensitization with repeated administration of nondependence-inducing doses (e.g., doses that do not induce physical signs of withdrawal upon abstinence). This psychomotor sensitization has been extended with certain drugs under certain situations to the reinforcing effects of drugs ( Schenk and Partridge 1997 ) where animals with a history of drug administration initiate drug self-administration at lower doses than drug-naive subjects. Thus, a history of drug intake could shift the dose-effect function to the left to initiate drug-seeking behavior, increase self-administration at low doses that do not normally sustain self-administration, and produce a larger neurobiological effect in an experienced subject than if given to a drug-naive subject. Generating the molecular and cellular resources necessary for increased turnover of dopamine or opioid peptide function may leave these systems vulnerable to dysregulation that contributes to the allostatic state of reward dysfunction.
Addiction, however, is associated with or even defined as ( Russell 1976 ) a negative affective state including dysphoria, irritability, and anxiety during abstinence from a more continuous or closely spaced pattern of administration of drug, particularly as the severity of symptoms increases. This state appears to reflect a decrease in baseline reward mechanisms and an increase in drug intake to compensate for the shift in reward baseline from an opponent-process perspective.
The increase in drug intake is known classically as tolerance. In this state, more drug is required to produce an effect than in an individual not in a state of dependence. However, more recent conceptualizations in the domain of opiate/pain research have argued that tolerance in fact may be more of an “apparent tolerance” in that the b-process simply gets larger and larger requiring recruitment of more and more drug to maintain normal reward by counteracting the increasingly larger b-process ( Colpaert 1996 ; Laulin et al. 1999 ). Such neuroadaptive effects may be long-lasting and are illustrated in studies regarding opiates and pain modulation. In opiate-naive rats, subcutaneous injection of heroin induces analgesia as an unconditioned immediate response, followed by allodynia and a decrease in pain threshold.
Allodynia is defined as manifestation of pain in the absence of noxious stimuli that can last several days. This phenomenon can be exaggerated by administration of the mu-opiate receptor antagonist naloxone. For example, when injected shortly after administration of a dose of morphine or fentanyl, naloxone both abolishes the acute opiate-induced increase in nociceptive threshold and also reduces it to below basal value (hyperalgesia). This hyperalgesia can be blocked by noncompetitive NMDA receptor antagonists suggesting a critical role for glutamatergic systems ( Celerier et al. 1999 ; Larcher et al. 1998 ; Laulin et al. 1998 ). This hyperalgesia and allodynia (b-process) also sensitizes both with repeated drug administration and over time leading to an apparent tolerance. Thus, in addition to the acute unconditioned immediate response of the drug, there is recruitment of other systems such as glutamate leading to opposite effects that sensitize with time and additional administration of the drug. Such a neuroadaptive change has been interpreted as a form of structural memory and may be related to the same processes underlying what has been described as apparent tolerance ( Colpaert 1996 ; Slot and Colpaert 1999 ). Also, acute motivational withdrawal (negative emotional state) has been observed following a single injection of morphine that gets larger following a subsequent morphine injection ( Schulteis et al. 1997 ). While an initial hyperalgesic response simply may reflect a homeostatic change, the persistence of the overshoot (allodynia) represents more of an allostatic change. From the addiction perspective, the allostatic changes to the reward system have taken on a new dimension where to maintain normal reward function the subject must marshal substantial neurobiological resources ( Figure 4 ).
The conundrum with such a conceptualization is how do these two neuroadaptive processes–psychomotor sensitization and counteradaptation–coexist to produce what we know as the signs and symptoms of addiction. One hypothesis is that in the early stages of compulsive drug-taking, where negative affect post-drug is minimal and the subject appears driven mainly by the positive reinforcing effects of a drug or anticipation of the positive reinforcing effects of the drug, psychomotor sensitization plays an important role. Here, drug-seeking is driven by positive reinforcement and resembles more a classical impulse control disorder. Sensitization may be involved in the preoccupation/anticipation stage during acquisition of drug-taking and may be recruited again during reinstatement of drug-taking following detoxification and prolonged abstinence ( Koob and Le Moal 1997 ).
Impulsivity, in the context of impulse control disorders, has been defined as when an individual feels an increasing sense of tension or arousal before committing an act and then experiences pleasure, gratification or relief at the time of committing an act ( American Psychiatric Association 1994 ). One prediction such a formulation would make is that before or during the early stages of transition to addiction, repeated administration of drugs at doses that do not produce negative affective states upon cessation of drug intake are more likely to manifest psychomotor sensitization. Psychomotor sensitization, in turn, could enhance drug intake by incentive motivational (salience) processes rather than tolerance or apparent tolerance. However, when negative affective states (b-processes) begin to grow during the course of more and more drug-taking, the counteradaptation mechanisms may begin to predominate with tolerance or “apparent tolerance” to the reinforcing effects and manifestation of affective withdrawal. Interestingly, from the perspective of a broad definition of the sensitization process, an allostatic-like change in negative affective states which gets larger with repeated exposure to the drug also represents a true “sensitization” ( Figure 4 ). This would lead to addiction being driven by negative reinforcement, and here the addiction cycle takes on characteristics more of a compulsive disorder where the addiction cycle presumably recruits the same neural circuits associated with a compulsive disorder (cortico-striatal-thalamic loop) ( Figure 3 ). Compulsion is defined in this context as when an individual performs repetitive behaviors, the goal of which is to prevent or reduce anxiety or distress, not to provide pleasure or gratification ( American Psychiatric Association 1994 ).
Neurosubstrates of Allostatic Neuroadaptation
Thus, sensitization as a process may have a more general role in the development of allostasis in the reward system that accompanies the transition to addiction. First, dopamine and glucocorticoids participate in a “sensitized” (psychomotor sensitization) response to a history of drug intake as measured by locomotor activation and drug threshold for initiating drug self-administration. Second, the negative affective state becomes “sensitized” (negative affective state sensitization) and grows larger with repeated administration of drug, recruiting not only more brain circuits but also additional brain neurochemical systems such as the brain stress systems (Figures 4 and 5 . The combination of a recruited brain stress system and an already compromised reward circuit (as reflected in “depletion” or “dysfunction” in the dopamine, opioid peptide, and even serotonergic domains) provides an additional powerful contribution to the negative affective state that reflects the allostatic state of reward system dysfunction.
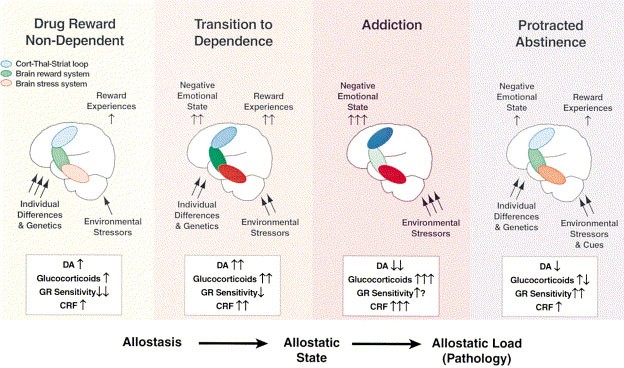
Diagram depicting the relationship between the continuum from allostasis to pathology and the transition from drug-taking to addiction to protracted abstinence. Three simplified brain circuits are conceptualized to change and contribute to the allostatic state in the brain reward system: the reward system itself (green), the brain stress systems (red), and the cortico-thalamic-striatal (CTS) loop (blue). In the nondependent state, environmental stressors are minimal, reward experiences are normal, and the brain stress and CTS loop are not engaged. During the transition to dependence all three circuits are engaged, and in the state known as addiction, the brain reward system is in a major underactivated state while both the brain stress system and the CTS loop are highly activated. Protracted abstinence is characterized by a return toward a normal state but with residual activation of the brain stress systems and residual underactivation of the brain reward system. Note that activation of the hypothalamic pituitary adrenal axis is hypothesized to drive the brain stress axis to contribute to the allostatic state of addiction. DA, dopamine; CRF, corticotropin-releasing factor; GR, glucocorticoid receptor. The following definitions apply: allostasis , the process of achieving stability through change; allostatic state , a state of chronic deviation of the regulatory system from its normal (homeostatic) operating level; allostatic load , the cost to the brain and body of the deviation, accumulating over time, and reflecting in many cases pathological states and accumulation of damage
Both of these theoretical positions would allow one to predict neuroadaptation at the molecular, cellular and system levels. Neurochemical changes that are altered during the development of sensitization have been hypothesized to involve the same neurotransmitters implicated in the acute reinforcing effects of drugs ( Koob and Bloom 1988 ). For example, sensitization to psychostimulants is associated with a number of neurochemical changes within the mesolimbic dopamine system ( White 1996 ). These include subsensitivity of dopamine autoreceptors in the ventral tegmental area, a supersensitivity of D1-mediated responses ( Henry and White 1991 ), increased levels of adenylyl cyclase and protein kinase A, and decreased levels of Gi proteins ( Nestler 1996 ; Self and Nestler 1995 ). In addition, there is cross-sensitization to stressors, and elevated glucocorticoids are associated with a propensity to self-administer doses of drugs that are not usually reinforcing ( Piazza and Le Moal 1996 ; Piazza et al. 1996a ).
At the molecular level, there is a decrease in the ability of cocaine to induce the intermediate early gene c-fos but a sustained expression of the AP-1 transcription factor ( Self and Nestler 1995 ). In addition, recent evidence suggests that novel isoforms of delta-FosB, a member of the Fos family of transcription factors, accumulate in specific regions of the brain in response to repeated administration of drugs of abuse and other psychoactive drugs ( Nestler et al. 1999 ). Particularly intriguing is evidence provided by inducible transgenic mice upon overexpression of delta-FosB showing biochemical and behavioral changes which mimic the chronic drug-treated state ( Nestler et al. 1999 ). Chronic administration of other major drugs of abuse such as opiates and alcohol produce similar molecular changes in second messenger, signal transduction pathways and transcription factors ( Koob et al. 1998b ). Thus, the sensitization associated with locomotor activation and the mesolimbic dopamine system involves neuroadaptations that can be long-lasting and contribute to drug-seeking. Unknown at this time is whether these molecular adaptations persist into the counteradaptation stage where other neurochemical systems are recruited.
Overactivity in the brain reward system produced by excessive drug-taking is hypothesized to engage the after-effects of allostatic adaptations in an attempt to maintain functional hedonic states. The functional state of neurotransmitter systems activated to produce drug reward ultimately may be depleted or unable to keep up with the demand involved in mediating the acute reinforcing effects of drugs of abuse. Examples of such allostatic adaptive neurochemical events might include decreases in dopaminergic and serotonergic transmission in the nucleus accumbens during drug withdrawal as measured by in vivo microdialysis ( Parsons et al. 1995 ; Weiss et al. 1992b ), increased sensitivity of opioid receptor function in the nucleus accumbens during opiate withdrawal ( Stinus et al. 1990 ), decreased GABAergic and increased NMDA glutamatergic transmission presumably in the accumbens-amygdala reward circuit during alcohol withdrawal ( Fitzgerald and Nestler 1995 ; Roberts et al. 1996 ; Weiss et al., 1996 ), and differential regional changes (reward system versus nonreward systems) in nicotine receptor function during nicotine addiction ( Collins et al. 1990 ; Dani and Heinemann 1996 ).
Allostatic Neuroadaptation and the Stress Axis
Such allostatic adaptations also may involve the recruitment of neurotransmitters that mediate behavioral and physiological responses to stressors. Neurochemical components of stress responses that appear to be changed during acute withdrawal from alcohol, cocaine, THC or opiates are increases in CRF systems and decreases in NPY systems, and thus may mediate aspects of stress associated with abstinence ( Koob et al. 1994 ). These effects have been hypothesized to involve an action on CRF systems in limbic areas implicated in behavioral responses to stressors ( Koob et al. 1994 ), but also may include activation of the hypothalamic pituitary adrenal axis ( Kreek and Koob 1998 ; Piazza and Le Moal 1996 ). Dysregulation of the HPA axis has important functional consequences that range from activating the brain reward system, to serving as a stimulus for self-medication, to actual neurotoxicity ( Kreek and Koob 1998 ). Activation of the HPA axis also may in turn “sensitize” the limbic neurotropic stress systems that may contribute to the negative affective state of the addiction cycle and set up an important source of negative reinforcement (e.g., self-administration of a drug such as alcohol to suppress an overactive limbic CRF system; see above) ( Schulkin et al. 1994 ; Shepard et al. 2000 ).
Norepinephrine systems emanating from the nucleus locus coeruleus also long have been hypothesized to be involved in mediating behavioral constructs associated with alertness, arousal and stress. Central nervous system norepinephrine systems have been considered as having a role in arousal/alerting functions ( Robbins and Everitt 1995 ). Stress increases the turnover of norepinephrine in many terminal projection areas of the locus coeruleus ( Korf et al. 1973 ) and increases extracellular norepinephrine in the hippocampus ( Abercrombie et al. 1988 ), and a variety of stressors increase the discharge rate of locus coeruleus neurons ( Valentino et al. 1993 ).
A number of brain sites currently hypothesized to be particularly important for the behavioral effects of CRF, specifically with regard to the role of CRF in arousal and behavioral responses to stress, are closely linked to the brain norepinephrine systems including the locus coeruleus, the paraventricular nucleus of the hypothalamus, the bed nucleus of the stria terminalis, and the central nucleus of the amygdala ( Valentino et al. 1993 ; Van Bockstaele et al. 1998 ). Thus, stress, either autonomic or emotional, activates CRF release in the nucleus locus coeruleus which in turn stimulates activity in the locus coeruleus. This activation of the locus coeruleus in turn produces release of norepinephrine in forebrain terminal projections. Particularly intriguing are the data suggesting that norepinephrine in these terminal areas in turn stimulates the release of CRF (for example, in the paraventricular nucleus of the hypothalamus, the bed nucleus of the stria terminalis, and the central nucleus of the amygdala). These are brain structures with a high density of CRF-containing cell bodies and terminals and are well-implicated in mediating the role of CRF in behavioral responses to stress.
Finally, the evidence that CRF originating from these noradrenergic terminal areas also innervates the region of the locus coeruleus ( Van Bockstaele et al. 1998 ) suggests a potentially powerful feed-forward system ( Koob 1999a ). Such a feed-forward system may be a mechanism for the progressive augmentation of stress responsivity with repeated exposure. Conceptualized as stress syndrome dysregulation ( Chrousos and Gold 1992 ), kindling ( Post and Weiss 1995 ), or a change in allostatic set point ( Schulkin et al. 1998 ), a feed-forward system involving CRF/norepinephrine interactions could be hypothesized to be a potential additional stress mechanism contributing to the allostasis during the development of addiction. A challenge for future studies will be to revisit the role of brain norepinephrine systems in drug addiction in the context of allostatic changes in arousal and stress, particularly with such drugs as alcohol and opiates where withdrawal is associated with a dramatic activation of the sympathetic nervous system.
Allostatic Neuroadaptation and Drug Reward Set Point
Support for an allostatic view of reward regulation comes from increasing evidence that chronic exposure to drugs of abuse can change the “set point” for drug reward. Animals with prolonged access to cocaine or heroin will continue to increase their cocaine intake on a daily basis and show enhanced intake of cocaine at all doses tested (Ahmed and Koob 1997 , 1998 , 1999 ; Ahmed et al. 2000 ). Indeed, the dose-effect function appears to shift upward instead of to the right (tolerance) or to the left (sensitization) ( Ahmed and Koob 1998 ). Animals with a history of alcohol dependence show prolonged increases in alcohol self-administration long past acute withdrawal ( Roberts et al. 2000a ). Such changes in drug reward set point may reflect an allostatic change rather than simply sensitization or homeostatic adaptation. This same phenomenon has been demonstrated in animals selected on the basis of their inherent differential vulnerability to drugs ( Piazza et al. 2000 ; Sutton et al. 2000 ).
The allostatic changes set in motion by a history of drug use may begin with sensitization and the overactivation of the mesolimbic dopamine system described above which may in part be driven by an activation of the hypothalamic pituitary adrenal axis. This in turn drives further drug use, which depletes reward transmission, but also recruits brain stress systems to counteract the increased hedonic processing. The combination of high dopamine turnover (example of driving positive reward) in the face of high CRF activity (recruited anti-reward), and possible low NPY activity, leads to a severe allostatic state in the brain reward system should the drug become unavailable (Figures 3 and 5 ).
VULNERABILITY TO ADDICTION AND ALLOSTASIS: GENETIC CONTRIBUTIONS
Human genetic studies.
Genetic influences on human substance abuse vulnerability have been documented by using classical and molecular genetic approaches. More recently, research to find specific genes contributing to human vulnerability is being developed in parallel with studies in experimental animal models in order to define the limits of possible genetic and environmental factors in vulnerability ( Cadoret et al. 1986 ; Devor and Cloninger 1989 ; George and Goldberg 1989 ; Uhl et al. 1995 ). From clinical and naturalistic approaches, it is generally agreed that preexisting intrinsic vulnerability does exist that leads from recreational use to abuse and dependence for a small percentage of users ( Anthony et al. 1994 ). Why some individuals succumb and others do not is not a trivial question, but it has been generally neglected despite clinical evidence. Genetic interactions with compulsive drug use may be explained by an individual differences-centered approach to the problem. Here, abuse is the consequence of a peculiar, possibly pathological reaction to the drug, and individuals are vulnerable because of an intrinsic functional brain state that interacts with the drug.
Clinical epidemiological studies, and more recently experimental studies, have shown that individual vulnerability to drug abuse is not simply a drug-specific phenomenon. If drug abuse was only a drug-specific phenomenon, then drug-vulnerable subjects would differ from drug-resistant ones only for specific drug-induced behaviors and would not show any other psychobiological perturbations. In fact, vulnerability to drug addiction may involve certain personality characteristics, sometimes in the context of comorbidity with other psychopathological disorders. In other words, such vulnerability — whatever the cause, genetic and/or environmental life events — may reflect a potential or already constituted state of reward system allostasis. Such vulnerability may be a drug-specific phenomenon in that a large component of the heritability is specific for a particular drug ( Bierut et al. 1998 ; Merikangas et al. 1998 ).
Substantial evidence exists for an important genetic contribution to the vulnerability to develop alcoholism and tobacco addiction. Twin studies with adoption have provided strong evidence for an important genetic influence on the risk of becoming an alcoholic in both men and women ( Heath et al. 1997a ). Twin studies in Scandinavia and the United States have shown consistently higher rates of alcoholism in monozygotic compared to dizygotic twins of male alcoholics ( Hrubec and Omenn 1981 ; Kaij 1960 ; Koskenvuo et al. 1984 ), and adoption studies have shown consistently higher rates of alcoholism in the adopted-away sons of alcoholic biological parents than in control adoptees ( Cadoret et al. 1994 ; Goodwin et al. 1973 ). While a genetic influence on alcoholism in women generally has been considered weaker, recent analyses suggest that this may be the result of low statistical power due to the low base rate of alcoholism in women ( Heath et al. 1997b ; Kessler et al. 1994 ).
The biological bases for such genetic contributions are largely unknown, but a number of avenues have been suggested by studies of high risk individuals. Alcohol challenge research suggests a genetic contribution to differences in sensitivity to alcohol with sons of alcoholics showing less of a response to alcohol in such measures as body sway, subjective measures of intoxication (self-rated), and electrophysiological measures ( Schuckit 1994 ). Impulsivity, behavioral undercontrol and negative affectivity also have been identified as potential mediators of the genetic contribution to alcoholism in prospective studies ( Sher 1991 ).
Recent evidence also suggests that genetic factors have an important influence on the onset and development of tobacco smoking ( Heath and Madden 1998 ). Using twin data and a logistic regression model approach, data suggest that in men genetic factors play an important role in predicting which individuals who become cigarette smokers progress to longterm persistent smoking ( Heath and Madden 1998 ). For example, using multiple logistic regression analyses predicting persistence in smoking among smokers, the odds ratios are significantly higher for monozygotic twins than for dizygotic twins ( Heath and Madden 1998 ). Similar conclusions have been drawn using a genetic liability model approach with monozygotic and dizygotic twins ( True et al. 1997 ).
One untested hypothesis is that individuals at risk for drug abuse in fact have a biological vulnerability within the same domains as those biological changes hypothesized to develop over the course of heavy drug intake regardless of genetic history (see above). In the study of the genetics of alcoholism, there is evidence of genetic control over various aspects of drinking but to a modest degree for any one dimension. This suggests that both environmental factors and environment-genetic interactions may play a role ( Sher 1991 ).
Animal Genetic Studies
Animal studies have established a number of models of various aspects of alcoholism including excessive drinking through the use of inbred strains and selective breeding programs ( Crabbe 1989 ; Crabbe et al. 1999 ). The animals selectively bred for excessive drinking show many of the characteristics of human alcoholics such as high alcohol intake, a generalized decreased sensitivity to alcohol, and drinking to the point of showing withdrawal effects upon cessation of drinking ( McBride and Li 1998 ). Studies with inbred strains of mice allow for crossing and the identification of specific gene loci linked to a given trait by use of quantitative trait locus analysis ( Phillips et al. 1994 ). Both approaches have begun to identify neurobiological substrates that may be responsible for excessive drinking including in the selectively bred animals alterations in dopaminergic and serotonergic function and in the inbred mouse strains identification of gene loci associated with specific neurotransmitters such as GABA and NPY ( Carr et al. 1998 ; Crabbe et al. 1999 ; Li 2000 ). Parallel studies have yet to be performed with other drugs of abuse. However, there are major differences in the avidity with which inbred strains of mice will self-administer drugs that could provide the basis for future genetic studies of vulnerability to addiction or even protection from drug-seeking. For example, the BALBc mouse will not self-administer cocaine but if pretreated with an anti-anxiety drug such as diazepam will avidly self-administer cocaine ( David et al. 2000 ). These results suggest that in these animals the acute anxiogenic- or stress-like actions of cocaine protect against self-administration.
Thus, genetic factors can act as constitutive (trait) mechanisms in the organism to produce the differential sensitivity within the brain reward and stress systems that can interact with environmental factors to produce a greater or lesser state of allostasis within the brain reward system upon drug-taking. Decreased sensitivity to a drug, including sensitivity to the initial aversive effects of a drug, can lead to increased drug intake which can set up further challenges to the reward circuits and interacting neural systems ( Figure 5 ). However, although largely neglected to date in genetic studies, one also can envision differential sensitivity to the sensitization of negative affective state or stress associated with removal of chronic administration of drugs, even independent of initial sensitivity to the drug or initial avidity for the drug. Clearly, the combination of all three factors–an initial high avidity for the drug, decreased sensitivity to the drug's initial aversive effects and increased sensitivity to the untoward consequences to the brain reward system of removal of chronic administration of the drug–would be a powerful contribution to vulnerability to addiction.
VULNERABILITY TO ADDICTION AND ALLOSTASIS: NEUROBIOLOGICAL MECHANISMS
Opponent-process counteradaptation in vulnerability to addiction.
The brain reward system presumably evolved to provide a motivational mechanism for survival and propagation of the species ( Nesse and Berridge 1997 ; Panksepp 1998 ; Stellar and Stellar 1985 ; Toates 1990 ). Rewards in the natural environment generally are associated with the seeking of nourishment, shelter and preservation of the species (i.e., reproduction). One can speculate that the basic mechanisms involved in reward regulation are the same mechanisms usurped by drugs of abuse. It follows then that the neuroadaptations in the brain reward system associated with an abundance of rewards produced by changes in the environment are the same neuroadaptations observed to drug rewards. As such, drugs of abuse have powerful neuropharmacological effects that may short circuit the neurochemistry of reward.
Using a conceptualization originally grounded in opponent-process theory ( Solomon and Corbit 1974 ), the effects of natural rewards on hedonic state are dependent on the nature of the reward and the frequency of delivery ( Figure 4 ). Rewards that have initial pleasurable effects can show some apparent tolerance and some evidence of a negative affective opponent-process. In contrast, rewards that have initial aversive effects can show tolerance to the aversive effects, a gradual increase in the positive affective opponent-process, and then subsequently a second opponent-process that may represent yet another negative affective state. Evidence for allostasis to the point of addiction with natural rewards is controversial, but compulsive behavior with impairment in social and occupational functioning has been observed with such events as gambling, shopping, exercise, eating, sex, and computer use ( Baumeister et al. 1994 ; Orford 1985 ). With the possible exception of gambling and perhaps certain eating disorders, the intensity and frequency of such disorders is less than that of drug addiction. A challenge for future research will be to understand whether such nondrug pathology has the same neurochemical underpinnings as drug addiction and produces a state of allostasis in the brain reward system that predisposes to drug addiction.
As described above, in drug addiction itself, the evidence for neuroadaptations to the reward system is overwhelming and can produce profound changes in reward set point ( Figure 4 ). Here, again grounded in opponent-process theory, drugs are conceptualized to have dramatic effects on hedonic processing that are time and drug dependent. Closely spaced administration of powerful reinforcing drugs can produce “apparent” tolerance to the initial rewarding effects and a pronounced “sensitization” of the negative affective state. This negative affective state is conceptualized to grow over time until the subject in effect simply is trying to return to a normal hedonic level ( Kissin and Gross 1968 ) ( Figure 4 ). Translated to the situation of the drug addict, the addict's reward system must work harder and harder to stay in the same place. Thus, in an allostatic view, stability is purchased at the cost of increased resources and decreased margin of error or reserve (B. Carroll, personal communication). This contrasts with a cost-neutral means of maintaining stability which characterizes homeostasis. The allostatic state conceptualized here is comprised of the residual neurochemical changes that may or may not be manifested as a behavioral phenotype but provide a biological transformation or “brain signature” that may be long lasting and involve neurobiological domains from motivation to memory.
Allostasis in the reward system can be the result of chronic drug administration ( Figure 5 ) but also may result from a history of environmental insult that falls into the realm of stress. Both perinatal and developmental stressors can contribute to the vulnerability of the brain and pituitary stress systems ( Caldji et al. 1998 ; Ladd et al. 2000 ), and such a vulnerability may impact directly on negative affective states that contribute to reward system allostasis. One also could imagine that particular experiences with nondrug rewards could interact with the same neurobiological substrates. Clearly, there appears to be a relationship between novelty-seeking, antisocial personality disorder, impulsivity and drug addiction (see above). Alternatively, impulse control per se may be a separate dimension which interacts with motivational states. Whether these disorders have some related or common neurochemical substrate with addiction remains to be determined.
Neurobiological Mechanisms in Vulnerability to Addiction
The neurobiological systems hypothesized to be responsible for the allostatic state of the reward system by the transition to addiction and the continuation and maintenance of addiction derive from multiple sources. First, the neurotransmitter systems involved in the acute reinforcing effects of drugs of abuse, such as dopamine, serotonin, opioid peptides, GABA and glutamate, are hypo-functional (see above). Second, the neurotransmitter systems associated with anti-reward and stress may be recruited to contribute to the state of allostasis in the reward system (see above). The hypothesis proposed here is that transmitter function involved in the acute reinforcing effects of drugs of abuse is compromised with the establishment of drug dependence ( Figure 5 , green) and that neurotransmitter function associated with anti-reward and stress ( Figure 5 , red), and the “compulsive” circuit of the cortico-striatal-thalamic loop ( Figure 5 , blue), are recruited during the development of drug dependence ( Figure 5 ).
A subhypothesis is that the core circuitry involved in the adaptations that form the state of allostasis in the reward system can be localized to elements of the extended amygdala, in particular the central nucleus of the amygdala, the bed nucleus of the stria terminalis, and the transition zone in the posterior shell region of the nucleus accumbens. Later, as allostasis continues to develop, it grows by recruitment of additional perturbed systems: an overactive brain stress system and an overactive compulsive behavior circuit. The allostatic state actually may pass into overt neural pathology as hypothesized for the role of cortical damage in the development of alcoholism ( Crews 1999 ). Neurotoxicity to the prefrontal cortex from high alcohol exposure has been hypothesized to contribute to disruption of executive function and consequent perseveration that could be an extension of dysfunction of the compulsive circuit of the cortico-striatal-thalamic loop ( Crews 1999 ).
A particular challenge for future research is to determine how cellular and molecular perturbations produced by chronic administration of drugs of abuse lead to the major changes in neurobiological systems outlined above. Data to date suggest that there are multiple molecular/cellular changes in brain neuronal systems produced by chronic administration of drugs of abuse. Noteworthy effects that appear common to many or all drugs of abuse with chronic administration are activation of glucocorticoid neuromodulatory systems in the nucleus accumbens and/or ventral tegmental area ( Barrot et al. 2000 ; Piazza and Le Moal 1996 , 1997 ), subsensitivity of dopamine autoreceptors in the ventral tegmental area ( White 1996 ), hypersensitivity of dopamine D1 receptor-mediated responses in the nucleus accumbens, increases in adenylyl cyclase activity, increases in protein kinase activity, increases in phosphorylation in the nucleus accumbens, and induction of transcription factors in the nucleus accumbens ( Koob et al. 1998b ; Nestler and Aghajanian 1997 ). While dysregulation of dopamine function can be linked to some of these cellular/molecular changes, in models even as well defined as psychomotor sensitization, the phenotypic manifestation of the behavioral response does not always correspond to the cellular/molecular mechanisms hypothesized. This suggests that there may be subtle underlying contributions to the phenotype that are as yet undiscovered, and that the precise nature of cellular/molecular changes underlying allostasis in the reward system remain to be uncovered.
DRUG RELAPSE AND ALLOSTASIS
Drug addiction is a chronic relapsing disorder characterized by cycles of drug abuse, abstinence and repeated attempts to stop. The vulnerability for relapse is poorly understood but clearly involves multiple factors such as drug availability, conditioning factors and stress, and hypothetically can be driven by a residual “brain signature” in any one of the three circuits dysregulated during the addiction cycle (see Figures 4 and 5 ). The majority of relapses occur during states of stress ( Marlatt and Gordon 1985 ) or situations with high drug cue or drug availability ( Marlatt and Gordon 1985 ). Behavioral treatments have been traditionally viewed as effective in helping prevent relapse and a limited number of effective pharmacological treatments have proven effective in preventing relapse ( Mason and Ownby 2000 ; Mason et al. 1999 ; Project MATCH Research Group 1997 ).
Embedded in the construct of vulnerability to relapse is another construct termed protracted abstinence which can be defined as a subtle state of residual abstinence after acute withdrawal. While originally defined in the context of physical symptoms associated with abstinence, the present conceptualization refers to a residual allostatic state within the brain reward system ( Figure 5 , green). It is important to emphasize that protracted abstinence can contribute to a vulnerability to relapse by a state change as opposed to a change in perception of any given stimulus. For example, a priming-like activation by a conditioned positive reinforcer in the context of high-circulating stress steroids superimposed upon an activated brain stress neurotransmitter system ( Figure 5 , red) may be a very powerful impetus for relapse (see below).
Stress Hormones, Dopamine and Vulnerability to Relapse
As established above, the relationship between stress and dopamine is to a large extent mediated by corticosterone (dopamine, stress, and stress hormones are elements of a pathophysiological chain of events and thus at the basis of various vulnerabilities, drug sensitivity included) ( McEwen 1998b ; Piazza et al. 1996a ). Stress hormones are highly lipophilic and enter into the brain where they are ubiquitously distributed to two families of receptors. These cytosolic receptors, while activated by the hormone, are translocated toward the nucleus and bind to DNA at specific regulatory genes to modulate their expression. The Type I mineralocorticoid receptors have a high affinity for corticosterone or cortisol and are concentrated mainly in limbic regions — the septum, amygdala and hippocampus. They have been shown to play a role in the modulation of responses to environmental and emotional stimuli and in the modulation of the stress axis. They modulate basal cortisol levels, circadian rhythms and hippocampal inhibition of the HPA axis (fast feedback) ( Figure 2A ).
The Type II glucocorticoid receptors have a lower affinity for corticosterone and are widely distributed with high density in the hypothalamus, on CRF and pro-opiomelanocortin (POMC) neurons, and also in the amygdala and hippocampus. They have been shown to regulate the genes involved in CRF and POMC secretion. They terminate the stress HPA response and are active in the delayed feedback regulation induced by glucocorticoids ( Albeck et al. 1994 ).
As a biological participant in the state of stress, stress hormones interact with limbic and cortical regions and regulate CRF-sympathetic systems ( Figure 2 ). Such stress hormones, via alterations of neuronal activity, consequently can induce neurobehavioral pathology. As noted above, dopaminergic neurons have receptors for glucocorticoids ( Härfstrand et al. 1986 ), and increases in the circulating levels of the hormones induce an increase of dopamine utilization in mesocorticolimbic neurons, an effect that is state-dependent (i.e., higher during the dark phase, or during food intake) ( Piazza and Le Moal 1997 ). Thus, increases in corticosteroids renders animals more vulnerable to the acute reinforcing effects of drugs of abuse ( Piazza and Le Moal 1997 ). Moreover, the hormones have by themselves reinforcing properties, both orally or intravenously at very high levels similar to those triggered by stressful situations ( Piazza et al. 1993 ). These stress hormones also elevate CRF gene expression in limbic regions and participate in anxiety, dysregulation of affective states, and chronic anticipatory angst ( Chrousos and Gold 1992 ; Johnson et al. 1992 ). Thus, stress hormones, by actions on dopamine neurons and on brain regions involved in drug actions, are directly involved in the dysregulation of reinforcement and globally involved in the development of dependence ( Piazza and Le Moal 1996 ).
Dysregulation of hypothalamic pituitary adrenal responsiveness may be a common element associated with protracted abstinence and vulnerability to relapse. In alcoholism, compromised HPA function has been demonstrated in actively drinking alcoholics and persists long after alcoholics cease alcohol consumption and includes blunted ACTH and/or cortisol responses to CRF or stress ( Adinoff et al. 1990 ). In addition, non-alcoholic subjects with a family history of alcoholism have abnormally blunted HPA responses to CRF ( Waltman et al. 1994 ). Animal studies have revealed that chronic administration of alcohol produces a decrease in anterior pituitary POMC mRNA concentrations weeks after withdrawal and led to the hypothesis that increased alcohol intake vulnerability may result from a dysregulation of the HPA axis (D. Rasmussen, personal communication). In heroin and cocaine addiction, both drug-free former heroin addicts and former cocaine addicts have shown hyperresponsivity to the metyrapone challenge test with excessive levels of ACTH released in response to the abrupt cutoff of the cortisol feedback at the level of the pituitary and hypothalamus (Kreek 1987 , 1992 ; Kreek et al 1984 ; Schluger et al. 1997 ). Whether such hyperresponsivity applies also to stressor challenges remains to be determined. Since, as described above, activation of the HPA axis can “drive” (e.g., activate) central extrahypothalamic CRF systems, such an activation of both brain and hormonal stress systems could contribute to a residual negative affective state and could be a powerful impetus for relapse.
Brain Stress Systems and Vulnerability to Relapse
Central nervous system stress systems also may be engaged during the development of drug dependence (see above), and residual changes in the activity of such brain stress systems during protracted abstinence may contribute to vulnerability to relapse ( Figure 5 , red). CRF and norepinephrine activity in ascending noradrenergic systems have been shown to be activated, while NPY systems may be inactivated, during acute withdrawal from most drugs of abuse ( Aston-Jones and Druhan 1999 ; Koob 1999a ). CRF antagonists have been shown to block stress-induced reinstatement of drug-seeking behavior in rats extinguished from intravenous self-administration ( Shaham et al. 1998 ). Even more compelling is the evidence suggesting that during protracted abstinence from alcohol, CRF antagonists show efficacy in rats with a history of dependence (A.J. Roberts, personal communication). Also, one hypothesis to explain the increased drinking in NPY knockout mice and alcohol-preferring rats is drinking to suppress a compensatory increase in a brain stress system such as CRF ( Richter et al. 2000 ; Thiele et al. 1998 ). How the combined dysregulation of circulating corticosteroids and central nervous system CRF and NPY systems interact during protracted abstinence to different drugs of abuse contribute to allostasis and increase vulnerability to relapse remains a challenge for future studies.
As noted above, relapse to drug-seeking in subjects with a history of addiction can be triggered by many factors such as stress, psychiatric comorbidity and psychosocial factors ( Figure 5 , blue). In the domain of psychosocial factors, conditioned responses to drug-related stimuli have been hypothesized to play an important role in triggering a state of “craving” and in initiating relapse. Animal models of such conditioned phenomena have centered on stimuli associated with drug-taking and a number of self-administration situations. Animals can be trained to self-administer a drug, and when drug delivery is paired with a previously neutral stimulus which can come to predict drug availability, animals can be trained in second-order schedules to work for a previously neutral stimulus that ultimately predicts drug availability. Using conditioned place preference, previously neutral stimuli (specific location) can be paired with drug administration and the animals can be tested for preference for the paired stimulus. Studies using these models have begun to establish a neurobiological substrate and the neuropharmacological components of such conditioned effects.
Conditioned reinforcement in general, and for drugs of abuse in particular, has been hypothesized to be integrated within the ventral striatum through convergent interactions with the amygdala ( Everitt et al. 1999 ). Lesions of the basolateral amygdala block the development of conditioned reinforcement in a variety of paradigms including an operant procedure where animals must learn a new task to obtain a previously neutral stimulus paired with a primary reinforcer ( Burns et al. 1993 ), conditioned place preference ( Hiroi and White 1991 ), and a second-order schedule of cocaine self-administration ( Whitelaw et al. 1996 ). Basolateral amygdala lesions also block the development of conditioned withdrawal in rats ( Schulteis et al. 2000 ). In contrast, the ventral subiculum appears to mediate the potentiation of locomotor activation and conditioned reinforcement by stimulant drugs but does not mediate informational aspects of the conditioned reinforcement process ( Everitt et al. 1999 ).
In a series of elegant studies extending this dichotomy to the projections of the basolateral amygdala and ventral subiculum it appears that excitotoxic lesions of the shell of the nucleus accumbens block the potentiation of conditioned reinforcement by stimulant drugs, similar to ventral subiculum lesions, but do not block the control over the instrumental behavior by the conditioned reinforcer ( Cador et al. 1989 ; Parkinson et al. 1999 ), whereas excitotoxic lesions of the core of the nucleus accumbens produce effects similar to basolateral amygdala lesions ( Parkinson et al. 1999 ). Consistent with these animal models, brain imaging studies in humans have shown that cue-induced cocaine craving is associated with activation of the amygdala and anterior cingulate cortex ( Childress et al. 1999 ; Maas et al. 1998 ). The neurochemical substrates underlying such conditioned reinforcement effects are under intense investigation but have been hypothesized to involve glutamatergic afferents from the basolateral amygdala and ventral subiculum ( Everitt et al. 1999 ) as well as dopaminergic projections to the basolateral amygdala and other cortical areas ( Berke and Hyman 2000 ). Low doses of dopaminergic antagonists can block the conditioned responding produced by a cocaine cue ( Weissenborn et al. 1996 ) and a D3 partial agonist-attenuated responding for the stimulus predicting cocaine reinforcement in a second-order schedule ( Pilla et al. 1999 ). However, acquisition of responding as a measure of conditioned reinforcement is unaltered by dopaminergic blockade suggesting that the dopaminergic component is the activation of responding for the conditioned reinforcement, not the informational content of the stimuli.
The implications of understanding the neurocircuitry of conditioned reinforcement for an allostatic view of addiction is that these pathways can provide the information necessary to activate primary reward circuits that may already be in a state of dysregulation. Recent emphasis has been placed on the role of “memory” in drug craving ( Berke and Hyman 2000 ). Others have argued that increased mesolimbic dopamine release may identify novel stimuli important for learning but that this activation rapidly habituates. However, with drugs of abuse this habituation does not occur ( Di Chiara 1999 ). Clearly, the amygdala and specifically the basolateral amygdala has a critical role in memory consolidation and particularly memory involving emotional arousal ( Cahill and McGaugh 1998 ; Cahill et al. 1995 ; McGaugh 2000 ). From the present perspective, memory of previous drug experiences and the associations formed by drugs being paired with previously neutral stimuli clearly have a role in unbalancing a reward system already under a heavy allostatic state. How the motivational impact of drug “memories” and drug conditioned reinforcers change with subjects under different levels of allostasis in the reward system, and how specific these drug-related memories are compared to generic emotional memories remains to be determined.
SUMMARY AND CONCLUSIONS
To summarize, the present perspective is that drug addiction represents an allostatic state in the brain reward system reflected in new reward set points, not only by dysregulation of reward circuits per se but also by the activation and recruitment of brain and hormonal stress responses. Allostasis is defined here as the process of achieving stability through change, and allostatic state is defined as a state of chronic deviation of the regulatory system from its normal operating (homeostatic) level. Allostasis from the drug addiction perspective is the process of maintaining apparent reward function stability by recruitment of changes in reward and stress system neurocircuitry. The allostatic state is a chronic deviation of reward thresholds. The hypothesis generated here is that counteradaptive processes such as opponent-processes, that are part of a normal homeostatic limitation of reward function, fail to return within the homeostatic range. Such dysregulations grow with repeated drug intake producing an allostatic state that drives further drug intake, and ultimately compulsive drug intake, and in turn exaggerates the allostatic state.
Dysregulation of reward neurotransmission is hypothesized to involve compromised function in the dopamine and opioid peptide systems at the molecular and cellular levels in specific components of the extended amygdala and constitutes part of the allostatic state of the brain reward system. Recruitment of brain and hormonal stress systems is hypothesized to involve both HPA axis and brain stress neurotransmission, notably CRF, NPY and norepinephrine systems, and also contributes to the allostatic state of the brain reward system. The manifestation of this allostatic state as compulsive drug-taking may be expressed through the neural circuits of the cortico-striatal-thalamic loops. The hypothesis is that these are the same circuits that are activated by other psychopathology involving repetitive compulsive behavior. The challenges for future research lie in understanding the vulnerability to addiction in all these neural circuits at all stages of the addiction cycle that contribute to such an allostatic-like pathology.
Abercrombie ED, Keller RW Jr, Zigmond MJ . (1988): Characterization of hippocampal norepinephrine release as measured by microdialysis perfusion: Pharmacological and behavioral studies. Neuroscience 27 : 897–904
Article CAS PubMed Google Scholar
Adinoff B, Martin PR, Bone GH, Eckardt MJ, Roehrich L, George DT, Moss HB, Eskay R, Linnoila M, Gold PW . (1990): Hypothalamic-pituitary-adrenal axis functioning and cerebrospinal fluid corticotropin releasing hormone and corticotropin levels in alcoholics after recent and long-term abstinence. Arch Gen Psychiatry 47 : 325–330
Ahima RS, Garcia MM, Harlan RE . (1992): Glucocorticoid regulation of preproenkephalin gene expression in the rat forebrain. Mol Brain Res 16 : 119–127
Ahmed SH, Koob GF . (1997): Cocaine- but not food-seeking behavior is reinstated by stress after extinction. Psychopharmacology 132 : 289–295
Ahmed SH, Koob GF . (1998): Transition from moderate to excessive drug intake: Change in hedonic set point. Science 282 : 298–300
Ahmed SH, Koob GF . (1999): Long-lasting increase in the set point for cocaine self-administration after escalation in rats. Psychopharmacology 146 : 303–312
Ahmed SH, Walker JR, Koob GF . (2000): Persistent increase in the motivation to take heroin in rats with a history of drug escalation. Neuropsychopharmacology 22 : 413–421
Albeck DS, Hastings NB, McEwen BS . (1994): Effects of adrenalectomy and type I or type II glucocorticoid receptor activation on AVP and CRH mRNA in the rat hypothalamus. Mol Brain Res 26 : 129–134
Alheid GF, Heimer L . (1988): New perspectives in basal forebrain organization of special relevance for neuropsychiatric disorders: The striatopallidal, amygdaloid, and corticopetal components of substantia innominata. Neuroscience 27 : 1–39
American Psychiatric Association. (1994): Diagnostic and Statistical Manual of Mental Disorders , 4th ed. Washington DC, American Psychiatric Press
Angulo JA, McEwen BS . (1994): Molecular aspects of neuropeptide regulation and function in the corpus striatum and nucleus accumbens. Brain Res Rev 19 : 1–18
Article PubMed Google Scholar
Anthony JC, Warner LA, Kessler RC . (1994): Comparative epidemiology of dependence on tobacco, alcohol, controlled substances, and inhalants: Basic findings from the National Comorbidity Survey. Exp Clin Psychopharmacol 2 : 244–268
Article Google Scholar
Aston-Jones G, Druhan J . (1999): Breaking the chain of addiction. Nature 400 : 317–319
Barrot M, Marinelli M, Abrous DN, Rouge-Pont F, Le Moal M, Piazza PV . (2000): The dopaminergic hyper-responsiveness of the shell of the nucleus accumbens is hormone-dependent. Eur J Neurosci 12 : 973–979
Baumeister RF, Heatherton TF, Tice DM . (1994): Losing Control: How and Why People Fail at Self-Regulation. San Diego, Academic Press
Baxter LR Jr, Sanjaya S, Brody AL, Ackermann RF, Colgan M, Schwartz JM, Allen-Martinez Z, Fuster JM, Phelps ME . (1996): Brain mediation of obsessive-compulsive disorder symptoms: Evidence from functional brain imaging studies in the human and nonhuman primate. Seminars Clin Neuropsychiatry 1 : 32–47
Google Scholar
Baxter LR Jr, Schwartz JM, Bergman KS, Szuba MP, Guze BH, Mazziotta JC, Alazraki A, Selin CE, Ferng HK, Munford P, Phelps ME . (1992): Caudate glucose metabolic rate changes with both drug and behavior therapy for obsessive-compulsive disorder. Arch Gen Psychiatry 49 : 681–689
Beninger RJ, Hahn BL . (1983): Pimozide blocks establishment but not expression of amphetamine-produced environment-specific conditioning. Science 220 : 1304–1306
Beninger RJ, Herz RS . (1986): Pimozide blocks establishment but not expression of cocaine-produced environment-specific conditioning. Life Sci 38 : 1425–1431
Beninger RJ, Hoffman DC, Mazurski EJ . (1989): Receptor subtype-specific dopaminergic agents and conditioned behavior. Neurosci Biobehav Rev 13 : 113–122
Bergman J, Kamien JB, Spealman RD . (1990): Antagonism of cocaine self-administration by selective dopamine D1 and D2 antagonists. Behav Pharmacol 1 : 355–363
Berke JD, Hyman SE . (2000): Addiction, dopamine, and the molecular mechanisms of memory. Neuron 25 : 515–532
Bernard C . (1865): Introduction a l'Etude de la Medecine Experimentale [Introduction to the Study of Experimental Medicine]. New York, J.B. Ballierre
Bierut LJ, Dinwiddie SH, Begleiter H, Crowe RR, Hesselbrock V, Nurnberger JI Jr, Porjesz B, Schuckit MA, Reich T . (1998): Familial transmission of substance dependence: Alcohol, marijuana, cocaine, and habitual smoking — a report from the Collaborative Study on the Genetics of Alcoholism. Arch Gen Psychiatry 55 : 982–988
Burchfield S . (1979): The stress responses: A new perspective. Psychosom Med 41 : 661–672
Burns LH, Robbins TW, Everitt BJ . (1993): Differential effects of excitotoxic lesions of the basolateral amygdala, ventral subiculum and medial prefrontal cortex on responding with conditioned reinforcement and locomotor activity potentiated by intra-accumbens infusions of D-amphetamine. Behav Brain Res 55 : 167–183
Cador M, Ahmed SH, Koob GF, Le Moal M, Stinus L . (1992): Corticotropin-releasing factor induces a place aversion independent of its neuroendocrine role. Brain Res 597 : 304–309
Cador M, Robbins TW, Everitt BJ . (1989): Involvement of the amygdala in stimulus-reward associations: interaction with the ventral striatum. Neuroscience 30 : 77–86
Cadoret R, Troughton E, Woodworth G . (1994): Evidence of heterogeneity of genetic effect in Iowa adoption studies. Ann N Y Acad Sci 708 : 59–71
Cadoret RJ, Troughton E, O'Gorman TW, Heywood E . (1986): An adoption study of genetic and environmental factors in drug abuse. Arch Gen Psychiatry 43 : 1131–1136
Cahill L, Babinsky R, Markowitsch HJ, McGaugh JL . (1995): The amygdala and emotional memory. Nature 377 : 295–296
Cahill L, McGaugh JL . (1998): Mechanisms of emotional arousal and lasting declarative memory. Trends Neurosci 21 : 294–299
Caine SB, Heinrichs SC, Coffin VL, Koob GF . (1995): Effects of the dopamine D1 antagonist SCH 23390 microinjected into the accumbens, amygdala or striatum on cocaine self-administration in the rat. Brain Res 692 : 47–56
Caine SB, Koob GF . (1993): Modulation of cocaine self-administration in the rat through D-3 dopamine receptors. Science 260 : 1814–1816
Caldji C, Tannenbaum B, Sharma S, Francis D, Plotsky PM, Meaney MJ . (1998): Maternal care during infancy regulates the development of neural systems mediating the expression of fearfulness in the rat. Proc Natl Acad Sci U S A 95 : 5335–5340
Article CAS PubMed PubMed Central Google Scholar
Cannon WB, Rosenblueth A . (1933): Studies on conditions of activity in endocrine organs. XXIX. Sympathin E and sympathin I. Am J Physiol 104 : 557–574
Article CAS Google Scholar
Carr LG, Foroud T, Bice P, Gobbett T, Ivashina J, Edenberg H, Lumeng L, Li TK . (1998): A quantitative trait locus for alcohol consumption in selectively bred rat lines. Alcohol Clin Exp Res 22 : 884–887
Carroll ME . (1985): The role of food deprivation in the maintenance and reinstatement of cocaine-seeking behavior in rats. Drug Alcohol Depend 16 : 95–109
Carroll ME, France CP, Meisch RA . (1979): Food deprivation increases oral and intravenous drug intake in rats. Science 205 : 319–321
Carroll ME, Lac ST . (1993): Autoshaping i.v. cocaine self-administration in rats: Effects of nondrug alternative reinforcers on acquisition. Psychopharmacology 110 : 5–12
Carroll ME, Lac ST, Nygaard SL . (1989): A concurrently available nondrug reinforcer prevents the acquisition or decreases the maintenance of cocaine-reinforced behavior. Psychopharmacology 97 : 23–29
Carroll ME, Rodefer JS . (1993): Income alters choice between drug and an alternative nondrug reinforcer in monkeys. Exp Clin Psychopharmacol 1 : 110–120
Celerier E, Laulin J-P, Larcher A, Le Moal M, Simonnet G . (1999): Evidence for opiate-activated NMDA processes masking opiate analgesia in rats. Brain Res 847 : 18–25
Chao HM, McEwen BS . (1990): Glucocorticoid regulation of preproenkephalin messenger ribonucleic acid in the rat striatum. Endocrinology 126 : 3124–3130
Chen JP, Paredes W, Lowinson JH, Gardner EL . (1991): Strain-specific facilitation of dopamine efflux by delta 9-tetrahydrocannabinol in the nucleus accumbens of rat: An in vivo microdialysis study. Neurosci Lett 129 : 136–180
Childress AR, Mozley PD, McElgin W, Fitzgerald J, Reivich M, O'Brien CP . (1999): Limbic activation during cue-induced cocaine craving. Am J Psychiatry 156 : 11–18
Chrousos GP, Gold PW . (1992): The concepts of stress and stress system disorders. Overview of physical and behavioral homeostasis. J Am Med Assoc 267 : 1244–1252
Collins AC, Bhat RV, Pauly JR, Marks MJ . (1990): Modulation of nicotine receptors by chronic exposure to nicotinic agonists and antagonists. In Bock G, Marsh J (eds), The Biology of Nicotine Dependence (Series title: Ciba Foundation Symposium, Vol 152 ). New York, John Wiley, pp 87–105
Colpaert FC . (1996): System theory of pain and of opiate analgesia: No tolerance to opiates. Pharmacol Rev 48 : 355–402
CAS PubMed Google Scholar
Corrigall WA, Coen KM, Adamson KL . (1994): Self-administered nicotine activates the mesolimbic dopamine system through the ventral tegmental area. Brain Res 653 : 278–284
Corrigall WA, Franklin KBJ, Coen KM, Clarke PBS . (1992): The mesolimbic dopaminergic system is implicated in the reinforcing effects of nicotine. Psychopharmacology 107 : 285–289
Crabbe JC . (1989): Genetic animal models in the study of alcoholism. Alcohol Clin Exp Res 13 : 120–127
Crabbe JC, Phillips TJ, Buck KJ, Cunningham CL, Belknap JK . (1999): Identifying genes for alcohol and drug sensitivity: Recent progress and future directions. Trends Neurosci 22 : 173–179
Crews FT . (1999): Alcohol and neurodegeneration. CNS Drug Rev 5 : 379–394
Cummings S, Elde R, Ells J, Lindall A . (1983): Corticotropin-releasing factor immunoreactivity is widely distributed within the central nervous system of the rat: An immunohistochemical study. J Neurosci 3 : 1355–1368
Dani JA, Heinemann S . (1996): Molecular and cellular aspects of nicotine abuse. Neuron 16 : 905–908
David V, Gold LH, Koob GF, Cazala P . (2000): Anxiogenic-like effects limit rewarding effects of cocaine in BALB/cByJ mice. Neuropsychopharmacology , In Press.
Davis M . (1997): Neurobiology of fear responses: The role of the amygdala. J Neuropsychiatry Clin Neurosci 9 : 382–402
De Kloet ER . (1991): Brain corticosteroid receptor balance and homeostatic control. Frontiers Neuroendocrinol 12 : 95–164
De la Garza R, Johanson CE . (1987): The effects of food deprivation on the self-administration of psychoactive drugs. Drug Alcohol Depend 19 : 17–27
de Quidt ME, Emson PC . (1986): Distribution of neuropeptide Y-like immunoreactivity in the rat central nervous system. II. Immunohistochemical analysis. Neuroscience 18 : 545–618
Deminiere JM, Piazza PV, Guegan G, Abrous N, Maccari S, Le Moal M, Simon H . (1992): Increased locomotor response to novelty and propensity to intravenous amphetamine self-administration in adult offspring of stressed mothers. Brain Res 586 : 135–139
Deminiere JM, Piazza PV, Le Moal M, Simon H . (1989): Experimental approach to individual vulnerability to psychostimulant addiction. Neurosci Biobehav Rev 13 : 141–147
Deroche V, Marinelli M, Le Moal M, Piazza PV . (1997): Glucocorticoids and behavioral effects of psychostimulants: II. Cocaine intravenous self-administration and reinstatement depend on glucocorticoid levels. J Pharmacol Exp Ther 281 : 1401–1407
Devor EJ, Cloninger CR . (1989): Genetics of alcoholism. Annu Rev Genet 23 : 19–36
Di Chiara G . (1999): Drug addiction as dopamine-dependent associative learning disorder. Eur J Pharmacol 375 : 13–30
Eckardt MJ, File SE, Gessa GL, Grant KA, Guerri C, Hoffman PL, Kalant H, Koob GF, Li TK, Tabakoff B . (1998): Effects of moderate alcohol consumption on the central nervous system. Alcohol Clin Exp Res 22 : 998–1040
Ehlers CL, Somes C, Lumeng L, Li TK . (1999): Electrophysiological response to neuropeptide Y (NPY) in alcohol-naive preferring and non-preferring rats. Pharmacol Biochem Behav 63 : 291–299
Engel JA, Enerback C, Fahlke C, Hulthe P, Hard E, Johannessen K, Svensson L, Soderpalm B . (1992): Serotonergic and dopaminergic involvement in ethanol intake. In Naranjo CA, Sellers EM (eds), Novel Pharmacological Interventions for Alcoholism. New York, Springer, pp 68–82
Chapter Google Scholar
Epping-Jordan MP, Markou A, Koob GF . (1998): The dopamine D-1 receptor antagonist SCH 23390 injected into the dorsolateral bed nucleus of the stria terminalis decreased cocaine reinforcement in the rat. Brain Res 784 : 105–115
Ettenberg A, Pettit HO, Bloom FE, Koob GF . (1982): Heroin and cocaine intravenous self-administration in rats: Mediation by separate neural systems. Psychopharmacology 78 : 204–209
Everitt BJ, Parkinson JA, Olmstead MC, Arroyo M, Robledo P, Robbins TW . (1999): Associative processes in addiction and reward: The role of amygdala-ventral striatal subsystems. Ann NY Acad Sci 877 : 412–438
Exner E, Clark D . (1993): Behaviour in the novel environment predicts responsiveness to d-amphetamine in the rat: A multivariate approach. Behav Pharmacol 4 : 47–56
Fitzgerald LW, Nestler EJ . (1995): Molecular and cellular adaptations in signal transduction pathways following ethanol exposure. Clin Neurosci 3 : 165–173
Fratta W, Martellotta MC, Cossu G, Fattore L . (1997): WIN 55, 212-2 induces intravenous self-administration in drug-naive mice. Society Neurosci Abstr 23 : 1869
Fride E, Weinstock M . (1989): Alterations in behavioral and striatal dopamine asymmetries induced by prenatal stress. Pharmacol Biochem Behav 32 : 425–430
Frye GD, Breese GR . (1982): GABAergic modulation of ethanol-induced motor impairment. J Pharmacol Exp Ther 223 : 750–756
Gardner EL, Paredes W, Smith D, Donner A, Milling C, Cohen D, Morrison D . (1988): Facilitation of brain stimulation reward by delta-9-tetrahydrocannabinol. Psychopharmacology 96 : 142–144
Gatto GJ, McBride WJ, Murphy JM, Lumeng L, Li TK . (1994): Ethanol self-infusion into the ventral tegmental area by alcohol-preferring rats. Alcohol 11 : 557–564
Gawin FH, Kleber HD . (1986): Abstinence symptomatology and psychiatric diagnosis in cocaine abusers: Clinical observations. Arch Gen Psychiatry 43 : 107–113
George FR, Goldberg SR . (1989): Genetic approaches to the analysis of addiction processes. Trends Pharmacol Sci 10 : 78–83
Goeders NE, Guerin GF . (1994): Non-contingent electric footshock facilities the acquisition of intravenous cocaine self-administration in rats. Psychopharmacology 114 : 63–70
Goldberg SR, Woods JH, Schuster CR . (1971): Nalorphine-induced changes in morphine self-administration in rhesus monkeys. J Pharmacol Exp Ther 176 : 464–471
Goodwin DW, Schulsinger F, Hermansen L, Guze SB, Winokur G . (1973): Alcohol problems in adoptees raised apart from alcoholic biological parents. Arch Gen Psychiatry 28 : 238–243
Grant S, London ED, Newlin DB, Villemagne VL, Liu X, Contoreggi C, Phillips RL, Kimes AS, Margolin A . (1996): Activation of memory circuits during cue-elicited cocaine craving. Proc Natl Acad Sci U S A 93 : 12040–12045
Gray TS, Bingaman EW . (1996): The amygdala: Corticotropin-releasing factor, steroids, and stress. Crit Rev Neurobiol 10 : 155–168
Graybiel AM, Aosaki T, Flaherty AW, Kimura M . (1994): The basal ganglia and adaptive motor control. Science 265 : 1826–1831
Härfstrand A, Fuxe K, Cintra A, Agnati LF, Zini I, Wikstrom AC, Okret S, Yu ZY, Goldstein M, Steinbusch H, Verhofstad A, Gustafsson J-A . (1986): Glucocorticoid receptor immunoreactivity in monoaminergic neurons of rat brain. Proc Natl Acad Sci U S A 83 : 9779–9783
Article PubMed PubMed Central Google Scholar
Heath AC, Bucholz KK, Madden PA, Dinwiddie SH, Slutske WS, Bierut LJ, Statham DJ, Dunne MP, Whitfield JB, Martin NG . (1997a): Genetic and environmental contributions to alcohol dependence risk in a national twin sample: Consistency of findings in women and men. Psychol Med 27 : 1381–1396
Heath AC, Madden PAF . (1998): Statistical methods in genetic research on smoking. Stat Methods Med Res 7 : 165–186
Heath AC, Slutske WS, Madden PAF . (1997b): Gender differences in the genetic contribution to alcoholism risk and to alcohol consumption patterns. In Wilsnack RW, Wilsnack SC (eds), Gender and Alcohol (Series title: Alcohol, Culture, and Social Control Monograph Series, Vol 3 ). New Brunswick NJ, Rutgers University Press, pp 114–149
Heilig M, Koob GF, Ekman R, Britton KT . (1994): Corticotropin-releasing factor and neuropeptide Y: Role in emotional integration. Trends Neurosci 17 : 80–85
Heimer L, Alheid G . (1991): Piercing together the puzzle of basal forebrain anatomy. In Napier TC, Kalivas PW, Hanin I (eds), The Basal Forebrain: Anatomy to Function. New York, Plenum Press, pp 1–42
Heimer L, Zahm DS, Churchill L, Kalivas PW, Wohltmann C . (1991): Specificity in the projection patterns of accumbal core and shell in the rat. Neuroscience 41 : 89–125
Heinrichs SC, Britton KT, Koob GF . (1991): Both conditioned taste preference and aversion induced by corticotropin-releasing factor. Pharmacol Biochem Behav 40 : 717–721
Heinrichs SC, Menzaghi F, Schulteis G, Koob GF, Stinus L . (1995): Suppression of corticotropin-releasing factor in the amygdala attenuates aversive consequences of morphine withdrawal. Behav Pharmacol 6 : 74–80
Hennessy JW, Levine S . (1979): Stress, arousal, and the pituitary-adrenal system: A psychoendocrine hypothesis. In Sprague JM, Epstein AN (eds), Progress in Psychobiology and Physiological Psychology , 8th ed. New York, Academic Press, pp 133–178
Henry C, Kabbaj M, Simon H, Le Moal M, Maccari S . (1994): Prenatal stress increases the hypothalamo-pituitary-adrenal axis response in young and adult rats. J Neuroendocrinol 6 : 341–345
Henry DJ, White FJ . (1991): Repeated cocaine administration causes persistent enhancement of D1 dopamine receptor sensitivity within the rat nucleus accumbens. J Pharmacol Exp Ther 258 : 882–890
Herkenham M, Lynn AB, Little MD, Johnson MR, Melvin LS, de Costa BR, Rice KC . (1990): Cannabinoid receptor localization in brain. Proc Natl Acad Sci U S A 87 : 1932–1936
Heyser CJ, Roberts AJ, Schulteis G, Koob GF . (1999): Central administration of an opiate antagonist decreases oral ethanol self-administration in rats. Alcohol Clin Exp Res 23 : 1468–1476
Higgins ST, Bickel WK, Hughes JR . (1994): Influence of an alternative reinforcer on human cocaine self-administration. Life Sci 55 : 179–187
Hiroi N, White NM . (1991): The lateral nucleus of the amygdala mediates expression of the amphetamine-produced conditioned place preference. J Neurosci 11 : 2107–2116
Hoffman PL, Rabe CS, Moses F, Tabakoff B . (1989): N-methyl-D-aspartate receptors and ethanol: Inhibition of calcium flux and cyclic GMP production. J Neurochem 52 : 1937–1940
Hooks MS, Colvin AC, Juncos JL, Justice JB Jr . (1992a): Individual differences in basal and cocaine-stimulated extracellular dopamine in the nucleus accumbens using quantitative microdialysis. Brain Res 587 : 306–312
Hooks MS, Jones GH, Juncos JL, Neill DB, Justice JB . (1994a): Individual differences in schedule-induced and conditioned behaviors. Behav Brain Res 60 : 199–209
Hooks MS, Jones GH, Liem BJ, Justice JB Jr . (1992b): Sensitization and individual differences to IP amphetamine, cocaine, or caffeine following repeated intracranial amphetamine infusions. Pharmacol Biochem Behav 43 : 815–823
Hooks MS, Jones GH, Neill DB, Justice JB Jr . (1992c): Individual differences in amphetamine sensitization: Dose-dependent effects. Pharmacol Biochem Behav 41 : 203–210
Hooks MS, Jones GH, Smith AD, Neill DB, Justice JB Jr . (1991): Response to novelty predicts the locomotor and nucleus accumbens dopamine response to cocaine. Synapse 9 : 121–128
Hooks MS, Juncos JL, Justice JB Jr, Meiergerd SM, Povlock SL, Schenk JO, Kalivas PW . (1994b): Individual locomotor response to novelty predicts selective alterations in D1 and D2 receptors and mRNAs. J Neurosci 14 : 6144–6152
Hrubec Z, Omenn GS . (1981): Evidence of genetic predisposition to alcoholic cirrhosis and psychosis: Twin concordances for alcoholism and its biological end points by zygosity among male veterans. Alcohol Clin Exp Res 5 : 207–215
Hwang BH, Zhang JK, Ehlers CL, Lumeng L, Li TK . (1999): Innate differences of neuropeptide Y (NPY) in hypothalamic nuclei and central nucleus of the amygdala between selectively bred rats with high and low alcohol preference. Alcohol Clin Exp Res 23 : 1023–1030
Hyman SE . (1996): Addiction to cocaine and amphetamine. Neuron 16 : 901–904
Hyytia P, Koob GF . (1995): GABAA receptor antagonism in the extended amygdala decreases ethanol self-administration in rats. Eur J Pharmacol 283 : 151–159
Jenck F, Moreau JL, Martin JR, Kilpatrick GJ, Reinscheid RK, Monsma FJ Jr, Nothacker HP, Civelli O . (1997): Orphanin FQ acts as an anxiolytic to attenuate behavioral responses to stress. Proc Natl Acad Sci USA 94 : 14854–14858
Jentsch JD, Taylor JR . (1999): Impulsivity resulting from frontostriatal dysfunction in drug abuse: Implications for the control of behavior by reward-related stimuli. Psychopharmacology 146 : 373–390
Joels M, de Kloet ER . (1992): Control of neuronal excitability by corticosteroid hormones. Trends Neurosci 15 : 25–30
Joels M, de Kloet ER . (1994): Mineralocorticoid and glucocorticoid receptors in the brain. Implications for ion permeability and transmitter systems. Prog Neurobiol 43 : 1–36
Johnson EO, Kamilaris TC, Chrousos GP, Gold PW . (1992): Mechanisms of stress: A dynamic overview of hormonal and behavioral homeostasis. Neurosci Biobehav Rev 16 : 115–130
Johnston JB . (1923): Further contributions to the study of the evolution of the forebrain. J Comp Neurol 35 : 337–481
June HL, Colker RE, Domangue KR, Perry LE, Hicks LH, June PL, Lewis MJ . (1992): Ethanol self-administration in deprived rats: Effects of Ro15–4513 alone, and in combination with flumazenil (Ro15–1788). Alcohol Clin Exp Res 16 : 11–16
Kaij L . (1960): Alcoholism in Twins: Studies on the Etiology and Sequels of Abuse of Alcohol. Stockholm, Almqvist and Wiksell
Kavaliers M . (1990): Inhibitory influences of mammalian FMRFamide (Phe-Met-Arg-Phe-amide)-related peptides on nociception and morphine- and stress-induced analgesia in mice. Neurosci Lett 115 : 307–312
Kessler RC, McGonagle KA, Zhao S, Nelson CB, Hughes M, Eshleman S, Wittchen HU, Kendler KS . (1994): Lifetime and 12-month prevalence of DSM-III-R psychiatric disorders in the United States: Results from the National Comorbidity Survey. Arch Gen Psychiatry 51 : 8–19
Kissin B, Gross MM . (1968): Drug therapy in alcoholism. Am J Psychiatry 125 : 31–41
Koob GF . (1992): Drugs of abuse: Anatomy, pharmacology, and function of reward pathways. Trends Pharmacol Sci 13 : 177–184
Koob GF . (1995): Animal models of drug addiction. In Bloom FE, Kupfer DJ (eds), Psychopharmacology: The Fourth Generation of Progress. New York, Raven Press, pp 759–772
Koob GF . (1999a): Corticotropin-releasing factor, norepinephrine and stress. Biol Psychiatry 46 : 1167–1180
Koob GF . (1999b): The role of the striatopallidal and extended amygdala systems in drug addiction. Ann N Y Acad Sci 877 : 445–460
Koob GF, Bloom FE . (1988): Cellular and molecular mechanisms of drug dependence. Science 242 : 715–723
Koob GF, Carrera R, Gold L, Heyser C, Maldonado-Irizarry C, Markou A, Parsons L, Roberts A, Schulteis G, Stinus L, Walker J, Weissenborn R, Weiss F . (1998a): Substance dependence as a compulsive behavior. J Psychopharmacol 12 : 39–48
Koob GF, Heinrichs SC, Menzaghi F, Pich EM, Britton KT . (1994): Corticotropin releasing factor, stress and behavior. Semin Neurosci 6 : 221–229
Koob GF, Le HT, Creese I . (1987): The D-1 dopamine receptor antagonist SCH 23390 increases cocaine self-administration in the rat. Neurosci Lett 79 : 315–320
Koob GF, Le Moal M . (1997): Drug abuse: Hedonic homeostatic dysregulation. Science 278 : 52–58
Koob GF, Markou A, Weiss F, Schulteis G . (1993): Opponent process and drug dependence: Neurobiological mechanisms. Semin Neurosci 5 : 351–358
Koob GF, Pettit HO, Ettenberg A, Bloom FE . (1984): Effects of opiate antagonists and their quaternary derivatives on heroin self-administration in the rat. J Pharmacol Exp Ther 229 : 481–486
Koob GF, Sanna PP, Bloom FE . (1998b): Neuroscience of addiction. Neuron 21 : 467–476
Korf J, Aghajanian GK, Roth RH . (1973): Increased turnover of norepinephrine in the rat cerebral cortex during stress: Role of the locus coeruleus. Neuropharmacology 12 : 933–938
Kornetsky C, Bain G . (1990): Brain-stimulation reward: A model for drug induced euphoria. In Adler MW, Cowan A (eds), Modern Methods in Pharmacology Testing and Evaluation of Drugs of Abuse. New York, Wiley-Liss, pp 211–231
Koskenvuo M, Langinvainio H, Kaprio J, Lonnqvist J, Tienari P . (1984): Psychiatric hospitalization in twins. Acta Genet Med Gemellol 33 : 321–332
Kreek MJ . (1987): Multiple drug abuse patterns and medical consequences. In Meltzer HY (ed), Psychopharmacology: The Third Generation of Progress. New York, Raven Press, pp 1597–1604
Kreek MJ . (1992): Rationale for maintenance pharmacotherapy of opiate dependence. In O'Brien CP, Jaffe JH (eds), Addictive States. New York, Raven Press, pp 205–230
Kreek MJ, Koob GF . (1998): Drug dependence: Stress and dysregulation of brain reward pathways. Drug Alcohol Depend 51 : 23–47
Kreek MJ, Ragunath J, Plevy S, Hamer D, Schneider B, Hartman N . (1984): ACTH, cortisol and beta-endorphin response to metyrapone testing during chronic methadone maintenance treatment in humans. Neuropeptides 5 : 277–278
Ladd CO, Huot RL, Thrivikraman KV, Nemeroff CB, Meaney MJ, Plotsky PM . (2000): Long-term behavioral and neuroendocrine adaptations to adverse early experience. Prog Brain Res 122 : 81–103
Lake JR, Hebert KM, Payza K, Deshotel KD, Hausam DD, Witherspoon WE, Arcangeli KA, Malin DH . (1992): Analog of neuropeptide FF attenuates morphine tolerance. Neurosci Lett 146 : 203–206
Larcher A, Laulin JP, Celerier E, Le Moal M, Simonnet G . (1998): Acute tolerance associated with a single opiate administration: Involvement of N-methyl-D-aspartate-dependent pain facilitatory systems. Neuroscience 84 : 583–589
Laulin JP, Celerier E, Larcher A, Le Moal M, Simonnet G . (1999): Opiate tolerance to daily heroin administration: An apparent phenomenon associated with enhanced pain sensitivity. Neuroscience 89 : 631–636
Laulin J-P, Larcher A, Celerier E, Le Moal M, Simonnet G . (1998): Long-lasting increased pain sensitivity in rat following exposure to heroin for the first time. Eur J Neurosci 10 : 782–785
Lee Y, Schulkin J, Davis M . (1994): Effect of corticosterone on the enhancement of the acoustic startle reflex by corticotropin releasing factor (CRF). Brain Res 666 : 93–98
Legault M, Wise RA . (1994): Effects of withdrawal from nicotine on intracranial self-stimulation. Society Neurosci Abstr 20 : 1032
Leith NJ, Barrett RJ . (1976): Amphetamine and the reward system: Evidence for tolerance and post-drug depression. Psychopharmacologia 46 : 19–25
Leone P, Di Chiara G . (1987): Blockade of D1 receptors by SCH 23390 antagonizes morphine- and amphetamine-induced place preference conditioning. Eur J Pharmacol 135 : 251–254
Le Moal M . (1995): Mesocorticolimbic dopaminergic neurons: Functional and regulatory roles. In Bloom FE, Kupfer DJ (eds), Psychopharmacology: The Fourth Generation of Progress. New York, Raven Press, pp 283–294
Le Moal M, Simon H . (1991): Mesocorticolimbic dopaminergic network: Functional and regulatory roles. Physiol Rev 71 : 155–234
Lepore M, Liu X, Savage V, Matalon D, Gardner EL . (1996): Genetic differences in delta 9-tetrahydrocannabinol-induced facilitation of brain stimulation reward as measured by a rate-frequency curve-shift electrical brain stimulation paradigm in three different rat strains. Life Sci 58 : PL365–PL372
Lepore M, Vorel SR, Lowinson J, Gardner EL . (1995): Conditioned place preference induced by delta 9-tetrahydrocannabinol: Comparison with cocaine, morphine, and food reward. Life Sci 56 : 2073–2080
Le Sage MG, Stafford D, Glowa JR . (1999): Preclinical research on cocaine self-administration: Environmental determinants and their interaction with pharmacological treatment. Neurosci Biobehav Rev 23 : 717–741
Leshner AI . (1999): Science is revolutionizing our view of addiction–and what to do about it. Am J Psychiatry 156 : 1–3
Li TK . (2000): Pharmacogenetics of responses to alcohol and genes that influence alcohol drinking. J Stud Alcohol 61 : 5–12
Liljequist S, Engel J . (1982): Effects of GABAergic agonists and antagonists on various ethanol-induced behavioral changes. Psychopharmacology 78 : 71–75
Lovinger DM, White G, Weight FF . (1989): Ethanol inhibits NMDA-activated ion current in hippocampal neurons. Science 243 : 1721–1724
Maas LC, Lukas SE, Kaufman MJ, Weiss RD, Daniels SL, Rogers VW, Kukes TJ, Renshaw PF . (1998): Functional magnetic resonance imaging of human brain activation during cue-induced cocaine craving. Am J Psychiatry 155 : 124–126
Maccari S, Piazza PV, Deminiere JM, Lemaire V, Mormede P, Simon H, Angelucci L, Le Moal M . (1991): Life events-induced decrease of corticosteroid type I receptors is associated with reduced corticosterone feedback and enhanced vulnerability to amphetamine self-administration. Brain Res 547 : 7–12
Macey DJ, Koob GF, Markou A . (2000): CRF and urocortin decreased brain stimulation reward in the rat: Reversal by a CRF receptor antagonist. Brain Res 866 : 82–91
Maldonado R, Robledo P, Chover AJ, Caine SB, Koob GF . (1993): D1 dopamine receptors in the nucleus accumbens modulate cocaine self-administration in the rat. Brain Res 622 : 105–112
Malin DH, Lake JR, Carter VA, Cunningham JS, Hebert KM, Conrad DL, Wilson OB . (1994): The nicotine antagonist mecamylamine precipitates nicotine abstinence syndrome in the rat. Psychopharmacology 115 : 180–184
Malin DH, Lake JR, Carter VA, Cunningham JS, Wilson OB . (1993): Naloxone precipitates nicotine abstinence syndrome in the rat. Psychopharmacology 112 : 339–342
Malin DH, Lake JR, Hammond MV, Fowler DE, Rogillio RB, Brown SL, Sims JL, Leecraft BM, Yang HYT . (1990): FMRF-NH2-like mammalian octapeptide: Possible role in opiate dependence and abstinence. Peptides 11 : 969–972
Malin DH, Lake JR, Short PE, Blossman JB, Lawless BA, Schopen CK, Sailer EE, Burgess K, Wilson OB . (1996): Nicotine abstinence syndrome precipitated by an analog of neuropeptide FF. Pharmacol Biochem Behav 54 : 581–585
Markou A, Koob GF . (1991): Postcocaine anhedonia: An animal model of cocaine withdrawal. Neuropsychopharmacology 4 : 17–26
Markou A, Koob GF . (1992): Construct validity of a self-stimulation threshold paradigm: Effects of reward and performance manipulations. Physiol Behav 51 : 111–119
Markou A, Weiss F, Gold LH, Caine SB, Schulteis G, Koob GF . (1993): Animal models of drug craving. Psychopharmacology 112 : 163–182
Marlatt GA, Gordon JR . (1985): Relapse Prevention. New York, Guilford
Mason BJ, Ownby RL . (2000): Acamprosate for the treatment of alcohol dependence: A review of double-blind, placebo-controlled trials. CNS Spectrums 5 : 58–69
Mason BJ, Salvato FR, Williams LD, Ritvo EC, Cutler RB . (1999): A double-blind, placebo-controlled study of oral nalmefene for alcohol dependence. Arch Gen Psychiatry 56 : 719–724
Matthes HW, Maldonado R, Simonin F, Valverde O, Slowe S, Kitchen I, Befort K, Dierich A, Le Meur M, Dolle P, Tzavara E, Hanoune J, Roques B, Kieffer BL . (1996): Loss of morphine-induced analgesia, reward effect and withdrawal symptoms in mice lacking the mu-opioid-receptor gene. Nature 383 : 819–823
McBride WJ, Li TK . (1998): Animal models of alcoholism: Neurobiology of high alcohol-drinking behavior in rodents. Crit Rev Neurobiol 12 : 339–369
McEwen BS . (1995): Adrenal steroid actions on brain: Dissecting the fine line between protection and damage. In Friedman MJ, Charney DS, Deutch AY (eds), Neurobiological and Clinical Consequences of Stress: From Normal Adaptation to PTSD. Philadelphia, Lippincott-Raven, pp 135–147
McEwen BS . (1998a): Stress, adaptation, and disease: Allostasis and allostatic load. Ann N Y Acad Sci 840 : 33–44
McEwen BS . (1998b): Protective and damaging effects of stress mediators. Seminars Med Beth Israel Deaconess Med Ctr 338 : 171–179
CAS Google Scholar
McEwen BS . (2000): Homeostasis. In Fink G (ed), Encyclopedia of Stress , Vol 2 , E-M. San Diego, Academic Press, pp 399–400
McEwen BS, Stellar E . (1993): Stress and the individual: Mechanisms leading to disease. Arch Intern Med 153 : 2093–2101
McGaugh JL . (2000): Memory: A century of consolidation. Science 287 : 248–251
McLellan AT, Arndt IO, Metzger DS, Woody GE, O'Brien CP . (1993): The effects of psychosocial services in substance abuse treatment. J Am Med Assoc 269 : 1953–1959
Merikangas KR, Stolar M, Stevens DE, Goulet J, Preisig MA, Fenton B, Zhang H, O'Malley SS, Rounsaville BJ . (1998): Familial transmission of substance use disorders. Arch Gen Psychiatry 55 : 973–979
Merlo-Pich E, Lorang M, Yeganeh M, Rodriguez de Fonseca F, Raber J, Koob GF, Weiss F . (1995): Increase of extracellular corticotropin-releasing factor-like immunoreactivity levels in the amygdala of awake rats during restraint stress and ethanol withdrawal as measured by microdialysis. J Neurosci 15 : 5439–5447
Modell JG, Mountz JM, Curtis GC, Greden JF . (1989): Neurophysiologic dysfunction in basal ganglia/limbic striatal and thalamocortical circuits as a pathogenetic mechanism of obsessive-compulsive disorder. J Neuropsychiatry Clin Neurosci 1 : 27–36
Mogil JS, Grisel JE, Zhangs G, Belknap JK, Grandy DK . (1996): Functional antagonism of mu-, delta- and kappa-opioid antinociception by orphanin FQ. Neurosci Lett 214 : 131–134
Moller C, Wiklund L, Sommer W, Thorsell A, Heilig M . (1997): Decreased experimental anxiety and voluntary ethanol consumption in rats following central but not basolateral amygdala lesions. Brain Research 760 : 94–101
Morency MA, Beninger RJ . (1986): Dopaminergic substrates of cocaine-induced place conditioning. Brain Res 399 : 33–41
Moyer JA, Herrenkohl LR, Jacobowitz DM . (1978): Stress during pregnancy: Effect on catecholamines in discrete brain regions of offspring as adults. Brain Res 144 : 173–178
Negus SS, Henriksen SJ, Mattox A, Pasternak GW, Portoghese PS, Takemori AE, Weinger MB, Koob GF . (1993): Effect of antagonists selective for mu, delta and kappa opioid receptors on the reinforcing effects of heroin in rats. J Pharmacol Exp Ther 265 : 1245–1252
Nesse RM, Berridge KC . (1997): Psychoactive drug use in evolutionary perspective. Science 278 : 63–66
Nestler EJ . (1996): Under siege: The brain on opiates. Neuron 16 : 897–900
Nestler EJ, Aghajanian GK . (1997): Molecular and cellular basis of addiction. Science 278 : 58–63
Nestler EJ, Kelz MB, Chen J . (1999): FosB: A molecular mediator of long-term neural and behavioral plasticity. Brain Res 835 : 10–17
O'Brien CP . (1997): A range of research-based pharmacotherapies for addiction. Science 278 : 66–70
Orford J . (1985): A Psychological View of Addiction. Chichester, John Wiley
Palmiter RD, Erickson JC, Hollopeter G, Baraban SC, Schwartz MW . (1998): Life without neuropeptide Y. Recent Prog Horm Res 53 : 163–199
Panksepp J . (1998): Affective Neuroscience: The Foundations of Human and Animal Emotions. New York, Oxford University Press
Parkinson JA, Olmstead MC, Burns LH, Robbins TW, Everitt BJ . (1999): Dissociation in effects of lesions of the nucleus accumbens core and shell on appetitive pavlovian approach behavior and the potentiation of conditioned reinforcement and locomotor activity by D-amphetamine. J Neurosci 19 : 2401–2411
Parsons LH, Koob GF, Weiss F . (1995): Serotonin dysfunction in the nucleus accumbens of rats during withdrawal after unlimited access to intravenous cocaine. J Pharmacol Exp Ther 274 : 1182–1191
Pettit HO, Ettenberg A, Bloom FE, Koob GF . (1984): Destruction of dopamine in the nucleus accumbens selectively attenuates cocaine but not heroin self-administration in rats. Psychopharmacology 84 : 167–173
Pfeffer AO, Samson HH . (1988): Haloperidol and apomorphine effects on ethanol reinforcement in free-feeding rats. Pharmacol Biochem Behav 29 : 343–350
Phillips AG, Fibiger HC . (1987): Anatomical and neurochemical substrates of drug reward determined by the conditioned place preference technique. In Bozarth MA (ed), Methods of Assessing the Reinforcing Properties of Abused Drugs. New York, Springer-Verlag, pp 275–290
Phillips TJ, Crabbe JC, Metten P, Belknap JK . (1994): Localization of genes affecting alcohol drinking in mice. Alcohol Clin Exp Res 18 : 931–941
Piazza PV, Deminiere JM, Le Moal M, Simon H . (1989): Factors that predict individual vulnerability to amphetamine self-administration. Science 245 : 1511–1513
Piazza PV, Deroche V, Deminiere JM, Maccari S, Le Moal M, Simon H . (1993): Corticosterone in the range of stress-induced levels possesses reinforcing properties: Implications for sensation-seeking behaviors. Proc Natl Acad Sci U S A 90 : 11738–11742
Piazza PV, Deroche V, Rouge-Pont F, Le Moal M . (1998): Behavioral and biological factors associated with individual vulnerability to psychostimulant abuse. NIDA Res Monogr 169 : 105–133
Piazza PV, Deroche-Gamonent V, Rouge-Pont F, Le Moal M . (2000): Vertical shifts in self-administration dose-response functions predict a drug-vulnerable phenotype predisposing to addiction. J Neurosci 20 : 4226–4232
Piazza PV, Le Moal M . (1997): Glucocorticoids as a biological substrate of reward: Physiological and pathophysiological implications. Brain Res Rev 25 : 359–372
Piazza PV, Le Moal ML . (1996): Pathophysiological basis of vulnerability to drug abuse: Role of an interaction between stress, glucocorticoids, and dopaminergic neurons. Annu Rev Pharmacol Toxicol 36 : 359–378
Piazza PV, Maccari S, Deminiere JM, Le Moal M, Mormede P, Simon H . (1991a): Corticosterone levels determine individual vulnerability to amphetamine self-administration. Proc Natl Acad Sci U S A 88 : 2088–2092
Piazza PV, Marinelli M, Rouge-Pont F, Deroche V, Maccari S, Simon H, Le Moal M . (1996a): Stress, glucocorticoids, and mesencephalic dopaminergic neurons: A pathophysiological chain determining vulnerability to psychostimulant abuse. Nida Res Monogr 163 : 277–299
Piazza PV, Rouge-Pont F, Deminiere JM, Kharoubi M, Le Moal M, Simon H . (1991b): Dopaminergic activity is reduced in the prefrontal cortex and increased in the nucleus accumbens of rats predisposed to develop amphetamine self-administration. Brain Res 567 : 169–174
Piazza PV, Rouge-Pont F, Deroche V, Maccari S, Simon H, Le Moal M . (1996b): Glucocorticoids have state-dependent stimulant effects on the mesencephalic dopaminergic transmission. Proc Natl Acad Sci U S A 93 : 8716–8720
Pilla M, Perachon S, Sautel F, Garrido F, Mann A, Wermuth CG, Schwartz JC, Everitt BJ, Sokoloff P . (1999): Selective inhibition of cocaine-seeking behaviour by a partial dopamine D3 receptor agonist. Nature 400 : 371–375
Pontieri FE, Tanda G, Di Chiara G . (1995): Intravenous cocaine, morphine, and amphetamine preferentially increase extracellular dopamine in the “shell” as compared with the “core” of the rat nucleus accumbens. Proc Natl Acad Sci U S A 92 : 12304–12308
Pontieri FE, Tanda G, Orzi F, Di Chiara G . (1996): Effects of nicotine on the nucleus accumbens and similarity to those of addictive drugs. Nature 382 : 255–257
Post RM, Weiss SRB . (1995): The neurobiology of treatment-resistant mood disorders. In Bloom FE, Kupfer DJ (eds), Psychopharmacology: The Fourth Generation of Progress. New York, Raven Press, pp 1155–1170
Poulos CX, Cappell H . (1991): Homeostatic theory of drug tolerance: A general model of physiological adaptation. Psychol Rev 98 : 390–408
Project MATCH Research Group. (1997): Matching alcoholism treatments to client heterogeneity: Project MATCH posttreatment drinking outcomes. J Stud Alcohol 58 : 7–29
Rassnick S, D'Amico E, Riley E, Koob GF . (1993a): GABA antagonist and benzodiazepine partial inverse agonist reduce motivated responding for ethanol. Alcohol Clin Exp Res 17 : 124–130
Rassnick S, Heinrichs SC, Britton KT, Koob GF . (1993b): Microinjection of a corticotropin-releasing factor antagonist into the central nucleus of the amygdala reverses anxiogenic-like effects of ethanol withdrawal. Brain Res 605 : 25–32
Rassnick S, Stinus L, Koob GF . (1993c): The effects of 6-hydroxydopamine lesions of the nucleus accumbens and the mesolimbic dopamine system on oral self-administration of ethanol in the rat. Brain Res 623 : 16–24
Richards G, Schoch P, Haefely W . (1991): Benzodiazepine receptors: New vistas. Semin Neurosci 3 : 191–203
Richter RM, Weiss F . (1999): In vivo CRF release in rat amygdala is increased during cocaine withdrawal in self-administering rats. Synapse 32 : 254–261
Richter RM, Zorrilla EP, Basso AM, Koob GF, Weiss F . (2000): Altered amygdalar CRF release and increased anxiety-like behavior in Sardinian alcohol preferring rats: A microdialysis and behavioral study. Alcohol Clin Exp Res , in press.
Robbins TW, Everitt BJ . (1995): Central norepinephrine neurons and behavior. In Bloom FE, Kupfer DJ (eds), Psychopharmacology: The Fourth Generation of Progress. New York, Raven Press, pp 363–372
Roberts AJ, Cole M, Koob GF . (1996): Intra-amygdala muscimol decreases operant ethanol self-administration in dependent rats. Alcohol Clin Exp Res 20 : 1289–1298
Roberts AJ, Heyser CJ, Cole M, Griffin P, Koob GF . (2000a): Excessive ethanol drinking following a history of dependence: Animal model of allostasis. Neuropsychopharmacology 22 : 581–594
Roberts AJ, Heyser CJ, McDonald JS, Kieffer BL, Matthes HWD, Koob GF, Gold LH . (2000b): Mu opioid receptor knockout mice do not self-administer alcohol. J Pharmacol Exp Ther 293 : 1002–1008
Roberts AJ, McArthur RA, Hull EE, Post C, Koob GF . (1998): Effects of amperozide, 8-OH-DPAT, and FG 5974 on operant responding for ethanol. Psychopharmacology 137 : 25–32
Roberts DC, Corcoran ME, Fibiger HC . (1977): On the role of ascending catecholaminergic systems in intravenous self-administration of cocaine. Pharmacol Biochem Behav 6 : 615–620
Roberts DCS, Koob GF, Klonoff P, Fibiger HC . (1980): Extinction and recovery of cocaine self-administration following 6-hydroxydopamine lesions of the nucleus accumbens. Pharmacol Biochem Behav 12 : 781–787
Robinson TE, Berridge KC . (1993): The neural basis of drug craving: An incentive-sensitization theory of addiction. Brain Res Rev 18 : 247–291
Rodriguez de Fonseca F, Carrera MRA, Navarro M, Koob GF, Weiss F . (1997): Activation of corticotropin-releasing factor in the limbic system during cannabinoid withdrawal. Science 276 : 2050–2054
Rouge-Pont F, Marinelli M, Le Moal M, Simon H, Piazza PV . (1995): Stress-induced sensitization and glucocorticoids. II. Sensitization of the increase in extracellular dopamine induced by cocaine depends on stress-induced corticosterone secretion. J Neurosci 15 : 7189–7195
Rouge-Pont F, Piazza PV, Kharouby M, Le Moal M, Simon H . (1993): Higher and longer stress-induced increase in dopamine concentrations in the nucleus accumbens of animals predisposed to amphetamine self-administration: A microdialysis study. Brain Res 602 : 169–174
Rudnick G, Clark J . (1993): From synapse to vesicle: The reuptake and storage of biogenic amine neurotransmitters. Biochim Biophys Acta 1144 : 249–263
Russell MAH . (1976): What is dependence? In Edwards G (ed), Drugs and Drug Dependence. Lexington MA, Lexington Books, pp 182–187
Samson HH, Tolliver GA, Pfeffer AO, Sadeghi KG, Mills FG . (1987): Oral ethanol reinforcement in the rat: Effect of the partial inverse benzodiazepine agonist RO15-4513. Pharmacol Biochem Behav 27 : 517–519
Sarnyai Z, Biro E, Gardi J, Vecsernyes M, Julesz J, Telegdy G . (1995): Brain corticotropin-releasing factor mediates “anxiety-like” behavior induced by cocaine withdrawal in rats. Brain Res 675 : 89–97
Schenk S, Partridge B . (1997): Sensitization and tolerance in psychostimulant self-administration. Pharmacol Biochem Behav 57 : 543–550
Schluger J, Bodner G, Gunduz M, Ho A, Kreek MJ . (1997): Abnormal metyrapone tests during cocaine abstinence. NIDA Res Monogr 178 : 105
Schoffelmeer AN, Voorn P, Jonker AJ, Wardeh G, Nestby P, Vanderschuren LJ, De Vries TJ, Mulder AH, Tjon GH . (1996): Morphine-induced increase in D-1 receptor regulated signal transduction in rat striatal neurons and its facilitation by glucocorticoid receptor activation: Possible role in behavioral sensitization. Neurochem Res 21 : 1417–1423
Schuckit MA . (1994): Low level of response to alcohol as a predictor of future alcoholism. Am J Psychiatry 151 : 184–189
Schulkin J, Gold PW, McEwen BS . (1998): Induction of corticotropin-releasing hormone gene expression by glucocorticoids: Implication for understanding the states of fear and anxiety and allostatic load. Psychoneuroendocrinology 23 : 219–243
Schulkin J, McEwen BS, Gold PW . (1994): Allostasis, amygdala, and anticipatory angst. Neurosci Biobehav Rev 18 : 385–396
Schulteis G, Ahmed SH, Morse AC, Koob GF, Everitt BJ . (2000): Conditioning and opiate withdrawal: The amygdala links neutral stimuli with the agony of overcoming drug addiction. Nature 405 : 1013–1014
Schulteis G, Heyser CJ, Koob GF . (1997): Opiate withdrawal signs precipitated by naloxone following a single exposure to morphine: Potentiation with a second morphine treatment. Psychopharmacology 129 : 56–65
Schulteis G, Markou A, Gold LH, Stinus L, Koob GF . (1994): Relative sensitivity to naloxone of multiple indices of opiate withdrawal: A quantitative dose-response analysis. J Pharmacol Exp Ther 271 : 1391–1398
Schwartz JM . (1997): Obsessive-compulsive disorder. Sci Med 4 : 14–23
Schwartz JM . (1999): A role for volition and attention in the generation of new brain circuitry: Towards a neurobiology of mental force. J Consciousness Stud 6 : 115–142
Self DW, Nestler EJ . (1995): Molecular mechanisms of drug reinforcement and addiction. Annu Rev Neurosci 18 : 463–495
Selye H . (1936): A syndrome produced by diverse nocuous agents. Nature 138 : 32
Selye H . (1976): The Stress of Life. New York, McGraw-Hill
Shaham Y, Erb S, Leung S, Buczek Y, Stewart J . (1998): CP-154,526, a selective, non-peptide antagonist of the corticotropin-releasing factor1 receptor attenuates stress-induced relapse to drug seeking in cocaine- and heroin-trained rats. Psychopharmacology 137 : 184–190
Shepard JD, Barron KW, Myers DA . (2000): Corticosterone delivery to the amygdala increases corticotropin-releasing factor mRNA in the central amygdaloid nucleus and anxiety-like behavior. Brain Res 861 : 288–295
Sher KJ . (1991): Children of Alcoholics: A Critical Appraisal of Theory and Research. Chicago, University of Chicago Press
Shippenberg TS, Herz A, Spanagel R, Bals-Kubik R, Stein C . (1992): Conditioning of opioid reinforcement: Neuroanatomical and neurochemical substrates. Ann N Y Acad Sci 654 : 347–356
Siegel S . (1975): Evidence from rats that morphine tolerance is a learned response. J Comp Physiol Psychol 89 : 489–506
Slot LA, Colpaert FC . (1999): Opiate states of memory: Receptor mechanisms. J Neurosci 19 : 10520–10529
Article CAS PubMed Central Google Scholar
Solomon RL, Corbit JD . (1974): An opponent-process theory of motivation. I. Temporal dynamics of affect. Psychol Rev 81 : 119–145
Spyraki C, Fibiger HC, Phillips AG . (1983): Attenuation of heroin reward in rats by disruption of the mesolimbic dopamine system. Psychopharmacology 79 : 278–283
Stanford SC, Salmon P . (1993): Stress: From Synapse to Syndrome. San Diego, Academic Press
Stellar JR, Stellar E . (1985): The Neurobiology of Motivation and Reward. New York, Springer-Verlag
Sterling P, Eyer J . (1988): Allostasis: A new paradigm to explain arousal pathology. In Fisher S, Reason J (eds), Handbook of Life Stress, Cognition and Health. Chichester, John Wiley, pp 629–649
Sterling P, Eyer J . (1981): Biological basis of stress-related mortality. Social Sci Med Part E: Med Psychol 15 : 3–42
Stinus L, Le Moal M, Koob GF . (1990): Nucleus accumbens and amygdala are possible substrates for the aversive stimulus effects of opiate withdrawal. Neuroscience 37 : 767–773
Stinus L, Nadaud D, Deminiere JM, Jauregui J, Hand TT, Le Moal M . (1989): Chronic flupentixol treatment potentiates the reinforcing properties of systemic heroin administration. Biol Psychiatry 26 : 363–371
Sutton MA, Karanian DA, Self DW . (2000): Factors that determine a propensity for cocaine-seeking behavior during abstinence in rats. Neuropsychopharmacology 22 : 626–641
Suzdak PD, Glowa JR, Crawley JN, Schwartz RD, Skolnick P, Paul SM . (1986): A selective imidazobenzodiazepine antagonist of ethanol in the rat. Science 234 : 1243–1247
Swerdlow NR, Koob GF . (1987): Dopamine, schizophrenia, mania, and depression: Toward a unified hypothesis of cortico-striato-pallido-thalamic function. Behav Brain Sci 10 : 197–245
Tanda G, Munzar P, Goldberg SR . (2000): Self-administration behavior is maintained by the psychoactive ingredient of marijuana in squirrel monkeys. Nature Neurosci 3 : 1073–1074
Tanda G, Pontieri FE, Di Chiara G . (1997): Cannabinoid and heroin activation of mesolimbic dopamine transmission by a common mu1 opioid receptor mechanism. Science 276 : 2048–2050
Thiele TE, Marsh DJ, Ste. Marie L, Bernstein IL, Palmiter RD . (1998): Ethanol consumption and resistance are inversely related to neuropeptide Y levels. Nature 396 : 366–369
Toates F . (1990): Motivational Systems. New York, Cambridge University Press
True WR, Heath AC, Scherrer JF, Waterman B, Goldberg J, Lin N, Eisen SA, Lyons MJ, Tsuang MT . (1997): Genetic and environmental contributions to smoking. Addiction 92 : 1277–1287
Uhl GR, Elmer GI, LaBuda MC, Pickens RW . (1995): Genetic influences in drug abuse. In Bloom FE, Kupfer DJ (eds), Psychopharmacology: The Fourth Generation of Progress. New York, Raven Press, pp 1793–1806
Vaccarino FJ, Pettit HO, Bloom FE, Koob GF . (1985): Effects of intracerebroventricular administration of methyl naloxonium chloride on heroin self-administration in the rat. Pharmacol Biochem Behav 23 : 495–498
Valentino RJ, Foote SL, Page ME . (1993): The locus coeruleus as a site for integrating corticotropin-releasing factor and noradrenergic mediation of stress responses. Ann N Y Acad Sci 697 : 173–188
Van Bockstaele EJ, Colago EE, Valentino RJ . (1998): Amygdaloid corticotropin-releasing factor targets locus coeruleus dendrites: Substrate for the co-ordination of emotional and cognitive limbs of the stress response. J Neuroendocrinol 10 : 743–757
Vezzani A, Sperk G, Colmers WF . (1999): Neuropeptide Y: Emerging evidence for a functional role in seizure modulation. Trends Neurosci 22 : 25–30
Volkow ND, Fowler JS . (2000): Addiction, a disease of compulsion and drive: Involvement of the orbitofrontal cortex. Cerebral Cortex 10 : 318–325
Volkow ND, Wang GJ, Fowler JS, Hitzemann R, Angrist B, Gatley SJ, Logan J, Ding YS, Pappas N . (1999): Association of methylphenidate-induced craving with changes in right striato-orbitofrontal metabolism in cocaine abusers: Implications in addiction. Am J Psychiatry 156 : 19–26
Wahlestedt C, Karoum F, Jaskiw G, Wyatt RJ, Larhammar D, Ekman R, Reis DJ . (1991): Cocaine-induced reduction of brain neuropeptide Y synthesis dependent on medial prefrontal cortex. Proc Natl Acad Sci USA 88 : 2078–2082
Waltman C, McCaul ME, Wand GS . (1994): Adrenocorticotropin responses following administration of ethanol and ovine corticotropin-releasing hormone in the sons of alcoholics and control subjects. Alcohol Clin Exp Res 18 : 826–830
Watkins SS, Stinus L, Koob GF, Markou A . (2000): Reward and somatic changes during precipitated nicotine withdrawal in rats: Centrally and peripherally mediated effects. J Pharmacol Exp Ther 292 : 1053–1064
Weddington WW Jr, Brown BS, Haertzen CA, Hess JM, Mahaffey JR, Kolar AF, Jaffe JH . (1991): Comparison of amantadine and desipramine combined with psychotherapy for treatment of cocaine dependence. Am J Drug Alcohol Abuse 17 : 137–152
Weeks JR, Collins RJ . (1976): Changes in morphine self-administration in rats induced by prostaglandin E1 and naloxone. Prostaglandins 12 : 11–19
Weiss F, Hurd YL, Ungerstedt U, Markou A, Plotsky PM, Koob GF . (1992a): Neurochemical correlates of cocaine and ethanol self-administration. Ann N Y Acad Sci 654 : 220–241
Weiss F, Markou A, Lorang MT, Koob GF . (1992b): Basal extracellular dopamine levels in the nucleus accumbens are decreased during cocaine withdrawal after unlimited-access self-administration. Brain Research 593 : 314–318
Weiss F, Parsons LH, Schulteis G, Hyytia P, Lorang MT, Bloom FE, Koob GF . (1996): Ethanol self-administration restores withdrawal-associated deficiencies in accumbal dopamine and 5-hydroxytryptamine release in dependent rats. J Neurosci 16 : 3474–3485
Weissenborn R, Deroche V, Koob GF, Weiss F . (1996): Effects of dopamine agonists and antagonists on cocaine-induced operant responding for a cocaine associated stimulus. Psychopharmacology 126 : 311–322
White FJ . (1996): Synaptic regulation of mesocorticolimbic dopamine neurons. Annu Rev Neurosci 19 : 405–436
Whitelaw RB, Markou A, Robbins TW, Everitt BJ . (1996): Excitotoxic lesions of the basolateral amygdala impair the acquisition of cocaine-seeking behaviour under a second-order schedule of reinforcement. Psychopharmacology 127 : 213–224
Wikler A . (1973): Dynamics of drug dependence: Implications of a conditioning theory for research and treatment. Arch Gen Psychiatry 28 : 611–616
Woldbye DP, Klemp K, Madsen TM . (1998): Neuropeptide Y attenuates naloxone-precipitated morphine withdrawal via Y5-like receptors. J Pharmacol Exp Ther 284 : 633–636
Woolverton WL, Virus RM . (1989): The effects of a D1 and D2 dopamine antagonist on behavior maintained by cocaine or food. Pharmacol Biochem Behav 32 : 691–697
World Health Organization. (1992): International Statistical Classification of Diseases and Related Problems, 10th ed. Geneva, World Health Organization
Yokel RA, Wise RA . (1975): Increased lever pressing for amphetamine after pimozide in rats: Implications for a dopamine theory of reward. Science 187 : 547–549
Download references
Acknowledgements
This is publication number 13306-NP from The Scripps Research Institute. GFK was supported by National Institutes of Health grants AA06420 and AA08459 from the National Institute on Alcohol Abuse and Alcoholism, DA04043 and DA04398 from the National Institute on Drug Abuse, and DK26741 from the National Institute of Diabetes and Digestive and Kidney Diseases. MLM was supported by Institut National de la Sante et de la Recherche Medicale, Universite de Bordeaux II, and Institut Universitaire de France. The authors would like to thank Drs. Barbara Mason, Bernard Carroll, Floyd Bloom, Markus Heilig, and Ms. Carrie Armel for their extremely helpful comments on the manuscript. The authors also would like to thank Mike Arends, Pat Brennan, and Isabelle Batby for their valuable assistance with manuscript preparation, and Janet Hightower for her tremendous help with the production of the illustrations.
Author information
Authors and affiliations.
Department of Neuropharmacology, The Scripps Research Institute, La Jolla, CA, USA
George F Koob Ph.D
Psychobiologie des Comportements Adaptatifs, INSERM, Unite 259, Universite de Bordeaux II, Bordeaux, France
Michel Le Moal MD, Ph.D
You can also search for this author in PubMed Google Scholar
Rights and permissions
Reprints and permissions
About this article
Cite this article.
Koob, G., Le Moal, M. Drug Addiction, Dysregulation of Reward, and Allostasis. Neuropsychopharmacol 24 , 97–129 (2001). https://doi.org/10.1016/S0893-133X(00)00195-0
Download citation
Received : 05 July 2000
Revised : 18 August 2000
Accepted : 25 August 2000
Issue Date : 01 February 2001
DOI : https://doi.org/10.1016/S0893-133X(00)00195-0
Share this article
Anyone you share the following link with will be able to read this content:
Sorry, a shareable link is not currently available for this article.
Provided by the Springer Nature SharedIt content-sharing initiative
- Drug addiction
- Reward dysregulation
This article is cited by
High-intensity sweet taste as a predictor of subjective alcohol responses to the ascending limb of an intravenous alcohol prime: an fmri study.
- Jonathan Alessi
- Mario Dzemidzic
- David A. Kareken
Neuropsychopharmacology (2024)
Cannabis Use and Emotions in Romantic Relationships
- Aviya Ashwal-Malka
- Daniel Feingold
Current Addiction Reports (2024)
Managing Pain in Patients With Comorbid Alcohol Use Disorder and Chronic Pain
- Nasim Maleki
- Benjamin L. Thompson
Prazosin Enhances the Effectiveness of Mirtazapine on Anxiety- and Depression-Like Behaviors in Rats during Cocaine Withdrawal
- Susana Barbosa-Méndez
- Alberto Salazar-Juárez
International Journal of Mental Health and Addiction (2024)
Disentangling the role of NAc D1 and D2 cells in hedonic eating
- Mathilde C. C. Guillaumin
- Paulius Viskaitis
- Daria Peleg-Raibstein
Molecular Psychiatry (2023)
Quick links
- Explore articles by subject
- Guide to authors
- Editorial policies


An official website of the United States government
The .gov means it’s official. Federal government websites often end in .gov or .mil. Before sharing sensitive information, make sure you’re on a federal government site.
The site is secure. The https:// ensures that you are connecting to the official website and that any information you provide is encrypted and transmitted securely.
- Publications
- Account settings
Preview improvements coming to the PMC website in October 2024. Learn More or Try it out now .
- Advanced Search
- Journal List
- Int J Environ Res Public Health

Risky Substance Use Environments and Addiction: A New Frontier for Environmental Justice Research
Jeremy mennis.
1 Department of Geography and Urban Studies, Temple University, Philadelphia, PA 19122, USA; ude.elpmet@relhatsj
Gerald J. Stahler
Michael j. mason.
2 Commonwealth Institute for Child & Family Studies, Department of Psychiatry, Virginia Commonwealth University, Richmond, VA 23298, USA; [email protected]
Substance use disorders are widely recognized as one of the most pressing global public health problems, and recent research indicates that environmental factors, including access and exposure to substances of abuse, neighborhood disadvantage and disorder, and environmental barriers to treatment, influence substance use behaviors. Racial and socioeconomic inequities in the factors that create risky substance use environments may engender disparities in rates of substance use disorders and treatment outcomes. Environmental justice researchers, with substantial experience in addressing racial and ethnic inequities in environmental risk from technological and other hazards, should consider similar inequities in risky substance use environments as an environmental justice issue. Research should aim at illustrating where, why, and how such inequities in risky substance use environments occur, the implications of such inequities for disparities in substance use disorders and treatment outcomes, and the implications for tobacco, alcohol, and drug policies and prevention and treatment programs.
1. Introduction
One of the fundamental aims of environmental justice research is to investigate if, how, and why environmental risks are distributed inequitably with regards to race and socioeconomic status. Academic research in environmental justice has expanded from its early focus on environmental risks due to exposure to technological hazards to a variety of other outcomes [ 1 , 2 ], such as vulnerability to natural hazards [ 3 , 4 ], accessibility to environmental amenities [ 5 , 6 ], and consequential health conditions, such as asthma [ 7 , 8 ]. The environmental justice framework has also been applied to address racial and socioeconomic disparities in access to health resources such as recreational opportunities and healthy food that can promote healthy behaviors, such as engaging in regular physical activity and healthy eating [ 9 , 10 , 11 ]. In the present paper, we build on this research to address the environmental risks associated with another important health behavior that, as yet, has been given only sparse attention by environmental justice researchers—substance use and addiction.
Substances such as alcohol, nicotine, and illicit and prescription drugs affect the pleasure and reward circuitry of the brain, and long-term, heavy use can permanently change the structure and function of the brain [ 12 ]. Addiction to substances is a chronic brain disease that affects judgment and behavior by altering cognitive functions such as learning, memory formation, and impulse control [ 13 ]. Addiction is characterized by intense craving for substances and a compulsion to acquire and use substances, even when there are substantial negative consequences to doing so [ 14 ]. Addiction often co-occurs with, and may contribute to, many other adverse health conditions, such as heart disease and cancer, as well as such mental health problems as depression and other mood disorders [ 15 ].
Substance abuse and addiction represent an enormous threat to human health, with approximately 246 million people between the ages of 15 and 64 years old worldwide estimated to have used an illicit drug in 2013, including the use of cannabis, opioids/opiates, cocaine, amphetamine-type stimulants, and other drugs. Of these 246 million users, more than one out of ten can be considered problem drug users, and drug use can be attributed to approximately 187,000 deaths in 2013 alone [ 16 ]. In the U.S., approximately 10.2% of the population age 12 years and over had used an illicit drug in the past month, which represents an increase over the past decade [ 17 ]. Notably, more Americans suffer from addiction to tobacco, alcohol, or other drugs than suffer from diabetes, cancer, or heart conditions [ 15 ]. While evidence-based treatment for addictive disorders has been shown to be effective, with outcomes comparable to other chronic diseases such as diabetes and asthma [ 18 ], few of those suffering from substance use disorders receive it. In the U.S., for example, only approximately 11% of those who need treatment receive it at a specialty facility [ 19 ], and the rate is likely much lower in developing countries. Of those that do complete treatment, there is a substantial likelihood of relapse back into substance use because of the chronic nature of this disease.
While substance use disorders are known to affect people of all regions, races, and socioeconomic statuses, certain segments of the population are more likely to use particular substances. According to recent 2013 statistics available for the U.S., rates of past-month illicit drug use by those aged 12 years and older was highest among African Americans, followed by whites, Hispanics, and Asians, respectively—a pattern that has remained constant over the past decade [ 19 ]. Substance use is also more likely among those with lower educational attainment, among those who are unemployed, and those residing in urbanized areas [ 19 ]. Overall alcohol use, on the other hand, was higher for whites, those with full-time employment, those with higher educational attainment, and those living in urbanized regions, as compared to other groups [ 19 ]. Rates of tobacco use were slightly higher for whites as compared to African Americans, and lower for Hispanics and Asians [ 19 ]. Tobacco use was also higher for those with lower educational attainment and those who were unemployed, as well as those living in rural areas [ 19 ]. Rates of substance use disorder treatment completion also show substantial disparities by race and socioeconomic status in the U.S., with whites, the employed, and those with higher educational attainment generally having a higher likelihood of treatment completion as compared to other groups [ 20 ].
Initiation into substance use may stem from a variety of factors, including genetic, biological, cognitive, affective, family, and peer characteristics [ 21 ]. Of particular interest for environmental justice researchers, however, are the environmental factors related to substance use. Contextual characteristics of the neighborhood environment within which individuals reside have long been theorized to affect human development [ 22 , 23 ] and health [ 24 ], and this framework has been extended to address substance use behaviors specifically, including the initiation, continued use, and abuse of alcohol, tobacco, and illicit drugs [ 25 , 26 ]. Recent research on place and behavior that utilizes geographic methods and technologies has provided evidence to support these theories, and has shown that certain environments embody greater risk regarding substance use [ 27 , 28 ]. The environmental effects on substance use are particularly troubling for adolescents, given the vulnerability of youth to contextual environmental influence on substance use initiation, and the multiple negative health consequences of early substance use initiation throughout adulthood [ 29 ]. In addition, early drug use in adolescence is associated with an increased likelihood for later substance dependence [ 30 ].
There is a straightforward connection between more “conventional” environmental justice research on disparities in exposure to environmental hazards and research on disparities in exposure to environmental characteristics that may encourage substance use and addictive behaviors. While substantial research has shown that the spatial distribution of environmental risks regarding air pollution and other industrial environmental hazards are inequitably distributed with regards to race and class [ 31 ], many of these same characteristics of the built and socioeconomic environment which are associated with technologically generated environmental risks are also associated with risky substance use behaviors, including factors associated with land use and neighborhood level indicators of socioeconomic status [ 25 ]. Thus, it follows that the distribution of risky environments with regards to substance use is also inequitably distributed according to race and socioeconomic status. Such inequities may produce, in concert with other factors, racial and socioeconomic disparities in substance use behaviors, treatment outcomes, and associated health outcomes.
Below, we detail the contextual characteristics of the built and socioeconomic environments that may encourage or facilitate substance use, or reduce the effectiveness of substance use disorder treatment, environments that we refer to here as ‘risky substance use environments’. The connections between characteristics of risky substance use environments with race and socioeconomic status are discussed, as are the substantive, methodological, and policy questions that may direct environmental justice research in this area. We focus our discussion primarily on evidence drawn from studies in the U.S., though we note that the fundamental principles we describe are widely applicable to other countries and regions.
2. Characteristics of Risky Substance Use Environments
2.1. access to substances.
Perhaps the most basic manner in which a risky environment can be considered to affect substance use behaviors is by facilitating access to substances of abuse. Ready access to substances lowers the barriers to acquiring, using, and abusing substances, thus facilitating substance use initiation and potential abuse. A substantial body of research indicates that the presence of, proximity to, and density of alcohol outlets ( i.e. , stores and/or bars selling liquor, wine, and/or beer) is associated with increased alcohol-related mortality [ 32 , 33 ] and alcohol consumption among teenagers [ 34 , 35 ], college students [ 36 ], and adults [ 37 ]. Proximity to alcohol sales has also been found to be associated to other negative outcomes, including violence in the home and in the community [ 38 , 39 ].
Similar results have been found for tobacco, where exposure to tobacco outlets, including convenience stores, gas stations, pharmacies, and other stores that typically sell tobacco products, is associated with increased rates of smoking initiation among youth [ 40 , 41 ] and young adults [ 42 ]. The mechanism for tobacco use initiation stems not only from access to tobacco but also from exposure to tobacco advertisements, promotions, and marketing, which are often concentrated at the point-of-sale, particularly in countries where there are restrictions on tobacco advertising on television and other media, such as in the U.S. [ 43 ]. Such advertising can glamorize smoking and increase the intention to smoke among youth [ 44 , 45 , 46 ], a strong predictor of future smoking behavior [ 47 ]. Similar effects have been found for alcohol advertising on consumption [ 48 ].
For those suffering from substance use disorders, environments with high accessibility to tobacco, alcohol, and illicit drugs can not only facilitate the acquisition of substances but can also contain environmental cues that trigger substance craving [ 49 ]. Such environmental cues can have substantially negative consequences for those in treatment for substance use disorders as well as for those in long term recovery who are attempting to maintain abstinence from substance use. Research suggests that simply the exposure to the visual cues involved in seeing alcohol or tobacco outlets or other places associated with prior substance acquisition and use can activate craving for those substances among those in recovery [ 49 ]. Evidence indicates, for example, that the likelihood of smoking cessation among smokers is lower among those living near to tobacco outlets as compared to those living farther away [ 50 , 51 ].
Research suggests that racial and socioeconomic inequities persist in the residential proximity to, and density of, stores selling tobacco and alcohol. Many U.S.-based studies at the state, metropolitan area, and county levels have found that stores selling tobacco are disproportionately located in neighborhoods with higher percentages of minorities, particularly African Americans and Hispanics [ 52 , 53 , 54 ], and lower income [ 55 , 56 , 57 ]. Similar patterns of racial and economic inequity have been found for alcohol outlets [ 58 , 59 , 60 ]. Evidence indicates that racial and economic inequities also persist not only for stores selling alcohol and tobacco but also for alcohol and tobacco advertising [ 61 , 62 , 63 ]. Though stores or dispensaries legally selling marijuana, for medical or recreational purposes, are still limited to certain states in the U.S., preliminary evidence indicates a higher prevalence of legal marijuana outlets in minority and impoverished neighborhoods [ 64 , 65 ].
Because data on the locations of illicit drug selling are more difficult to collect as compared to alcohol and tobacco sales (which can be acquired from databases of business listings or alcohol and tobacco licensing), research on exposure to illicit drug markets is more limited. However, research suggests that proximity to illicit drug sales and consumption is associated with higher rates of illicit drug use [ 66 ] and relapse among those in substance use disorder treatment [ 67 ].
2.2. Neighborhood Concentrated Disadvantage and Disorder
Neighborhoods with concentrated disadvantage, or economic deprivation, are generally characterized by low income, low educational attainment, and high unemployment. Concentrated disadvantage embodies the idea that such neighborhoods are not only impoverished economically but also removed from mainstream economic activity such that there are few opportunities for economic advancement, and thus residents of neighborhoods characterized by concentrated disadvantage are caught in a vicious cycle of poverty from which it is difficult to escape [ 68 ]. Notably, neighborhood disadvantage and other community level characteristics have important implications for health beyond that of the socioeconomic status of individuals and families. The additive effect of neighborhood level disadvantage on health, as a burden levied on top of individual-level poverty, has been referred to as “deprivation amplification”, i.e. , the negative effect of individual-level poverty on health is amplified by also residing in an impoverished neighborhood [ 69 ].
Neighborhood concentrated disadvantage is also often associated with neighborhood disorder. Disordered environments are typified by indicators of a lack of social control over the environment, including graffiti, trash, noise, vandalism, and dilapidated or abandoned infrastructure, as well as the presence of violence and crime in the community. Residing in neighborhoods characterized by disadvantage and disorder can produce chronic stress due to the trauma of continuous economic struggle and exposure to this disorder, violence, and crime in the community [ 70 , 71 , 72 ]. Substance use is often employed as a coping mechanism to deal with such chronic stress [ 73 ], and a number of studies have found that exposure to, and perceptions of, neighborhood disadvantage and disorder are associated with higher levels of stress and substance use [ 74 , 75 , 76 ], particularly among adolescents [ 27 , 71 , 77 ].
In the U.S. and many other nations, segregation by race and/or ethnicity is an important component of neighborhood concentrated disadvantage, where a legacy of racism and restrictive land use and development policies have encouraged the creation of highly disadvantaged neighborhoods composed primarily of certain racial and ethnic minorities with little access to the economic and other resources available to other population groups [ 78 ]. The consequence of such segregation is that minorities and the poor reside disproportionally in disadvantaged and disordered neighborhoods as compared to non-minorities and the non-poor, and thus may be more prone to environmentally-based chronic stress mechanisms of substance abuse [ 28 , 79 , 80 ].
Neighborhood social cohesion, or the strength of social relationships among community members, may also influence substance use. Research indicates that high social cohesion among neighbors can mitigate the stress associated with disadvantaged and disordered neighborhoods, and thus lower the deleterious effects of neighborhood disadvantage and disorder on substance use via chronic stress [ 81 ]. High social cohesion can facilitate the ability of neighbors to cooperate and work together to address community problems, such as the presence of crime or graffiti in a community, as well as deviant behavior, such as substance use [ 82 ]. The social relationships that high social cohesion affords can also provide resources and pro-social influences regarding substance use cessation or abstinence, though the opposite effect may be observed in social networks where substance use is common or socially accepted [ 83 ]. Unfortunately, however, social cohesion tends to be lower in disadvantaged neighborhoods, due in part to the prevalence of residential instability in such neighborhoods where housing tenure is relatively short, people are inclined to move residences often, and there is a low rate of homeownership as compared to rentals [ 84 ].
Disadvantaged neighborhoods may also be characterized by a lack of access to pro-social and environmental resources, such as libraries, recreation centers, parks and other greenspaces, and medical and social services [ 6 ]. Recreation centers, libraries, and after school programs can offer alternative leisure activities in structured social settings that act to discourage substance use, particularly for youth. Research has shown an association between access to places of worship and lower rates of substance use, with evidence suggesting that the social supports available to individuals with access to religious institutions play a key role [ 85 ]. Exposure to greenspace has been shown to alleviate psychological stress and have a calming effect [ 86 , 87 ], thus countering, to a limited extent, the stressful conditions of disadvantaged neighborhoods [ 88 ] and consequent substance use as a coping behavior. The effect of such resources, however, may be moderated by other community level characteristics. For instance, the presence of crime in a park, drug markets on a residential street, or a recreation center where violence among youth is common, may engender greater, not lesser, stress and/or deviant behavior, such as substance use.
2.3. Environmental Barriers to Substance Use Disorder Treatment
Environmental characteristics can also play an important role in substance use disorder treatment completion and abstinence from substance use. Travel to treatment is one of the most fundamental environmental influences on treatment, as the majority of substance use disorder treatments occur via outpatient versus residential treatment programs [ 20 ], such that clients must travel from their homes to the treatment location. For the poor, who are less likely to own a car, access to public transportation from both the home and the outpatient treatment program may be an important contributor to attending treatment. Research indicates that distance and travel time from the home to the treatment program can affect treatment attendance and completion [ 89 , 90 ], which is itself a key indicator of post-treatment success regarding future abstinence from substance use, employment, less involvement in the criminal justice system, and other positive outcomes [ 91 ].
In addition to the physical distance and time required to attend outpatient treatment, another environmental characteristic concerns the degree to which attending treatment requires traveling from one’s own neighborhood to a neighborhood with a different cultural or socioeconomic orientation. Research indicates that culturally sensitive treatment settings engender treatment success, and that clients feel more comfortable and are more responsive to treatment settings in which they are not socially, culturally, or linguistically isolated [ 92 , 93 ]. These same issues extend to geographic differences between home and outpatient treatment locations, where clients traveling to neighborhoods socially and culturally different than their own may be less likely to attend or complete treatment [ 89 , 94 ]. In addition, for those who receive brief inpatient treatment for their substance use disorders and then are discharged to outpatient treatment in their community, such environmental factors as living in greater proximity to alcohol outlets and in areas with high vacant housing rates represent particular environmental risk factors that can act to reduce the likelihood of treatment continuity [ 95 , 96 ].
These environmental barriers to treatment may contribute to the observed racial inequities in overall access to treatment and treatment attendance. Several studies have shown that minorities in the U.S. tend to have poorer access to substance use disorder treatment, lower utilization rates, and are less satisfied with treatment as compared to whites [ 97 , 98 , 99 ]. Consequently, there are marked racial and ethnic disparities in measures of treatment completion, where African Americans and Hispanics in the U.S. are less likely to complete treatment as compared to whites [ 100 , 101 ]. Though admittedly, completion represents only one type of treatment outcome, as discussed above, it is an important one that is highly predictive of longer term positive outcomes.
2.4. Moderating Factors among Environment, Race and Poverty, and Substance Use Outcomes
While there is ample evidence that greater access and exposure to the sale and advertising of substances, residing in neighborhoods characterized by concentrated disadvantage and disorder, and various barriers to accessing treatment for substance use disorders all influence substance use behaviors and treatment outcomes, it should be noted that other factors may moderate these relationships. For instance, evidence indicates that contextual environmental conditions differ in their effect based on such individual characteristics as gender and age [ 77 , 102 ] and level of motivation, and such social characteristics as parental supervision and peer behaviors and attitudes [ 103 ].
Of particular interest here, however, are factors that may moderate the relationships among risky substance use environments, substance use and treatment outcomes, and race and socioeconomic status. Research suggests that the association between risky substance use environments and substance use is stronger in poorer neighborhoods for tobacco and alcohol [ 50 , 104 , 105 ]. Certain substances of abuse are also more popular among certain racial and ethnic groups; for instance, among adults in the U.S. seeking treatment for substance use disorders, alcohol use disorder is more common among whites than other racial groups, while cannabis use disorder is more common among African Americans [ 101 ]. The intersection of race and choice of substance of abuse has implications for treatment, where environmental conditions may play a differential role depending on the substance of abuse. For instance, race itself has been shown to moderate the effect of treatment modality on treatment completion, where whites have been shown to accrue greater benefits from residential, as compared to outpatient treatment [ 20 ], which may be due to the predominance of risky substance use environments in the residential neighborhoods of many minorities.
3. Research Questions for the Environmental Justice of Substance Use
3.1. where and why does inequity in risky substance use environments occur.
As with other domains of environmental justice research, racial and socioeconomic inequities in the distribution of risky substance use environments engender similar types of research questions. These questions encompass substantive issues, methodological issues, and policy implications. Regarding substantive issues, perhaps the most fundamental question is where, and for what types of environmental risk, is there racial and/or socioeconomic inequity in risky substance use environments? Research in environmental justice has shown that types of environmental risk due to technological hazards, and their relationships with certain population subgroups, vary from place to place [ 31 ]. Certainly, we would expect similar variations in risky substance use environments. Indeed, while certain national level studies in the U.S. have shown broad associations between indicators of risky substance use environments and race and class [ 54 ], other research has shown that such associations differ in form and strength across different regions and metropolitan areas [ 52 , 53 ], and even states in regard to treatment outcomes [ 100 ]. Understanding how, and why, the particular regulatory and socioeconomic development legacies of particular regions have (or have not) produced patterns of risky substance use environment inequity is important.
One question that has been central to environmental justice research on industrial hazards addresses the distinction between race and class as mechanisms of environmental inequity [ 106 ], with some researchers suggesting that when left to compete statistically for explanation, racial inequities are artifacts of the effect of socioeconomic status on environmental risk [ 107 ]. While later research argued that race and class cannot be neatly divided, and that explanations of environmental inequity should incorporate the historical narratives of how race and class intertwine in legacies of urban land development [ 108 ], the relationship between race and class is also of interest to studies of risky substance use environments. For instance, socioeconomic status may moderate the relationship between race and indicators of risky substance use environments or between indicators of risky substance use environments and substance use outcomes.
Finally, there is the question of why racial and socioeconomic inequities in risky substance use environments occur. We might consider several theoretical explanations drawn from the environmental justice literature [ 108 , 109 , 110 ]. First, there might be discrimination on the part of businesses that sell alcohol or tobacco, where such sales or advertising are targeted towards minorities and/or the poor, or on the part of agencies that regulate such sales and advertising. Second, businesses selling alcohol and tobacco, or attendant advertising, may choose to locate in neighborhoods that lack the political power to fight the presence of stores selling liquor and tobacco (though we note that such stores, which may include supermarkets, pharmacies, and convenience stores, may be perceived as innocuous and also provide benefits to the community). Third, economic processes, such as those that drive commercial development and residential real estate prices, may play a role in creating neighborhoods where minority residents and poverty coincide with alcohol and tobacco sales and advertising. Fourth, structural and historical racism may play a role in creating disadvantaged and disordered neighborhoods that put minorities and the poor at risk for chronic stress and subsequent risk for substance use.
3.2. How Should Environmental Justice Analyses of Risky Substance Use Environments Be Conducted?
Methodological questions analogous to those that have been addressed in more conventional environmental justice analyses [ 111 ] also arise when studying risky substance use environments, including how to represent and measure exposure to risk, and what health outcomes can be connected to such exposure. Specifically, research should inquire how environmental risk for substance use should be quantified. Measures of access or exposure to sales and advertising of substances generally utilize metrics of distance to, or density of, stores selling tobacco or alcohol [ 46 ], whereas measures of neighborhood concentrated disadvantage and disorder generally rely on spatially aggregated census or other survey data [ 28 , 77 , 96 ]. Sometimes, subject perceptions of neighborhood disorder or social cohesion are employed [ 71 ]. However, no standard approach for quantifying the level of environmental risk for substance use has yet emerged.
In addition, questions remain as to how indicators of risky substance use environments should be linked to exposure for individuals. The majority of environmental justice research has employed aggregated population data and, consequently, developed risk measures estimated according to spatial units of population enumeration [ 109 ]. While these same strategies can be employed with exposure to risky substance use environments, most research on substance use outcomes uses data at the individual level. Challenges persist in linking exposure to risky substance use environments to individual substance use and treatment outcomes. Given that individuals have different substance use histories and experiences, identifying unique, individualized risky substance use environments may be of greatest utility for treatment and long term recovery purposes.
A common approach to estimating an environmental exposure for an individual is to identify the characteristics of the neighborhood within which that individual resides, where the neighborhood is often defined by political jurisdiction (e.g., U.S. municipalities), census enumeration (e.g., U.S. Census Bureau tracts), or postal code (e.g., U.S. Postal Service zip codes) [ 80 ]. However, such approaches have been criticized for failing to capture the actual environmental exposures experienced by individuals [ 112 ]. In addition, behavioral health researchers have recognized that measurements of environmental exposure should incorporate not only the home neighborhood but also the locations of friends and family, work, and leisure which they frequent throughout their daily lives [ 27 , 113 ]. This constellation of places where one resides and regularly travels to engage in daily activities is referred to as an individual’s activity space [ 113 , 114 ].
Recent advances in integrating geospatial technologies, such as global positioning systems (GPS) and geographic information systems (GIS), with ecological momentary assessment (EMA), an approach for collecting survey data on individuals’ moods, behaviors, and social interactions in real-time via mobile phone, show promise in this regard. Studies have employed geospatially-enabled EMA to capture the exposure to risky substance use environments, not only at an individual’s residence but also throughout their activity space [ 103 , 115 ]. These geographic and longitudinal data can be used to develop statistical models representing the causal mechanisms that link exposure to risky substance use environments to consequent substance use behaviors [ 116 ], as well as incorporate the moderating or mediating role of other mechanisms, such as peer and family relationships, that can shed light on how risky substance use environments produce substance use outcomes.
3.3. What Are the Policy Implications for the Environmental Justice of Risky Substance Use Environments?
The environmental justice community has been successful in bringing environmental justice issues to bear in federal and state environmental regulations. Likewise, there are substantial regulatory and policy implications of the environmental justice of risky substance use environments. If, indeed, racial and socioeconomic inequity in the access and exposure to tobacco, alcohol, and illicit drugs contributes to health disparities in substance use disorders and treatment outcomes, then regulatory agencies might consider racial and socioeconomic factors in licensing and siting alcohol and tobacco stores and advertising. While sales and advertising of tobacco and alcohol are already substantially regulated, such regulations are typically not considered within an environmental justice frame [ 117 ]. Environmental justice researchers should explore how regulations on land development that affects the access and exposure to tobacco and alcohol can be adapted to address racial and socioeconomic inequities, as well as vulnerable populations, such as children and adolescents.
Addressing problems of concentrated disadvantage and disorder clearly encompasses policy implications beyond substance use and addiction. However, environmental justice researchers should investigate the role of such environmental factors in substance use policies. Of particular relevance here are policies related to the criminalization of drug use and the consequent disproportionate incarceration of minorities in the U.S. for drug crimes. A recent 2014 National Research Council report [ 118 ] details some of these inequalities. With about 2.2 million adults in county jails and state and federal prisons, the U.S. has both the largest incarcerated population and the highest rate of incarceration in the world. These prisoners tend to come from the most disadvantaged areas of the nation, and minority groups are greatly overrepresented, comprising about 60% of the incarcerated population [ 119 ]. Moreover, the rate of incarceration among African Americans is six times that of whites, and for Hispanics three times that of whites. In fact, among recent cohorts of African American male high school dropouts, it has been estimated that 70% have been incarcerated at some point in their young lives [ 118 ].
Federal and state drug policies, and harsher sentencing laws for drug crimes, are the primary contributors to this mass incarceration that has so disproportionally impacted minorities and the disadvantaged. Recently, there has been a greater recognition and public discourse about this problem, with various legislative and policy efforts aimed at reducing these extremely high levels of incarceration [ 120 ]. However, as ex-offenders are released back into their communities of origin, the risk of recidivism and re-offending may be influenced by many of the same neighborhood environmental risk factors discussed above that relate to both continued substance use, a major risk factor for recidivism, as well as criminal behavior [ 121 , 122 , 123 ]. Environmental justice researchers are well-positioned to investigate the dynamics among neighborhood disadvantage and disorder, inter-related substance use and criminal behaviors, and the consequent disproportionate incarceration of minorities and the poor.
Prevention programs that seek to suppress substance use initiation, and treatment programs that facilitate substance use cessation and long term recovery, both of which may reduce the likelihood of incarceration and recidivism, should explicitly consider environmental characteristics as an important component. For example, treatment programs often teach clients about behavioral cues that are associated with substance use (e.g., smoking after a meal, drinking after work) and the linkages between these conditioned cues and relapse [ 124 , 125 ]. Embedding cue awareness with place-based anchors, such that clients become more fully aware of their own personal and particular set of places that may act as cues that trigger craving, could increase the effectiveness of treatment programs by making clients more aware of their own individual spatial behaviors that can lead to relapse [ 126 , 127 ]. Often these programs seek to identify clients’ social contacts who are either involved with substance use (and therefore represent a risky association), or not involved (and therefore represent a protective association) to further the client’s long term recovery. These networks of associates can be understood within a place-based contextual framework, grounding people, places, and substance use within a geography of substance use risk or protection that is unique to each individual. Such individual-level information can be utilized to develop personalized substance use prevention and treatment programs [ 128 , 129 ]. Questions remain, however, as to how to create substance use prevention and treatment programs informed by these contextual mechanisms in a manner that is cost-effective and easily adaptable for use in community-based programs.
4. Conclusions
Substance use disorders are widely recognized as one of the most pressing public health problems, and recent research indicates that environmental factors influence substance use behaviors. Evidence suggests that racial and socioeconomic inequities in the environmental factors that may engender substance abuse, including access and exposure to substances of abuse, neighborhood disadvantage and disorder, and environmental barriers to treatment, contribute to observed health disparities in rates of substance use disorders and treatment outcomes. Environmental justice researchers, with substantial experience in addressing racial and ethnic inequities in environmental risk due to technological and other hazards, should consider similar inequities in risky substance use environments as an environmental justice issue. Research should aim at illustrating where, why, and how such inequities in risky substance use environments occur, the implications of such inequities for disparities in substance use disorders and treatment outcomes, and the implications for tobacco, alcohol, and drug policies and prevention and treatment programs.
Acknowledgments
This research was supported by grant No. 1R01 DA031724-01A1 from the National Institute on Drug Abuse. The findings and conclusions are those of the authors and do not necessarily represent the views of the National Institute on Drug Abuse, or the National Institutes of Health.
Author Contributions
Jeremy Mennis conceptualized the manuscript. Jeremy Mennis, Gerald Stahler, and Michael Mason all contributed to writing and editing the manuscript.
Conflicts of Interest
The authors declare no conflict of interest.
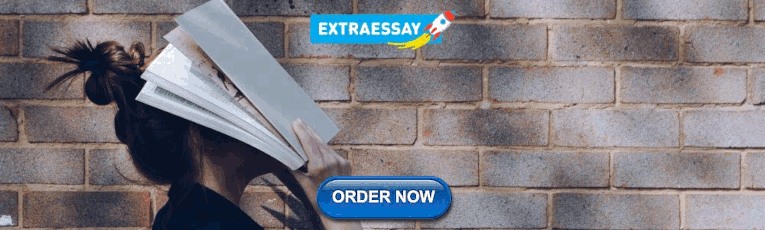
COMMENTS
The astonishing hypothesis: the scientific search for the soul. ... National Institute on Drug Abuse Intramural Research Program and National Institute on Alcohol Abuse and Alcoholism Division of ...
Abstract. Dopamine (DA) transmission is deeply affected by drugs of abuse, and alterations in DA function are involved in the various phases of drug addiction and potentially exploitable therapeutically. In particular, basic studies have documented a reduction in the electrophysiological activity of DA neurons in alcohol, opiate, cannabinoid ...
Research demonstrating that addiction is driven by changes in the brain has helped to reduce the negative attitudes associated with substance use disorders and provided support for integrating treatment for substance use disorders into mainstream health care. ... The glutamate homeostasis hypothesis of addiction. Nature Reviews Neuroscience ...
II. DRUG REWARD. Dopamine (DA) lies at the center of drug reward (85, 182).Every drug with addiction potential increases DA, either through direct or indirect effects on DA neurons in the ventral tegmental area (VTA) with the consequent release of DA in the nucleus accumbens (NAc) (FIGURE 1).Drugs of abuse increase DA through their initial action on different molecular targets and, depending ...
Addiction is one of the biggest health problems facing the world today. Each year, many millions of people die as a result of addiction to substances such as tobacco and alcohol 1, and currently ...
Here, we propose several clues to reconcile these unexpected results with the habit theory of addiction, and we highlight the need in experimental research to face the complexity of drug addicts ...
It is essential to develop theories and models that enable us to understand addiction's genesis and maintenance, providing a theoretical and empirical framework for designing more effective interventions. Numerous clinical and preclinical research studies have investigated the various brain and physiological mechanisms involved in addictive behavior. Some researchers have gone a step further ...
Addictive drugs are habit-forming. Addiction is a learned behavior; repeated exposure to addictive drugs can stamp in learning. Dopamine-depleted or dopamine-deleted animals have only unlearned reflexes; they lack learned seeking and learned avoidance. Burst-firing of dopamine neurons enables learning—long-term potentiation (LTP)—of search and avoidance responses.
A leading hypothesis guiding current molecular and cellular research into drug addiction conceptualizes key aspects of addiction as a form of memory in which common neuroplasticity mechanisms that mediate normal learning and memory processes are 'hijacked' by exposure to drugs of abuse to produce pathologic addiction-related memories.
The dopamine hypothesis of drug addiction and its potential therapeutic value. Dopamine (DA) transmission is deeply affected by drugs of abuse, and alterations in DA function are involved in the various phases of drug addiction and potentially exploitable therapeutically. In particular, basic studies have documented a reduction in the ...
"Common factors will explain the use of drugs in general, and specific factors will explain why young people use specific drugs and do so in a particular sequence," according to Nobel Prize-winning psychiatrist Eric Kandel, M.D., and his wife and collaborator, sociologist Denise Kandel, Ph.D., about their research on how tobacco use can serve as a "gateway" to cocaine addiction.
1. Introduction. The concept of "gateway hypothesis" has been studied since the 1970s (Kandel, 1975, Kandel and Faust, 1975) as the theory suggests that an adolescent's early experimentation with alcohol or tobacco or cannabis escalates to more addictive illicit drugs later in adulthood (Lynskey et al., 2003).Most commonly used illicit substances include heroin/opioids, cocaine and or ...
Dopamine (DA) transmission is deeply affected by drugs of abuse, and alterations in DA function are involved in the various phases of drug addiction and potentially exploitable therapeutically. In particular, basic studies have documented a reduction in the electrophysiological activity of DA neurons in alcohol, opiate, cannabinoid, and other drug-dependent rats. Further, DA release in the ...
Abstract. Dopamine (DA) transmission is deeply affected by drugs of abuse, and alterations in DA function are involved in the various phases of drug addiction and potentially exploitable therapeutically. In particular, basic studies have documented a reduction in the electrophysiological activity of DA neurons in alcohol, opiate, cannabinoid ...
Abstract and Figures. Dopamine (DA) transmission is deeply affected by drugs of abuse, and alterations in DA function are involved in the various phases of drug addiction and potentially ...
Neuropsychopharmacology 42, 985-986 (2017) Cite this article. The gateway drug hypothesis refers to the pattern of substance use during adolescence whereby legal substances, such as nicotine and ...
At the National Institute on Drug Abuse (NIDA), we believe that increased understanding of the basics of addiction will empower people to make informed choices in their own lives, adopt science-based policies and programs that reduce drug use and addiction in their communities, and support scientific research that improves the Nation's well ...
The numbers for substance use disorders are large, and we need to pay attention to them. Data from the 2018 National Survey on Drug Use and Health suggest that, over the preceding year, 20.3 million people age 12 or older had substance use disorders, and 14.8 million of these cases were attributed to alcohol.When considering other substances, the report estimated that 4.4 million individuals ...
The dopamine hypothesis of drug addiction: hypodopaminergic state. Int Rev Neurobiol. 2005:63:101-54. doi: 10.1016/S0074-7742 (05)63005-X.
Testing the Gateway Hypothesis. The gateway drug hypothesis refers to the pattern of substance use during adolescence whereby legal substances, such as nicotine and alcohol, precede the progressive use of illicit substances like cocaine and heroin. This concept of a gateway progression related to addiction vulnerability has had important ...
The importance of drug addiction research cannot be overstated. With millions of lives hanging in the balance, scientists are working tirelessly to unravel the mysteries of addiction and find new ways to combat its devastating effects. ... This self-medication hypothesis has gained significant traction in recent years, leading to a greater ...
The characterisation of the role of glia and the extracellular matrix (ECM) in drug-induced synaptic plasticity is an exciting emerging field of drug addiction research as it comes with promising ...
Treatment of Parkinson's disease is in large part based on the administration of levodopa. The use of this drug is limited by the development of psychiatric complications, including a disorder ...
June 14-15, 2024 Montreal, Canada. After three years of virtual meetings, the 2024 NIDA International Forum returned to an in-person format. The meeting, which was held on June 14 and 15 in Montreal in conjunction with the College on Problems of Drug Dependence (CPDD) Annual Scientific Meeting, was cochaired by NIDA International Program's leadership, Dr. Tom Clarke and Dr. Lindsey Friend.
Drug addiction is not a static phenomenon, and as with other biobehavioral dysregulation, such as compulsive gambling and binge eating, there are different components that constitute a cycle or ...
This research was supported by grant No. 1R01 DA031724-01A1 from the National Institute on Drug Abuse. The findings and conclusions are those of the authors and do not necessarily represent the views of the National Institute on Drug Abuse, or the National Institutes of Health.