- Skip to navigation
- Skip to content
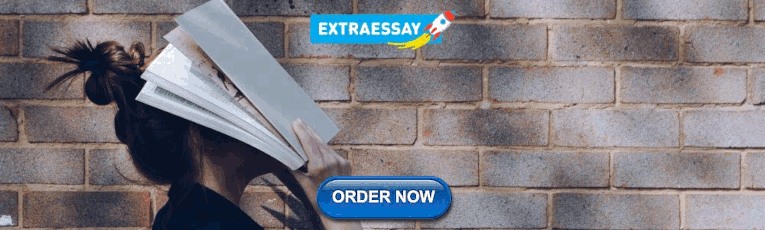
Natural disasters and climate risk
- Parliamentary Library Briefing Book
Tessa Satherley, Economic Policy and Dr Daniel May, Science, Technology, Environment and Resources
Key issue Recent natural disasters have been traumatic and costly for Australian communities, and recovery will take many years. Under climate change projections, natural disasters are expected to impose a worsening burden on emergency responders and communities, and to further challenge the management capabilities of governments. The 47th Parliament has an opportunity to build on its predecessor’s legacy of reforming federal disaster management arrangements, and careful scrutiny of Australian Government spending to promote preparedness, response and recovery.
This article recaps recent disasters and climate change projections, and outlines current governance arrangements for disaster response and climate adaptation. It explores key issues for the 47th Parliament, including the inadequacy of federal–state emergency management arrangements in light of recent disasters; the right balance between investment in prevention, mitigation and response; and planning for the growing fiscal impact of natural disasters.
Background: natural disasters in Australia
Australia has always experienced natural disasters, but their frequency, severity and cost is increasing as climate change progresses. As concluded in the 2020 report of the Royal Commission into National Natural Disaster Arrangements (RCNNDA) : ‘Natural disasters have changed, and … the nation’s disaster management arrangements must also change’ (p. 22).
Recent major disasters
During the 46th Parliament, Australia experienced multiple large-scale disasters in succession, starting with the continuation of the 2017–19 drought . The year 2019 was Australia’s warmest and driest year ever recorded . Climatologists said the drought surpassed the Federation, Second World War and Millennium droughts in severity . Parliament heard that farmers struggled to put food on their own tables , and ‘financial wellbeing, mental health, employment and family relationships’ suffered across broader rural and regional communities (p. 39). The impact on Murray–Darling Basin communities- human and ecological- was severe . Multiple mass fish die-offs occurred, and the competing environmental and socio-economic stresses threatened to ‘ destroy the Murray–Darling Basin plan ’ (see the article ‘ Water, including the Murray-Darling Basin ’ in this Briefing book ).
Drought is sometimes described as a tragedy in slow motion , requiring different response mechanisms to more sudden disasters. The arrangements for federal–state cooperation on drought measures are set out in the 2018 National Drought Agreement . The 2019–21 Senate Inquiry into the Federal Government’s Response to the Drought identified gaps in the available support payments, including restrictive eligibility criteria, burdensome application processes, unclear communication about available support and a pattern of mostly ‘reactive’ expenditure.
The 2019–20 Black Summer bushfires were characterised by an unprecedented extent of high-severity fire across eastern Australia. Thirty-three people died , 24–33 million hectares were burnt , a pall of smoke covered east coast cities and thousands were hospitalised due to smoke inhalation . Researchers have warned some rainforests and peatland may never recover . At the commencement of the 47th Parliament, some survivors are still in temporary accommodation .
The Morrison Government was criticised for allegedly failing to act decisively on warnings of a catastrophic fire season, reducing aerial firefighting funding, an ad-hoc approach to resource deployment and poor communication with relevant state authorities- a state firefighting chief claimed he first learned of planned Australian Defence Force (ADF) deployments through the media. Longer-term recovery measures have been found wanting , with ongoing homelessness in fire-affected regional communities in Victoria and NSW. The RCNNDA , the Senate Finance and Public Administration Committee and separate state inquiries reported on the disaster, and the RCNNDA recommended an overhaul of national disaster management arrangements.
The catastrophic February–March 2022 east coast floods saw rainfall records fall across south-east Queensland and north-east NSW, leading to flash and riverine flooding from Maryborough in Queensland to Grafton in NSW. Flooding also occurred on NSW’s Central Coast, in Sydney , in the Illawarra and on the NSW South Coast . In Lismore, northern NSW, the unexpectedly high flood peak of 14.4 metres ( dwarfing the previous record of 12.1 metres ) meant thousands had to be rescued . Local reporting suggested most rescues were conducted by civilians , as the scale of the task overwhelmed official response capabilities. More than 20 people died nationally , and the Insurance Council of Australia estimated $3.35 billion in insured losses, making it ‘ the costliest flood in Australia’s history ’.
Criticism of the federal, state and local flood response has been particularly fierce in northern NSW, where residents and media reported triple zero calls going unanswered , civilians chartering their own helicopters to meet the unmet need for aerial rescues and food drops; miscommunication about ADF deployments; the unequal provision (at first) of federal financial support to flood victims in adjacent electorates, and mixed messages from federal and local government about Lismore’s future , preventing informed repair and reconstruction decisions. The National Resilience and Recovery Agency (NRRA) was forced to defend its earlier decision ‘to omit Lismore from its priority areas for flood mitigation funding ’ and the slow delivery of relief payments . The NSW Government has commissioned an independent inquiry , which at the time of writing was hearing from flood victims in northern NSW . Media report that the inquiry has ‘ heard countless tales of government unpreparedness during the peak of the crisis, in the recovery centres and as efforts continue to find accommodation for the thousands left homeless’.
The Inquiries and reviews database catalogues 315 disaster inquiries and reviews from 1886 to 2020, and all recommendations from 186 inquiries and reviews from 2003 to 2020. Inquiries provide a space for survivors to tell their stories. However, some researchers have suggested less adversarial and more holistic methods of identifying lessons , and others have argued for greater focus on implementation .
The natural disaster and extreme weather outlook
The 2020 RCNNDA report stated that ‘Australia’s disaster outlook is alarming’, with climate change exacerbating bushfires, extreme rainfall and flooding (p. 68), although the CSIRO has also reported that the implications of climate change for droughts, damaging hail, tropical cyclones and other storms are less clear .
Actuaries specialise in assessing the financial consequences of risks, and are often employed by insurers, banks and investment managers. The Australian Actuaries Climate Index (AACI) tracks changes in the frequency of extreme temperatures, heavy precipitation, dry days, strong wind and changes in sea level across Australia, ‘because extremes have the greatest potential impact on people and, often, the largest cost to the economy’. The AACI shows significantly worsening extreme weather risk, shown by an index number above zero (see Figure 1).
Figure 1 Australian Actuaries Climate Index
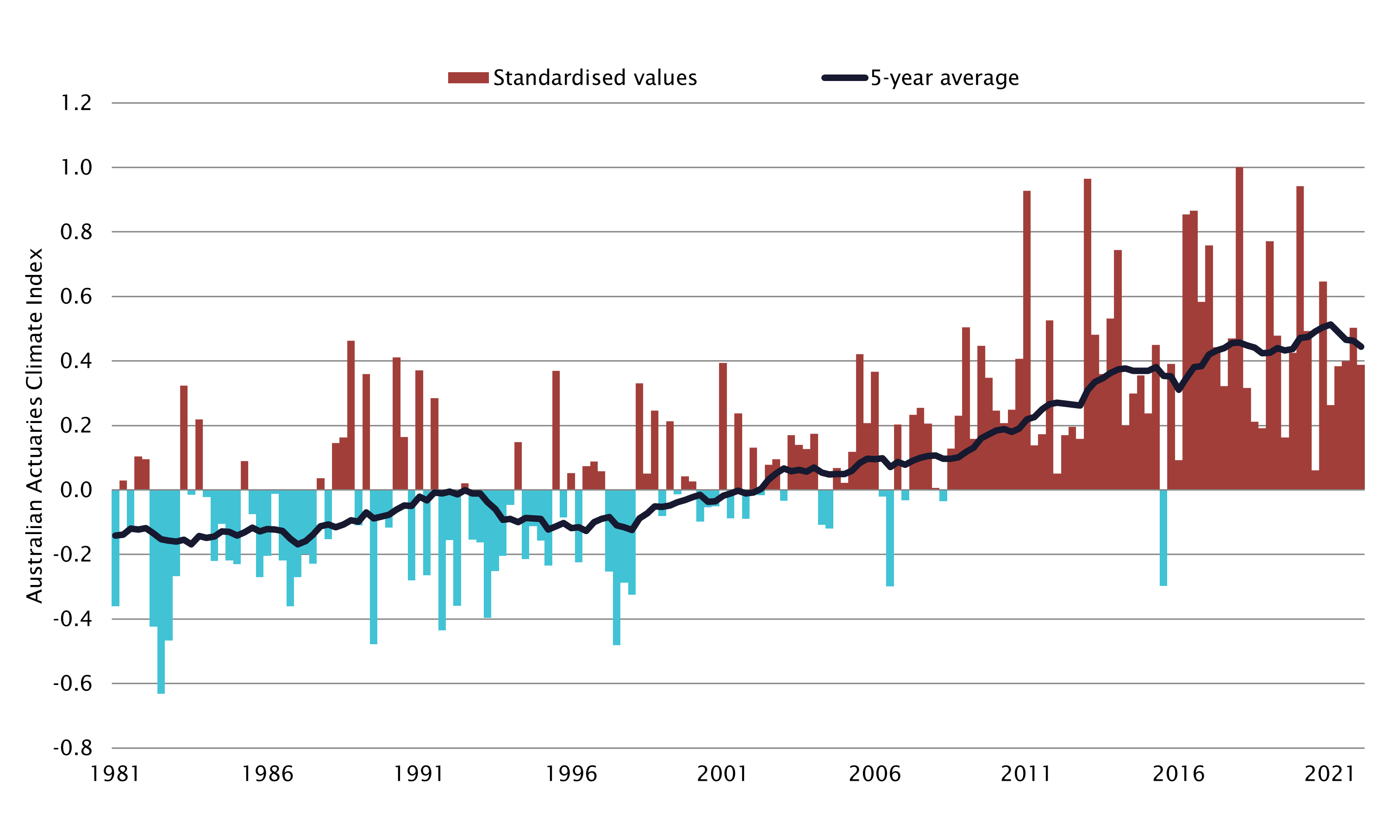
Source: Actuaries Institute, Australian Actuaries Climate Index website .
The RCNNDA quoted evidence from the Australian Business Roundtable for Disaster Resilience and Safer Communities that ‘Direct and indirect disaster costs in Australia are projected to increase from an average of $18.2 billion per year to $39 billion per year by 2050, even without accounting for climate change '.
The Business Roundtable, in partnership with Deloitte Access Economics, published an Update to the economic costs of natural disasters in Australia in October 2021, which concluded that even under a low emissions scenario, the cost of natural disasters in Australia is estimated to increase to $73 billion per year by 2060 . Scenarios with higher global emissions can expect commensurately higher disaster costs (p. 1). Under a high-emissions, 3 °C warming scenario:
By 2060 costs reach $94 billion, representing a 29% increase relative to the low emissions scenario. Over the next 40 years the different trajectories will lead to a $125 billion difference in cumulative cost in present value. Even if a low emission scenario is achieved, the cost of natural disasters is forecast to be $1.2 trillion in cumulative costs over the next forty years.
Current disaster response and climate adaptation arrangements
Strategic foundation: ‘shared responsibility’.
The 2011 National strategy for disaster resilience (NSDR) , negotiated through the former Council of Australian Governments, is the strategic foundation of current federal disaster arrangements. It frames disaster resilience as ‘a shared responsibility for individuals, households, businesses and communities, as well as for governments’. It has an objective of ‘community empowerment’, but also outlines very significant roles for all levels of government.
Academics have criticised the ‘shared responsibility’ framing for its ambiguity on the division of responsibilities , and for the tension between the placement of governments at the centre of disaster risk management and the emphasis on ‘community empowerment’ .
Further, the Productivity Commission has warned of ‘the risk that the expectation of government financial assistance will create “moral hazard” … by reducing incentives for individuals and businesses to take out insurance and invest in mitigation’ (p. 25) - and that the same risk of moral hazard could apply to Australian Government assistance to states and territories (p. 86).
Federal disaster response arrangements
Constitutional power for emergency management largely rests with the states and territories. The Australian government crisis management framework (AGCMF) , first released in 2012, outlines that ‘states and territories are the first responders to any incident that occurs within their jurisdiction and have primary responsibility for the protection of life, property and the environment within the bounds of their jurisdiction’ (p. 7).
However, the AGCMF acknowledges that ‘the Australian Government possesses operational and strategic capabilities that can ensure decisive action is taken during a nationally significant crisis’ and ‘recognises the expectations of the Australian public that it will take a leadership role in nationally significant emergencies’ (p. 7).
The AGCMF outlines the financial and non-financial assistance the Australian Government may provide at various phases during a crisis. The main forms of direct Australian Government financial support for individuals are the Australian Government Disaster Recovery Payment and the Disaster Recovery Allowance , delivered via Services Australia (see ‘Further reading’).
The Australian Government can also provide disaster funding to states and territories. Most is coordinated through National Cabinet or Council on Federal Financial Relations mechanisms.
The main formal mechanism is an Australian Government ‘disaster relief’ payment, typically a contingent payment made to a state or territory on a cost-sharing basis. The bases for these payments are the Intergovernmental Agreement on Federal Financial Relations , the Natural Disaster Relief and Recovery Arrangements (NDRRA) and the Disaster Recovery Funding Arrangements (DRFA) .
In essence, a state or territory makes an application to the Australian Government for payments in response to an eligible emergency, and some costs are shared. The Australian Government has also historically made a range of ad hoc and one-off payments for specific disasters.
These expenditure types are budgeted as ‘contingent payments’. In simple terms, the legislative and governance frameworks are in place to enable these payments following an eligible event, but the timing and quantum of funding are unknowable- and not forecast- before the trigger event (see ‘Natural disaster expenditure in the Australian Government Budget’ below).
Additional assistance may be provided through disaster resilience programs, grants to local government and non-government organisations, one-off payments to individuals or businesses and special tax concessions or exemptions.
Under the Australian government disaster response plan (COMDISPLAN) , the Australian Government may also provide non-financial aid to the states and territories, such as mapping services, planning expertise and physical and logistics support (for example, the airlift capabilities of the ADF). This non-financial support is coordinated through Emergency Management Australia (EMA) , a division of the Department of Home Affairs. EMA also provides national ‘ 24/7 all-hazards situational awareness and monitoring ’ (p. 14) through the National Situation Room , formerly known as the Australian Government Crisis Coordination Centre.
Changes to natural disaster arrangements during the 46th Parliament
The Morrison Government oversaw changes to the institutional architecture for managing national-scale emergencies. Under the National Emergency Declaration Act 2020 , the Governor-General, on the advice of the Prime Minister, may now declare a ‘national emergency’.
This makes a range of powers available to ministers to assist with response and recovery, including the ability to suspend the usual administrative rules welfare recipients must comply with in order to receive Services Australia payments, and the ability to mandate the reporting of emergency stockpiles and assets.
Chapter 7 of the RCNNDA report discussed the role of the ADF following the 2019–20 bushfires, and noted ‘a lack of understanding about the role, capacity and capability of the ADF in relation to natural disasters’ (p. 186). In response, the COMDISPLAN was updated in December 2020 to outline the process for the Australian Government to respond to requests for assistance from state and territory governments, including for ADF assets.
The NRRA was launched in May 2021 . It brought together the former National Bushfire Recovery Agency and the National Drought and North Queensland Flood Response and Recovery Agency, in addition to disaster-related functions from other departments. The NRRA was intended to ‘provide support to local communities during the relief and recovery phases following major disasters’, and also provide advice on mitigation programs for future major disasters.
Former emergency managers have been critical of the NRRA. For example, a former Commissioner of the ACT Emergency Services Authority has criticised it for undertaking ‘almost no’ disaster mitigation work , and argued its creation- sitting alongside EMA- was ‘a recipe for duplication and administrative hubris’.
The Emergency Response Fund (ERF) was created through the Emergency Response Fund Act 2019 . As outlined in the Parliamentary Library’s Bills digest , the ERF was initially funded through the transfer of approximately $4 billion from the Education Investment Fund, and is to be used for financial assistance for projects, services or technologies aimed at enhancing disaster recovery or improving post-disaster resilience.
The Act enables the Government to access up to $150 million each year to fund emergency response and recovery efforts following natural disasters, and up to $50 million each year to fund initiatives that build resilience and reduce the risk of future disasters.
As at 30 June 2021, the ERF was valued at $4.7 billion . Following the 2019–20 bushfires and 2022 floods, the Morrison Government faced criticism for not having disbursed the full available annual funding from the ERF since its foundation . According to the Department of Finance, the ERF paid out $50 million in 2020–21 and a total of $150 million in 2021–22 as at 31 March 2022.
In the last days of the 46th Parliament, the Treasury Laws Amendment (Cyclone and Flood Damage Reinsurance Pool) Act 2022 established a reinsurance pool for cyclone damage backed by a $10 billion Australian Government guarantee. While the legislation was originally developed to address cost pressures in northern Australia, parliamentary debate- against the backdrop of the 2022 floods- focused on the possibility of extending the model to cover all flood or natural disaster risks nationally, to address other communities’ worsening risk profile under climate change (see ‘Further reading’).
Both major parties, the Insurance Council of Australia (ICA) and most other stakeholders supported the reinsurance pool. However, the ICA’s 2022 policy platform Building a more resilient Australia called for greater investment in disaster mitigation measures as the only way to sustainably reduce natural peril insurance premiums long term. The ICA also called for federal–state–local government collaboration on land planning and development reform in consideration of worsening extreme weather risk.
In disaster management, mitigation refers to steps to lessen or minimise the adverse impacts of foreseeable hazardous events , such as building a flood levee, cyclone-proofing a house or creating a fire break. The word is used in that sense in this article. In the context of combatting climate change, mitigation refers to ‘ making the impacts of climate change less severe by preventing or reducing the emission of greenhouse gases ’. Since the UK’s landmark 2006 Stern Review , experts have recommended an economic strategy of both reducing global emissions as fast as possible and investing early in climate change adaptation and/or disaster mitigation, to manage locked-in climate change impacts. Recent Australian Government-funded mitigation programs include the Preparing Australia Program , the Disaster Risk Reduction Package , the Future Drought Fund and the National Flood Mitigation Infrastructure Program .
Climate adaptation governance arrangements
The former Council of Australian Governments agreed a federal framework on climate change adaptation roles and responsibilities in 2012. Under this framework, the Australian Government’s role and responsibilities include:
- providing leadership on national adaptation reform
- managing Australian Government assets and programs
- providing national science and information
- maintaining a strong, flexible economy and a well-targeted social safety net.
Australia’s National climate resilience and adaptation strategy 2021–2025 was updated and released in October 2021 , prior to COP26 (see the article ‘ Climate change and emissions reduction ’ in this Briefing book ). The strategy’s 3 main objectives are to:
- drive investment and action through collaboration
- improve climate information and services
- assess progress and improve over time.
Critics have said the strategy lacks substance , citing its lack of specific policies, detailed targets and funding. Other commentators have conceded its limitations, but see it as ‘ a good start ’.
The national adaptation strategy’s second objective will largely be met by the Australian Climate Service , established in July 2021 . The service functions by compiling Australian Government climate and natural hazard research into one platform , which can be used to increase the capacity to plan and prepare for natural disasters and ‘build a more resilient Australia’.
The service was provided with $209.7 million over 4 years (and $37.3 million annually ongoing) in the 2021–22 Budget (p. 66).
Natural disaster expenditure in the Australian Government Budget
Extreme weather is already resulting in significant unplanned Budget expenditure, although budgetary reporting conventions make monitoring the overall picture challenging.
The Morrison Government’s 2022–23 Budget anticipated significant expenditure from past natural disasters. For example, Budget paper no. 2: 2022–23 included:
- the multi-billion dollar cross-portfolio ‘Flood Package’ measure in response to the east coast floods (pp. 61–63)
- an additional $116.4 million over 3 years from 2021–22 for Black Summer Bushfire Recovery Grants (p. 155)
- at least $20.1 million for ‘Disaster Support’, including the establishment of a national emergency management stockpile, mental health support for first responders and support following flood and storm events in 2021, to be delivered jointly with the states (p. 159).
Forward estimates in the Budget for ‘natural disaster relief’ in aggregate only show the expected future expenditure due to past disasters.
This is consistent with the funding mechanism by which payments to the states are contingent on an eligible trigger event (see ‘Federal disaster response arrangements’ above). The Budget does not make guesses as to whether and when future trigger events might occur, or how big the funding quantum will be.
Given this approach, it is unsurprising that Australian Government natural disaster relief has consistently exceeded previous ‘estimates’ in recent years (see Table 1), as new widespread disasters occur on the heels of previous ones.
Table 1 Spending on natural disaster relief: forward estimates and actual expenditure
253 | 763 | TBC | |
832 | 327 | 5,176 | |
2 | 482 | 748 | |
10 | 11 | 1,863 | |
2 | 17 | 775 |
(a) The forecast expenditure for the year in the first column according to the Budget for the year prior. (b) The expenditure listed for that year in the Budget of the same year. (c) The expenditure listed for that year in the following year’s Budget. (d) The figures for this year include funding for the establishment of the National Recovery and Resilience Agency. Sources: Australian Government, Budget Paper No. 1: 2022–23 , 171–2; Budget Paper No. 1: 2021–22 , 191; Budget Paper No. 1: 2020–21 , 6–52; Budget Paper No. 1: 2019–20 , 5–42; Budget Paper No. 1: 2018–19 , 6–43; Budget Paper No. 1: 2017–18 , 6–44.
This makes it difficult to use the Budget’s forward estimates to project the future fiscal burden of natural disaster costs relating to climate change.
In addition, the ‘Statement of Risks’ in recent Budgets has been silent with regard to climate-related fiscal risk. This is arguably at odds with recent prudential advice from the Australian Prudential Regulation Authority and the Actuaries Institute .
In contrast, recent Budgets have had a dedicated section on ‘climate spending’, to clarify the implications of spending on environmental action for the national debt.
Albanese Government disaster readiness commitments
The Albanese Government's election platform included policies to implement the Productivity Commission recommendation to invest ‘up to $200 million per year on disaster prevention and resilience’ (to be matched by the states and territories) through a Disaster Ready Fund , on top of existing arrangements. (The Productivity Commission’s 2014 Inquiry into Natural Disaster Funding Arrangements had reported that ‘ Australian Government mitigation spending was only 3 per cent of what it spent post-disaster ’, p. 9.)
Labor also committed to establishing a sovereign aerial firefighting fleet , in partnership with the National Aerial Firefighting Centre (NAFC) and state and territory governments, and to funding Disaster Relief Australia to expand its national veterans-led volunteer corps .
Australia’s National Aerial Firefighting Centre (NAFC) ‘provides a cooperative national arrangement for the provision of aerial firefighting resources for combating bushfires’ (emphasis added). While the NAFC contracts aerial firefighting resources on a national basis, state and territory land management agencies may directly contract resources. The NAFC recently released the National aerial firefighting strategy 2021–26 , which provides an overview of the current NAFC fleet, consideration of the RCNNDA recommendations on national aerial firefighting capabilities and arrangements , and the costs of and opportunities for further aerial firefighting roles. Traditionally, fire seasons in Australia and North America have been well separated, which allowed for aircraft and personnel sharing. However, fire seasons in both the northern and southern hemispheres are lengthening, placing pressure on resource-sharing and leading to calls from prominent serving and former emergency managers for boosts to our national aerial firefighting fleet capability. In contrast, NAFC’s National aerial firefighting strategy 2021–26 states that Australia already possesses ‘good sovereign fleet capability … albeit largely contracted’ , with the exceptions of Large Air Tanker aircraft and - to some extent - Type 1 Rotary Wing aircraft (p. 17).
Other high-level Labor election commitments included :
- reducing red tape around accessing disaster resilience funding
- improving the efficiency of disaster recovery processes
- assisting with rising insurance premiums in disaster-prone regions by reducing the risk of expensive damage; that is, through mitigation.
Intense media and community scrutiny is likely to continue as the new Albanese Government and 47th Parliament navigate the legacy of recent disasters and federal reforms, and seek to improve the nation’s position to manage future disasters in a changing climate.
Further reading Tessa Satherley, ‘ Treasury Laws Amendment (Cyclone and Flood Damage Reinsurance Pool) Bill 2022 ’, Bills Digest , 54, 2021–22 (Canberra: Parliamentary Library, 2022). Michael Klapdor, Income Support for Households Affected by Natural Disasters: a Quick Guide , Research paper series, 2019–20 (Canberra: Parliamentary Library, 2020). Karen Elphick, National Emergency and Disaster Response Arrangements in Australia: a Quick Guide , Research paper series, 2019–20 (Canberra: Parliamentary Library, 2020).
Back to Parliamentary Library Briefing Book
For copyright reasons some linked items are only available to members of Parliament.
© Commonwealth of Australia
Creative Commons
With the exception of the Commonwealth Coat of Arms, and to the extent that copyright subsists in a third party, this publication, its logo and front page design are licensed under a Creative Commons Attribution-NonCommercial-NoDerivs 3.0 Australia licence.
- Work of the Senate
- Dynamic Red
- Seating plan
- House of Representatives
- House of Representatives chamber and business documents
- Live Minutes
- House of Representatives Seating Plan
Get informed
- News & Events
- Watch, Read, Listen
- About Parliament
- Education Resources
- Parliamentary Library
- International Program
- Bills before Parliament
- Assented Bills of previous Parliaments
- Tariff Proposals
- Legislative Instruments
Get involved
- Getting involved in Parliamentary Committees
- Connect with us
- Contact Parliament House
Visit Parliament
- Things to Do
- Art at Parliament
- Plan your Visit
- Media Rules and Filming Applications
Website features
- My Parliament - Log in
- Remote Users - Log in
- ParlInfo Search
- APH website survey
- A - Z Index
Parliamentary Departments
- Department of the Senate
- Department of the House of Representatives
- Department of Parliamentary Services
- Parliamentary Budget Office

Who we are, how to contact us, and where to learn more.

- About Moovaz
- Country & City Guides
- Covid-19 Updates
- Relo Checklist
- Employee Relocation

- Global Citizen Blog
Your source of inspiration, motivation, information and more to spur your next move. Browse Now
- October 13, 2021
- Australia , Safety , The Finder
Natural disasters are catastrophic events that result from the Earth’s natural processes. Some even say they are nature’s way of warning us – noting that some disasters are actually man-made because of climate change. Natural disasters in Australia predominantly can include heatwaves, bushfires, droughts, floods, severe storms, tropical cyclones, earthquakes, tsunamis and landslides.
Table of Contents
Natural disasters in Australia
1. heatwaves.
Just in November 2020, The Washington Post reported that the Australian heatwave in Sydney recorded sustained day temperatures exceeding 40 degrees Celsius – “a feat that had not been accomplished during the month of November in 160 years of record-keeping ,” says weather Editor Andrew Freedman. 2019 was also recorded by the Australian Climate Council as Australia’s hottest and driest year ever (ranking second in a 110-year history).
This is not the first time the nation has saw such temperatures. In 2013 , temperatures have regularly gone beyond the 40 degrees Celsius, resulting in a heatwave lasting more than two weeks. Places like Moomba in South Australia have also regularly recorded temperatures above 48 degrees Celsius.
But this problem is not unique to Australia, as global average temperature rises, hot days get hotter and heatwaves last longer and becomes a more frequent affair. Due to its geographical location and large spatial arid lands, Australia has long been susceptible to especially high temperatures.
As result, skin cancer is a major cause of illness in Australia, accounting for approximately 80 percent for all newly diagnosed cancers yearly. As a result, Australia has one of the highest rates of skin cancer in the world.
2. Bushfires
Record-breaking temperatures and months of severe drought have also caused a series of massive bushfires across Australia over the years, with the 2019 to 2020 bushfires receiving huge international attention. 33 lives were lost and over 11 million hectares of bush, forests and parks to perish (along with many wildlife species). The 2009 Black Saturday bushfire was by the far the most deadly in Australian history, with 173 lives lost, over 4,000 homes and structures destroyed.
According to the BBC, scientists warned that hotter, drier climates will contribute to frequent and intense bushfires. Given that many parts of Australia have been in drought conditions due to its climate, this makes it much easier for bushfires to spread and grow rapidly. Sometimes, it happens sporadically, too: “Grassland fires frequently occur after good periods of rainfall which result in abundant growth that dries out in hot weather,” says the Geoscience Australia department.
Natural climate patterns like are also said to contribute El Niño and La Niña to the ecosystem, causing wetter or drier weather across the globe, depending on geographical location.
Just this March, an estimated 18,000 people were evacuated in New South Wales due to severe floods resulting from heavy rainfall. The BBC reported, “days of torrential downpours have caused rivers and dams to overflow around Sydney and in southeast Queensland,” resulting in massive efforts for disaster relief, with the military being deployed to help with search and rescue. Named the 2021 Eastern Australia floods, over one billion Australian dollars’ worth of property have been destroyed and five casualties were recorded.
There are three main types of flooding experienced in Australia, according to the Australian government and the Australian Institute for Disaster Resilience: Riverine floods, flash floods and coastal floods.
Riverine floods are the most common form of flooding in Australia. This happens when contributors like heavy rainfall exceeds the land’s ability to absorb water, causing the latter to become saturated and in turn, causing excess water to overflow into low-lying areas.
Flash floods occur whenever there are short and intense bursts of rainfall. This is one of the more dangerous forms of flooding as it happens very quickly, leaving less time for disaster warning and response.
Coastal floods are caused by rising sea levels, which can be due to several factors like waves, astronomical tides, storm surges, strong onshore winds and more.
Natural climate patterns like El Niño and La Niña also contribute to the ecosystem, causing wetter or drier weather across the globe, depending on geographical location.
4. Droughts
Defined as a decline (or lack) in precipitation over a three-month period by the Australian Bureau of Meteorology, Australia is no stranger to droughts. Since the 1860s, severe drought has occurred in Australia at least once every 18 years, on average. On record, there have been nine major Australian droughts – largely owing to the semi-arid climate that spans across the largest parts of Australian lands.
In the years 2017 to 2019, Australia experienced a decrease in rainfall which led to record warm temperatures and exacerbated dry conditions. Natural climate patterns like El Niño and La Niña also contribute to the ecosystem, causing some regions to experience less rainfall than normal, which in turn results in drier lands. Drier lands also mean an increased risk for bushfires, this explains why droughts and bushfires often go hand-in-hand.
5. Cyclones
Also known as hurricanes or typhoons, cyclones are fairly common in Australia. On average, Australia experiences about 13 cyclones a year and cyclone season typically runs from November to April. However out of all cyclones, only half will ever become severe and reach landfall. North-western Australia is known to be the most cyclone-prone region.
A cyclone’s destructive effect is measured based on five categories: 1 being the lowest impact and 5 being catastrophic. However, it is important to note that the extent of destruction also depends on where it makes landfall – a location with a huge population and many buildings, or an arid land.
For example, one of the worst cyclones to hit Australia is Cyclone Marcia – a category 5 cyclone that hit Queensland in 2015, destroying over 350 homes, damaged nearly 2,000 properties and resulted in nearly USD $600 million dollars in damage. However, due to quick disaster warning and response efforts, there were no fatalities.
Due to the warm oceans in the tropical regions surrounding Australia, cyclones are fairly common as the conditions for it to take place requires sea surface temperatures to be above 26.5 degrees Celsius. In addition, storms also tend to happen more frequently in Australia than any other natural hazard.
6. Hailstorms
Hail is a form of solid precipitation, hailstones form when raindrops are carried upward by thunderstorms into extremely cold areas of the atmosphere and freezes. Due to an unstable atmosphere caused by climate change, Australia is said to expect more hail events in some of its largest cities, as noted by a researcher at the UNSW Sydney’s Climate Change Research Centre.
In January 2020, Melbourne and Canberra experienced golf-ball sized hail during separate storms and a series of flash floods. Winds of up to 116 kilometre per hour was also recorded. More than 1,500 people reported damaged property including cars and houses.
In general hailstorms are not as destructive to human life as long as people stay indoors. However, the damage to property can take quite a big hit depending on the size of the hail. Hence, hailstorm fatality is relatively low, but it can be a huge inconvenience to daily life.
7. Earthquakes
According to Geoscience Australia , the land experiences about 100 earthquakes measuring below a magnitude of 5 (measured on the Richter scale) each year. Earthquakes of such magnitudes can be life-threatening if no proper evacuation system is implemented within a state. However, given Australia’s swift modern earthquake monitoring, alert and evacuation system, earthquakes of such magnitudes generally result in little to no human fatalities. However, buildings with poor structure or weak foundations may suffer damage regardless.
Earthquakes are primarily caused by tectonic plate shifts that take place in the earth’s crust. The Australian plate is also the fastest moving continental land mass on Earth and is colliding into the Pacific and Eurasian Plate. This generates a lot of compressive stress on the Australian continent. The earthquakes are therefore caused by the sudden release of this stress whenever rocks deep underground break and move along a fault line.
Earthquakes measuring above a magnitude of 5 usually occur once every two years. Australia’s largest recorded earthquake happened in 1988 at Tennant Creek, measuring a magnitude of 6.6.
8. Tsunamis
Tsunamis are primarily caused by tectonic plate convergence – this is an event when two tectonic plates collide, causing a rupture in the earth’s lithospheric crust and sending tidal waves into land masses. In short, yes, tsunamis are caused by violent seafloor movement, or earthquakes. However other things like landslides, undersea volcanic activity and meteorite impact can also cause tsunamis.
While not a common occurrence in Australia, tsunamis are always a possibility given Australia’s geographical location that is bounded by the Pacific and Eurasian plates where convergence occur quite frequently (see above).
The most recent tsunami in Australia was triggered by the devastating 9.0 magnitude earthquake that occurred in the Tohoku, Japan in March 2011. The earthquake sent waves measuring 35 centimetres high into the Port Kembla coastline. Strong currents were also recorded in several locations throughout Sydney harbour. Though this tsunami was mild and an after-effect of Japan’s devastation, the threat is still present.
9. Landslides
A landslide refers to the movement of mass (rock, debris or earth) down a slope. They are also known as a type of “mass wasting” – any down-slop movement of soil and rock as a result of gravity. Landslides can occur in a variety of environments that has steep or gentle slopes – some common environments being mountain ranges, coastal cliffs and even underwater. The main cause of landslide is gravity, but other natural factors like heavy rainfall or earthquake can trigger a landslide, too.
The last catastrophic landslide occurred in 1997. The Thredbo landslide happened on July 1997 at the village and ski resort of Thredbo, New South Wales. Two ski lodges were destroyed and there were 18 fatalities.
Landslides are most common in hilly or mountainous landscapes, coastlines and river valleys. Regions with poor bedrock and soil conditions are also most susceptible to landslides. It is important to note that landslides can also be caused by human activities like coal mining.
How to prepare for natural disasters
While we can never escape the effects of Mother Nature, we can do our part to prepare for it. Peruse the following tips below on how to best prepare yourself:
- Australian Red Cross: REDiPlan
REDiPlan is an all-hazards emergency preparedness tool for all individuals and households in Australia. This includes a simple four-step approach: Get in the know, get connected, get organised and get packing.
You can even create an emergency plan with its guidelines and build your own survival kit with this checklist . Or, download its Get Prepared app to have access to all its emergency resources.
- Get insured
Ensure that all valuable liabilities are protected by an insurance plan. This includes your home, valuable materials, vehicles and most importantly, yourself and your family members. Your insurance plan should also cover damages caused by natural occurrences.
- Familiarise yourself with disaster alerts and warning systems
The Australian Bureau of Meteorology and Standard Emergency Warning Signal (SEWS) are handy resources and tools to keep you updated on potential natural threats. Listen out for the SEWS siren broadcasted over radio and national TV channels, or head to the Bureau of Meteorology’s website for updates on climate conditions.
Additionally, never ignore Emergency Alert text messages sent by your local government and always heed their warnings or advice on evacuation protocols.
- Know your numbers
In case of any life-threatening emergencies, below are the important numbers to take note of:
000: Police, ambulance or fire services
106: If you have a hearing or speech impediment, dial this number from a teletypewriter to access the Text Emergency Relay Service.
112: This is the international standard emergency number. (Should you need an interpretation, call 131 450.)
132 500: This directs to the State Emergency Services (SES) for emergency help during natural disasters
13-11-14: 24-hour counselling service by Lifeline Australia . If a life is in danger, contact 000.
For state-specific contacts, visit the state and territory emergency services organisations page to contact various Emergency Services Organisations (ESO) for advice on what to do during an emergency.
If you do find yourself in a disaster-stricken area and are safe, register yourself as “safe” or locate family or friends in other affected areas using the Register.Find.Reunite service by the Red Cross.
- Be emotionally prepared
This is vital as it helps you stay in control to properly execute emergency plans. It also helps to reduce psychological distress and long-term mental health problems that can ensure as a result of trauma.
The Australian Psychological Society advises on three simple steps (AIM) to being psychologically prepared for emergences:
A nticipate that feeling worried or anxious are normal and natural responses to any possible life-threatening situation.
I dentify specific physical sensations associated with your feelings of anxiety and whether you might have additional thoughts that might exacerbate your existing fears.
M anage your responses using controlled breathing techniques. Self-talk to stay calm as possible in order to focus on the immediate task at hand.
How to cope after a natural disaster
Surviving a life-threatening event is a very distressing experience and should not be ignored. Feelings of fear, anxiety and even trauma can lead to mental health illnesses like post-traumatic stress disorder (PTSD), survivor’s guilt and more.
Below are some organisations you can consult to receive post-disaster relief and support:
- Helplines: Hotlines like Beyond Blue (1300-22-4636) and Lifeline (see above) provide support and advice for dealing with emotional impacts after surviving a natural disaster.
- Mental health professionals: Speak to your nearest doctor, counsellor or therapist about your situation.
- Financial assistance: Contact Services Australia (180-22-66) to find out if you’re eligible for government relief payments after a natural disaster. Visit here to see the various recovery payments available.
Natural disasters are distressing catastrophic events that happen regardless of human intervention. Even as the world progresses with various disaster-adapting systems, no amount of technological progress can prevent natural disasters. The best we can do is to prepare for it, and reduce casualties and fatalities as much as possible.
Feeling ready to make the big move?
Head over here to begin your journey Or start ticking off your relocation checklist here
Still unsure?
Learn more about Australia here Explore more destinations here

Join the Moovaz Global Citizen Community
Get inspiration, motivation, information and more to kickstart your potential next chapter in life.
© 2023 Moovaz. All rights reserved

- Privacy Overview
- Strictly Necessary Cookies
- 3rd Party Cookies
- Cookie Policy
This website uses cookies so that we can provide you with the best user experience possible. Cookie information is stored in your browser and performs functions such as recognising you when you return to our website and helping our team to understand which sections of the website you find most interesting and useful.
Strictly Necessary Cookie should be enabled at all times so that we can save your preferences for cookie settings.
If you disable this cookie, we will not be able to save your preferences. This means that every time you visit this website you will need to enable or disable cookies again.
This website uses Google Analytics to collect anonymous information such as the number of visitors to the site, and the most popular pages.
Keeping this cookie enabled helps us to improve our website.
Please enable Strictly Necessary Cookies first so that we can save your preferences!
More information about our Cookie Policy
- Share full article

First Fires, Then Floods: Climate Extremes Batter Australia
Many of the same areas that suffered through horrific bush fires in 2019 and 2020 are now dealing with prodigious rainfall that could leave some people stranded for weeks.
Supported by
By Damien Cave
Photographs and Video by Matthew Abbott
- Dec. 11, 2021
WEE WAA, Australia — Two years ago, the fields outside Christina Southwell’s family home near the cotton capital of Australia looked like a dusty, brown desert as drought-fueled wildfires burned to the north and south.
Last week, after record-breaking rains, muddy floodwaters surrounded her, along with the stench of rotting crops. She had been trapped for days with just her cat, and still didn’t know when the sludge would recede.
“It seems to take for bloody ever to go away,” she said, watching a boat carry food into the town of Wee Waa. “All it leaves behind is this stink, and it’s just going to get worse.”
Life on the land has always been hard in Australia, but the past few years have delivered one extreme after another, demanding new levels of resilience and pointing to the rising costs of a warming planet. For many Australians, moderate weather — a pleasant summer, a year without a state of emergency — increasingly feels like a luxury.
The Black Summer bush fires of 2019 and 2020 were the worst in Australia’s recorded history. This year, many of the same areas that suffered through those epic blazes endured the wettest, coldest November since at least 1900. Hundreds of people, across several states, have been forced to evacuate. Many more, like Ms. Southwell, are stranded on floodplain islands with no way to leave except by boat or helicopter, possibly until after Christmas.

Murray-Darling Basin
Bedgerabong
We are having trouble retrieving the article content.
Please enable JavaScript in your browser settings.
Thank you for your patience while we verify access. If you are in Reader mode please exit and log into your Times account, or subscribe for all of The Times.
Thank you for your patience while we verify access.
Already a subscriber? Log in .
Want all of The Times? Subscribe .
Advertisement
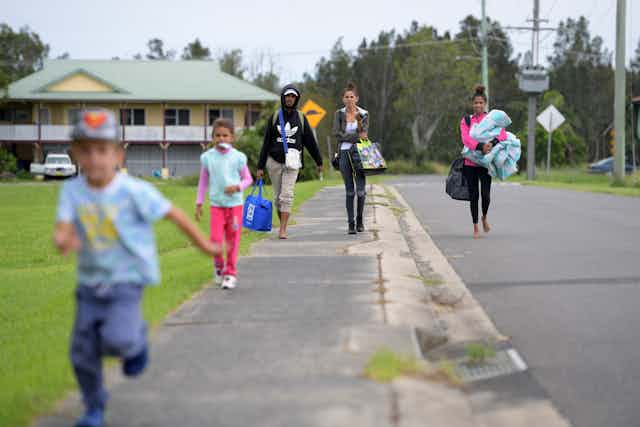
Like many disasters in Australia, Aboriginal people are over-represented and under-resourced in the NSW floods
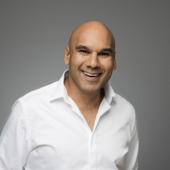
Research Associate & PhD Candidate, Australian National University
Disclosure statement
I thank Dr Francis Markham for his contributions in the development of this article.
Australian National University provides funding as a member of The Conversation AU.
View all partners
The flooding crisis that has engulfed much of Australia’s east coast is yet more evidence of the catastrophic impacts of climate change. While all people are forced to confront similar challenges such as forced evacuations, loss of property and damage to businesses, Aboriginal people are once again over-represented in the number of people impacted by disaster.
Northern NSW is home to many Aboriginal people living in large townships such as Lismore, Ballina, Casino and Kempsey. It is also home to a vast array of discrete Aboriginal communities such as Cabbage Tree Island, Box Ridge, Gundurimba, Wardell, Maclean (Hill Crest), and more.
A quick glance at the regions that have been officially declared a natural disaster zone in NSW reveals that approximately 36,509 Aboriginal and Torres Strait Islander people have been directly impacted by the floods in NSW, or 4.2% of those affected by floods.
Focusing on regional areas outside of Sydney, the proportion of Aboriginal and Torres Strait Islander people affected by the floods jumps to 6.2%. Compare this with the fact Aboriginal people make up 3.3% of the general population and it becomes clear Aboriginal and Torres Strait Islander people are once more disproportionately affected by disaster.
But these numbers are just one dimension. Aboriginal and Torres Strait Islander people are a youthful population, with more than half being under 24 years old . This means that of the Aboriginal and Torres Strait Islander people affected, most are children and young people.
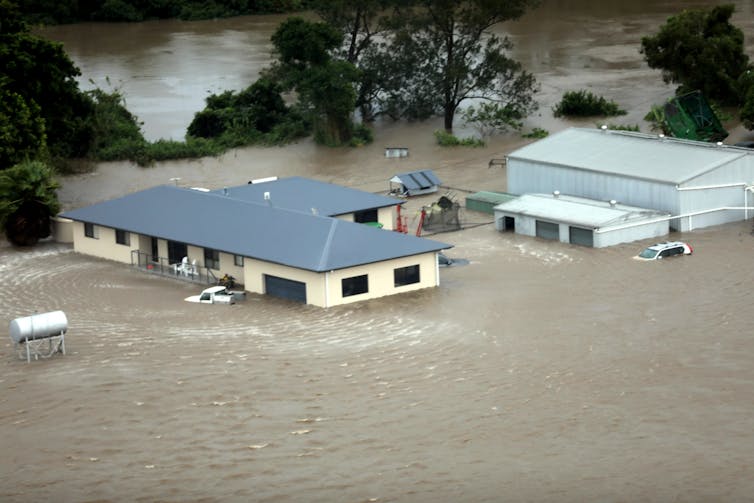
Read more: 5 big ideas: how Australia can tackle climate change while restoring nature, culture and communities
Stories of survival and strength
With such widespread flooding, emergency services and disaster relief organisations have struggled to keep up, and in the midst of the chaos, Aboriginal communities have found themselves isolated and in some cases forgotten.
At Cabbage Tree Island outside of Ballina, the entire community has been inundated. In the midst of the evacuation, elders and families did not want to evacuate because they had nowhere to go. Bundjalung woman Delia Rhodes shared with ABC PM Radio :
You have Elders and families with children sitting around for hours waiting to get accommodation. It’s very frustrating.
Community members with access to boats took it upon themselves to drop food, baby supplies, and medicine to people who found themselves stranded.
Naomi Moran, General Manager of Indigenous newspaper The Koori Mail shared with NITV :
You know what Blackfullas are like, if it happens to one of us, it happens to all of us. The outpouring of offers to support our communities. You know, taking calls from Sydney mob, from Melbourne mob, saying “how can we help?”
In Dubbo, Bundjalung woman Noelle King is organising donations and relief for families in Lismore and surrounding communities, she said to me:
I have lots of family in Lismore who have lost everything but are so grateful they all made it out to safety in time. I have family in Corakai, on the mission who were isolated with no access to supplies and the same seeing Gundurimba and Cabbo going under […] So I’ve reached out to the community of Dubbo and donations have flowed in of clothes, household items, food and other supplies. We will be organising a truck next week and heading over to help where we can.
Sadly, these stories of Aboriginal communities being left behind with little support, or none at all, are all too common. I have written about similar experiences during the 2019-20 bushfires and the COVID-19 pandemic in Aboriginal communities.
There are also questions about when or even if communities can return to their home communities, as Dyonne Anderson, Principle of Cabbage Tree Island public school shared with The Guardian :
We may not return for many months.
These disasters have exposed the consequences of a lack of planning and preparation in and with Aboriginal communities. They have also shown how entrenched inequality produces further vulnerabilities in times of crisis. The same consequences are now being felt in northern NSW.
Read more: The COVID-19 crisis in western NSW Aboriginal communities is a nightmare realised
How can First Nations communities be better supported during climate change?
Disasters such as this have always been a common feature in Australia, but as more and more emissions are pumped into the atmosphere, the impacts of climate change mean the scale and severity of these disasters will continue to increase. Aboriginal and Torres Strait Islander communities are already bearing the brunt of these disasters. A number of things need to happen now, before climate impacts worsen.
It’s vitally important Aboriginal people throughout flood impacted areas are provided with as much community and government support as they require to return home and recover what has been lost. Aboriginal community members have organised a number of crowdfunding campaigns to support Aboriginal people in flood-affected areas.
Two of note include the Bundjalung community flood relief and Support Aboriginal families of Lismore .
It is also time to look beyond individual disasters and develop an integrated response that is led by and centres Indigenous peoples. I suggest this can be done in two ways.
Firstly, we need a national Indigenous disaster resilience framework. My research has uncovered how Indigenous peoples have been made absent in national disaster resilience policies in the past. Without a framework that focuses on our communities, including the distinct impacts of disasters on our peoples, culture and heritage, and the unique legal and governing arrangements within our communities, future policies and responses will continue to fall short.
Second, there is an urgent need to develop and resource an Indigenous climate resilience and adaptation strategy. The recent report by the Intergovernmental Panel on Climate Change, Impacts, Adaptation and Vulnerability , recognises the specific challenges for Indigenous peoples in a changing climate. While closer to home Future Earth Australia, a peak expert group based at the Australian Academy of Science, is developing a National Strategy for Just Adaptation .
But even these strategies and reports are incapable of capturing and communicating our voices, experiences, and ambitions if they are not Indigenous-led and owned.
What these floods have shown, as the bushfires and COVID-19 have shown before, is the indomitable strength of Aboriginal people.
Perhaps it is fitting the national NAIDOC theme for 2022 is “ Get Up! Stand Up! Show Up! ” - because that is exactly what we continue to do for our people in times of disaster.
- Natural disasters
- Aboriginal and Torres Strait Islander peoples
- Access to health care
- Natural disaster mitigation
- First Nations people

Head of Evidence to Action

Supply Chain - Assistant/Associate Professor (Tenure-Track)

Education Research Fellow

OzGrav Postdoctoral Research Fellow

Casual Facilitator: GERRIC Student Programs - Arts, Design and Architecture
Thank you for visiting nature.com. You are using a browser version with limited support for CSS. To obtain the best experience, we recommend you use a more up to date browser (or turn off compatibility mode in Internet Explorer). In the meantime, to ensure continued support, we are displaying the site without styles and JavaScript.
- View all journals
- Explore content
- About the journal
- Publish with us
- Sign up for alerts
- Open access
- Published: 08 December 2021
Likelihood of unprecedented drought and fire weather during Australia’s 2019 megafires
- Dougal T. Squire ORCID: orcid.org/0000-0003-3271-6874 1 ,
- Doug Richardson ORCID: orcid.org/0000-0001-5397-0201 1 ,
- James S. Risbey ORCID: orcid.org/0000-0003-3202-9142 1 ,
- Amanda S. Black ORCID: orcid.org/0000-0002-4775-7540 1 ,
- Vassili Kitsios ORCID: orcid.org/0000-0002-2543-0264 2 ,
- Richard J. Matear ORCID: orcid.org/0000-0002-3225-0800 1 ,
- Didier Monselesan ORCID: orcid.org/0000-0002-0310-8995 1 ,
- Thomas S. Moore ORCID: orcid.org/0000-0003-3930-1946 1 &
- Carly R. Tozer ORCID: orcid.org/0000-0001-8605-5907 1
npj Climate and Atmospheric Science volume 4 , Article number: 64 ( 2021 ) Cite this article
6006 Accesses
35 Citations
82 Altmetric
Metrics details
- Atmospheric science
- Climate and Earth system modelling
- Climate sciences
- Environmental impact
Between June 2019 and March 2020, thousands of wildfires spread devastation across Australia at the tragic cost of many lives, vast areas of burnt forest, and estimated economic losses upward of AU$100 billion. Exceptionally hot and dry weather conditions, and preceding years of severe drought across Australia, contributed to the severity of the wildfires. Here we present analysis of a very large ensemble of initialized climate simulations to assess the likelihood of the concurrent drought and fire-weather conditions experienced at that time. We focus on a large region in southeast Australia where these fires were most widespread and define two indices to quantify the susceptibility to fire from drought and fire weather. Both indices were unprecedented in the observed record in 2019. We find that the likelihood of experiencing such extreme susceptibility to fire in the current climate was 0.5%, equivalent to a 200 year return period. The conditional probability is many times higher than this when we account for the states of key climate modes that impact Australian weather and climate. Drought and fire-weather conditions more extreme than those experienced in 2019 are also possible in the current climate.
Similar content being viewed by others
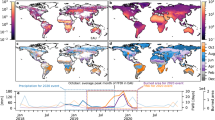
Global increase in wildfire potential from compound fire weather and drought

Increasing frequency and intensity of the most extreme wildfires on Earth
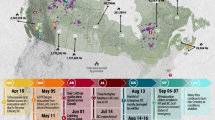
Drivers and Impacts of the Record-Breaking 2023 Wildfire Season in Canada
Introduction.
The 2019–2020 wildfire season in Australia was among the most catastrophic in recorded history, causing severe social, environmental, ecological and economic impacts across the continent. An area larger than the size of the United Kingdom was burned (estimates range from 24 to 34 million hectares 1 ), including at least 21 percent of Australia’s temperate forests 2 , 3 and over 3000 homes 1 . Thirty-three deaths occurred as a direct result of the fires 4 . Hundreds more deaths, and thousands of hospital and emergency-department admissions, have been attributed to the extreme levels of air pollution resulting from the wildfire smoke 5 , 6 . The estimated death toll for animals is in the billions 1 , with fears that some species have been driven to extinction 7 , 8 . Recent estimates of the total economic loss to Australia resulting from the 2019–2020 wildfires are in the order of AU$100 billion 9 , 10 .
Two key factors have been linked to the severity of the 2019–2020 wildfires. First, the exceptionally dry conditions in the years and months leading up to the fire season produced very low fuel-moisture content, especially in eastern Australia 11 , 12 , 13 , 14 . The widespread drought conditions have been connected to the states of the El Niño Southern Oscillation (ENSO) and Indian Ocean Dipole (IOD) in the years and months preceding 2020 15 , 16 , 17 . Second, the extremely hot and dry weather conditions experienced across Australia during the 2019–2020 summer were particularly favorable to fire ignition and spread 11 , 18 , 19 , 20 , 21 . A number of extreme weather records were broken over this period, which included Australia’s highest national daily-averaged temperature (41.9 o C) 15 and record-high values of the Forest Fire Danger Index (FFDI) in areas of all Australian States and Territories 19 . The Southern Annular Mode (SAM), which was strongly in its negative phase during the spring and summer of 2019, has been implicated in the unusually hot and dry conditions across eastern Australia 22 .
Many studies have investigated how climate and weather conditions favorable to wildfires in Australia have changed historically and how they will continue to change into the future. Paleoclimate records indicate an increase in the last century in the occurrence of the fire-promoting phases of both ENSO 23 and the IOD 15 , 24 . These increases may continue in the coming decades 25 , 26 . Observed records since the mid-twentieth century show a trend towards more dangerous fire-weather conditions for much of Australia 27 , 28 , 29 and a corresponding reduction in the time between major wildfires 30 . Future projections of Australian fire weather are strongly region- and model-dependent, but generally indicate increased severity in southeast Australia 31 , 32 , 33 , 34 .
Estimates of the likelihoods of increased susceptibility to fire from extreme climate and weather are essential for policy makers, contingency planners, and insurers. However, such likelihoods are difficult to quantify from observed records, which are limited to approximately the past century and thus provide few samples of extremely susceptible conditions in a given region 28 , 35 , 36 . There was, for example, no direct observational precedent for the high values of FFDI nor the low annual accumulated rainfall total experienced in southeast Australia in 2019 15 . Further, assessment of likelihoods is compounded by nonstationarity in the observed record, resulting, for example, from climate change. Even if past likelihoods could be well determined from the observed record, they may not be representative of current wildfire susceptibility.
Climate models can provide large samples of plausible conditions over short time periods that can be used to reduce uncertainties in quantifying risk. Previous studies have used ensemble seasonal and weather prediction systems to estimate return periods of surge levels in the Netherlands 37 , 38 and of significant wind and wave heights globally 39 , 40 , 41 , 42 . More recently, the approach of quantifying risks of extremes using ensemble climate simulations has been popularized under the acronym UNSEEN, standing for UNprecedented Simulated Extremes using ENsembles. The UNSEEN approach has been used to assess the risk of droughts and heat waves 43 , 44 and to quantify likelihoods of extreme meteorological events, such as instances of unprecedented rainfall 45 , 46 and temperature 47 , and sudden stratospheric warming in the Southern Hemisphere 48 .
In this paper, we use a decadal ensemble climate forecast model to quantify the likelihood of concurrent extreme drought and fire weather in a region of southeast Australia where the 2019–2020 wildfires burned significant area (Fig. 1 ). We focus on two indices averaged over this region (below, overlines denote regional averaging). Our drought index, \(\overline{{{{{{\rm{DI}}}}}}}\) , is defined as the total accumulated rainfall from January to December and quantifies how preconditioned for fires the landscape may be leading into the wildfire season of a given year. We use the December-average FFDI, \({\overline{{{{{{\rm{FFDI}}}}}}}}_{{{{{{\rm{Dec}}}}}}}\) , to quantify how severe fire-weather conditions are near the peak of the fire season of a given year. These indices were selected to capture the unprecedented nature of the drought and fire weather in southeast Australia in 2019. Simultaneous low values of \(\overline{{{{{{\rm{DI}}}}}}}\) and high values of \({\overline{{{{{{\rm{FFDI}}}}}}}}_{{{{{{\rm{Dec}}}}}}}\) indicate elevated susceptibility to wildfires in southeast Australia. Therefore, hereafter, we will refer to their vector as simply “fire susceptibility”.
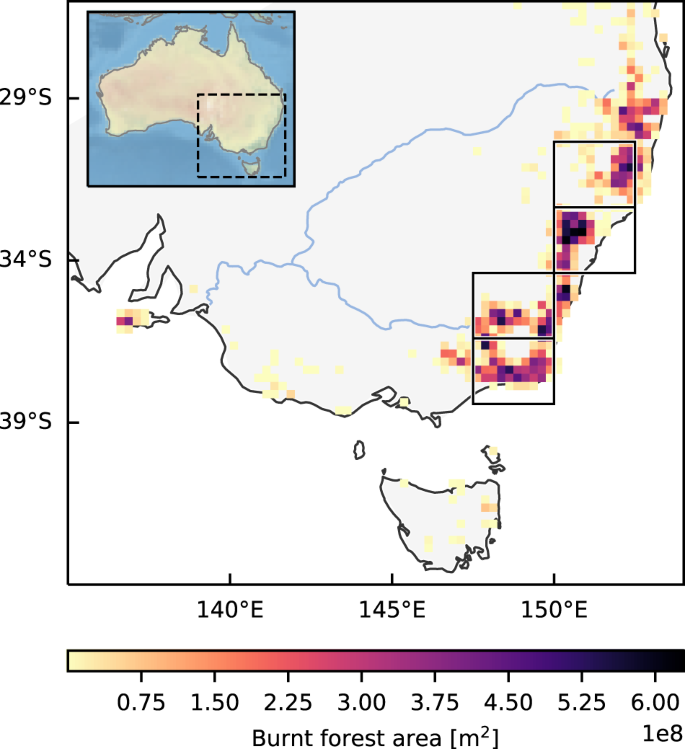
Total burnt forest area between and including October 2019 and February 2020. The black boxes show the forecast model grid cells where the total burnt area is greater than 10% of the cell area (greater than approximately 500,000 hectares). The region covered by these cells is the focus of this paper.
Our climate forecast dataset comprises 10-year long, daily forecasts, each with 96 ensemble members, initialized at the beginning of every May and November over the period 2005–2020. By pooling forecast ensemble members and lead times, these forecasts provide up to 1920 times more samples of \(\overline{{{{{{\rm{DI}}}}}}}\) and \({\overline{{{{{{\rm{FFDI}}}}}}}}_{{{{{{\rm{Dec}}}}}}}\) in the current climate than are available from observed records (“Methods”). After first checking that these many samples provide accurate and independent representations of the real world (“Model fidelity”), we use them to estimate the likelihoods of exceeding extreme values of \(\overline{{{{{{\rm{DI}}}}}}}\) and \({\overline{{{{{{\rm{FFDI}}}}}}}}_{{{{{{\rm{Dec}}}}}}}\) , including the unprecedented values experienced during the 2019–2020 wildfires (“Likelihoods of exceedance”). The very large number of forecast samples yields many years with \({\overline{{{{{{\rm{FFDI}}}}}}}}_{{{{{{\rm{Dec}}}}}}}\) and \(\overline{{{{{{\rm{DI}}}}}}}\) that are simultaneously more severe than the observed 2019 values. This enables us to test the correspondence between unprecedented drought and fire weather in southeast Australia and the states of ENSO, IOD and SAM (“Extreme susceptibility to fire and climate drivers”).
Historical record of fire susceptibility
The historical record of \({\overline{{{{{{\rm{FFDI}}}}}}}}_{{{{{{\rm{Dec}}}}}}}\) and \(\overline{{{{{{\rm{DI}}}}}}}\) is shown in Fig. 2 . These data are calculated from high quality atmospheric reanalysis and gridded rainfall data (“Methods”) and are referred to hereafter as “observations”. The data points in Fig. 2 are shaded according to the year for which they are calculated, with colored shading for years in which severe wildfires occurred in summer in southeast Australia.
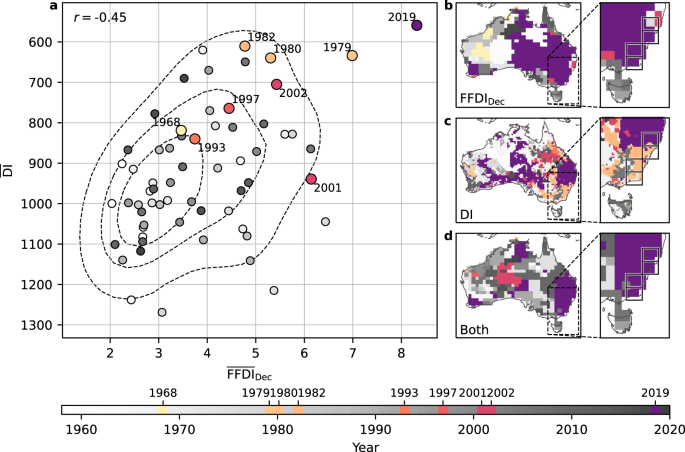
a Values of \({\overline{{{{{{\rm{FFDI}}}}}}}}_{{{{{{\rm{Dec}}}}}}}\) and \(\overline{{{{{{\rm{DI}}}}}}}\) for the period 1958-2020. The dashed lines show contour levels of a two-dimensional kernel-density estimate of the data points shown (levels at 1.5e–4, 3.5e–4 and 5.5e–4, enclosing approximately 87%, 62%, and 27% of the data points) and the value in the top left shows the Pearson correlation coefficient between the two indices. b Year of maximum December-averaged FFDI. c Year of minimim DI. d Year of maximum normalized distance from the mean DI and December-averaged FFDI over 1958-2020. In all panels, data points are shaded according to their year, with colored shading showing years where unplanned summer fires burned at least 250,000 hectares of the region covered by the four model cells shown in Fig. 1 (“Methods”).
Severe fires have generally been associated with extreme values of \({\overline{{{{{{\rm{FFDI}}}}}}}}_{{{{{{\rm{Dec}}}}}}}\) and \(\overline{{{{{{\rm{DI}}}}}}}\) (Fig. 2 ). The values of both indices recorded in 2019 were the most extreme in the 63 years (1958–2020) of observational data available for both indices (Fig. 2 a). Indeed, there was no precedent for the 2019 \(\overline{{{{{{\rm{DI}}}}}}}\) values in the full 121 years (1900–2020) of data available for this index (see Supplementary Fig. 1 ). A large proportion of Australia experienced unprecedented values of December-averaged FFDI in 2019 (Fig. 2 b). Similarly, much of eastern and central Australia accumulated the lowest rainfall annually in 2019 relative to all other years in the joint historical record (Fig. 2 c). Over much of our region of interest, the minimum \(\overline{{{{{{\rm{DI}}}}}}}\) occurred in 1980 and 1982, which were also years in which unplanned summer fires burned extensive areas of southeast Australia. We can quantify the joint extremity of DI and December-average FFDI in a given year as the normalized distance from the mean index values over 1958–2020: \(\sqrt{{{{{{\rm{FFD{I}}}}}_{{{\rm{Dec}}}}^{^{\prime} 2}}}+{{{{{{\rm{DI}}}}}}}^{^{\prime} 2}}\) , where primes indicate the difference from the mean index over 1958–2020, normalized by the standard deviation of the index over the same period. Even at a local scale, this joint quantity was unprecedented in 2019 over much of southeast Australia (Fig. 2 d).
The small number of samples in the observed record makes quantification of the probabilities of extreme \({\overline{{{{{{\rm{FFDI}}}}}}}}_{{{{{{\rm{Dec}}}}}}}\) and \(\overline{{{{{{\rm{DI}}}}}}}\) events very difficult. It is not possible, for example, to directly determine the likelihood of an event more severe than that observed in 2019 simply because no such event has ever been observed. Statistical techniques enable extrapolation of fitted distributions, but require assumptions about the shape of the distribution and still suffer from large uncertainties when the sample size is small 49 , 50 , 51 . Issues with sampling become increasingly restrictive as dimensionality—that is, the number of variables—increases 52 . With its very large sample size, our forecast model (hereafter “model”) provides many in-sample estimates of rare extreme events, allowing for the probabilities of these events to be determined directly from the empirical probability density function 53 .
Model fidelity
In order to provide reliable estimates of likelihoods of fire susceptibility, the model samples must be stable, independent and realistic estimates of the real world 46 . Stability here refers to an absence of systematic changes in the estimates of \({\overline{{{{{{\rm{FFDI}}}}}}}}_{{{{{{\rm{Dec}}}}}}}\) and \(\overline{{{{{{\rm{DI}}}}}}}\) with model lead time. Model stability is necessary for pooling samples at different lead times. Dependence between model samples inflates the sample size without adding new information and arises at short lead times because the ensemble forecasts are initialized from similar initial conditions. We remove dependent samples by considering only model lead times for which ensemble members are uncorrelated (≥37 months: Fig. 3 , see also “Methods”).

The mean Spearman correlation between all combinations of ensemble members at each lead time. Purple shading shows the 2.5–97.5% percentile range from the estimated distribution for uncorrelated data.
To assess the model fidelity, we compare the modeled joint and marginal distributions of \({\overline{{{{{{\rm{FFDI}}}}}}}}_{{{{{{\rm{Dec}}}}}}}\) and \(\overline{{{{{{\rm{DI}}}}}}}\) to the observed distributions over a common time period (Fig. 4 ). Initial assessment of the marginal distributions revealed a systematic dry bias in the modeled \(\overline{{{{{{\rm{DI}}}}}}}\) that was corrected for by applying a simple additive adjustment to the mean \(\overline{{{{{{\rm{DI}}}}}}}\) at each lead time (“Methods”). With this correction applied, we compare the distributions using the 96-member forecasts—denoted f \({}_{96\ {{{{{\rm{mem}}}}}}}^{2005\to }\) —in Fig. 4 a–c. These model distributions show little dependence on lead time (colored lines) and agree generally with the observed data. However, because these forecasts are initialized over a relatively short period of time (2005–2020), there are very limited observations to which they can be compared. Only data within the 7-year period 2014–2020 (comprising 9408 model samples) are shown in Fig. 4 a–c so that the distributions at each lead time are constructed from the same number of samples, thus enabling comparison of the distributions across lead times (“Methods”).
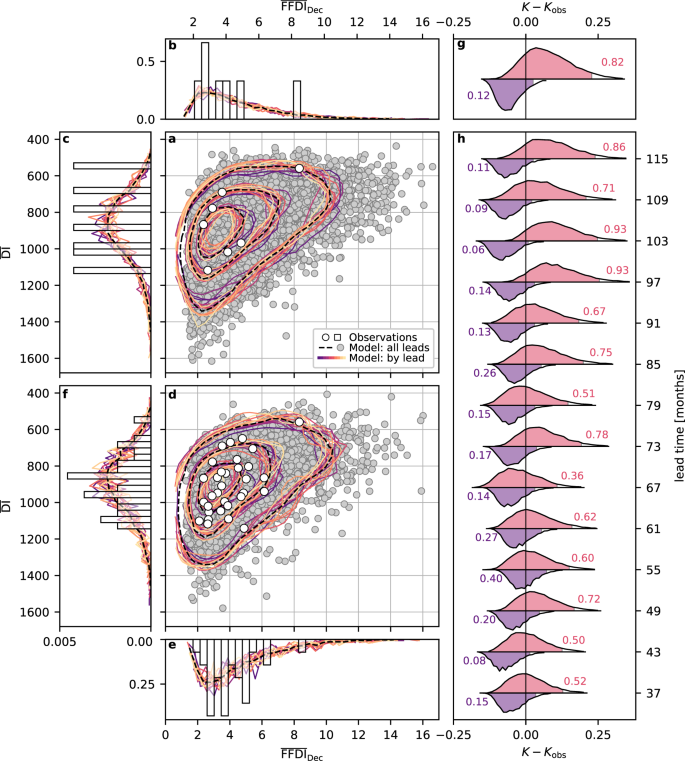
The joint ( a ) and marginal ( b , c ) distributions of observed (white circles and bars) and modeled (gray circles and lines) \({\overline{{{{{{\rm{FFDI}}}}}}}}_{{{{{{\rm{Dec}}}}}}}\) and \(\overline{{{{{{\rm{DI}}}}}}}\) from the bias-corrected f \({}_{96\ {{{{{\rm{mem}}}}}}}^{2005\to }\) model dataset over the period 2014-2020. Lines show probability densities from the model for each lead time (colors) and for all lead times together (black dashed). For the joint distribution, probability densities are from a two-dimensional kernel density estimate and are presented with contour levels at 0.5e–4, 2e–4, and 4e–4 (enclosing approximately 92%, 63%, and 27% of the model data across all lead times). d – f As in a – c , but using f \({}_{10\ {{{{{\rm{mem}}}}}}}^{1980\to }\) data over the period 1989-2020. g Null distributions of the Kolmogorov–Smirnov statistic, K , resulting from bootstrapping the f \({}_{10\ {{{{{\rm{mem}}}}}}}^{1980\to }\) (purple shading) and f \({}_{96\ {{{{{\rm{mem}}}}}}}^{2005\to }\) (pink shading) datasets using all independent lead times. h As in g , but for each lead time separately. In g and h , the distributions are presented as a difference between K and the KS-statistic calculated between the observed and model data, K obs , such that the vertical black line (at K − K obs = 0) indicates the location of K obs in the distributions of K . Colored numbers show the right-tail p -value for the f \({}_{96\ {{{{{\rm{mem}}}}}}}^{2005\to }\) (pink) and f \({}_{10\ {{{{{\rm{mem}}}}}}}^{1980\to }\) (purple) distributions.
We therefore also assess the distributions computed from another set of forecast model data, denoted f \({}_{10\ {{{{{\rm{mem}}}}}}}^{1980\to }\) . These data are produced using the same decadal forecast system and initialization dataset as f \({}_{96\ {{{{{\rm{mem}}}}}}}^{2005\to }\) . However, they have only 10 ensemble members and they are initialized over the longer period 1980-2020. The latter enables comparison to a much larger set of observed values of \({\overline{{{{{{\rm{FFDI}}}}}}}}_{{{{{{\rm{Dec}}}}}}}\) and \(\overline{{{{{{\rm{DI}}}}}}}\) . In Fig. 4 d–f we compare the f \({}_{10\ {{{{{\rm{mem}}}}}}}^{1980\to }\) distributions over the period 1989–2020 (comprising 4480 samples) with observations over the same period (“Methods”). As for the f \({}_{96\ {{{{{\rm{mem}}}}}}}^{2005\to }\) data, the f \({}_{10\ {{{{{\rm{mem}}}}}}}^{1980\to }\) data distributions are stable and show good agreement with observations over the matched time period.
We use a two-dimensional, two-sample Kolmogorov–Smirnov (KS) test 54 , 55 to test the null hypothesis that the modeled and observed joint distributions (gray and white points in Fig. 4 ) are the same (“Methods”). Proxy time series are generated by randomly subsampling the model data for sets of equal length to the observed record. These sets are compared with the full model distribution to produce a null distribution for the KS-statistic, K . The KS-statistic calculated between the observed and modeled distributions, K obs , is compared with the null distribution. For both f \({}_{10\ {{{{{\rm{mem}}}}}}}^{1980\to }\) and f \({}_{96\ {{{{{\rm{mem}}}}}}}^{2005\to }\) , the observed KS-statistic falls below the 95 th percentile of the null distribution ( p -value > 0.05) and hence the model is considered to provide values of \({\overline{{{{{{\rm{FFDI}}}}}}}}_{{{{{{\rm{Dec}}}}}}}\) and \(\overline{{{{{{\rm{DI}}}}}}}\) that are consistent with the observed record (Fig. 4 g). The KS test also confirms consistent modeled and observed distributions when applied using model data at each lead time independently (Fig. 4 h), indicating that the independent model samples are both realistic and stable.
Likelihoods of exceedance
In 2019 unprecedented values of \({\overline{{{{{{\rm{FFDI}}}}}}}}_{{{{{{\rm{Dec}}}}}}}\) and \(\overline{{{{{{\rm{DI}}}}}}}\) were observed that were respectively 19% higher and 8% lower than previous record values (since 1958). These record values coincided with one of the worst wildfire seasons in recorded history 1 , 2 , 3 . Our model simulations show that the likelihoods of experiencing \({\overline{{{{{{\rm{FFDI}}}}}}}}_{{{{{{\rm{Dec}}}}}}}\) or \(\overline{{{{{{\rm{DI}}}}}}}\) values equal to or more extreme than those experienced in 2019 are 7.8% and 1.5%, respectively, in the current climate (2014-2023 comprising 13,440 samples, Fig. 5 a, b). The likelihood of exceeding both simultaneously is roughly 0.5%, indicating a return period for the 2019 event of approximately 200 years (Fig. 5 c). Note that for \(\overline{{{{{{\rm{DI}}}}}}}\) to be “more extreme” or to “exceed” is to have a lower value, since lower values of \(\overline{{{{{{\rm{DI}}}}}}}\) indicate drier conditions that are more conducive to wildfires.
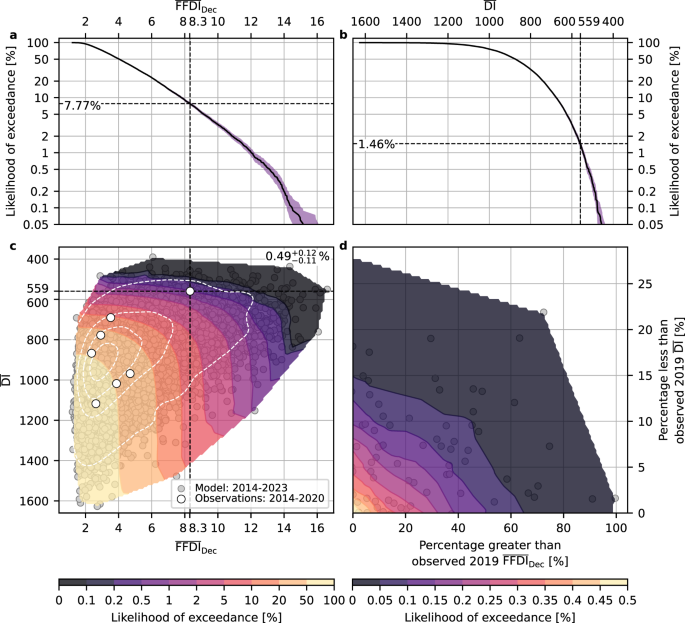
a Likelihoods of exceeding values of \({\overline{{{{{{\rm{FFDI}}}}}}}}_{{{{{{\rm{Dec}}}}}}}\) . The observed 2019 value of \({\overline{{{{{{\rm{FFDI}}}}}}}}_{{{{{{\rm{Dec}}}}}}}\) and the associated likelihood of exceeding this value are shown with dashed lines. b As in a , but for \(\overline{{{{{{\rm{DI}}}}}}}\) . c Likelihoods of simultaneously exceeding \({\overline{{{{{{\rm{FFDI}}}}}}}}_{{{{{{\rm{Dec}}}}}}}\) and \(\overline{{{{{{\rm{DI}}}}}}}\) . The observed 2019 values of \({\overline{{{{{{\rm{FFDI}}}}}}}}_{{{{{{\rm{Dec}}}}}}}\) and \(\overline{{{{{{\rm{DI}}}}}}}\) are shown with dashed black lines and the likelihood of exceeding both these values together is shown by the text in the upper right. Dashed white lines show contours of a two dimensional kernel density estimate using the forecast data (levels at 2e–05, 1.8e–04, 3.4e–04, and 5e–04, enclosing approximately 97%, 68%, 39%, and 13% of the data, respectively). d As in c but showing only values of \({\overline{{{{{{\rm{FFDI}}}}}}}}_{{{{{{\rm{Dec}}}}}}}\) and \(\overline{{{{{{\rm{DI}}}}}}}\) that are more extreme than the observed 2019 values. In all panels, likelihoods are calculated using model data over the period 2014-2023. Note that here “exceeding” a value of \(\overline{{{{{{\rm{DI}}}}}}}\) is defined as having a smaller magnitude since lower values of \(\overline{{{{{{\rm{DI}}}}}}}\) are indicative of increased susceptibility to fire.
Values of \({\overline{{{{{{\rm{FFDI}}}}}}}}_{{{{{{\rm{Dec}}}}}}}\) and \(\overline{{{{{{\rm{DI}}}}}}}\) substantially more extreme than observed records occur within the model sample (Fig. 5 d). This is especially true for \({\overline{{{{{{\rm{FFDI}}}}}}}}_{{{{{{\rm{Dec}}}}}}}\) , for which nearly 8% of all model samples are more extreme than the observed 2019 value, with some model realizations up to twice as high. Indeed, there are realizations from the model where \({\overline{{{{{{\rm{FFDI}}}}}}}}_{{{{{{\rm{Dec}}}}}}}\) and \(\overline{{{{{{\rm{DI}}}}}}}\) are simultaneously 60% higher and 20% lower, respectively, than in 2019, though such events are very unlikely (<0.05% chance, or >2000 year return period). What such extreme events would mean for the severity of wildfires in southeast Australia is an important question that requires further investigation.
Extreme susceptibility to fire and climate drivers
Climate and weather extremes prior to and during the fire season in southeast Australia are influenced by multiple drivers of climate variability, including the El Niño Southern Oscillation (ENSO), Indian Ocean Dipole (IOD), and Southern Annular Mode (SAM) 15 , 27 . The positive phase of ENSO (El Niño) is associated with warm and dry conditions across eastern Australia, generally leading up to and during the fire season (spring and summer) 56 . Similarly, positive IOD events reduce atmospheric moisture availability to the continent and are generally concomitant with drier conditions in southeast Australia 56 , 57 . Negative phases of the SAM in spring and summer are characterized by an equatorward shift of both the westerly storm track and the descending branch of the southern hemisphere Hadley Cell, resulting in dry westerly winds and warm conditions over eastern Australia 58 . These three climate drivers are not independent and the compounding effects of their co-occurrence can impact wildfire susceptibility 15 . Positive phases of ENSO and IOD tend to co-occur 59 , for example, as do the fire-promoting states of ENSO and SAM 60 , 61 .
Every sample from our model provides a simulated realization of the earth system, including the ocean and atmosphere. We can quantify for every modeled sample of \({\overline{{{{{{\rm{FFDI}}}}}}}}_{{{{{{\rm{Dec}}}}}}}\) and \(\overline{{{{{{\rm{DI}}}}}}}\) the corresponding states of ENSO, IOD and SAM over the period leading into the wildfire season using the Nino 3.4, DMI and SAM I indices (defined in “Methods” and assessed in Supplementary Figs. 2 and 3 ). The model provides 66 simulated Earths over the period 2014–2023 with values of \({\overline{{{{{{\rm{FFDI}}}}}}}}_{{{{{{\rm{Dec}}}}}}}\) and \(\overline{{{{{{\rm{DI}}}}}}}\) worse than the most extreme event in the observed record (2019). Of these samples, approximately 80% are associated with simultaneous positive ENSO, positive IOD and negative SAM states (Fig. 6 a). Composites of sea surface temperature and 500 hPa geopotential height anomalies generated from years of unprecedented \({\overline{{{{{{\rm{FFDI}}}}}}}}_{{{{{{\rm{Dec}}}}}}}\) and \(\overline{{{{{{\rm{DI}}}}}}}\) exhibit the typical patterns associated with these states (Fig. 6 b, c). While these results indicate a clear correspondence between extreme susceptibility to fire and the driver states, there is little evidence that the strength of the driver states is related to the joint magnitude of unprecedented \({\overline{{{{{{\rm{FFDI}}}}}}}}_{{{{{{\rm{Dec}}}}}}}\) and \(\overline{{{{{{\rm{DI}}}}}}}\) (as quantified by the normalized distance from the mean—see the shading of points in Fig. 6 a). That is, the severity of extreme wildfire susceptibility is apparently associated mostly with the concurrency of fire-conducive Nino 3.4, DMI, and SAM I states and not with their individual magnitudes, a characteristic of compound events previously described in frameworks for understanding extreme impacts 62 , 63 , 64 .
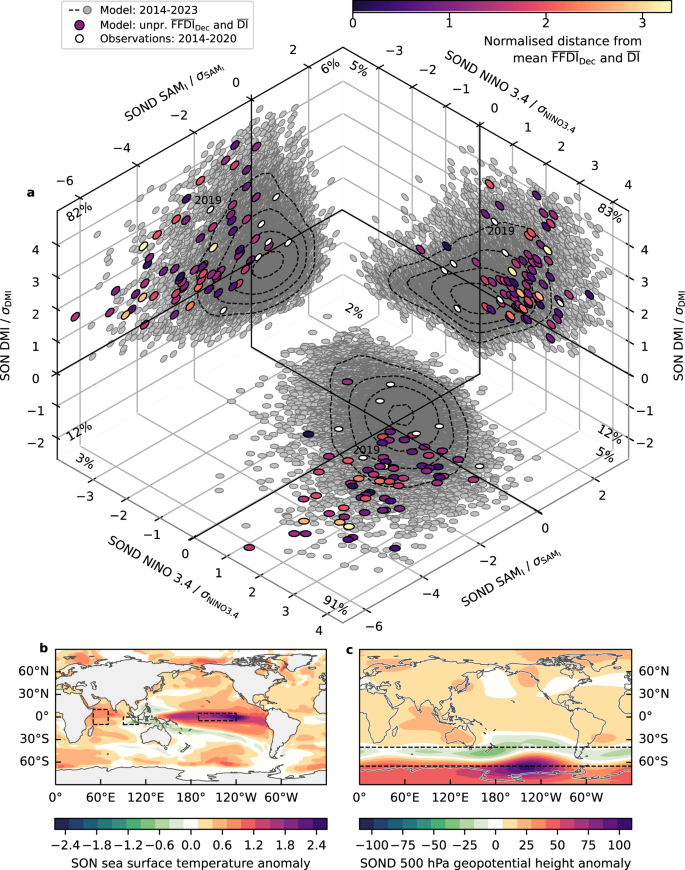
a Values of average Nino 3.4 over September–December (SOND), average DMI over September–November (SON) and average SAM I over SOND from the model data (gray dots, 2014–2023) and from observations (white dots, 2014–2020). Indices are normalized by their standard deviation, σ (calculated over 2014–2023 for the model data and over 1980-2020 for the observed data). Colored dots show the subset of model data points for which both \({\overline{{{{{{\rm{FFDI}}}}}}}}_{{{{{{\rm{Dec}}}}}}}\) and \(\overline{{{{{{\rm{DI}}}}}}}\) are unprecedented—i.e., values more extreme than the respective observed 2019 values—where the color indicates the normalized distance from the mean modeled \({\overline{{{{{{\rm{FFDI}}}}}}}}_{{{{{{\rm{Dec}}}}}}}\) and \(\overline{{{{{{\rm{DI}}}}}}}\) over 2014–2023. The text in each quadrant gives the percentage of colored points that fall within each quadrant. Where no text is given, the percentage is 0%. Dashed black lines show contours of two dimensional kernel-density estimates using the model forecast data with levels at 2e–2, 5e–2, 1e–1 and 2e–1. b Composite of average sea surface temperature anomalies over September–November from forecast years in the period 2014-2023 with unprecedented values of \({\overline{{{{{{\rm{FFDI}}}}}}}}_{{{{{{\rm{Dec}}}}}}}\) and \(\overline{{{{{{\rm{DI}}}}}}}\) . Dashed boxes show the regions used to calculate the Nino 3.4 and DMI indices. c As in b but showing the composite of average 500-hPa geopotential height anomalies over September–December. Dashed lines at 40 o S and 65 o S show the locations of the longitudinal averages used in the calculation of SAM I .
Conditioning on the states of ENSO, IOD, and SAM has a large impact on the likelihood of simultaneously experiencing unprecedented values of \({\overline{{{{{{\rm{FFDI}}}}}}}}_{{{{{{\rm{Dec}}}}}}}\) and \(\overline{{{{{{\rm{DI}}}}}}}\) (Fig. 7 ). The SAM appears to be the strongest driver; when SAM I is strongly negative (< −1 standard deviation) over spring the likelihood of an unprecedented event is over 5 times higher (nearly 3%) than if the state of the SAM is not considered. Likelihoods are higher still when conditioned on more than one of the drivers being strongly in their fire-conducive phases (up to approximately 4% for strongly positive Nino 3.4 and strongly negative SAM I ). In 2019, both the IOD and SAM were strongly in their fire-conducive phases (Fig. 6 a). According to the model, the likelihood under such conditions of the unprecedented values of \({\overline{{{{{{\rm{FFDI}}}}}}}}_{{{{{{\rm{Dec}}}}}}}\) and \(\overline{{{{{{\rm{DI}}}}}}}\) in 2019 was approximately 3%.
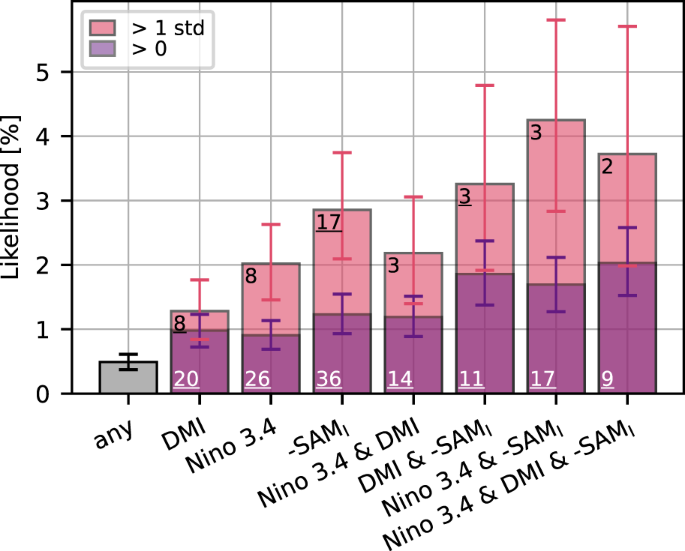
The likelihoods of simultaneously exceeding 2019 \({\overline{{{{{{\rm{FFDI}}}}}}}}_{{{{{{\rm{Dec}}}}}}}\) and \(\overline{{{{{{\rm{DI}}}}}}}\) conditions given that one or more of Nino 3.4, DMI and − SAM I are positive (purple shading) or strongly positive (>1 standard deviation, pink shading) over the period leading into the wildfire season (September, October, November, and December for Nino 3.4 and SAM I ; September, October, and November for DMI). Likelihoods and standard deviations are calculated using model data over the period 2014–2023 and error bars show 2.5–97.5% confidence bounds (“Methods”). Numbers show the number of years in the 63-year historical record (1958–2020) that satisfy each condition, where underlines indicate that 2019 is one such year.
We have used a very large ensemble of climate model simulations to quantify the likelihood of high susceptibility to wildfire from concurrent extreme fire-weather and drought in southeast Australia. Our analysis shows that the likelihood of experiencing the unprecedented conditions that occurred leading into and during the catastrophic 2019-2020 wildfire season was approximately 0.5% in the current climate. Substantially more extreme conditions are also realized by the model, and the impact of such conditions on the severity of wildfires in southeast Australia is a potential area for future research. A very high proportion (~80%) of the model realizations with more extreme fire susceptibility than that observed during 2019 occur when ENSO, IOD and SAM are all in their fire-promoting phases—positive, positive and negative, respectively—during the austral spring and early summer. Accounting for the observed phases and strengths of these climate modes, the likelihood of the fire-weather and drought conditions experienced in 2019 was approximately 3%.
ENSO and IOD are predictable on seasonal timescales, particularly during austral winter and spring when any event has already started to establish itself and persistence plays a first order role in predictability 65 , 66 . The neutral ENSO and positive IOD conditions experienced during spring (SON) of 2019, for example, were predicted by the Australian Bureau of Meteorology in June 67 and their implications on the fire season were foreshadowed in August 68 . SAM events are generally shorter lived and less predictable, although there is evidence that the significant stratospheric polar vortex weakening in spring 2019 and subsequent development of negative SAM was forecast as early as late July 58 . Thus the corresponding quantitative increases in likelihoods of extreme fire susceptibility demonstrated in this paper were potentially predictable months in advance of the peak in the 2019–2020 fire season.
The work in this paper builds on a growing area of research using climate simulations to assess and explore extreme events. There is enormous value to policy planners and decision makers in the ability to quantify the probability of impactful climate and weather events, particularly those that are unprecedented in the observed record. However, the use of climate models to quantify real-world risk is not without its difficulties. Foremost, the accuracy of estimates of probabilities is dependent entirely on the climate model’s ability to realistically represent the full range of plausible states that could be experienced in the real world. This is inherently very difficult to test because limited observed records provide very few samples of real world states and one is left trying to verify model states that have never been observed. We designed a statistical test to check for consistency between our modeled and observed indices and applied this in a way to maximize the number of observations in the test period. However, our test still suffers from small numbers of observations, particularly of extreme events. Thus, there is still some inherent reliance on the model’s ability to simulate the indices used.
Ideally, a climate model should be able to represent the real world without any correction, and indeed this can be the case for specific models simulating specific variables in specific regions 45 , 69 . But more generally, climate models have systematic biases that must be accounted for prior to their use. In this study we used relatively low resolution climate simulations because of the uniquely large number of realizations they provide of the current climate, enabling sufficient samples to empirically deduce likelihoods of rare concurrent extremes. Our model required minimal adjustment—only bias correction of the mean \(\overline{{{{{{\rm{DI}}}}}}}\) —to produce joint distributions of \({\overline{{{{{{\rm{FFDI}}}}}}}}_{{{{{{\rm{Dec}}}}}}}\) and \(\overline{{{{{{\rm{DI}}}}}}}\) that are statistically consistent with the real world over our region of focus. However, this is not necessarily the case for other indices and/or regions. Where more sophisticated calibrations are necessary, one must be careful not to overly constrain the model to observations, since this could negate the purpose of using the model in the first place (to provide plausible, yet unobserved, realizations of the earth system). Ongoing improvements to climate models and their resolutions will likely reduce model biases, allowing analyses like ours to be applied more broadly and with more confidence.
Our focus here has been on susceptibility to wildfire in the current climate (2014—2023) because of our focus on the 2019-2020 wildfire season. It is important to note, however, that occurrences of extreme drought and fire weather are expected to increase over the coming century due to anthropogenic influences. Projections indicate that winter and spring rainfall over Australia’s eastern seaboard will decrease 70 and that drought duration and frequency across southern Australia are likely to increase 71 . Increases in mean and extreme temperatures this century are virtually certain 70 and likely to contribute to increases in the number and severity of dangerous fire weather events 15 , 31 , 32 , 33 , 34 and drier, more volatile, fuel loads 72 . Indeed, some studies suggest that temperature may play an increasing role over precipitation in global fire occurrence over the next century 18 , 73 . Exactly how these changes will impact wildfire risk is a potential area for future research.
Burnt area data
To produce Fig. 1 , burnt forest areas are taken from FireCCI v5.1 provided by the European Space Agency Climate Change Initiative 74 (2001–2019) and from C3S v1.0 provided by the Copernicus Climate Change Service (2020). The data are gridded monthly burnt areas for different vegetation classes with a resolution of 0.25 o . Here we consider only burnt areas associated with land cover categories 50–90, corresponding to forested areas.
The burnt area data used in Fig. 2 are calculated from New South Wales National Parks and Wildlife Service Fire History data 75 . These data are provided as polygons of burnt areas of wildfires and prescribed burns, sometimes including associated start and/or end dates, over the period 01/01/1920–18/02/2021. The polygon data are converted to a gridded product with 0.05 o resolution in latitude and longitude. We consider only wildfire data and exclude data that have start or end dates that do not fall within 28 days of December or do not span a period encompassing December. The regional burnt areas used in Fig. 2 are calculated by summing the 0.05 o resolution data over the region shown in Fig. 1 .
Calculation of forest fire and drought indices
The daily McArthur Forest Fire Danger Index 76 , 77 , FFDI, is defined here as:
where T ( o C) is the maximum daily temperature; H (%) is the daily average relative humidity at 1000 hPa; W (km/h) is the daily average 10m wind speed; and D is the rolling 20-day total precipitation scaled to range between 0 and 10, with larger D for lower precipitation totals.
Note that this formulation differs from standard formulations of the FFDI 76 , 77 in a number of ways, principally in its use of daily average humidity and wind speed. These changes were necessitated by the data that were available to us across the various datasets used in this paper. FFDI estimates herein are likely to be attenuated relative to the standard formulations as a result of these differences. We calculate the FFDI from equation ( 1 ) from both the forecast model data (see “Use of forecast model data”) and the Japanese 55-year reanalysis (JRA-55) 78 , 79 which spans 1958–2020. For the latter, the 1.25 o resolution fields of the individual components in equation ( 1 ) are first interpolated linearly to the forecast model grid (the only exception to this is in Fig. 2 b, d, where the FFDI is presented at the JRA-55 grid resolution). For both the forecast and JRA-55 data, the regional December-averaged FFDI, \({\overline{{{{{{\rm{FFDI}}}}}}}}_{{{{{{\rm{Dec}}}}}}}\) , is calculated for a given year by averaging all daily December values of FFDI over the four model grid cells in Fig. 1 .
The Drought Index, DI, of a given year is defined as the accumulated total precipitation (mm) between January and December (both inclusive) of that year. Thus, lower values of DI indicate drier conditions. We calculate DI using the daily forecast data and using data from the Australian Gridded Climate Dataset (AGCD), which provides interpolated in situ observations on a 0.05 o × 0.05 o grid over the period 1900-2020 80 , 81 . AGCD v1 data are used for the period 1900–2018 and AGCD v2 data are used for the period 2019–2020. In Fig. 2 c, we show DI calculated from AGCD at the native grid resolution. We also spatially average DI calculated from AGCD to the JRA-55 grid resolution in order to generate the combined index presented in Fig. 2 d. Everywhere else, we focus in this paper on the regional Drought Index, \(\overline{{{{{{\rm{DI}}}}}}}\) , which is defined for each dataset as the average DI over the region in Fig. 1 .
The JRA-55 and AGCD data provide joint historical records of \({\overline{{{{{{\rm{FFDI}}}}}}}}_{{{{{{\rm{Dec}}}}}}}\) and \(\overline{{{{{{\rm{DI}}}}}}}\) spanning 1958–2020 and are referred to herein as “observations”. By pooling forecast ensemble members and lead times, the forecast model provides many estimates of plausible values of \({\overline{{{{{{\rm{FFDI}}}}}}}}_{{{{{{\rm{Dec}}}}}}}\) and \(\overline{{{{{{\rm{DI}}}}}}}\) for every available forecast year.
Use of forecast model data
We use the Commonwealth Science and Industrial Research Organization (CSIRO) Climate Analysis Forecast Ensemble (CAFE) near-term climate prediction system to produce many simulations of contemporary climate. The system uses the Geophysical Fluid Dynamics Laboratory Coupled Model version 2.1 82 , with an upgraded oceanic component (MOM5.1) and an atmospheric model resolution of 2 o in latitude and 2. 5 o in longitude 83 . Retrospective forecasts were run from initial conditions taken from the CAFE60v1 reanalysis 84 , 85 , which provides an ensemble of estimates of the states of the atmosphere, ocean, land and sea-ice over the period 1960–2020 using the same underlying model as the forecasts.
The retrospective climate forecasts provide a very large sample of possible values of \({\overline{{{{{{\rm{FFDI}}}}}}}}_{{{{{{\rm{Dec}}}}}}}\) and \(\overline{{{{{{\rm{DI}}}}}}}\) under contemporaneous anthropogenic and natural forcings that enable the likelihoods of exceeding rare events (like those in 2019) to be estimated empirically. Our methodology is similar to those introduced in previous papers using the UNSEEN approach 46 :
Remove model samples at short lead times where there is dependence between forecast ensemble members due to their similar initial conditions (see “Testing of ensemble member independence”). Dependence between samples artificially inflates the sample size without adding new information.
Test that the model provides stable (with lead time) and realistic estimates of \({\overline{{{{{{\rm{FFDI}}}}}}}}_{{{{{{\rm{Dec}}}}}}}\) and \(\overline{{{{{{\rm{DI}}}}}}}\) . We apply a simple bias correction to the modeled \(\overline{{{{{{\rm{DI}}}}}}}\) (see “Bias correction”) and check that the joint distributions of modeled \({\overline{{{{{{\rm{FFDI}}}}}}}}_{{{{{{\rm{Dec}}}}}}}\) and \(\overline{{{{{{\rm{DI}}}}}}}\) are consistent with the observed record (see “Testing of model fidelity”).
Calculate likelihoods of exceedance (see “Calculation of likelihoods of exceedance”).
Two sets of forecasts are used in this paper, denoted f \({}_{10\ {{{{{\rm{mem}}}}}}}^{1980\to }\) and f \({}_{96\ {{{{{\rm{mem}}}}}}}^{2005\to }\) . The f \({}_{10\ {{{{{\rm{mem}}}}}}}^{1980\to }\) dataset comprises 10-year long forecasts, each with 10 ensemble members, initialised at the beginning of every May and November over the period 1980–2020. The f \({}_{96\ {{{{{\rm{mem}}}}}}}^{2005\to }\) dataset is identical to f \({}_{10\ {{{{{\rm{mem}}}}}}}^{1980\to }\) , except that the initialization period is shorter—2005–2020—and the number of ensemble members per forecast is much larger—96 members. In this paper, f \({}_{10\ {{{{{\rm{mem}}}}}}}^{1980\to }\) is used only to bias-correct f \({}_{96\ {{{{{\rm{mem}}}}}}}^{2005\to }\) and to demonstrate the efficacy of the forecast model. The large-ensemble f \({}_{96\ {{{{{\rm{mem}}}}}}}^{2005\to }\) data are used for all other analyses.
When using forecast data for the purpose of providing multiple realizations of a given time period, it is important that equal numbers of samples are included for each year in the period, since this avoids over/under-sampling the conditions of a particular year. Figure 8 shows the number of samples per calendar year for the f \({}_{10\ {{{{{\rm{mem}}}}}}}^{1980\to }\) (pink labels) and f \({}_{96\ {{{{{\rm{mem}}}}}}}^{2005\to }\) (purple labels) datasets after removing lead times with dependent ensemble members. Fewer samples are available for calendar forecast years toward the start and end of each forecast period because these years have fewer lead times available. For both \({\overline{{{{{{\rm{FFDI}}}}}}}}_{{{{{{\rm{Dec}}}}}}}\) and \(\overline{{{{{{\rm{DI}}}}}}}\) , we define the lead time of a given forecast as the number of elapsed months between initialization and December of the forecast year (e.g., the 2020 forecast initialised in Nov 2020 is at 1-month lead). Note that no \(\overline{{{{{{\rm{DI}}}}}}}\) forecast is available at 1-month lead, since this index requires the accumulation of rainfall from January to December. Thus, the shortest available lead time for \(\overline{{{{{{\rm{DI}}}}}}}\) is 13 months.
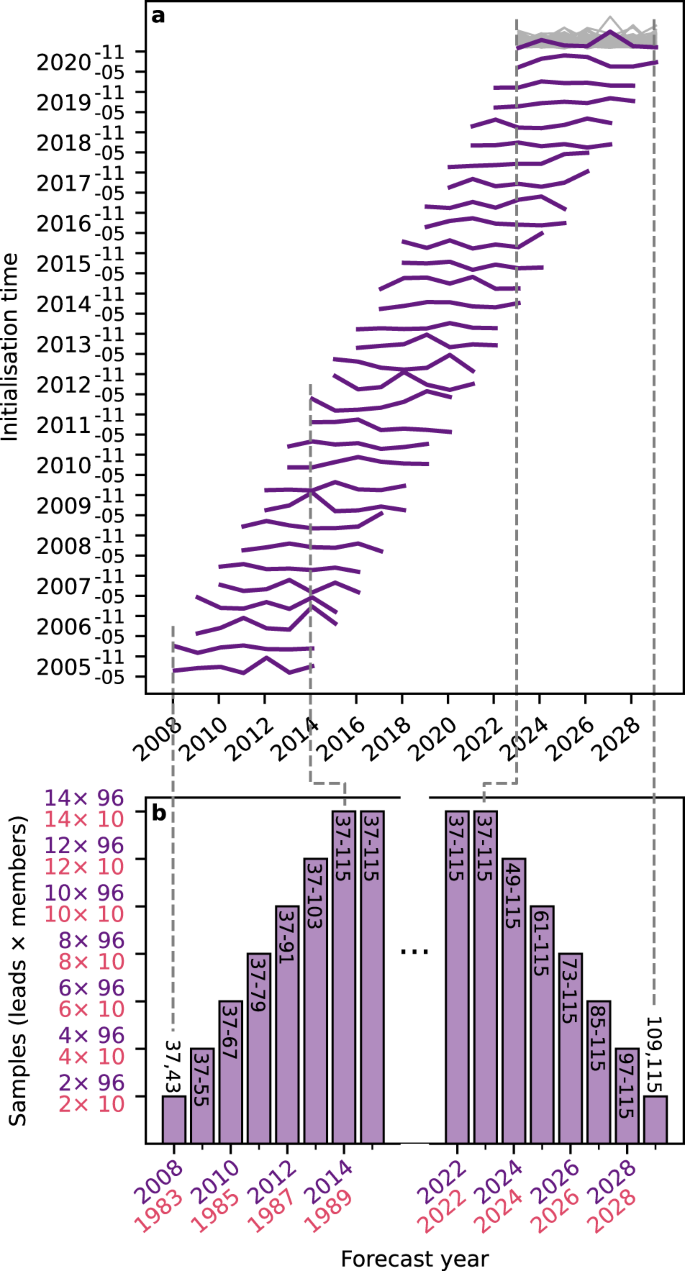
a The initialization and forecast periods for the f \({}_{96\ {{{{{\rm{mem}}}}}}}^{2005\to }\) forecasts and b the resulting number of samples per year after removing lead times with dependent ensemble members (see “Testing of ensemble member independence”). In a , each purple line represents a single ensemble member and the forecast in the top right shows all 96 ensemble members in gray. The dashed lines show, for specific years, why different forecast years have different numbers of samples. In b , purple (pink) labels apply to the f \({}_{96\ {{{{{\rm{mem}}}}}}}^{2005\to }\) (f \({}_{10\ {{{{{\rm{mem}}}}}}}^{1980\to }\) ) dataset and the numbers in or above each bar show the lead times that are available for each year (defined as the number of elapsed months between initialization and December of the forecast year). Where a range is specified, lead times are available in increments of 6 months.
The f \({}_{10\ {{{{{\rm{mem}}}}}}}^{1980\to }\) dataset provides 140 simulations (14 × 10: lead times × ensemble members) for every year over the period 1989–2023. Likewise, the f \({}_{96\ {{{{{\rm{mem}}}}}}}^{2005\to }\) dataset provides 1344 simulations (14 × 96) for every year in 2014–2023. Thus, when testing the fidelity of the f \({}_{10\ {{{{{\rm{mem}}}}}}}^{1980\to }\) and f \({}_{96\ {{{{{\rm{mem}}}}}}}^{2005\to }\) forecasts in Fig. 4 g we use the periods 1989–2020 and 2014–2020, respectively, since these periods provide maximum and equal numbers of realizations per year and overlap with the observed record. To calculate the likelihoods of the 2019 \({\overline{{{{{{\rm{FFDI}}}}}}}}_{{{{{{\rm{Dec}}}}}}}\) and \(\overline{{{{{{\rm{DI}}}}}}}\) conditions using the f \({}_{96\ {{{{{\rm{mem}}}}}}}^{2005\to }\) data, we consider all lead times together and use the 10-year period centered on 2019 (2014–2023) as representative of current climate conditions.
Testing of ensemble member independence
Each multi-member forecast is initialised from a set of initial conditions that seek to estimate the state of the climate at the time of initialization and the uncertainty about that state. As such, ensemble members of a given forecast at short lead times are strongly dependent on each other. Inclusion of dependent ensemble members in our analysis results in artificial inflation of the sample size, without adding new information 39 , 40 , 41 , 46 . To determine the lead time at which the ensemble members can be considered independent, we apply a simple statistical test that the correlation between ensemble members at a given lead time is zero.
At each lead time, the f \({}_{96\ {{{{{\rm{mem}}}}}}}^{2005\to }\) model dataset provides 96 (members), 16-year timeseries of \({\overline{{{{{{\rm{FFDI}}}}}}}}_{{{{{{\rm{Dec}}}}}}}\) and \(\overline{{{{{{\rm{DI}}}}}}}\) (spanning, e.g., 2005–2020 at 1 month lead, or 2014–2029 at 115 months lead, see Fig. 8 ). We define our test statistic, ρ t , for each lead time and variable as the mean Spearman correlation 86 in time between all combinations of the 96 ensemble members (of which there are 4560: member 1 with 2, member 1 with 3 etc). Significance of ρ t is estimated using a permutation test, whereby 10,000 sets of 96 × 16 points are randomly drawn from the complete \({\mathrm f}_{96\ {{{{{\rm{mem}}}}}}}^{2005\to }\) model dataset to produce 10,000 estimates of the mean Spearman correlation for each variable in the same manner as above. Because these estimates are constructed from randomly drawn data, they represent the distribution of mean correlation values for uncorrelated data (i.e., the null distribution). Ensemble members of each variable are considered to be dependent (i.e., the null hypothesis of independence is rejected) at a given lead time if ρ t falls outside of confidence intervals calculated from the randomly sampled distribution using a 5% significance level (Fig. 3 ). The test is very similar to that described in previous studies 46 , however here we test only the mean correlation over combinations of ensemble members rather than all box-and-whisker statistics. Our approach reduces the number of simultaneous tests and the associated issues with multiple testing 87 .
Note that nonzero correlation between ensemble members at a given lead time can also come about because they share the same time-varying forcing. However, correlation from shared forcing is not a valid reason to reject ensemble members. Applying the same test as described above, but removing the ensemble mean temporal trend from each ensemble member prior to calculating ρ t produces negligible changes to Fig. 3 .
Bias correction
All climate models have systematic biases relative to the real world. There is a very large range of existing methods, of varying levels of complexity, for correcting for climate model biases 88 , 89 , 90 . Generally, these methods involve building a transfer function between the distributions of observed and modeled variables over a particular period of time. All such methods include potentially ad hoc assumptions regarding, for example, the shape and stationarity of the observed/modeled distributions. In the present analysis, we seek to use our forecast model to learn about events that are unprecedented in the historical record and therefore have no observations to constrain their correction. Our approach to model correction is to find the simplest justifiable method that produces model distributions that are statistically consistent with the limited historical record. In doing so, we minimize the extent to which the forecast model data are manipulated, and thus rely as much as possible on the ability of the model to simulate the range of contemporaneous climate conditions.
It is necessary to bias-correct the forecast \(\overline{{{{{{\rm{DI}}}}}}}\) to ensure that the simulated joint distribution of \({\overline{{{{{{\rm{FFDI}}}}}}}}_{{{{{{\rm{Dec}}}}}}}\) and \(\overline{{{{{{\rm{DI}}}}}}}\) is consistent with the real world. For each forecast lead time, we estimate the mean \(\overline{{{{{{\rm{DI}}}}}}}\) bias as the difference between the mean f \({}_{10\ {{{{{\rm{mem}}}}}}}^{1980\to }\) and observed \(\overline{{{{{{\rm{DI}}}}}}}\) over the period 1990–2020. These biases (which range between −141 mm and −68 mm, depending on the lead time) are subtracted from both the f \({}_{10\ {{{{{\rm{mem}}}}}}}^{1980\to }\) and f \({}_{96\ {{{{{\rm{mem}}}}}}}^{2005\to }\) forecasts to produce unbiased estimates of \(\overline{{{{{{\rm{DI}}}}}}}\) . No bias correction is necessary for \({\overline{{{{{{\rm{FFDI}}}}}}}}_{{{{{{\rm{Dec}}}}}}}\) . Note that the f \({}_{10\ {{{{{\rm{mem}}}}}}}^{1980\to }\) model dataset is used here so that the biases can be estimated using a relatively long time period (31 years). The f \({}_{96\ {{{{{\rm{mem}}}}}}}^{2005\to }\) model dataset is initialised over a shorter period (2005–2020), so provides, for example, only seven years of data at 115 months lead (2014–2020) that could be compared with observations to estimate biases (see Fig. 8 ).
Testing of model fidelity
We test the ability of our forecast model to simulate the real world by comparing the forecast and observed distributions of \({\overline{{{{{{\rm{FFDI}}}}}}}}_{{{{{{\rm{Dec}}}}}}}\) and \(\overline{{{{{{\rm{DI}}}}}}}\) over a common period of time. Previous studies assessing likelihoods of extremes using forecast ensembles have tested that the observed mean, standard deviation, skewness and kurtosis of the variable in question falls within 95% confidence intervals from bootstrapped distributions of each statistic computed from the forecast model 44 , 45 , 46 , 47 , 48 , 69 . We apply a different test for two reasons. First, our focus in this paper is on compound events and thus we seek to assess the fidelity of our model in simulating the joint distributions of \({\overline{{{{{{\rm{FFDI}}}}}}}}_{{{{{{\rm{Dec}}}}}}}\) and \(\overline{{{{{{\rm{DI}}}}}}}\) . Second, because the approach of previous studies simultaneously tests multiple statistics, each with their own statistical significance, it suffers from issues with multiple testing 87 . Indeed, Monte–Carlo simulations applying the above test to samples and bootstrapped distributions drawn from the same Gaussian population show that the rejection rate is approximately 18% (not 5%), with little dependence on sample size. For two variables, the rejection rate is higher still.
For these reasons, we instead apply a two-dimensional Kolmogorov–Smirnov (KS) test 54 , 55 to compare the joint distributions of \({\overline{{{{{{\rm{FFDI}}}}}}}}_{{{{{{\rm{Dec}}}}}}}\) and \(\overline{{{{{{\rm{DI}}}}}}}\) . The f \({}_{96\ {{{{{\rm{mem}}}}}}}^{2005\to }\) model dataset provides 96 (member) forecasts for the 7-year period 2014–2020 at all independent lead times (see Fig. 8 ). We calculate the two-dimensional KS statistic between the observed and forecast distributions, K obs , using all data in this period. To derive a p -value for this statistic, we bootstrap 10,000 7-year pseudo-timeseries of \({\overline{{{{{{\rm{FFDI}}}}}}}}_{{{{{{\rm{Dec}}}}}}}\) and \(\overline{{{{{{\rm{DI}}}}}}}\) from all forecasts that fall within the same period. For each bootstrapped sample, we calculate the two-dimensional KS statistic, K , relative to the full set of forecasts within the period, thus providing the null distribution for our KS test. If K obs falls below the 95 th percentile of the null distribution—i.e., the right-tail p -value is greater than 0.05—we cannot reject the null hypothesis that the joint distributions are the same. In this case, we consider that our forecast model provides a good representation of plausible values of \({\overline{{{{{{\rm{FFDI}}}}}}}}_{{{{{{\rm{Dec}}}}}}}\) and \(\overline{{{{{{\rm{DI}}}}}}}\) .
The results of the two-dimensional KS test are shown for the bias-corrected f \({}_{96\ {{{{{\rm{mem}}}}}}}^{2005\to }\) model data in Fig. 4 (pink shading). We run the test for all lead times together (Fig. 4 g) and for each lead month separately (Fig. 4 h). In the latter case, the period of time over which the test is applied is adjusted to maximize the number of observed points in the comparison. For example, f \({}_{96\ {{{{{\rm{mem}}}}}}}^{2005\to }\) forecasts at 37-months lead span 2008–2020 (see Fig. 8 ) and thus the test at 37 months lead is applied over this period. We also apply the same KS test to the bias-corrected f \({}_{10\ {{{{{\rm{mem}}}}}}}^{1980\to }\) data, which span a longer period of time and hence allow for comparison to a larger sample of observations (Fig. 4 g and h, purple shading). For the f \({}_{10\ {{{{{\rm{mem}}}}}}}^{1980\to }\) data, all KS tests are applied over the period 1989–2020.
Calculation of likelihoods of exceedance
Likelihoods of exceeding a given event are calculated from the empirical probability distribution as the proportion of total f \({}_{96\ {{{{{\rm{mem}}}}}}}^{2005\to }\) forecast samples that are more extreme than the event in question. For example, Fig. 5 a, b, respectively, show probabilities P ( \({\overline{{{{{{\rm{FFDI}}}}}}}}_{{{{{{\rm{Dec}}}}}}}\) > \({\overline{{{{{{\rm{FFDI}}}}}}}}_{{{{{{\rm{Dec}}}}}}}\) , i ) and P ( \(\overline{{{{{{\rm{DI}}}}}}}\) < \(\overline{{{{{{\rm{DI}}}}}}}\) i ) for every sample i of the 13,440 samples in the f \({}_{96\ {{{{{\rm{mem}}}}}}}^{2005\to }\) dataset over 2014-2023, and Fig. 5 c similarly show P ( \({\overline{{{{{{\rm{FFDI}}}}}}}}_{{{{{{\rm{Dec}}}}}}}\) > \({\overline{{{{{{\rm{FFDI}}}}}}}}_{{{{{{\rm{Dec}}}}}}}\) , i ∧ \(\overline{{{{{{\rm{DI}}}}}}}\) < \(\overline{{{{{{\rm{DI}}}}}}}\) i ). In the calculation of likelihoods of exceedance, we limit ourselves to the 10 year period, 2014-2023, since all independent lead times are available from the model for these years (Fig. 8 ).
Likelihood confidence bounds in Figs. 5 and 7 are constructed by repeatedly bootstrapping the set of \({\overline{{{{{{\rm{FFDI}}}}}}}}_{{{{{{\rm{Dec}}}}}}}\) and \(\overline{{{{{{\rm{DI}}}}}}}\) values used to calculate the likelihood in question and recomputing the likelihood for each boostrapped sample to produce 10,000 resampled estimates of the likelihoods of exceedance. These resampled likelihoods are used to calculate the 2.5–97.5% percentile ranges shown in the figures. In Fig. 5 c, d, the likelihoods of exceedance are interpolated onto a regular grid using the ‘griddata’ routine in the Python Scipy library.
Calculation of climate driver indices
We employ three simple indices for climate modes that impact Australia. To assess the strength and phase of the El Niño Southern Oscillation (ENSO), we use the Nino 3.4 index 91 , which is the average sea-surface temperature (SST) anomaly over the region 5 o N–5 o S, 120 o –170 o W. The Indian Ocean Dipole is quantified using the Dipole Mode Index, DMI 92 , which is the difference between the average SST anomalies over western (10 o N–10 o S, 50 o –70 o E) and south-eastern (0 o –10 o S, 90 o –110 o E) tropical Indian Ocean regions. We represent the strength of the Southern Annual Mode (SAM, also called the Antarctic Oscillation) using a Southern Annular Mode Index, SAM I , defined as the difference between the normalized monthly zonal mean sea level pressure at 40 o S and 65 o S 93 .
The climate mode indices are computed from the forecast model data and from reanalysis data: Hadley Centre Global Sea Ice and Sea Surface Temperature (HadISST1) 94 for Nino 3.4 and DMI; and JRA-55 for SAM I . Anomalies are computed relative to the climatological average over the period 1990–2020. In cases where forecast model data are used, a separate climatological average is constructed using the f \({}_{10\ {{{{{\rm{mem}}}}}}}^{1980\to }\) dataset and removed for each forecast lead time. This removes from the forecasts the mean model biases over the reference period at each lead time. We focus on the average Nino 3.4 and SAM I over September, October, November and December (SOND), and on the average DMI over September, October and November (SON), corresponding to when each index has its strongest influence on precipitation and FFDI in southeast Australia 15 . The fidelity of the model climate driver indices relative to observations and their relationships to \({\overline{{{{{{\rm{FFDI}}}}}}}}_{{{{{{\rm{Dec}}}}}}}\) and \(\overline{{{{{{\rm{DI}}}}}}}\) are assessed in Supplementary Figs. 2 and 3 .
Data availability
FireCCI v5.1 and C3S v1.0 fire burned area data are openly available from the Copernicus Climate Data Store at https://doi.org/10.24381/cds.f333cf85 . New South Wales National Parks and Wildlife Service Fire History data are available for download at https://data.nsw.gov.au/data/dataset/fire-history-wildfires-and-prescribed-burns-1e8b6 . JRA-55 data are available from the University Corporation for Atmospheric Research Research Data Archive at https://doi.org/10.5065/D6HH6H41 . HadISST1 data are available from the Met Office Hadley Centre at https://hadleyserver.metoffice.gov.uk/hadisst/data/download.html . AGCD v1 data are accessible from Australia’s National Computational Infrastructure Data Catalogue at https://doi.org/10.25914/6009600b58196 . AGCD v2 data are not publicly available, with access details provided by the Bureau of Meteorology at http://www.bom.gov.au/climate/austmaps/metadata-monthly-rainfall.shtml . The Natural Earth data used to generate the shading in the inset of Fig. 1 are in the public domain and are available at https://www.naturalearthdata.com/ . The forecast datasets are not yet available publicly but are available from the corresponding author upon request, bearing in mind that these datasets comprise hundreds of terabytes of data. Postprocessed versions of all the variables and indices presented in this paper are available from the corresponding author upon request.
Code availability
All code used to perform the analysis and generate the figures in this paper is openly available at https://doi.org/10.5281/zenodo.5566244 .
The Royal Commission into National Natural Disaster Arrangements: Report. Technical Report (The Royal Commission into National Natural Disaster Arrangements, 2020). https://naturaldisaster.royalcommission.gov.au/publications/royal-commission-national-natural-disaster-arrangements-report .
Boer, M. M., Resco de Dios, V. & Bradstock, R. A. Unprecedented burn area of Australian mega forest fires. Nat. Clim. Change 10 , 171–172 (2020).
Article Google Scholar
Hughes, L. et al. Summer of Crisis. Technical Report (The Climate Council of Australia, 2020). https://www.climatecouncil.org.au/resources/summer-of-crisis/ .
Davey, S. M. & Sarre, A. Editorial: the 2019/20 Black Summer bushfires. Aust. For. 83 , 47–51 (2020).
Borchers Arriagada, N. et al. Unprecedented smoke-related health burden associated with the 2019–20 bushfires in eastern Australia. Med. J. Aust. 213 , 282–283 (2020).
Vardoulakis, S., Jalaludin, B. B., Morgan, G. G., Hanigan, I. C. & Johnston, F. H. Bushfire smoke: urgent need for a national health protection strategy. Med. J. Aust. 212 , 349–353.e1 (2020).
Pickrell, J. As fires rage across Australia, fears grow for rare species. Science . https://www.sciencemag.org/news/2019/12/fires-rage-across-australia-fears-grow-rare-species . (2019).
Ward, M. et al. Impact of 2019–2020 mega-fires on Australian fauna habitat. Nat. Ecol. Evol. 4 , 1321–1326 (2020).
Read, P. & Denniss, R. With costs approaching $100 billion, the fires are Australia’s costliest natural disaster (2020). http://theconversation.com/with-costs-approaching-100-billion-the-fires-are-australias-costliest-natural-disaster-129433 .
Quiggin, J. Australia is promising $2 billion for the fires. I estimate recovery will cost $100 billion https://www.cnn.com/2020/01/10/perspectives/australia-fires-cost/index.html (2020).
Nolan, R. H. et al. Causes and consequences of eastern Australia’s 2019–20 season of mega-fires. Glob. Chang. Biol. 26 , 1039–1041 (2020).
Adams, M. A., Shadmanroodposhti, M. & Neumann, M. Causes and consequences of Eastern Australia’s 2019–20 season of mega-fires: a broader perspective. Glob. Chang. Biol. 26 , 3756–3758 (2020).
Bradstock, R. A. et al. A broader perspective on the causes and consequences of eastern Australia’s 2019–20 season of mega-fires: a response to Adams et al. Glob. Chang. Biol. 26 , e8–e9 (2020).
King, A. D., Pitman, A. J., Henley, B. J., Ukkola, A. M. & Brown, J. R. The role of climate variability in Australian drought. Nat. Clim. Change 10 , 177–179 (2020).
Abram, N. J. et al. Connections of climate change and variability to large and extreme forest fires in southeast Australia. Commun. Earth Environ. 2 , 1–17 (2021).
Wang, G. & Cai, W. Two-year consecutive concurrences of positive Indian Ocean Dipole and Central Pacific El Niño preconditioned the 2019/2020 Australian “black summer” bushfires. Geosci. Lett. 7 , 19 (2020).
Doi, T., Behera, S. K. & Yamagata, T. Predictability of the Super IOD Event in 2019 and Its Link With El Niño Modoki. Geophys. Res. Lett. 47 , e2019GL086713 (2020).
Sanderson, B. M. & Fisher, R. A. A fiery wake-up call for climate science. Nat. Clim. Change 10 , 175–177 (2020).
Special Climate Statement 72—dangerous bushfire weather in spring 2019. Technical Report (Australian Bureau of Meteorology, 2020). http://www.bom.gov.au/climate/current/statements/scs72.pdf .
Deb, P. et al. Causes of the widespread 2019–2020 Australian bushfire season. Earths Future 8 , e2020EF001671 (2020).
No authors listed. Biodiversity in flames. Nat. Ecol. Evol. 4 , 171–171 (2020).
Lim, E.-P. et al. Australian hot and dry extremes induced by weakenings of the stratospheric polar vortex. Nat. Geosci. 12 , 896–901 (2019).
Grothe, P. R. et al. Enhanced El Niño–southern oscillation variability in recent decades. Geophys. Res. Lett. 47 , e2019GL083906 (2020).
Abram, N. J. et al. Palaeoclimate perspectives on the Indian Ocean dipole. Quat. Sci. Rev. 237 , 106302 (2020).
Cai, W. et al. Increasing frequency of extreme El Niño events due to greenhouse warming. Nat. Clim. Change 4 , 111–116 (2014).
Cai, W. et al. Increased frequency of extreme Indian Ocean dipole events due to greenhouse warming. Nature 510 , 254–258 (2014).
Harris, S. & Lucas, C. Understanding the variability of Australian fire weather between 1973 and 2017. PLoS ONE 14 , e0222328 (2019).
Dowdy, A. J. Climatological variability of fire weather in Australia. J. Appl. Meteorol. Climatol. 57 , 221–234 (2018).
Clarke, H., Lucas, C. & Smith, P. Changes in Australian fire weather between 1973 and 2010. Int. J. Climatol. 33 , 931–944 (2013).
Sharples, J. J. et al. Natural hazards in Australia: extreme bushfire. Clim. Change 139 , 85–99 (2016).
Pitman, A. J., Narisma, G. T. & McAneney, J. The impact of climate change on the risk of forest and grassland fires in Australia. Clim. Change 84 , 383–401 (2007).
Clarke, H. et al. An investigation of future fuel load and fire weather in Australia. Clim. Change 139 , 591–605 (2016).
Clarke, H. & Evans, J. P. Exploring the future change space for fire weather in southeast Australia. Theor. Appl. Climatol. 136 , 513–527 (2019).
Touma, D., Stevenson, S., Lehner, F. & Coats, S. Human-driven greenhouse gas and aerosol emissions cause distinct regional impacts on extreme fire weather. Nat. Commun. 12 , 212 (2021).
Bradstock, R. A. et al. Prediction of the probability of large fires in the Sydney region of south-eastern Australia using fire weather. Int. J. Wildland Fire 18 , 932–943 (2010).
Louis, S. A. Gridded return values of McArthur Forest Fire Danger Index across New South Wales. Aust. Meteorol. Oceanogr. J. 64 , 243–260 (2014).
Brink, H. W. V. D., Können, G. P., Opsteegh, J. D., Oldenborgh, G. J. V. & Burgers, G. Improving 104-year surge level estimates using data of the ECMWF seasonal prediction system. Geophys. Res. Lett. 31 , L17210 (2004).
Google Scholar
Brink, H. W. V. D., Können, G. P., Opsteegh, J. D., Oldenborgh, G. J. V. & Burgers, G. Estimating return periods of extreme events from ECMWF seasonal forecast ensembles. Int. J. Climatol. 25 , 1345–1354 (2005).
Breivik, Ø., Aarnes, O. J., Bidlot, J.-R., Carrasco, A. & Saetra, Ø. Wave extremes in the Northeast Atlantic from ensemble forecasts. J. Clim. 26 , 7525–7540 (2013).
Breivik, Ø., Aarnes, O. J., Abdalla, S., Bidlot, J.-R. & Janssen, P. A. E. M. Wind and wave extremes over the world oceans from very large ensembles. Geophys. Res. Lett. 41 , 5122–5131 (2014).
Meucci, A., Young, I. R. & Breivik, Ø. Wind and wave extremes from atmosphere and wave model ensembles. J. Clim. 31 , 8819–8842 (2018).
Walz, M. A. & Leckebusch, G. C. Loss potentials based on an ensemble forecast: How likely are winter windstorm losses similar to 1990? Atmos. Sci. Lett. 20 , e891 (2019).
Kent, C. et al. Using climate model simulations to assess the current climate risk to maize production. Environ. Res. Lett. 12 , 054012 (2017).
Kent, C. et al. Maize drought hazard in the Northeast Farming Region of China: unprecedented events in the current climate. J. Appl. Meteorol. Climatol. 58 , 2247–2258 (2019).
Thompson, V. et al. High risk of unprecedented UK rainfall in the current climate. Nat. Commun. 8 , 107 (2017).
Kelder, T. et al. Using UNSEEN trends to detect decadal changes in 100-year precipitation extremes. npj Clim. Atmos. Sci. 3 , 1–13 (2020).
Kay, G. et al. Current likelihood and dynamics of hot summers in the UK. Environ. Res. Lett. 15 , 094099 (2020).
Wang, L. et al. What chance of a sudden stratospheric warming in the southern hemisphere? Environ. Res. Lett. 15 , 104038 (2020).
Tancredi, A., Anderson, C. & O’Hagan, A. Accounting for threshold uncertainty in extreme value estimation. Extremes 9 , 87 (2006).
Scarrott, C. & MacDonald, A. A review of extreme value threshold estimation and uncertainty quantification. REVSTAT Stat. J. 10 , 33–60 (2012).
Chowdhary, H. & Singh, V. P. Reducing uncertainty in estimates of frequency distribution parameters using composite likelihood approach and copula-based bivariate distributions. Water Resour. Res. 46 , W11516 (2010).
Altman, N. & Krzywinski, M. The curse(s) of dimensionality. Nat. Meth. 15 , 399–400 (2018).
Folland, C. & Anderson, C. Estimating changing extremes using empirical ranking methods. J. Clim. 15 , 2954–2960 (2002).
Fasano, G. & Franceschini, A. A multidimensional version of the Kolmogorov–Smirnov test. Mon. Not. R. Astron. Soc. 225 , 155–170 (1987).
Press, W. H. & Teukolsky, S. A. Kolmogorov-Smirnov test for two-dimensional data. Comput. Phys. 2 , 74–77 (1988).
Risbey, J. S., Pook, M. J., McIntosh, P. C., Wheeler, M. C. & Hendon, H. H. On the remote drivers of rainfall variability in Australia. Mon. Wea. Rev. 137 , 3233–3253 (2009).
Ummenhofer, C. C. et al. What causes southeast Australia’s worst droughts? Geophys. Res. Lett. 36 , L04706 (2009).
Lim, E.-P. et al. The 2019 Southern Hemisphere stratospheric polar vortex weakening and its impacts. Bull. Am. Meteorol. Soc. 1 , 1–50 (2021).
Cai, W. et al. Pantropical climate interactions. Science 363 , eaav4236 (2019).
Abram, N. J. et al. Evolution of the Southern Annular Mode during the past millennium. Nat. Clim. Change 4 , 564–569 (2014).
Dätwyler, C., Grosjean, M., Steiger, N. J. & Neukom, R. Teleconnections and relationship between the El Niño–Southern Oscillation (ENSO) and the Southern Annular Mode (SAM) in reconstructions and models over the past millennium. Clim. Past 16 , 743–756 (2020).
Leonard, M. et al. A compound event framework for understanding extreme impacts. WIREs Clim. Change 5 , 113–128 (2014).
Sadegh, M. et al. Multihazard scenarios for analysis of compound extreme events. Geophys. Res. Lett. 45 , 5470–5480 (2018).
Zscheischler, J. et al. A typology of compound weather and climate events. Nat. Rev. Earth Environ. 1 , 333–347 (2020).
Barnston, A. G., Tippett, M. K., L’Heureux, M. L., Li, S. & DeWitt, D. G. Skill of real-time seasonal ENSO model predictions during 2002–11: is our capability increasing? Bull. Am. Meteorol. Soc. 93 , 631–651 (2012).
Liu, H., Tang, Y., Chen, D. & Lian, T. Predictability of the Indian Ocean Dipole in the coupled models. Clim. Dyn. 48 , 2005–2024 (2017).
Climate Driver Update Archive. http://www.bom.gov.au/climate/enso/wrap-up/archive/20190625.archive.shtml (2019).
Australian seasonal bushfire outlook: August 2019. Technical Report (Bushfire & natural hazards CRC, 2019). https://www.bnhcrc.com.au/hazardnotes/63 .
Thompson, V. et al. Risk and dynamics of unprecedented hot months in South East China. Clim. Dyn. 52 , 2585–2596 (2019).
Grose, M. R. et al. Insights from CMIP6 for Australia’s future climate. Earths Future 8 , e2019EF001469 (2020).
Ukkola, A. M., Kauwe, M. G. D., Roderick, M. L., Abramowitz, G. & Pitman, A. J. Robust future changes in meteorological drought in CMIP6 projections despite uncertainty in precipitation. Geophys. Res. Lett. 47 , e2020GL087820 (2020).
Williams, A. P. et al. Observed impacts of anthropogenic climate change on wildfire in California. Earths Future 7 , 892–910 (2019).
Pechony, O. & Shindell, D. T. Driving forces of global wildfires over the past millennium and the forthcoming century. Proc. Natl Acad. Sci. USA 107 , 19167–19170 (2010).
Lizundia-Loiola, J., Otón, G., Ramo, R. & Chuvieco, E. A spatio-temporal active-fire clustering approach for global burned area mapping at 250 m from MODIS data. Remote Sens. Environ. 236 , 111493 (2020).
Data.NSW. NPWS Fire History - Wildfires and Prescribed Burns. https://data.nsw.gov.au/data/dataset/fire-history-wildfires-and-prescribed-burns-1e8b6 (2021).
Luke, R. H. & McArthur, A. G. Bush fires in Australia . (Australian Government Publishing Service, Canberra, Australia, 1978).
Noble, I. R., Gill, A. M. & Bary, G. A. V. McArthur’s fire-danger meters expressed as equations. Aust. J. Ecol. 5 , 201–203 (1980).
Harada, Y. et al. The JRA-55 reanalysis: representation of atmospheric circulation and climate variability. J. Meteorol. Soc. Japan Ser. II 94 , 269–302 (2016).
Kobayashi, S. et al. The JRA-55 reanalysis: general specifications and basic characteristics. J. Meteorol. Soc. Japan Ser. II 93 , 5–48 (2015).
Jones, D. A., Wang, W. & Fawcett, R. High-quality spatial climate data-sets for Australia. Aust. Meteorol. Oceanogr. J. 58 , 233 (2009).
Raupach, M. R. et al. Australian water availability project (AWAP): CSIRO marine and atmospheric research component: final report for phase 3. Technical Report, Centre for Australian weather and climate research (Bureau of Meteorology and CSIRO, 2009) https://www.droughtmanagement.info/literature/CAWCR_AWAP_Final_report_phase_3_2009.pdf .
Delworth, T. L. et al. GFDL’s CM2 global coupled climate models. Part I: formulation and simulation characteristics. J. Clim. 19 , 643–674 (2006).
O’Kane, T. J. et al. Coupled data assimilation and ensemble initialization with application to multiyear ENSO prediction. J. Clim. 32 , 997–1024 (2018).
O’Kane, T. J. et al. CAFE60v1: a 60-year large ensemble climate reanalysis. Part I: system design, model configuration and data assimilation. J. Clim. 1 , 1–48 (2021).
O’Kane, T. J. et al. CAFE60v1: a 60-year large ensemble climate reanalysis. Part II: evaluation. J. Clim. 1 , 1–62 (2021).
Spearman, C. The Proof and Measurement of Association Between Two Things . Studies in individual differences: The search for intelligence (Appleton-Century-Crofts, East Norwalk, 1961).
Wilks, D. S. Statistical methods in the atmospheric sciences , vol. 100 (Academic press, 2011).
Teutschbein, C. & Seibert, J. Bias correction of regional climate model simulations for hydrological climate-change impact studies: Review and evaluation of different methods. J. Hydrol. 456-457 , 12–29 (2012).
Maraun, D. Bias correcting climate change simulations - a critical review. Curr. Clim. Change Rep. 2 , 211–220 (2016).
Risbey, J. S. et al. Standard assessments of climate forecast skill can be misleading. Nat. Commun. 12 , 4346 (2021).
Trenberth, K. E. The definition of El Niño. Bull. Am. Meteorol. Soc. 78 , 2771–2778 (1997).
Saji, N. H., Goswami, B. N., Vinayachandran, P. N. & Yamagata, T. A dipole mode in the tropical Indian Ocean. Nature 401 , 360–363 (1999).
Gong, D. & Wang, S. Definition of Antarctic Oscillation index. Geophys. Res. Lett. 26 , 459–462 (1999).
Rayner, N. A. et al. Global analyses of sea surface temperature, sea ice, and night marine air temperature since the late nineteenth century. J. Geophys. Res. Atmos 108 , 4407 (2003).
Download references
Acknowledgements
This work was supported by the CSIRO Decadal Climate Forecasting Project and the Australasian Leadership Computing Grants scheme, with computational resources provided by NCI Australia and the Pawsey Supercomputing Centre, both funded by the Australian Government. The authors would like to acknowledge the Pangeo community for developing many of the open-source tools and workflows used in this paper.
Author information
Authors and affiliations.
CSIRO Oceans & Atmosphere, Castray Esplanade, Hobart, TAS, 7004, Australia
Dougal T. Squire, Doug Richardson, James S. Risbey, Amanda S. Black, Richard J. Matear, Didier Monselesan, Thomas S. Moore & Carly R. Tozer
CSIRO Oceans & Atmosphere, 107-121 Station Street, Aspendale, VIC, 3195, Australia
Vassili Kitsios
You can also search for this author in PubMed Google Scholar
Contributions
D.T.S. and V.K. ran the ensemble forecasts. D.T.S. devised and performed the analysis and wrote the draft paper. All authors contributed to the development of the method, the interpretation of results and reviewed the paper.
Corresponding author
Correspondence to Dougal T. Squire .
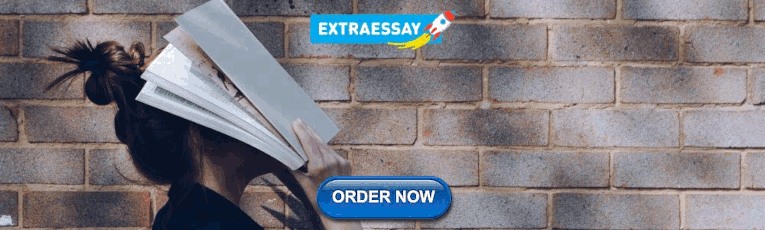
Ethics declarations
Competing interests.
The authors declare no competing interests.
Additional information
Publisher’s note Springer Nature remains neutral with regard to jurisdictional claims in published maps and institutional affiliations.
Supplementary information
Supplementary information, rights and permissions.
Open Access This article is licensed under a Creative Commons Attribution 4.0 International License, which permits use, sharing, adaptation, distribution and reproduction in any medium or format, as long as you give appropriate credit to the original author(s) and the source, provide a link to the Creative Commons license, and indicate if changes were made. The images or other third party material in this article are included in the article’s Creative Commons license, unless indicated otherwise in a credit line to the material. If material is not included in the article’s Creative Commons license and your intended use is not permitted by statutory regulation or exceeds the permitted use, you will need to obtain permission directly from the copyright holder. To view a copy of this license, visit http://creativecommons.org/licenses/by/4.0/ .
Reprints and permissions
About this article
Cite this article.
Squire, D.T., Richardson, D., Risbey, J.S. et al. Likelihood of unprecedented drought and fire weather during Australia’s 2019 megafires. npj Clim Atmos Sci 4 , 64 (2021). https://doi.org/10.1038/s41612-021-00220-8
Download citation
Received : 30 May 2021
Accepted : 05 November 2021
Published : 08 December 2021
DOI : https://doi.org/10.1038/s41612-021-00220-8
Share this article
Anyone you share the following link with will be able to read this content:
Sorry, a shareable link is not currently available for this article.
Provided by the Springer Nature SharedIt content-sharing initiative
This article is cited by
Climate change unevenly affects the dependence of multiple climate-related hazards in china.
- Xuezheng Zong
npj Climate and Atmospheric Science (2024)
Australia’s 2019/20 Black Summer fire weather exceptionally rare over the last 2000 years
- Danielle G. Udy
- Tessa R. Vance
- Nerilie Abram
Communications Earth & Environment (2024)
Identification and characterization of global compound heat wave: comparison from four datasets of ERA5, Berkeley Earth, CHIRTS and CPC
- Lijun Jiang
- Jiahua Zhang
Climate Dynamics (2024)
The characteristics and future projections of fire danger in the areas around mega-city based on meteorological data–a case study of Beijing
- Mengxin Bai
Frontiers of Earth Science (2024)
Research progresses and prospects of multi-sphere compound extremes from the Earth System perspective
- Zengchao Hao
Science China Earth Sciences (2024)
Quick links
- Explore articles by subject
- Guide to authors
- Editorial policies
Sign up for the Nature Briefing: Anthropocene newsletter — what matters in anthropocene research, free to your inbox weekly.

Disasters in Australia and New Zealand
Historical Approaches to Understanding Catastrophe
- © 2020
- Scott McKinnon 0 ,
- Margaret Cook 1
Australian Centre for Culture, Environment, Society and Space, University of Wollongong, Wollongong, Australia
You can also search for this editor in PubMed Google Scholar
School of Social Sciences, University of the Sunshine Coast, Sippy Downs, Australia
Offers the first edited collection of scholarly research on disaster history in Australia and New Zealand
Responds to a growing interest in disaster histories prompted by a changing climate
Incorporates cutting edge research in the field undertaken by established and emerging scholars
6101 Accesses
11 Citations
73 Altmetric
This is a preview of subscription content, log in via an institution to check access.
Access this book
Subscribe and save.
- Get 10 units per month
- Download Article/Chapter or eBook
- 1 Unit = 1 Article or 1 Chapter
- Cancel anytime
- Available as EPUB and PDF
- Read on any device
- Instant download
- Own it forever
- Compact, lightweight edition
- Dispatched in 3 to 5 business days
- Free shipping worldwide - see info
- Durable hardcover edition
Tax calculation will be finalised at checkout
Other ways to access
Licence this eBook for your library
Institutional subscriptions
About this book
Similar content being viewed by others, hazards and disasters in the anthropocene: some critical reflections for the future.
Critical Indigenous Disaster Studies: Doomed to Resilience?
Thinking About Disasters: A Call for Intersectionality and Transdisciplinarity in Disaster Studies
- earthquakes
- historical geography
- emergency management and disaster risk reduction
- disasters research
- Environmental Geography
Table of contents (10 chapters)
Front matter, introduction.
- Scott McKinnon, Margaret Cook
Lessons from Past Disasters
“best forgotten”: black saturday’s difficult stories, shaped by fire: how bushfires forged migrant environmental understandings and memories of place.
- Gretel Evans
Placing Memories of Unforgettable Fires: Official Commemoration and Community Recovery After the 2003 ACT Firestorm
Scott McKinnon
Human Understandings of Disaster
“shaken but not stirred”: the aftermath of urban disasters.
Margaret Cook
Escaping Water: Living Against Floods in Townsville, North Queensland, from Settlement to 2019
- Rohan Lloyd, Patrick White, Claire Brennan
A Perfect Storm
- Ian Townsend
Legacies of the Past
Shallow fire literacy hinders robust fire policy: black saturday and prescribed burning debates, decolonising settler hazardscapes of the waipā: māori and pākehā remembering of flooding in the waikato 1900–1950.
- Meg Parsons, Karen Fisher
Where the Wild Things Were
- Deb Anderson
Back Matter
Editors and affiliations, about the editors, bibliographic information.
Book Title : Disasters in Australia and New Zealand
Book Subtitle : Historical Approaches to Understanding Catastrophe
Editors : Scott McKinnon, Margaret Cook
DOI : https://doi.org/10.1007/978-981-15-4382-1
Publisher : Palgrave Macmillan Singapore
eBook Packages : Social Sciences , Social Sciences (R0)
Copyright Information : The Editor(s) (if applicable) and The Author(s), under exclusive license to Springer Nature Singapore Pte Ltd. 2020
Hardcover ISBN : 978-981-15-4381-4 Published: 08 July 2020
Softcover ISBN : 978-981-15-4384-5 Published: 08 July 2021
eBook ISBN : 978-981-15-4382-1 Published: 07 July 2020
Edition Number : 1
Number of Pages : XVI, 202
Number of Illustrations : 11 b/w illustrations
Topics : Human Geography , Oral History , Environmental Geography
- Publish with us
Policies and ethics
- Find a journal
- Track your research
NSW, Queensland floods on track to be among country's worst-ever natural disasters, Climate Council says
ABC Science
Topic: Climate Change
Floods account for the greatest property damage of any natural disaster in Australia. ( ABC North Coast: Samantha Turnbull )
The ongoing floods in Queensland and New South Wales are on track to be one of Australia's worst-ever natural disasters.
And if this news isn't bleak enough, we can expect more extensive and more frequent flooding as climate change intensifies.
These are the conclusions of a Climate Council report summarising the latest data on the scale of the disaster and underlining the link to global warming caused by human activity.
The Brisbane "rain bomb", for instance, dropped more water on the city than typically falls in London over an entire year, and the volume flowing through the Brisbane River far exceeded the 2010-11 floods.
Insurance claims along the eastern seaboard may be as great as the 2019-20 Black Summer bushfires.
So how bad is the property damage?
Insurance claims will exceed 2010-11 Queensland floods
The scale of the 2022 floods is emerging as reports filter out of the disaster zones, insurance claims are made, and statistics compiled.
The Climate Council report, published today, provides a snapshot of what we know so far — and it appears this natural disaster is going to rank highly.
The cost of weather-related disasters in Australia has risen since the 1970s. ( Supplied: Climate Council )
The total value of the insurance claims will exceed those of previous major floods, including the last time Brisbane flooded in 2010-11, said Nicki Hutley, economics spokesperson for the Climate Council.
"Obviously we're still counting the costs and don't know the full extent," said Ms Hutley, a former Deloitte economist.
"The Queensland floods of 2010-11 had property damage of $1.5 billion, and we're certainly probably already at that level, if not exceeding it."
Insurance claims could even exceed the 2019-20 Black Summer bushfires, which totalled about $2.5 billion.
"Certainly the property damage may be higher — the fires burnt through millions of hectares, but a lot was uninhabited," she said.
"In terms of the total number of people affected and total costs, I imagine this will be, if not the worst natural disaster, then certainly right up there."
Flood level records broken
Local records for rainfall, flood levels and flood rescues have been broken.
The "rain bomb" that broke over Brisbane on Friday, February 25, deposited 80 per cent of the region's annual rainfall within three days.
The downpour was greater than the 2010-11 floods: about 50 per cent more water poured into the Brisbane River's Wivenhoe Dam in half the time.
Brisbane's "rain bomb" dumped more than one metre of water on most of the city. ( Supplied: Climate Council )
Further to the south, flood level records that had stood for decades were broken.
Wilsons River in the Northern Rivers district of NSW broke the 1954 flood level record by more than two metres.
The Richmond River at Woodburn topped 7.18 metres, nearly 50 per cent high than its previous record.
NSW SES carried out more flood rescues in 24 hours than it had ever done before.
The mass of water in this flood is "quite remarkable", says Will Steffen from Australian National University's Climate Change Institute.
"It will certainly rank up with one of the biggest natural disasters around the country," he said.
Extreme events are becoming 'ordinary'
When Brisbane flooded in 2010-11, the disaster was reported in the media as a one-in-100-year event.
The same happened when Townsville flooded in 2019, Professor Steffen said.
"And now we've heard that term applies to the latest bout of flooding," he said.
"The point is we're having extreme events more often. 'Ordinary' extreme events are occurring now in rapid succession."
Parts of Brisbane were still recovering from the 2010-11 floods when the latest flood hit. ( ABC Radio Brisbane: Antonia O'Flaherty )
Extreme rainfall can occur for a variety of reasons and directly linking a single event, like a flood, to climate change requires a formal attribution study, which can take years to produce.
However, it is possible to say whether climate change contributed to the floods.
This is a complicated question and some experts say there's a risk of overstating the effect of climate change on the multi-day heavy rainfall that has led to the 2022 floods.
But Professor Steffen says there's no doubt that a "climate system on steroids" contributed to the floods.
A warmer atmosphere is both more energetic and can hold more water, which "stacks the odds" in favour of extreme rainfall, he said.
"For every one degree of temperature rise, the atmosphere can hold 7 per cent more water.
"The intensity of rainfall will continue to increase on that ratio of 7 per cent for every degree of temperature rise."
Floods cause the greatest damage of any natural disaster in Australia. ( Supplied: Climate Council )
This change is already being observed, he said.
The 2020 CSIRO and Bureau of Meteorology State of the Climate report found the intensity of short-duration extreme rainfall (i.e. flash flooding) had increased by around 10 per cent in some regions.
The recent Intergovernmental Panel on Climate Change (IPCC) report predicted the world was on track to warm by 1.5C in the early 2030s, and by 2C this century.
As the temperature of the atmosphere continues to rise, destructive floods are likely to happen more regularly, Professor Steffen says.
"They would happen at a regularity that would make it difficult to recover in between events — that's almost happening now.
"The economics of this is going to make a lot of places unviable."
Should every house be rebuilt?
On Wednesday, Prime Minister Scott Morrison declared a national floods emergency , which gives the federal government power to deploy money and resources faster.
Communities impacted by the floods will receive public funds to rebuild.
But some areas should not be rebuilt, Professor Steffen says.
"We need to have a very good look at zoning laws and where people are allowed to build."
Insurers are already refusing to cover flood damage in some areas, because climate change has made the risk so high, Ms Hutley said.
One solution to this is for the government to step in as the insurer, but this is risky and could eat up public funds.
"It's a never-ending bottomless liability and it becomes extremely dangerous from a financial security point of view," Ms Hutley said.
"We've been absolutely negligent on where people can build for a decade or more."
Politicians will have to make difficult and unpopular decisions about where people can rebuild — and where new housing developments can be located.
And these decisions will need to be made quickly, she said.
"People who want to rebuild now need an answer sooner rather than later."
Australian and Asian Natural Disaster Epidemiology Report (Assessment)
- To find inspiration for your paper and overcome writer’s block
- As a source of information (ensure proper referencing)
- As a template for you assignment
Introduction
Saudi arabia, turkmenistan.
Nowadays, epidemiology is an interdisciplinary science that studies the “occurrence and distribution of health-related events” and applies the gained knowledge to manage related issues (Porta, 2014, p. 76). Disaster epidemiology uses epidemiologic tools to gain knowledge that can help to control disasters (Bissell, 2013; Guha-Sapir, Santos, & Borde, 2013). The present paper discusses the disaster epidemiology of Australia and three countries that are located in the Asian region (Saudi Arabia, Turkmenistan, and Israel).
According to Subanthore (2011), the region is prone to natural disasters, but the specifics differ for its diverse areas due to their “varied landscape and human geography” (p. 22). Therefore, it appears improbable that the epidemiology of Australia can be compared to that of the whole region. For this paper, the Asian countries were chosen randomly in an attempt to represent different areas. The data was retrieved from the CRED (2016) database (the Center for Research on the Epidemiology of Disasters).
Australia has been very prone to cases of natural disasters, and CRED (2016) provides the data on 228 of them that happened between 1990 and 2016 (see Table 1). The deadliest of the disasters include droughts, heatwaves, wildfires, and floods; apart from that, floods were occurring most often and caused the greatest total damage.
The second most “common” disaster was storm followed by wildfire and drought. The cases of extreme temperatures were relatively rare, but they affected great numbers of people and caused many deaths; earthquakes caused relatively few deaths but result in significant damage. The relatively “safe” disasters included the epidemic and two infestations: none of them resulted in deaths, but the infestation caused noticeable damage. The landslides resulted in deaths.
Table 1 | ||||
Disaster | Count | Total Deaths | Total Affected | Total Damage (‘000 US$) |
Drought | 10 | 600 | 7080000 | 10573000 |
Earthquake | 4 | 12 | 11430 | 1009675 |
Epidemic | 1 | 0 | 6 | 0 |
Extreme temperature | 6 | 509 | 4602784 | 0 |
Flood | 95 | 337 | 3844983 | 15230172 |
Insect infestation | 2 | 0 | 0 | 120000 |
Landslide | 2 | 28 | 101 | 0 |
Storm | 71 | 212 | 573220 | 18487832 |
Wildfire | 37 | 501 | 90931 | 3494394 |
The information that CRED (2016) offers concerning Saudi Arabia includes a total of 26 natural disasters with two of them being epidemics. While the latter did not result in any financial damage, they led to the deaths of four people; the rest of the disasters were floods that took over 2,000 lives and were extremely costly for the country (see Table 2).
Table 2 | ||||
Disaster | Count | Total Deaths | Total Affected | Total Damage (‘000 US$) |
Epidemic | 2 | 4 | 3200 | |
Flood | 24 | 2056 | 12347948 | 17542800 |
As can be seen from Israel’s example, the countries of the Asian region may have a more diverse history of natural disasters than Saudi Arabia. CRED (2016) reports fourteen natural disasters in Israel, including a drought, an epidemic, a case of extreme temperatures (a cold wave), two floods, a case of a landslide, five storms, and three forest fires (see Table 4). The latter has resulted in the greatest total number of deaths (forty people), but it is noteworthy that one landslide caused almost half as many deaths as three wildfires (twenty people). The wildfires were also very costly, but the cold wave resulted in greater damages.
Table 4 | ||||
Disaster | Count | Total Deaths | Total Affected | Total Damage (‘000 US$) |
Drought | 1 | 0 | 0 | 75000 |
Epidemic | 1 | 12 | 139 | 0 |
Extreme temperature | 1 | 0 | 0 | 550000 |
Flood | 2 | 2 | 1000 | 0 |
Landslide | 1 | 20 | 13 | 0 |
Storm | 5 | 27 | 2003610 | 42750 |
Wildfire | 3 | 44 | 20262 | 315000 |
CRED (2016) reports only two disaster cases that took place in Turkmenistan: an earthquake that cost eleven lives, which made it the most deadly event of the two, and a flood, which affected over 400 people and resulted in significant damage but, fortunately, did not cause any deaths (see Table 3).
Table 3 | ||||
Disaster | Count | Total Deaths | Total Affected | Total Damage (‘000 US$) |
Earthquake | 1 | 11 | 0 | 0 |
Flood | 1 | 0 | 420 | 99870 |
The four countries differ in the total number of disaster cases with Australia having the biggest number followed by Saudi Arabia, Israel, and Turkmenistan. The data was available for the years 1900-2016, and it is noteworthy that Australia was founded in the year 1901 while Saudi Arabia was formed in 1932, Israel in 1948, and Turkmenistan was a part of the USSR until 1991 (Dalal, 2012).
The comparative “age” of the countries corresponds to the decreasing number of their total disaster count, but other factors (especially the geography) must also be taken into account when attempting to explain the difference. In general, the presented paper along with the CRED (2016) database demonstrates that the epidemiology of different countries represents their unique history of being prone to particular natural disasters, which does not allow finding meaningful similarities between them. Even the countries located in the same region (Asia) do not exhibit the same or similar patterns.
As stated earlier, this phenomenon is explained by the size of the region that encompasses diverse landscapes, climates, and countries (Subanthore, 2011). Despite these dissimilarities, all the data has one similar characteristic: since the information represents the specific, unique history of disasters of a particular country, it can help to make conclusions on disaster proneness, which is of interest for disaster management.
The presented paper used CRED (2016) data to analyse the epidemiology of four countries: three Asian region countries and Australia. The results of the comparison demonstrate notable diversity, which implies that the disaster history of particular countries and regions is unique to a certain extent. It can be concluded that the analysis of the data that is similar to that of CRED (2016), but which is more detailed and region-specific is likely to inform predictions and decisions in disaster preparedness and management.
Bissell, R. (2013). Preparedness and response for catastrophic disasters . Boca Raton: CRC Press/Taylor & Francis.
Commonwealth of Australia. (2011). National Strategy for Disaster Resilience .
CRED. (2016). Country Profile .
Dalal, R. (2012). The illustrated timeline of the history of the world . New York: Rosen.
Guha-Sapir, D., Santos, I., & Borde, A. (2013). The economic impacts of natural disasters . Oxford: Oxford University Press.
Hart, P. & Sundelius, B. (2013). Crisis management revisited: A new agenda for research, training and capacity building within Europe . Cooperation And Conflict , 48 (3), 444-461. Web.
Mpofu, E. (2014). Community-oriented health services . New York, NY: Springer Publishing Company.
Norman, S. (2006). New Zealand’s holistic framework for disaster recovery . The Australian Journal of Emergency Management , 21 (4), 16-20. Web.
Ostadtaghizadeh, A., Ardalan, A., Paton, D., Jabbari, H., & Khankeh, H. R. (2015). Community disaster resilience: a systematic review of assessment models and tools. PLoS currents , 7 , 1-24. Web.
Porta, M. (2014). A dictionary of epidemiology . Oxford: Oxford University Press.
Queensland Government. (2016). The PPRR risk management model .
Rogers, P. (2011). Development of Resilient Australia: enhancing the PPRR approach with anticipation, assessment and registration of risks . The Australian Journal of Emergency Management , 26 (1), 54-58. Web.
Subanthore, A. (2011). Asia, West, Central, and South. In K. Penuel & M. Statler (Eds.), Encyclopedia of disaster relief (pp. 22-24). Thousand Oaks, CA: Sage Publications.
Templeman, D., & Bergin, A. (2008). Taking a punch: Building a more resilient Australia .
United Nations Office for Disaster Risk Reduction. (2013). Disaster Risk Reduction in the United Nations . Web.
- The Changing Meaning of Disaster
- Saudi Arabian and Asian Disaster Epidemiology
- Emergency Plan for Wildfires in California
- Documentary Review "Killer Landslides"
- Causes, Effects and Mitigation of Global Wildfires 2021
- Communication Analysis in Oil Spills
- New Orleans Life after Hurricane Katrina
- Natural Disasters and Global Social Issues
- Flooding Problem in Philippines
- Understanding Earthquake Statistics: Frequency, Magnitude, and Data Sources
- Chicago (A-D)
- Chicago (N-B)
IvyPanda. (2020, August 5). Australian and Asian Natural Disaster Epidemiology. https://ivypanda.com/essays/australian-and-asian-natural-disaster-epidemiology/
"Australian and Asian Natural Disaster Epidemiology." IvyPanda , 5 Aug. 2020, ivypanda.com/essays/australian-and-asian-natural-disaster-epidemiology/.
IvyPanda . (2020) 'Australian and Asian Natural Disaster Epidemiology'. 5 August.
IvyPanda . 2020. "Australian and Asian Natural Disaster Epidemiology." August 5, 2020. https://ivypanda.com/essays/australian-and-asian-natural-disaster-epidemiology/.
1. IvyPanda . "Australian and Asian Natural Disaster Epidemiology." August 5, 2020. https://ivypanda.com/essays/australian-and-asian-natural-disaster-epidemiology/.
Bibliography
IvyPanda . "Australian and Asian Natural Disaster Epidemiology." August 5, 2020. https://ivypanda.com/essays/australian-and-asian-natural-disaster-epidemiology/.
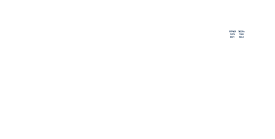
Natural Disasters
How many people die from disasters, and how are these impacts changing over time?
By: Hannah Ritchie and Pablo Rosado
Disasters – from earthquakes and storms to floods and droughts – kill approximately 40,000 to 50,000 people per year. This is the average over the last few decades.
While that’s a relatively small fraction of all deaths globally , disasters can have much larger impacts on specific populations. Single extreme events can kill tens to hundreds of thousands of people. In the 20th century, more than a million deaths per year were not uncommon.
Disasters have other large impacts, too. Millions of people are displaced – some left homeless – by them each year. And the economic costs of extreme events can be severe, and hard to recover from. This is particularly true in lower-income countries.
We are not defenceless against disasters: deaths from disasters have fallen significantly over the last century as a result of early warning systems, better infrastructure, more productive agriculture, and coordinated responses.
As climate change increases the risks of more extreme events, making societies even more resilient will be crucial to prevent our recent progress from reversing. To do so, we need to understand how disaster events are changing, who is most vulnerable, and what can be done to protect them.
On this page, you will find our complete collection of data, charts, and research on natural disasters and their costs.
Explore Data on Natural Disasters
Research & writing.
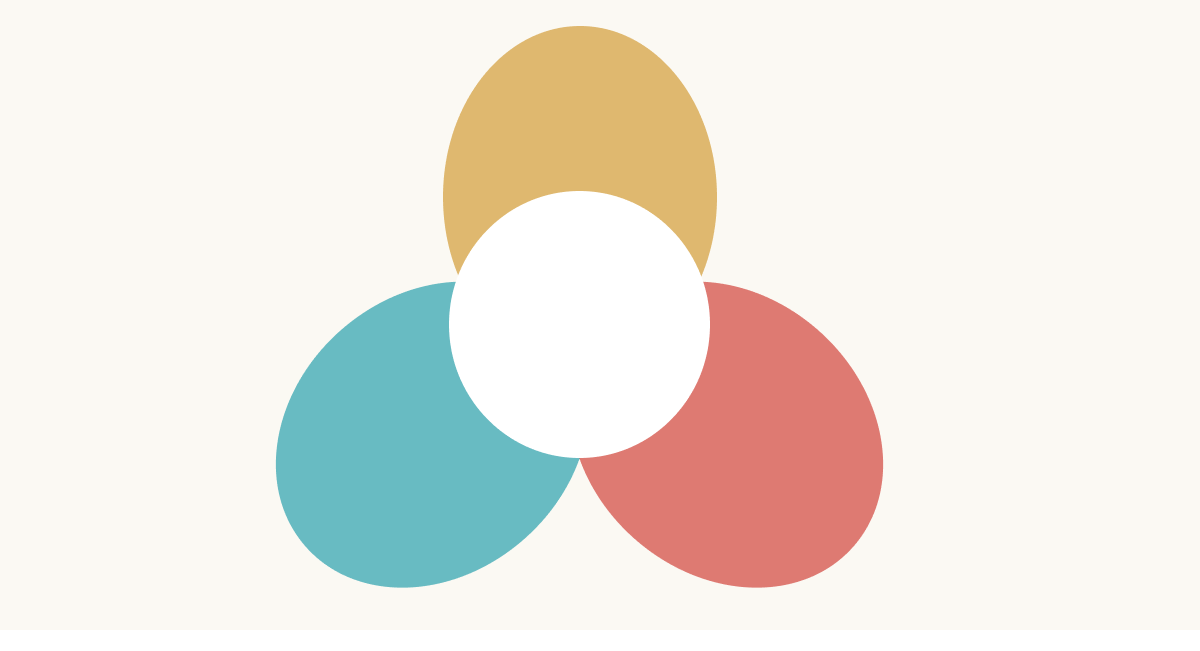
The world has become more resilient to disasters, but investment is needed to save more lives
Deaths from disasters have fallen, but we need to build even more resilience to ensure this progress doesn’t reverse.
Hannah Ritchie
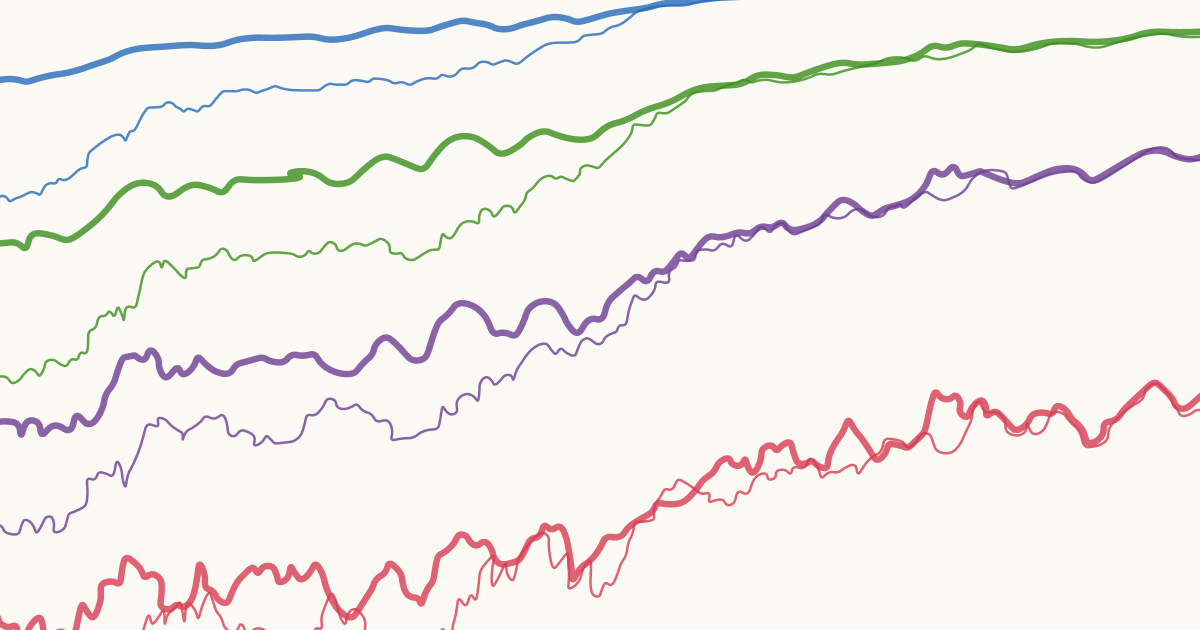
Weather forecasts have become much more accurate; we now need to make them available to everyone
A four-day forecast today is as accurate as a one-day forecast 30 years ago.
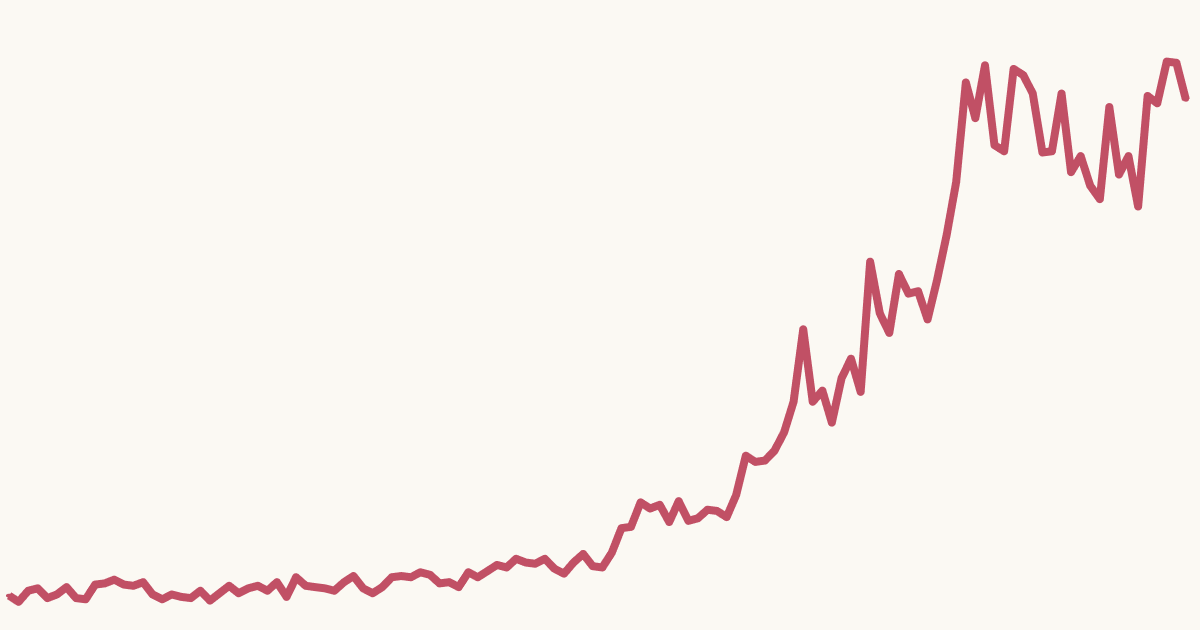
Is the number of natural disasters increasing?
A deep dive into missing data and the limitations of disaster databases.
Hannah Ritchie and Pablo Rosado
Articles on natural disasters
How are extreme weather events and the climate evolving in the united states.
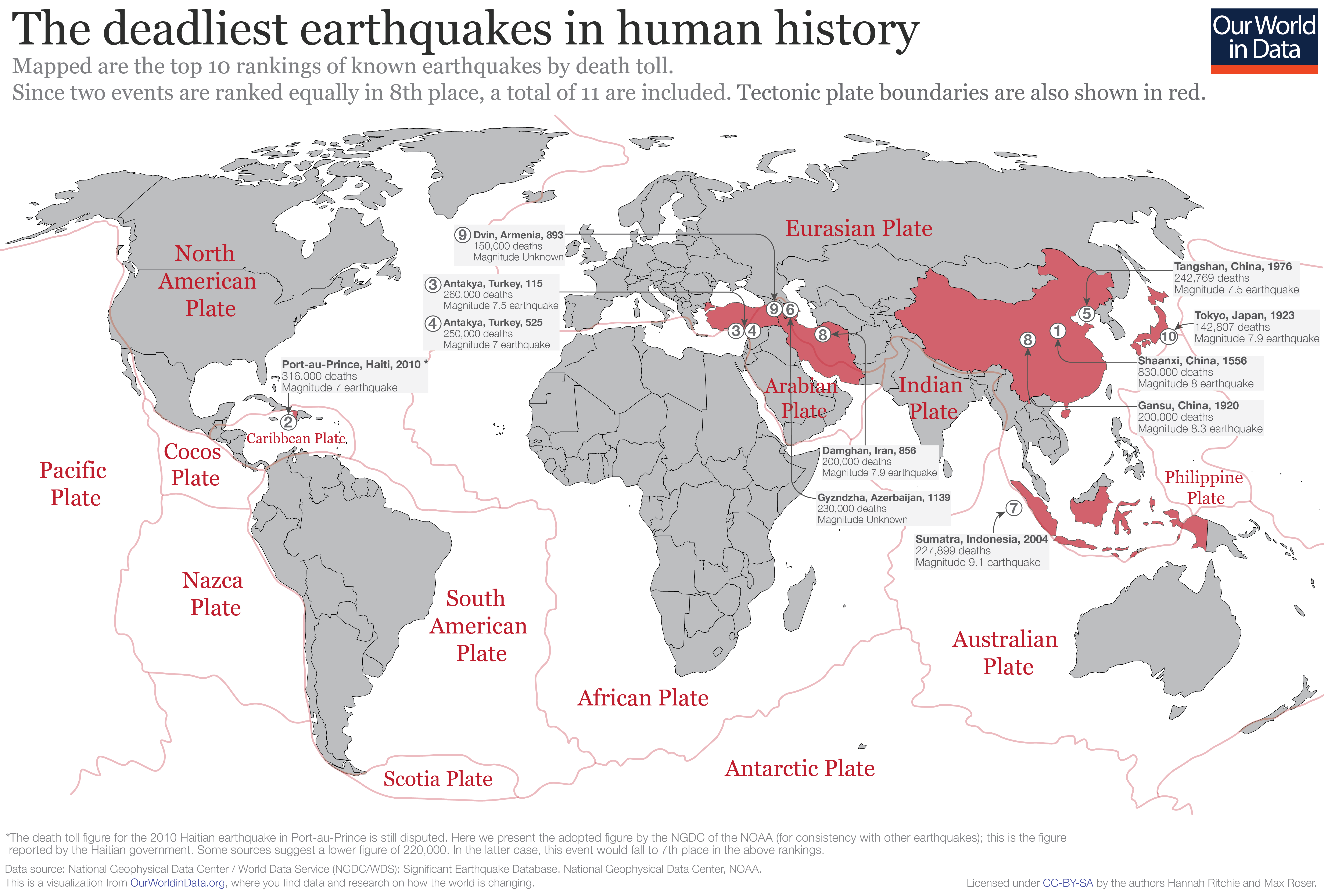
What were the world's deadliest earthquakes?
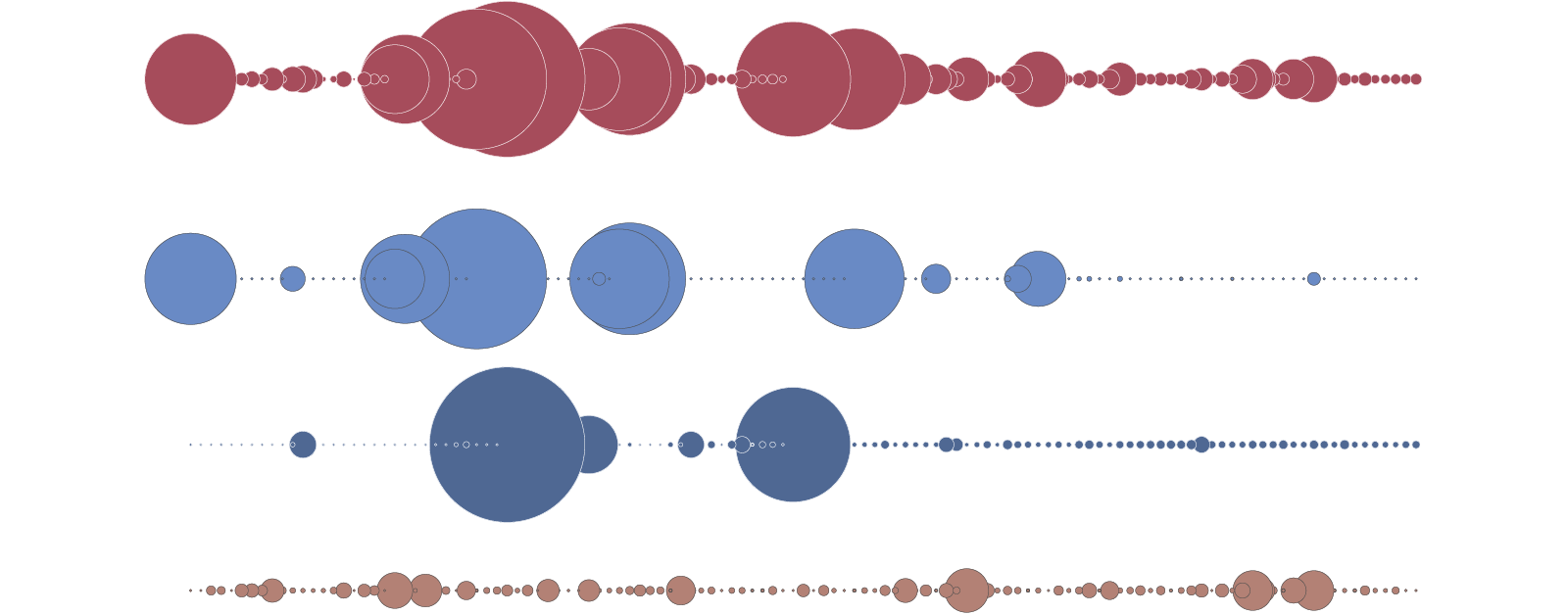
A century of global deaths from disasters
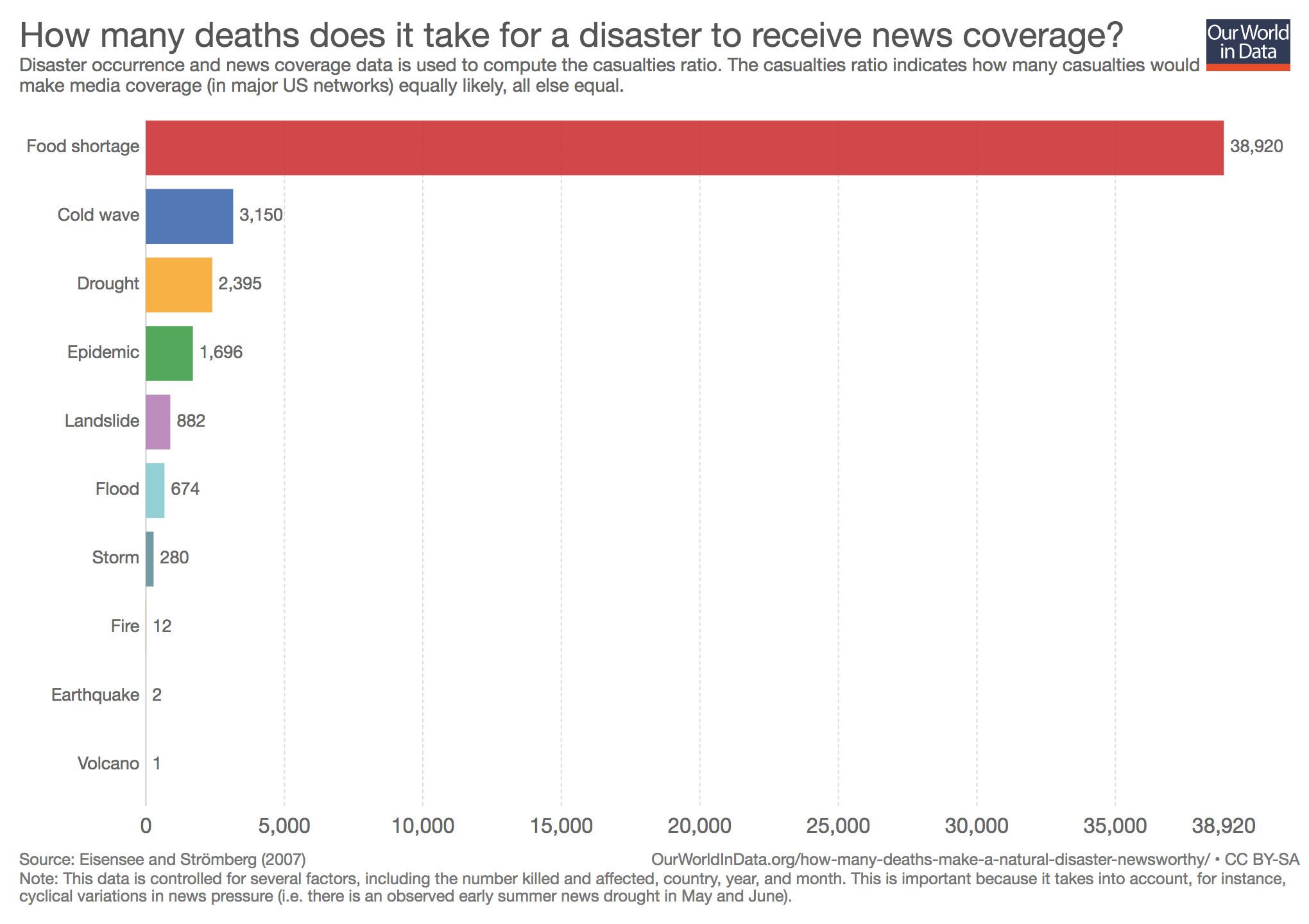
Not all deaths are equal: How many deaths make a natural disaster newsworthy?
Sandra Tzvetkova
Interactive charts on Natural Disasters
Cite this work.
Our articles and data visualizations rely on work from many different people and organizations. When citing this topic page, please also cite the underlying data sources. This topic page can be cited as:
BibTeX citation
Reuse this work freely
All visualizations, data, and code produced by Our World in Data are completely open access under the Creative Commons BY license . You have the permission to use, distribute, and reproduce these in any medium, provided the source and authors are credited.
The data produced by third parties and made available by Our World in Data is subject to the license terms from the original third-party authors. We will always indicate the original source of the data in our documentation, so you should always check the license of any such third-party data before use and redistribution.
All of our charts can be embedded in any site.
Our World in Data is free and accessible for everyone.
Help us do this work by making a donation.
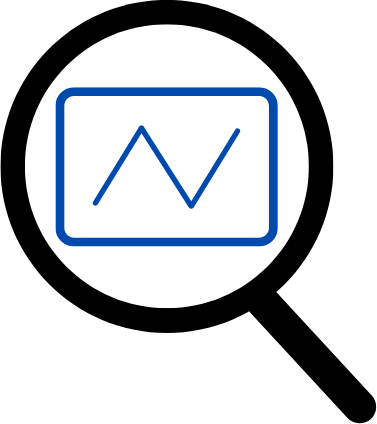
Presentations made painless
- Get Premium
103 Natural Disaster Essay Topic Ideas & Examples
Inside This Article
Natural disasters are events that occur in nature and can cause significant destruction and loss of life. They can take many forms, including hurricanes, earthquakes, floods, wildfires, and tsunamis. Writing an essay on natural disasters can be a daunting task, but with the right topic and approach, you can create a compelling and informative piece of writing. Here are 103 natural disaster essay topic ideas and examples to help you get started:
- The impact of climate change on natural disasters
- How do natural disasters affect the environment?
- The role of technology in predicting and mitigating natural disasters
- The psychological effects of natural disasters on survivors
- The economic impact of natural disasters on communities
- The social impact of natural disasters on vulnerable populations
- How do natural disasters affect wildlife and ecosystems?
- The role of government in responding to natural disasters
- The importance of disaster preparedness and planning
- The relationship between poverty and vulnerability to natural disasters
- The connection between deforestation and increased risk of natural disasters
- The impact of natural disasters on global food security
- The role of international aid in responding to natural disasters
- The long-term effects of natural disasters on communities
- The ethics of disaster response and recovery efforts
- The role of media in reporting on natural disasters
- The impact of natural disasters on mental health
- The connection between natural disasters and climate refugees
- The role of education in preparing communities for natural disasters
- The impact of natural disasters on infrastructure and development
- The relationship between natural disasters and disease outbreaks
- The connection between natural disasters and conflict
- The role of insurance in mitigating the financial impact of natural disasters
- The impact of natural disasters on agriculture and food production
- The connection between natural disasters and water scarcity
- The role of NGOs in responding to natural disasters
- The impact of natural disasters on tourism and the economy
- The relationship between natural disasters and climate justice
- The role of community resilience in recovering from natural disasters
- The impact of natural disasters on coastal communities
- The connection between natural disasters and mental health stigma
- The role of technology in tracking and monitoring natural disasters
- The impact of natural disasters on indigenous communities
- The relationship between natural disasters and climate change adaptation
- The role of gender in disaster response and recovery efforts
- The impact of natural disasters on human rights
- The connection between natural disasters and urbanization
- The role of social media in disaster response and recovery efforts
- The impact of natural disasters on education and schools
- The relationship between natural disasters and biodiversity loss
- The role of community-based organizations in responding to natural disasters
- The impact of natural disasters on clean water access
- The connection between natural disasters and food insecurity
- The role of preparedness drills in communities at risk of natural disasters
- The impact of natural disasters on children and families
- The relationship between natural disasters and displacement
- The role of volunteer groups in disaster response and recovery efforts
- The impact of natural disasters on cultural heritage sites
- The connection between natural disasters and environmental racism
- The role of early warning systems in predicting natural disasters
- The impact of natural disasters on small businesses and local economies
- The relationship between natural disasters and social inequality
- The role of faith-based organizations in disaster response and recovery efforts
- The impact of natural disasters on mental health access and services
- The connection between natural disasters and climate migration
- The role of community gardens in disaster recovery efforts
- The impact of natural disasters on access to healthcare
- The relationship between natural disasters and food deserts
- The role of cultural competency in disaster response and recovery efforts
- The impact of natural disasters on LGBTQ communities
- The connection between natural disasters and environmental justice
- The role of indigenous knowledge in disaster preparedness and response
- The impact of natural disasters on marginalized communities
- The relationship between natural disasters and gentrification
- The role of intersectionality in disaster response and recovery efforts
- The impact of natural disasters on mental health disparities
- The connection between natural disasters and climate denial
- The role of community centers in disaster response and recovery efforts
- The impact of natural disasters on immigrant communities
- The relationship between natural disasters and housing insecurity
- The role of community art projects in disaster recovery efforts
- The impact of natural disasters on access to public transportation
- The connection between natural disasters and environmental activism
- The role of community health clinics in disaster response and recovery efforts
- The impact of natural disasters on access to clean energy
- The relationship between natural disasters and environmental policy
- The role of community shelters in disaster response and recovery efforts
- The impact of natural disasters on access to affordable housing
- The connection between natural disasters and community organizing
- The role of community colleges in disaster response and recovery efforts
- The impact of natural disasters on access to mental health services
- The relationship between natural disasters and community gardens
- The impact of natural disasters on access to public libraries
- The connection between natural disasters and community resilience
- The role of community-based organizations in disaster response and recovery efforts
- The impact of natural disasters on access to affordable healthcare
- The relationship between natural disasters and community art projects
- The connection between natural disasters and environmental policy
- The relationship between natural disasters and community organizing
- The connection between natural disasters and community gardens
- The relationship between natural disasters and community resilience
- The connection between natural disasters and community art projects
These natural disaster essay topics cover a wide range of issues and perspectives, allowing you to explore different aspects of this important and timely subject. Whether you choose to focus on the environmental impact of natural disasters, the social and economic consequences, or the role of communities in responding and recovering, there are plenty of opportunities to explore and analyze in your essay. By selecting a topic that interests you and conducting thorough research, you can create a compelling and informative essay that sheds light on the complexities of natural disasters and their effects on our world.
Want to research companies faster?
Instantly access industry insights
Let PitchGrade do this for me
Leverage powerful AI research capabilities
We will create your text and designs for you. Sit back and relax while we do the work.
Explore More Content
- Privacy Policy
- Terms of Service
© 2024 Pitchgrade
- Free Samples
- Premium Essays
- Editing Services Editing Proofreading Rewriting
- Extra Tools Essay Topic Generator Thesis Generator Citation Generator GPA Calculator Study Guides Donate Paper
- Essay Writing Help
- About Us About Us Testimonials FAQ
- Studentshare
- Environmental Studies
- Natural Disasters in Australia
Natural Disasters in Australia - Essay Example
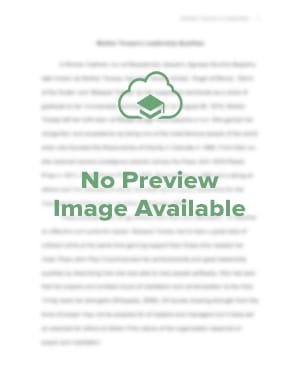
- Subject: Environmental Studies
- Type: Essay
- Level: Undergraduate
- Pages: 7 (1750 words)
- Downloads: 0
Extract of sample "Natural Disasters in Australia"
- Cited: 0 times
- Copy Citation Citation is copied Copy Citation Citation is copied Copy Citation Citation is copied
CHECK THESE SAMPLES OF Natural Disasters in Australia
Emergency management: ash wednesday, the consequences of the economic crisis in australia, japan, and new zealand, emergency event, strength and weaknesses of australian transport system design approach, business continuity management as a tool to solve problems that occurred after a serious dry in australia, early warning system, effective of emergency preparedness, integrated learning program.
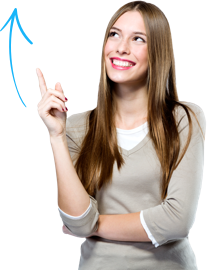
- TERMS & CONDITIONS
- PRIVACY POLICY
- COOKIES POLICY
Essays on Natural Disasters
Faq about natural disasters.
Natural Disasters Essay for Students and Children
500+ words essay on natural disasters.
A Natural disaster is an unforeseen occurrence of an event that causes harm to society. There are many Natural disasters that damage the environment and the people living in it. Some of them are earthquakes , cyclones, floods, Tsunami , landslides, volcanic eruption, and avalanches. Spatial extent measures the degree or severity of the disaster.

Levels of Disaster
The severity or degree of damage can be further divided into three categories:
Small Scale Disasters: Small scale disasters are those that extend from 50 Kms. to 100 Kms. So this kind of disasters does not cause much damage.
Medium-scale disasters: Medium Scale disasters extend from 100 Kms to 500 Kms. These cause more damage than a small scale disaster. Moreover, they can cause greater damage if they occur in colonial states.
Large Scale Disasters: These disasters cover an area of more than 1000 Kms. These cause the most severe damage to the environment. Furthermore, these disasters can even take over a country if the degree is high. For instance, the wiping out of the dinosaurs was because of a large scale natural disaster.
Get the huge list of more than 500 Essay Topics and Ideas
Types of Disasters

Causes: These can cause of releasing of the energy. This release is from the core of the earth. Furthermore, the release of energy causes seismic waves. Rupturing of geological faults causes earthquakes. But other events like volcanic eruptions, landslides mine blasts can also cause it.
Landslides: Landslides is the moving of big boulders of rocks or debris down a slope. As a result, landslides occur on mountains and hilly areas. Moreover, landslides can cause destruction to man-made things in many ways.
Causes: Gravitational pull, volcanic eruptions , earthquakes can cause landslides. Moreover, soil erosion due to deforestation is also a cause of landslides.
Avalanches: Avalanches are like landslides. But instead of rocks thousand tons of snow falls down the slope. Moreover, this causes extreme damage to anything that comes in its way. People who live in snowy mountains always have fear of it.
Causes: Avalanches takes places when there is a large accumulation of snow on the mountains. Moreover, they can also occur from earthquakes and volcanic eruptions. Furthermore, the chances of surviving an avalanche are very less. This is because people die of hypothermia in it.
Tsunami: Tsunami is the production of very high waves in oceans and seas. Moreover, the displacement of the ground causes these high waves. A tsunami can cause floods if it occurs near shores. A Tsunami can consist of multiple waves. Moreover, these waves have a high current. Therefore it can reach coastlines within minutes. The main threat of a tsunami is if a person sees a Tsunami he cannot outrun it.
Causes: Tsunami is unlike normal eaves that occur due to the wind. But Tsunami is waves that occur by ground displacement. Thus earthquakes are the main causes of Tsunamis.
FAQs on Essay on natural disaster
Q1.What are natural disasters?
A1. Natural Disasters are unforeseen events that cause damage to the environment and the people.
Q2.Name some Natural disasters.
A2. Some Natural Disasters are earthquakes, volcanic eruptions, Landslides, floods, Tsunami, avalanches. Natural disasters can cause great damage to human society. But preventive measures can be taken to reduce the damage from these disasters.
Customize your course in 30 seconds
Which class are you in.

- Travelling Essay
- Picnic Essay
- Our Country Essay
- My Parents Essay
- Essay on Favourite Personality
- Essay on Memorable Day of My Life
- Essay on Knowledge is Power
- Essay on Gurpurab
- Essay on My Favourite Season
- Essay on Types of Sports
Leave a Reply Cancel reply
Your email address will not be published. Required fields are marked *
Download the App
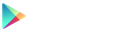
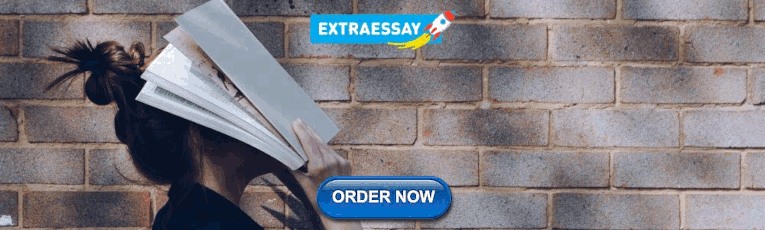
IMAGES
COMMENTS
Natural Disasters In Australia Essay. Decent Essays. 1374 Words; 6 Pages; Open Document. Australia Providing protection of life, property and the environment within the country of Australia is the duty that lies within the scope of the states and territories of the country. Similar to the United States, the Australian government provides a ...
Download CSV Display Table. The 1967 compound disaster commenced in January 1967, when category 3 Tropical Cyclone Elsie struck Western Australia. No deaths were inflicted, but a normalised insurance loss of nearly $200M was incurred as roads, railways and airfields across the state were damaged by floodwaters.
The Business Roundtable, in partnership with Deloitte Access Economics, published an Update to the economic costs of natural disasters in Australia in October 2021, which concluded that even under a low emissions scenario, the cost of natural disasters in Australia is estimated to increase to $73 billion per year by 2060. Scenarios with higher ...
This is a list of all major natural disasters in Australian European history. The natural disasters included here are all the notable events that resulted in significant loss of life or property due to natural, non-biological processes of the Earth within Australian territory. Due to inflation, the monetary damage estimates are not comparable. Unless otherwise noted, the year given is when the ...
Open Document. The Natural Disasters in Australia and Their Effects. Earthquakes are caused by the shifting of the Indian-Australian plate, which is being pushed north and is colliding with the Eurasian, Philippine and Pacific plates. The stress from this collision is released during earthquakes. Earthquakes can occur anywhere in Australia.
Natural disasters in Australia 1. Heatwaves. Just in November 2020, The Washington Post reported that the Australian heatwave in Sydney recorded sustained day temperatures exceeding 40 degrees Celsius - "a feat that had not been accomplished during the month of November in 160 years of record-keeping," says weather Editor Andrew Freedman. 2019 was also recorded by the Australian Climate ...
Australia and New Zealand have both faced a series of devastating floods triggered by climate change and the return of the La Niña weather pattern.So it makes sense that Australia has now sent ...
Dec. 11, 2021. WEE WAA, Australia — Two years ago, the fields outside Christina Southwell's family home near the cotton capital of Australia looked like a dusty, brown desert as drought-fueled ...
A quick glance at the regions that have been officially declared a natural disaster zone in NSW reveals that approximately 36,509 Aboriginal and Torres Strait Islander people have been directly ...
Our methodology is similar to those introduced in previous papers using the UNSEEN approach 46: 1. ... With costs approaching $100 billion, the fires are Australia's costliest natural disaster ...
Disasters in Australia and New Zealand brings together a collection of essays on the history of disasters in both countries. Leading experts provide a timely interrogation of long-held assumptions about the impacts of bushfires, floods, cyclones and earthquakes, exploring the blurred line between nature and culture, asking what are the anthropogenic causes of 'natural' disasters?
Check our natural disaster essay topics 💡 and examples in the article. IvyPanda® Free Essays. Clear. Free Essays; Study Hub. Study Blog. Q&A by Experts. Literature Guides. ... The present paper discusses the disaster epidemiology of Australia and three countries that are located in the Asian region. While the latter did not result in any ...
The ongoing floods in Queensland and New South Wales are on track to be one of Australia's worst-ever natural disasters, a Climate Council report says, linking the destruction to climate change.
Australia. Australia has been very prone to cases of natural disasters, and CRED (2016) provides the data on 228 of them that happened between 1990 and 2016 (see Table 1). The deadliest of the disasters include droughts, heatwaves, wildfires, and floods; apart from that, floods were occurring most often and caused the greatest total damage.
The Australian Institute for Disaster Resilience acknowledges the Traditional Owners of Country throughout Australia and recognises their continuing connection to land, waters and culture. We pay our respects to elders past, present and emerging. Explore Australian disasters using the interactive Disaster Mapper and browse historical records by ...
Reuse This Work. Disasters - from earthquakes and storms to floods and droughts - kill approximately 40,000 to 50,000 people per year. This is the average over the last few decades. While that's a relatively small fraction of all deaths globally, disasters can have much larger impacts on specific populations. Single extreme events can ...
103 Natural Disaster Essay Topic Ideas & Examples. Author: Luciano Colos. Published: Feb 01, 2024. Inside This Article. Natural disasters are events that occur in nature and can cause significant destruction and loss of life. They can take many forms, including hurricanes, earthquakes, floods, wildfires, and tsunamis.
Natural Disasters in Australia - Essay Example. Add to wishlist Delete from wishlist. Cite this document Summary. From the paper "Natural Disasters in Australia" it is clear that the human impact on the global climate system has been responsible for the climate and weather extremes since 1950 (IPCC 2013). One of the effects is an increase in ...
Disasters. Volume 8, Issue 2 p. 124-137. Australia's organized response to natural disaster: Background and theoretical analysis. Neil R. Britton, Neil R. Britton. Department of Behavioural Sciences and Centre for Disaster Studies James Cook University Queensland 4811 Australia. Search for more papers by this author. Neil R. Britton,
The folllowing sample essay on Natural Disasters In Zambia discusses it in detail, offering basic facts and pros and cons associated with it. To read the essay's introduction, body and conclusion, scroll down. The country has previously witnessed two major drought years in he last decade; 1991/92 and 1995/96.
500+ Words Essay on Natural Disasters. A Natural disaster is an unforeseen occurrence of an event that causes harm to society. There are many Natural disasters that damage the environment and the people living in it. Some of them are earthquakes, cyclones, floods, Tsunami, landslides, volcanic eruption, and avalanches.Spatial extent measures the degree or severity of the disaster.
Natural disasters are events that occur naturally such as earthquakes, cyclones, Storms, and floods. These kinds of disasters can cause significant physical damage, interruption of business services and personal injury/loss of life. Due to the effects of global warming and consequential climate change the rate of natural disasters in Australia ...