
An official website of the United States government
The .gov means it’s official. Federal government websites often end in .gov or .mil. Before sharing sensitive information, make sure you’re on a federal government site.
The site is secure. The https:// ensures that you are connecting to the official website and that any information you provide is encrypted and transmitted securely.
- Publications
- Account settings
The PMC website is updating on October 15, 2024. Learn More or Try it out now .
- Advanced Search
- Journal List
- Front Neurosci
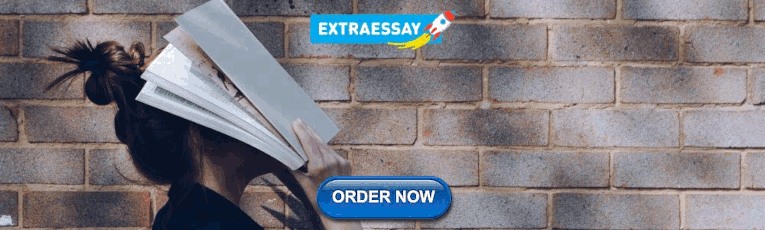
Zebra Finches As a Model Species to Understand the Roots of Rhythm
Michelle j. spierings.
1 Behavioural Biology, Institute Biology Leiden, Leiden University, Leiden, Netherlands
2 Leiden Institute for Brain and Cognition, Leiden University, Leiden, Netherlands
Carel ten Cate
Vocal timing in zebra finches.
Zebra finches are a widely used model species for neurobehavioral research, in particular in relation to song development and auditory processing. Males learn their songs from a tutor. Females don't sing, but do develop learned song preferences. Regardless of the differences, both sexes exchange calls in social interactions. Two fascinating recent studies looked at different aspects of rhythmicity in the production of zebra finch vocalizations (Benichov et al., 2016a ; Norton and Scharff, 2016 ), and together with several studies on the perception of rhythms, they make the zebra finch a promising model species to unravel the roots of rhythm production and perception.
Norton and Scharff ( 2016 ) analyzed the intervals from one note onset to the next one in both isolate and directed zebra finch songs. For each male they were able to derive an isochronous sequence of “time stamps” of which a subset aligned with all note onsets of a male's song. Moreover, these time stamps often also coincided with the transitions between phonetically different gestures within a complex note. This indicates that an isochronous rhythm might underlie zebra finch songs. Benichov et al. ( 2016a , b ) showed that both males and females can dynamically adjust the mutual timing of their calls. Individual birds were housed with a robotic zebra finch that emitted an isochronous call pattern. Within ample minutes the zebra finches adjusted their call rate to create a regular back-and-forth exchange with the robotic finch. The robot was then set to emit “jamming” calls that were produced at the moment when the zebra finch was most likely to respond. All zebra finches adjusted their call pattern to avoid the jamming calls, either by calling earlier, later or both earlier and later. Furthermore, when the robotic finch produced a pattern of alternating single and paired calls, the zebra finches timed their calls differently for the single compared to the paired calls, indicating they apparently detected the alternating pattern of the robot calls and used this to anticipate whether the next call would be single or paired. Interestingly, females performed better than males at these tasks (Benichov et al., 2016a ).
Benichov et al. ( 2016a ) next examined the role of the forebrain song system in the timing of calls. The female song system is reduced compared to the male system and lacks, for instance, the largest song nucleus, Area X. However, some nuclei of the song system are present in both sexes, like the RA, a nucleus related to the temporal aspects of zebra finch song learning. Lesioning the RA nucleus in zebra finches made them unable to correctly adjust the timing of their calls, although they were still responsive to the robotic finch. This indicates that for both males and females the RA is actively involved in call synchronization and vocal coordination. Further experiments showed that input to the RA from another forebrain nucleus, the HVC, was necessary to maintain call synchronization (Benichov et al., 2016a ). While Norton and Scharff ( 2016 ) did not examine the neural basis of the isochronous patterns they observed in male songs, they also suggest that it might originate from the activation pattern of HVC neurons firing to the RA. These HVC RA neurons fire in a rhythmic, clock-like pattern (Long and Fee, 2008 ). However, as the rhythms observed by Norton and Scharff are 3 to 10 times slower than the firing of these HVC neurons, they propose that additional mechanisms must operate to translate the clocklike firing into the higher complexity observed in the songs.
Whether animals can perceive beat and rhythmic patterns is a prominent question in relation to understanding the evolution of human musicality (Patel, 2006 , 2014 ; Fitch, 2013 ; Hoeschele et al., 2015 ; Honing et al., 2015 ) and Benichov et al. ( 2016a , b ) suggest that the link between the neural system involved in vocal learning and call synchronization provides the relation between beat perception and auditory-motor coordination as shown in a number of animal species. So, how do the above findings on the presence of rhythms in vocal production in zebra finches relate to studies that addressed the perception of rhythmic auditory patterns?
Recognizing regularity
Rhythm can be defined as a regular repeated pattern. In its simplest form it is an isochronous series of pulses, like the one produced by the robotic finch. More complicated rhythms can be created by repeating a heterochronous pattern, for example the repetition of single and double pulses as used in Benichov et al. ( 2016a ). Humans are skilled in perceiving various rhythms. We can easily detect whether a pulse or beat pattern is regular or irregular by integrating temporal information over a series of auditory events (e.g., Geiser et al., 2014 ). We thus have the cognitive ability to abstract a general, global, pattern from a string of sounds, enabling us to classify patterns as regular or not. However, the question whether or to what extent non-human animals are also able to integrate temporal information over a longer series of sounds to classify strings as being regular is still open. A telling example is a study by Hagmann and Cook ( 2010 ) that showed that pigeons were unable to learn to discriminate between an isochronous and an irregular pulse pattern. Pigeons are vocal non-learners and this might be the reason for their inability, as it has been suggested that vocal learning and vocal non-learning species might differ in this respect (Patel et al., 2009 ; Schachner et al., 2009 ; see also ten Cate et al. ( 2016 ) for a review on this relationship for birds). The rhythm perception by zebra finches in the study by Benichov et al. seems to support this view, as they show that the (vocal learning) zebra finches extracted the regularity of the call pattern and used this to time their calling. However, when we combine these findings with several other recent studies on zebra finch perception of regularity the picture becomes more complex.
Nagel et al. ( 2010 ) trained female zebra finches to discriminate between two different songs and showed that the females maintained the discrimination over a range of tempo changes. The songs were still categorized correctly up to a 25% speed increase or decrease. These results might indicate rhythm generalization by zebra finches. However, Nagel et al.'s conclusion was that zebra finches maintained the discrimination by attending to the spectral envelope of the songs. Attending to the sequence of local spectral features, rather than any timing pattern may have enabled the discrimination. Whether zebra finches do attend to regularity in the timing of songs was examined by Lampen et al. ( 2014 ). They compared ZENK expression in response to playback of rhythmic zebra finch songs, i.e., where all songs in a string had identical inter-element intervals, with the expression in response to arrhythmic songs, i.e., a string of songs in which inter-element intervals vary. Arrhythmic songs resulted in stronger ZENK expression in the caudomedial nidopallium (NCM), the caudomedial mesopallium (CMM), and the nucleus taeniae (Tn). This increased activity in auditory areas of the zebra finch brain might be related to the finding by Benichov et al. ( 2016a ) as, similar to their study, the repeated pattern in the regular song may have initiated predictive timing of the next song rendition, which would be lacking with the arrhythmic song.
Regularity or interval detection?
The above mentioned studies may indicate that zebra finches perceive “regularity” as such. However, as also noticed by Benichov et al. ( 2016b ) this need not be the case. The various findings may arise because repeated events with a fixed interval may give rise to a prediction for a next interval of the same absolute duration. So, when the birds respond to the robotic finch they can do so by attending to, and learning about, the absolute interval between successive robot calls, or by detecting other local contiguities of events (Benichov et al., 2016b ), without having formed some concept of “regularity.” The same accounts for the study by Lampen et al. ( 2014 ), in which the zebra finches could also have responded to identical consecutive intervals in a sound string.
If zebra finches can perceive regularity as such, one would expect that they are not only able to distinguish a regular from an irregular string, but also to transfer this distinction to strings with modified tempos. This has been tested in experiments in which van der Aa et al. ( 2015 ) trained zebra finches to discriminate between a set of regular, isochronous pulse strings and a set of irregular pulse strings. The irregularity in these strings was created by varying the duration of inter-pulse-intervals within a string. As expected from the studies discussed above, zebra finches of both sexes could learn to discriminate these strings. But this discrimination broke down when the zebra finches were tested with probe strings with novel tempo transformations. The birds seemed to distinguish and discriminate the different strings based on their specific inter-pulse-interval durations, suggesting that the zebra finches focused on local features, without attending to or learning about the global pattern of regularity-irregularity of the strings (van der Aa et al., 2015 ).
Another recent experiment on zebra finches used isochronous pulse strings with pulses of two types. These were present in a ratio of 1:3, with the rare type raised in both frequency and amplitude compared to the other (ten Cate et al., 2016 ). This variation was used to create a string with a fixed number of low tones between two high ones, creating a regular beat pattern, and a string with different numbers of low tones in each interval, making the beat pattern irregular. Again, the zebra finches discriminated these strings. They were next tested with strings in which the location of the beat within the strings or the duration of pulses and inter-pulse-intervals was changed. This revealed that here also, the zebra finches seemed to use different local features, like the inter-beat- or inter-pulse-intervals to distinguish the strings. However, some birds seemed to combine a sensitivity to such local features with one for the more global regularity (ten Cate et al., 2016 ). Nevertheless, the behavioral experiments so far do not indicate that zebra finches perceive the global pattern of regularity as such. We should therefore be cautious with concluding that the ability of zebra finches (and other non-human species) to distinguish regular from irregular sound patterns is similar to the ability of humans to detect rhythm. However, there may be a continuum among species ranging from those not being able to discriminate regular from irregular sounds up those able of beat detection in more complex rhythms (ten Cate et al., 2016 ).
Conclusion and outlook
The various studies discussed above show that zebra finches are very good at detecting fixed interval durations and can use preceding intervals to predict the next one in a string of sounds. This matches their ability to call and to produce songs with a fixed rhythmic periodicity. Furthermore, specific nuclei of the zebra finch forebrain song system (HVC, RA, CMM, and NCM) seem involved in producing and detecting rhythmicity. However, the results also call for further behavioral and neural studies on the links between perception and production of rhythmic patterns in the zebra finch. Are receivers sensitive to the rhythms underlying songs? And which brain areas are involved in the discrimination of the rhythmic patterns in the experiments of van der Aa et al. ( 2015 ) and ten Cate et al. ( 2016 )? Are these perceptual abilities and also the production of the song rhythms observed by Norton and Scharff ( 2016 ) affected by interfering with HVC and RA? The zebra finch has proven to be an excellent model species, and is very suitable for addressing these questions. However, we also need comparative studies on other species to understand the types of temporal patterns that birds can detect, how they do so and how this is related to vocal learning (see also Benichov et al., 2016b ). Ultimately, this may also shed light on the building blocks from which our human ability for rhythm perception and beat entrainment may have evolved.
This work was supported by NWO-GW, grant no. 360.70.452.
Conflict of interest statement
The authors declare that the research was conducted in the absence of any commercial or financial relationships that could be construed as a potential conflict of interest.
- Benichov J. I., Benezra S. E., Vallentin D., Globerson E., Long M. A., Tchernichovski O. (2016a). The forebrain song system mediates predictive call timing in female and male zebra finches . Curr. Biol. 26 , 309–318. 10.1016/j.cub.2015.12.037 [ PMC free article ] [ PubMed ] [ CrossRef ] [ Google Scholar ]
- Benichov J. I., Globerson E., Tchernichovski O. (2016b). Finding the beat: from socially coordinated vocalizations in songbirds to rhythmic entrainment in humans . Front. Hum. Neurosci. 10 : 255 10.3389/fnhum.2016.00255 [ PMC free article ] [ PubMed ] [ CrossRef ] [ Google Scholar ]
- Fitch W. T. (2013). Rhythmic cognition in humans and animals: distinguishing meter and pulse perception . Front. Syst. Neurosci. 7 : 68 . 10.3389/fnsys.2013.00068 [ PMC free article ] [ PubMed ] [ CrossRef ] [ Google Scholar ]
- Geiser E., Walker K. M. M., Bendor D. (2014). Global timing: a conceptual framework to investigate the neural basis of rhythm perception in humans and non-human species . Front. Psychol. 5 : 159 . 10.3389/fpsyg.2014.00159 [ PMC free article ] [ PubMed ] [ CrossRef ] [ Google Scholar ]
- Hagmann C. E., Cook R. G. (2010). Testing meter, rhythm, and tempo discrimination in pigeons . Behav. Process. 85 , 99–110. 10.1016/j.beproc.2010.06.015 [ PubMed ] [ CrossRef ] [ Google Scholar ]
- Hoeschele M., Merchant H., Kikuchi Y., Hattori Y., ten Cate C. (2015). Searching for the origins of musicality across species . Philos. Trans. R. Soc. B. 370 :20140094. 10.1098/rstb.2014.0094 [ PMC free article ] [ PubMed ] [ CrossRef ] [ Google Scholar ]
- Honing H., ten Cate C., Peretz I., Trehub S. E. (2015). Without it no music: cognition, biology, and evolution of musicality . Philos. Trans. R. Soc. B. 370 :20140088. 10.1098/rstb.2014.0088 [ PMC free article ] [ PubMed ] [ CrossRef ] [ Google Scholar ]
- Lampen J., Jones K., McAuley J. D., Chang S.-E., Wade J. (2014). Arrhythmic song exposure increases ZENK expression in auditory cortical areas and nucleus taeniae of the adult zebra Finch . PLoS ONE 9 :e108841. 10.1371/journal.pone.0108841 [ PMC free article ] [ PubMed ] [ CrossRef ] [ Google Scholar ]
- Long M. A., Fee M. S. (2008). Using temperature to analyse temporal dynamics in the songbird motor pathway . Nature 456 , 189–194. 10.1038/nature07448 [ PMC free article ] [ PubMed ] [ CrossRef ] [ Google Scholar ]
- Nagel K. I., McLendon H. M., Doupe A. J. (2010). Differential influence of frequency, timing, and intensity cues in a complex acoustic categorization task . J. Neurophysiol. 104 , 1426–1437. 10.1152/jn.00028.2010 [ PMC free article ] [ PubMed ] [ CrossRef ] [ Google Scholar ]
- Norton P., Scharff C. (2016). ‘Bird song metronomics’: isochronous organization of zebra finch song rhythm . Front. Neurosci. 10 : 309 10.3389/fnins.2016.00309 [ PMC free article ] [ PubMed ] [ CrossRef ] [ Google Scholar ]
- Patel A. D. (2006). Musical rhythm, linguistic rhythm, and human evolution . Music Percept. Interdiscip. J. 24 , 99–104. 10.1525/mp.2006.24.1.99 [ CrossRef ] [ Google Scholar ]
- Patel A. D. (2014). The evolutionary biology of musical rhythm: was Darwin wrong? PLoS Biol. 12 :e1001821. 10.1371/journal.pbio.1001821 [ PMC free article ] [ PubMed ] [ CrossRef ] [ Google Scholar ]
- Patel A. D., Iversen J. R., Bregman M. R. (2009). Experimental evidence for synchronization to a musical beat in a nonhuman animal . Curr. Biol. 19 , 827–830. 10.1016/j.cub.2009.03.038 [ PubMed ] [ CrossRef ] [ Google Scholar ]
- Schachner A., Brady T. F., Pepperberg I. M., Hauser M. D. (2009). Spontaneous motor entrainment to music in multiple vocal mimicking species . Curr. Biol. 19 , 831–836. 10.1016/j.cub.2009.03.061 [ PubMed ] [ CrossRef ] [ Google Scholar ]
- ten Cate C., Spierings M. J., Huberts J., Honing H. (2016). Can birds perceive rhythmic patterns? A review and experiments on a songbird and a parrot species . Front. Psychol. 7 : 730 10.3389/fpsyg.2016.00730 [ PMC free article ] [ PubMed ] [ CrossRef ] [ Google Scholar ]
- van der Aa J., Honing H., ten Cate C. (2015). The perception of regularity in an isochronous stimulus in zebra finches ( Taeniopygia guttata ) and humans . Behav. Process. 115 , 37–45. 10.1016/j.beproc.2015.02.018 [ PubMed ] [ CrossRef ] [ Google Scholar ]
Zebra Finches Dream a Little Dream of Melody
Zebra finches flex their singing muscles while snoozing, as if they’re lip-syncing in their sleep—and scientists want to know why
/https://tf-cmsv2-smithsonianmag-media.s3.amazonaws.com/accounts/headshot/10172852_10152012979290896_320129237_n.jpg)
Katherine J. Wu
Correspondent
/https://tf-cmsv2-smithsonianmag-media.s3.amazonaws.com/filer/40/36/4036d112-76e8-45f8-bcaf-83a121f49fe6/finches1.jpg)
Zebra finches are so good at singing, they could do it in their sleep. And, according to scientists, they do everything but: As they slumber, zebra finches fire up brain regions and vibrate their vocal cords in ways that mimic wakeful warbling.
In the past two decades, scientists have found that sleep is a critical part of the zebra finch song-learning repertoire. The finches aren’t born belting arias; instead, they learn their songs in adolescence , practicing and tinkering under the supervision of an adult tutor. The tutor will warble patiently for its students, who will then utter their own tentative vocalizations. Hearing their own voices seems to be a crucial element for little zebra finches as they test the notes and syllables out for themselves.
Certainly, practice makes perfect—but even more crucial? A good night’s sleep.
After a hard day at school, each zebra finch heads to bed with music on the mind—so much so that its brain will flicker in and out of the same patterns that occur during daily practice sessions. Its vocal cords vibrate, too, but don’t produce any sound: The birds aren't pushing enough air through their throats to generate audible noise.
Researchers theorize that the birds are, in effect, dreaming of song—more sleep lip-syncing than sleep singing. This may have a lot to do with crystallizing and reshaping material learned from their tutors—a phenomenon comparable to snoozing humans packaging memories and information into long-term storage . In the morning, the finches wake groggy and disoriented, babbling slightly less coherently than the previous day—but as time progresses, their songs improve.
Scientists think that studying how the birds use sleep to learn their songs can help us understand the mysterious process of human language acquisition. Like human speech, the melodies of birdsong are intricate and complex , and even activate brain pathways and muscles very similar to those in humans.
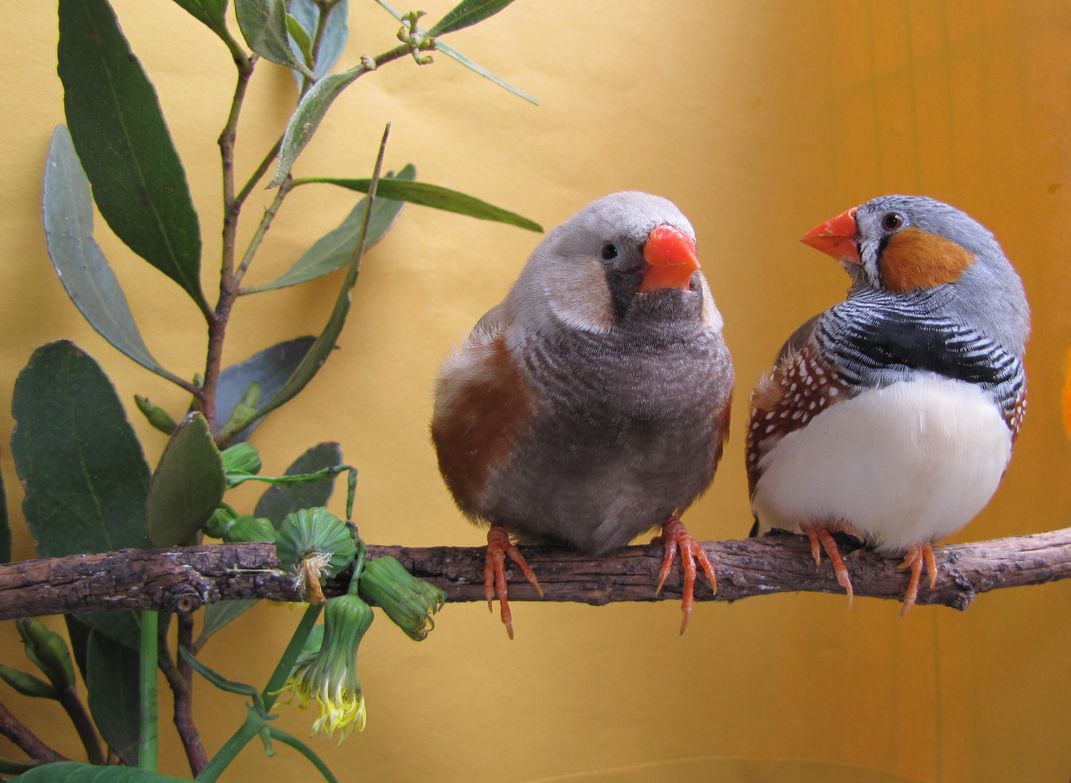
But studying the dreamscape of a slumbering zebra finch is no easy feat: It’s not like we can nudge the birds awake and ask. To better understand the inner workings of these drowsy birdbrains, Gabriel Mindlin’s research group at the University of Buenos Aires decided to move one step further in the chain of musical command. Brains control behavior, but there’s a muscley middleman between the two. To translate the pattern in the brain to a full-blown serenade, a bird’s vocal organ needs to first carry out the marching orders. Together with his colleagues, Mindlin, a professor of biophysics who studies the mechanics of birdsong, designed a system to directly measure the activity of the muscles involved.
In previous work , his research team, in partnership with scientists at the University of Utah , found that zebra finches move their vocal muscles in sync with song-like activity in the brain, indicating that the muscles’ readout could be a good proxy for peeking into the birds’ melodic reveries.
“Now instead of looking at one [brain] cell at a time, we can see the output of the whole system, and that’s very exciting,” says Daniel Margoliash , a professor of neurobiology at the University of Chicago who has studied birdsong for over three decades. Margoliash has previously collaborated with Mindlin’s group but was not involved in these studies.
The study also found that sleep wasn’t just a time to rehearse—it may also give the birds a chance to quietly improvise new tunes. The birds' vocal muscles appear to go slightly on the fritz at night, composing silent songs that don’t stick to the scripts of daytime. This new research was able to capture variation that previous studies of the brain couldn’t, and suggests that the musical mind meanders when zebra finches nod off.
For Mindlin, this was huge. “Now we have a model that doesn’t need the brain,” he says.
Last week, in two new studies, Mindlin’s group expands on their research. Both studies focus on the way scientists study the finches' vocal muscles, in hopes of unlocking more secrets of the slumbering birdbrain.
First, scientists led by Juan Doppler , a physicist working under Mindlin’s supervision, wanted to make it easier to study the birds' muscles. Focusing on the muscles may be a more direct way to capture the mechanics of the serenades of snoozing—but it still isn’t easy. Adult zebra finches must undergo surgery to allow scientists to attach electrodes to multiple muscles.
However, while these muscles are understood to directly control individual aspects of song production—for instance, the pitch of the sound produced—they also need to work together. Several are physically connected, including one particularly important muscle called the syringealis ventralis, which is known to control a song’s frequency.
The team found that measuring the activity of the syringealis ventralis alone could reliably capture the dynamics of birdsong ballads almost as accurately as the old technique of measuring multiple muscles. As expected, the muscle’s activity contained information about frequency, but could also predict when musical motifs started and stopped with over 70 percent accuracy.
“We are moving away from focusing completely on the brain and neurons, and also paying attention to biomechanics, where the information of the nervous system is processed,” says Doppler. “This is a powerful idea. In some cases, looking at the biomechanics can give you insights that are not so clear in the nervous system.”
With Doppler’s simpler system, described in the journal Chaos , the researchers can more easily study the birds’ dream-songs; what’s more, understanding the mechanics of this powerful muscle can also inform how the vocal system works as a functional unit.
“The features of the muscle really bleed through,” says Katherine Tschida , a neurobiologist at Duke University who has studied song learning in zebra finches. “You can get a readout from a single muscle [on] a lot of different features, despite the fact that the muscle isn’t primarily the functional driver of the system.” Tschida, who was not involved in the work, also praised the study for its “high quality” methodology.
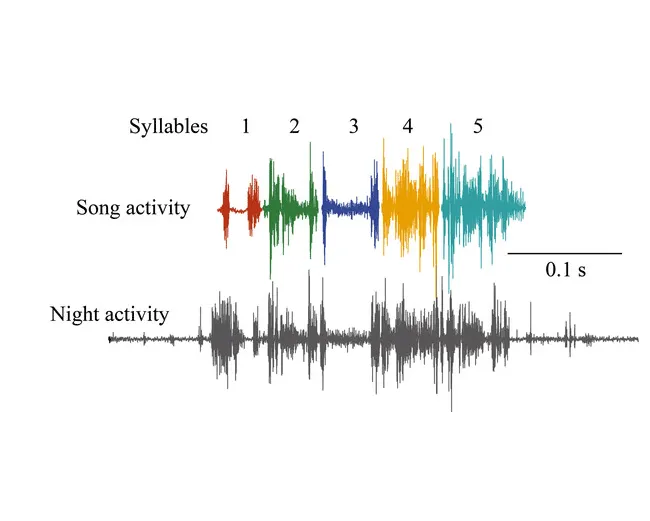
In the second study, a team led by Alan Bush , also a physicist in Mindlin’s group, found that they could manipulate the birds into flexing their vocal muscles by playing them versions of their own songs while they slept—a form of harmonic hypnosis. Bush was eager to study the patterns of how muscles actually fired in slumber. To him, the vocal organ is not just a puppet carrying out the brain’s set of master instructions—rather, it’s a creative liaison between brain and behavior that may add its own bells and whistles to the final product. “A lot of the complexity of the system is actually coming from the periphery, where the muscles are,” he explains.
Bush and his colleagues discovered that when the muscles are coaxed into activity, they behave in an all-or-nothing fashion. When played snippets of themselves trilling out tunes, the birds’ muscles would reliably twitch. Even synthetic versions of these songs, remixed in the lab, could sometimes elicit vocal organ responses. Often, the muscles were still—but when they were goaded into flexing, they would carry out the complete firing sequence of a vocalization.
The scientists say this knowledge opens up new ways to study how musical musculature is spurred into action.
Due to the invasiveness of the surgery, Mindlin and his team have only been able to test the muscle responses of adult zebra finches. However, future experiments with more advanced technology may shed light on young birds’ dream states. Such findings might corroborate previous research on how adolescent zebra finches toy with their tutor’s teachings and add their own personal touches during sleep. But what purpose could this serve in adults, who have already mastered their melodies?
Margoliash thinks it’s about maintaining expertise. “If you want to achieve a very high level of precision, you have to work to get there, and practice to stay there,” he explains. “Zebra finches—and humans—need to practice to maintain the quality of performance we achieve.”
Someday, with the combined forces of neuroscience and biophysics, scientists may actually be able to take a deeper dive into the songs of slumber. Technology hasn’t quite caught up to the task—but it’s becoming more powerful with each passing day. Until then? Dream on.
Get the latest Science stories in your inbox.
/https://tf-cmsv2-smithsonianmag-media.s3.amazonaws.com/accounts/headshot/10172852_10152012979290896_320129237_n.jpg)
Katherine J. Wu | | READ MORE
Katherine J. Wu is a Boston-based science journalist and Story Collider senior producer whose work has appeared in National Geographic , Undark magazine, Popular Science and more. She holds a Ph.D. in Microbiology and Immunobiology from Harvard University, and was Smithsonian magazine's 2018 AAAS Mass Media Fellow.
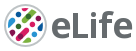
- Feature Article
- Neuroscience
The Natural History of Model Organisms: Neurogenomic insights into the behavioral and vocal development of the zebra finch

- Matthew IM Louder
- Simon C Griffith
- Department of Evolution, Ecology, and Behavior, School of Integrative Biology, University of Illinois at Urbana-Champaign, United States ;
- International Research Center for Neurointelligence, University of Tokyo, Japan ;
- Department of Biology, Texas A&M University, United States ;
- Department of Biological Sciences, Macquarie University, Australia ;
- Open access
- Copyright information
- Comment Open annotations (there are currently 0 annotations on this page).
- 2,485 views
- 226 downloads
- 13 citations
Share this article
Cite this article.
- Mark E Hauber
- Copy to clipboard
- Download BibTeX
- Download .RIS
- Figures and data
Introduction
An evolutionary history of the zebra finch, a model species for the analysis of sex differences in vocal learning and production, differences in captive vs. wild zebra finches and comparisons with northern hemisphere songbirds, genes and brains for vocal learning, the importance of studying female zebra finches, conclusions, data availability, decision letter, author response, article and author information.
The zebra finch ( Taeniopygia guttata ) is a socially monogamous and colonial opportunistic breeder with pronounced sexual differences in singing and plumage coloration. Its natural history has led to it becoming a model species for research into sex differences in vocal communication, as well as behavioral, neural and genomic studies of imitative auditory learning. As scientists tap into the genetic and behavioral diversity of both wild and captive lineages, the zebra finch will continue to inform research into culture, learning, and social bonding, as well as adaptability to a changing climate.
The zebra finch Taeniopygia guttata is the most intensively studied species of bird that is maintained in captivity in large numbers despite not being a species bred for its meat or eggs, like the chicken or the quail (reviewed in Zann, 1996 ). It became popular as a pet bird in the 19 th century because it bred well in captivity, and was adopted for scientific study in the third quarter of the 20 th century, initially for research into sexual behaviors ( Morris, 1954 ; Immelmann, 1972 ). Later, the zebra finch was used in studies of the de novo evolution of vocal culture (e.g. Fehér et al., 2009 ; Diez and MacDougall-Shackleton, 2020 ), the neuroethology of imitative vocal learning ( Terpstra et al., 2004 ; Vallentin et al., 2016 ; Yanagihara and Yazaki-Sugiyama, 2019 ), the neural mechanisms of sensorimotor learning ( Mandelblat-Cerf et al., 2014 ; Okubo et al., 2015 ; Mackevicius et al., 2020 ; Sakata and Yazaki-Sugiyama, 2020 ), and the role of early acoustic experience on the song-based preferences of female mate choice ( Riebel and Smallegange, 2003 ; Chen et al., 2017 ; Woolley, 2012 ; see the following video for a mating display in zebra finches: https://www.youtube.com/watch?v=TaC6D1cW1Hs ).
Due to the pronounced sexual differences in singing and plumage coloration found in the zebra finch ( Figure 1 ), earlier research quickly focused on when and how males learn to copy and produce a tutor(-like) song (e.g. Eales, 1987 ; Brainard and Doupe, 2002 ; Figure 2A ), and then eventually on how females learn from their (foster) fathers to prefer particular male vocal displays ( Braaten and Reynolds, 1999 ; Riebel, 2000 ). This allowed for the characterization and testing of the functions of male song and its female perception in the context of acoustic sexual dimorphism at the behavioral, endocrine, and neurophysiological levels (reviewed in Riebel, 2009 ; Hauber et al., 2010 ).
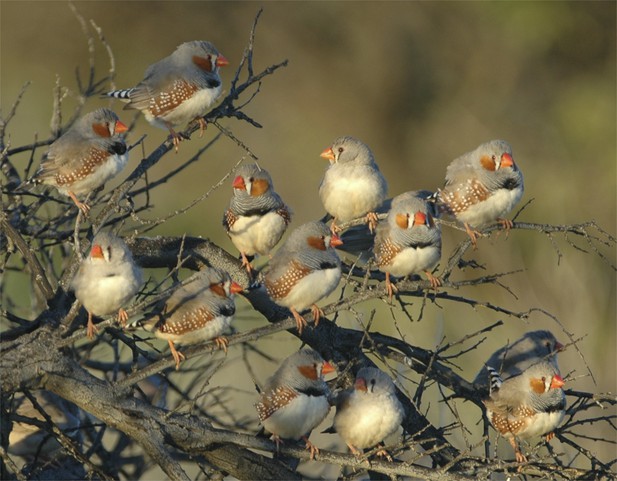
Adult zebra finches in the wild.
Four female and nine male adult zebra finches in the wild in Australia. As the species experiences increasingly extreme climatic fluctuations, future field studies of the zebra finch should also advance our understanding how opportunistically breeding species are able to adapt to accelerating climate change (photo credit: Simon C Griffith).
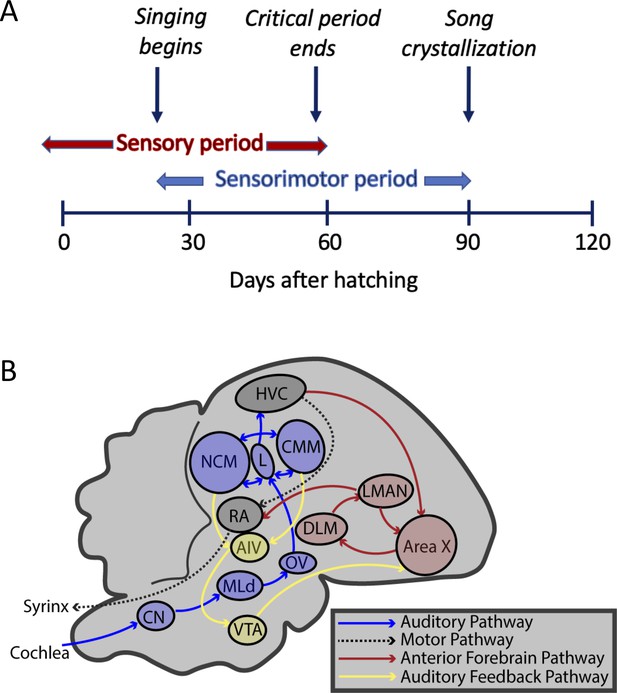
Timeline and brain pathways of auditory and vocal learning in the zebra finch.
( A ) Timeline of sensory (auditory learning) and sensory-motor (vocal self-assessment and song-production) critical periods in zebra finch song development. ( B ) Brain nuclei of male zebra finches for auditory learning (CN: cochlear nucleus; MLd: mesencephalicus lateralis pars dorsalis; OV: nucleus ovoidalis; field L: primary auditory forebrain input area; NCM: caudomedial nidopallium; CMM: caudomedial mesopallium; VTA: ventral tegmental area; and AIV: ventral portion of the intermediate arcopallium), vocal learning (HVC, Area X: basal ganglia; LMAN: lateral magnocellular nucleus of the anterior nidopallium; DLM: nucleus dorsolateralis anterior thalami, pars medialis), and vocal production (HVC, and RA: robust nucleus of the arcopallium).
The zebra finch was the second avian species to have its genome sequenced ( Warren et al., 2010 ), after the domestic fowl ( Gallus gallus ; International Chicken Genome Sequencing Consortium, 2004 ). Soon after the appearance of transgenic lines of domestic fowl and the Japanese quail Cortunix japonica (reviewed by Sato and Lansford, 2013 ), the first generations of transgenic zebra finches become available (e.g. Agate et al., 2009 ; Abe et al., 2015 ; Liu et al., 2015 ). The proven feasibility of genome editing in both developing zebra finches (e.g. Ahmadiantehrani and London, 2017 ) and adult poultry (reviewed in Woodcock et al., 2017 ), means that this bird may also be used as both a basic and an applied (i.e., biomedical) model for development and for human health and disease (e.g. Han and Park, 2018 ; London, 2020 ).
Studies of zebra finch natural history in Australia have been essential to establish and confirm the rationale for studying this species as a model for acoustic communication ( Zann, 1990 ; Elie et al., 2010 ), social behavior ( McCowan et al., 2015 ; Brandl et al., 2019a ; Brandl et al., 2019b ), reproductive physiology ( Perfito et al., 2007 ), life-long pair bonding ( Mariette and Griffith, 2012 ), and adaptations to heat ( Cade et al., 1965 ; Cooper et al., 2020a ; Cooper et al., 2020b ). Specifically, by understanding the natural history of the zebra finch, research in captivity can capitalize on the manipulation of the behavioral, neuroendocrine, and epigenetic bases of the bird’s phenotype, including conspecific brood parasitism, parent-offspring conflict, and sibling rivalry.
Finally, with Australia experiencing increasingly extreme climatic events and fluctuations, field studies of the zebra finch are also paving the way to understanding how this opportunistically breeding species is adapting to accelerating climate change. For example, recent wild studies have revealed the zebra finch's extensive behavioral and physiological plasticity to withstand extreme temperatures of over 40°C (e.g. Cooper et al., 2020a ; Cooper et al., 2020b ; Funghi et al., 2019 ). In turn, studies of captive zebra finches in controlled temperature conditions have already tested the effects of cool vs. hot climates on parental investment ( Nord et al., 2010 ), parent-offspring embryonic communication ( Mariette and Buchanan, 2016 ), offspring development ( Wada et al., 2015 ), tutor choice for song learning ( Katsis et al., 2018 ), adult phenotype (e.g. body size: Andrew et al., 2017 ), the level of DNA methylation ( Sheldon et al., 2020 ), and the effect of heat waves on sperm ( Hurley et al., 2018 ).
By tapping into the existing genetic and behavioral diversity of wild and captive lineages in zebra finches (e.g. Forstmeier et al., 2007 ; Knief et al., 2015 ) to perform comparative avian genomic analyses ( Jarvis et al., 2014 ; Feng et al., 2020 ), interspecific hybridization studies ( Woolley and Sakata, 2019 ; Wang et al., 2019 ), and direct genetic manipulations ( Liu et al., 2015 ; London, 2020 ), the zebra finch shall continue to serve as a focal subject of integrative research into human language-like vocal culture ( Hyland Bruno et al., 2021 ), auditory learning ( Theunissen et al., 2004 ), acoustically-mediated social bonding ( Tokarev et al., 2017 ), and genetic ( Balakrishnan et al., 2010 ) and behavioral (e.g. song) variability ( Lansverk et al., 2019 ; see Box 1 ).
Outstanding questions in zebra finch research.
Female zebra finches do not sing but have a diverse repertoire of cooperative calls and other social behavioral displays. What is the neurogenomic and ontogenetic basis of this lack of singing in females?
Can gene editing become standard practice in both ontogenetic and adult-onset manipulations of the genomic architecture and gene activational basis of focal zebra finch traits, including imitative song learning and auditory feedback in the maintenance of crystallized song production?
What is the genomic and transcriptomic mechanism of hair-cell regeneration in the songbird inner ear and can it be transferred to human hearing loss treatments?
What is the genomic and physiological basis of aseasonal reproduction in nomadic zebra finches?
The zebra finch is endemic to Australasia, and evolved there as part of the Australian grass finch radiation within the Estrildidae ( Olsson and Alström, 2020 ). The species shares a common ancestor with Poephila finches (long-tailed P. acuticauda ; black-throated P. cincta ; and masked finch P. personata ), diverging around 2.9 million years ago ( Singhal et al., 2015 ). Formerly, the zebra finch was placed in a genus with the double-barred finch ( Taeniopygia bichenovii ), but in fact these two lineages diverged around 3.5 million years ago ( Singhal et al., 2015 ).
Two subspecies of the zebra finch are recognized, with the continental Australian taxon ( T. guttata castanotis ) having no clear genetic structure and apparently mating randomly within its breeding population ( Balakrishnan and Edwards, 2009 ). The other subspecies is the Timor zebra finch ( T. g. guttata ), found to the north of Australia. The genetic divergence between the two lineages suggests that the latter taxon colonized the Lesser Sunda Islands around 1 million years ago and has a reduced diversity and genetic distance driven by founding effects and selection, relative to the continental subspecies ( Balakrishnan and Edwards, 2009 ). The insular subspecies has also been occasionally studied in captivity, and it differs from the continental Australian subspecies in morphological and behavioral traits, including song rate and mate choice ( Clayton, 1990 ; Clayton et al., 1991 ).
The two subspecies of the zebra finch are physically isolated from one another in the wild, but they can readily hybridize and be back-crossed in captivity to examine a range of questions in classical genetics and functional developmental biology. To date, this approach has seen limited application, with just one study looking at the divergence in gene regulation between the two subspecies ( Davidson and Balakrishnan, 2016 ). Whilst this direction could provide an extremely valuable new research opportunity, a major logistical challenge to overcome will be the capture and export of birds from Indonesia, or the continued maintenance of distinct (non-hybrid) domesticated populations of T. g. guttata in captivity.
Zebra finches have a relatively short generation time for altricial birds (those that are underdeveloped at the time of hatching): they become sexually mature at between 90 and 100 days of age in captivity, at which point they are ready to form pair bonds, build nests, and breed ( Zann, 1996 ). They are highly social and can be kept at great densities in shared housing with a relative absence of highly antagonistic behaviors. This is likely to be related to the level of sociality and the highly fluid flock-wide social relationships seen in the wild ( McCowan et al., 2015 ; Brandl et al., 2019a ), as individuals congregate around food and water, and nest in close proximity in loose colonies for apparent social benefits ( Brandl et al., 2019b ).
Provided with sufficient water, nesting sites, and nest materials, and one (or more) mate(s) of the opposite sex, zebra finches can successfully reproduce on a predominantly seed-based diet, simplifying husbandry, even during the nestling stage. Indeed, under a broad range of environmental and social conditions in captivity, when given the infrastructure (e.g. nesting platform or cavity and materials) to breed, most pairs will breed successfully within a short time frame ( Griffith et al., 2017 ), and the life history can be followed across many generations in a relatively short period of time (e.g. Briga et al., 2019 ).
With a clutch size of between 2 and 9 eggs (mode: 5), and with brood reduction rates that can be less than 30%, each reproductive bout is typically rapid and productive. In the wild, zebra finches pair for life, and partners are found in close proximity during both the breeding and non-breeding periods ( Mariette and Griffith, 2012 ; McCowan et al., 2015 ). In captivity, this strong pair bond is preceded by rapid pairing, with singletons forming pair bonds within days or weeks when introduced into a new cage or aviary ( Rutstein et al., 2007 ; Campbell et al., 2009 ). The strength of the pair bond, the high levels of affiliative behaviors, and the relative absence of antagonism between partners also allow zebra finches to be kept in easily monitored single-pair cages, rather than in communal aviaries ( Zann, 1996 ).
However, it was not just ease of breeding in captivity that turned the zebra finch into a popular model for studying the development of sexual dichromatism and vocal dimorphism. Rather, an initial interest in the distinct plumage and the vocal differences between adult female (drabber, non-singing) and male (more colorful, singing) zebra finches resulted in several, now classic, developmental studies. Some of these studies concentrated on the role of early life experience, through chromatic and vocal sexual imprinting, on females choosing attractive males as mates, while others focused on song production and song preference learning by male and female zebra finches (e.g. Clayton, 1987 ; Eales, 1987 ). For example, cross-fostering zebra finch chicks with the ‘universal estrildid foster species’, the Bengalese finch ( Lonchura striata vars. domestica ; Sonnemann and Sjölander, 1977 ), revealed that both visual and acoustic cues of social parents are learned during early development and used by young zebra finches of both sexes in mate preference following maturity ( ten Cate, 1987 ; Campbell and Hauber, 2009 ; Verzijden et al., 2012 ). This occurs through a two-stage process of sexual imprinting ( ten Cate, 1985 ; ten Cate and Voss, 1999 ).
These ontogenetic, physiological, and behavioral studies since the last quarter of the 20 th century (e.g. Price, 1979 ) have become increasingly coupled with the rapid advances of neuroanatomical and neurophysiological imaging, genome sequencing, and transcriptomic and epigenetic analyses of the neural circuitries of song production in the forebrains of songbirds (reviewed in Mooney, 2009 ; Mooney, 2014 ) and song perception (reviewed in Louder et al., 2019 ). For instance, neurophysiological ( Hauber et al., 2013 ), neuroanatomical ( Lauay et al., 2005 ), immediate-early gene ( Tomaszycki et al., 2006 ), and transcriptomic analyses ( Louder et al., 2018 ) performed on zebra finch females that were reared either in isolation from any male birdsong or in the presence of a different songbird species have confirmed the critical role of early life experience in generating adaptive cognitive-behavioral ( Price, 1979 ), neurogenomic ( Louder et al., 2018 ) and neurophysiological ( Moore and Woolley, 2019 ) responses to conspecific songs. Similarly, the known upregulation of stress responses of formerly pair-bonded, but then separated captive zebra finches ( Remage-Healey et al., 2003 ), is also reported to impact the epigenomic status of similarly treated birds ( George et al., 2020 ).
Despite the earlier prominence of the domestic canary ( Serinus canaria ) in the neurobiological study of song learning, two other research themes have also benefited significantly from follow-up studies of captive zebra finches. First, adult-onset neurogenesis, accompanying seasonal changes in song behavior, or damage to the underlying neural circuitry, was initially extensively studied in the canary (e.g. Nottebohm, 1981 ), but with ongoing critical contributions also coming from experiments on zebra finches (e.g. Walton et al., 2012 ; reviewed in Pytte, 2016 ). For example, when adult male zebra finches’ RA- (robust nucleus of the arcopallium) and Area X-projecting HVC neurons ( Figure 2B ) were experimentally ablated, only the RA-projecting neurons were regenerated ( Scharff et al., 2000 ). In turn, a new social environment (e.g. through the exposure to novel aviary mates: Barnea et al., 2006 , and/or ongoing auditory experiences: Pytte et al., 2010 ) may also contribute to the diminished apoptosis of newly generated caudomedial nidopallium (NCM) neurons ( Figure 2B ) in the forebrains of adults.
Second, hair cell regeneration following a loud noise or antibiotic treatment in both Bengalese ( Woolley and Rubel, 2002 ) and zebra finches ( Dooling and Dent, 2001 ) occurs rapidly, as it does in other, non-oscine birds ( Stone and Rubel, 2000 ) and in some other vertebrate lineages (e.g. fish: Monroe et al., 2015 ). Research into such auditory system regeneration abilities in birds and other animals had strongly promised, but has thus far evaded, broadly applicable biomedical solutions for curing cell-death based hearing losses in humans ( Brigande and Heller, 2009 ; Menendez et al., 2020 ).
Most of the populations of zebra finches in research laboratories around the world have been founded with birds held by aviculturists for over a hundred generations ( Zann, 1996 ; Griffith et al., 2017 ). These populations have therefore been subject to both direct and indirect forms of natural and artificial selection, as well as founding effects, genetic drift, and inbreeding ( Forstmeier et al., 2007 ; Knief et al., 2015 ). It has long been known that birds of the domesticated stocks are up to 30% larger in body size than their wild counterparts ( Zann, 1996 ), but reassuringly they appear to be similar with respect to several life history trade-offs, including, for example, slow juvenile feather development and low adult song rates when nestlings are raised in large brood sizes (e.g. Tschirren et al., 2009 ). Captive birds are also similar to their wild counterparts in respect to the genomic architecture underlying complex traits ( Kim et al., 2017 ; Knief et al., 2016 ; Knief et al., 2017a ), although some caution still needs to be applied, for instance, to known differences in linkage disequilibrium patterns within the genomes of captive and wild populations ( Knief et al., 2017b ).
The pattern of zebra finches being quite different from many of the species of small passerines that are well studied by researchers in the northern hemisphere may be of greater significance than the differences between captive and wild populations of zebra finches. The zebra finch is an estrildid ( Sorenson et al., 2004 ; Olsson and Alström, 2020 ), a family that is endemic to the tropics, and found across Africa, Southern Asia, and Australasia – with the whole lineage having evolved far from the ecological and evolutionary pressures of the temperate northern hemisphere. One of the almost ubiquitous characteristics of the estrildid family is the interseasonal strength of the socially monogamous pair-bond and biparental care for the young ( Payne, 2010 ).
Prior breeding experience enhances the success of subsequent breeding bouts by female zebra finches through increased output and shorter times between clutches, even when breeding with a new male in this otherwise lifetime pair-bonded species ( Adkins-Regan and Tomaszycki, 2007 ; Smiley and Adkins-Regan, 2016 ; Hurley et al., 2020 ). Relatively high within-pair sexual fidelity and cooperation in nest building, incubation, and provisioning also allow for the directed breeding of known pairs both in large aviaries and in small single-pair cages. Nevertheless, in socially housed groups, both conspecific brood parasitism – inducible by simulated nest predation in captivity ( Shaw and Hauber, 2009 ) and accounting for 5 to 11% of offspring ( Griffith et al., 2010 ) – as well as extra-pair paternity – accounting for around 30% of offspring in aviaries ( Forstmeier et al., 2011 ) – can partially confound social parentage, although extrapair paternity is almost entirely absent in the wild (accounting for ~1% of offspring; Griffith et al., 2010 ).
A major effort of laboratory-based work on the zebra finch has focused on females’ mate choices (especially with respect to beak color and learned song; Griffith and Buchanan, 2010a ). However, despite considerable variance in the reproductive success of individuals even in captive populations ( Griffith et al., 2017 ; Wang et al., 2017 ), one of the most comprehensive studies examining the consequence of mate choice on fitness found no evidence that either males or females are targeting this variation in individual quality when they choose a partner ( Wang et al., 2017 ). This finding supports the idea that the strength of a partnership is of greater value than the intrinsic quality of the individuals involved.
In this respect, zebra finches may differ from similarly-sized well studied small passerines of the northern hemisphere temperate zone. Since adult zebra finches are likely to live between 3 and 5 years in the wild ( Zann, 1996 ) and can breed continuously throughout the year if conditions are favorable ( Griffith et al., 2017 ), they can potentially accrue considerable experience as part of the sexual-parental partnership. The reproductive benefits of better physiological and behavioral coordination between partners (e.g. Adkins-Regan and Tomaszycki, 2007 ; Smiley and Adkins-Regan, 2016 ; Hurley et al., 2020 ) may outweigh the benefits of frequent and repeated partner switching and genetic infidelity ( Griffith, 2019 ). In turn, the value of the partnership may promote selection for diverse affiliative and cooperative traits, not always seen in the widely studied passerines of the more seasonally constrained northern hemisphere, where most individuals breed just once or twice in a lifetime ( Griffith, 2019 ). Rather, these traits are reminiscent of the long-term cooperative breeding partnerships formed (and the fitness costs paid following divorce or mate loss) by long-lived biparental seabirds (e.g. Ismar et al., 2010 ).
Indeed, the strength of the pair bond in the wild zebra finch is seen in the expression of acoustic communication throughout the year, and high levels of coordinated duetting between the male and female ( Elie et al., 2010 ). This close, and regular vocal interaction between the members of a pair also perhaps plays a role in individual vocal recognition in this species ( Levréro et al., 2009 ; Elie and Theunissen, 2018 ; Yu et al., 2020 ).
Highly coordinated acoustic interactions between female and male partners are a characteristic of the earliest passerine lineages as they had evolved in Australia ( Odom et al., 2014 ). The continuously high level of overall acoustic activity in the zebra finch, which has made it such an attractive model system for neurobiology, sets it apart from many other well studied passerines in the northern hemisphere. This serves to remind us that although most of the laboratory work is conducted in the northern hemisphere, the zebra finch is, in many respects, different from most of the short-lived highly seasonally breeding passerines native to the temperate zone of the northern hemisphere. Indeed, it is important to understand that the species’ adaptations to the highly unpredictable Australian climate and ecology – while making it so easy to maintain and breed in captivity – also set it apart from most other northern hemisphere lineages that could not be used in laboratories to anywhere near the same extent.
The process through which developing young memorize the acoustic communication signals of adults in humans and songbirds has been a critical research rationale and funding source supporting zebra finch studies. The learning of adult male songs by juveniles is particularly strong during early sensory periods, when embryos ( Antonson et al., 2021 ), nestlings ( Rivera et al., 2019 ), and juveniles ( Brainard and Doupe, 2000 ) likely form a sensory representation of the 'tutor song' ( Figure 3 ). Just as juvenile females develop long-term song-type preferences used for mate choice based on early experiences with their own fathers ( Riebel, 2000 ; Chen et al., 2017 ), young males also learn and then actively practice to produce songs that match their paternal (tutor) songs ( Tchernichovski et al., 2001 ; Figure 3 ). Tutors even alter their song structure when singing near young tutees, which influences the song learning process for young zebra finches, analogous to humans changing their speech when speaking to infants ( Chen et al., 2016 ; Carouso-Peck and Goldstein, 2019 ).
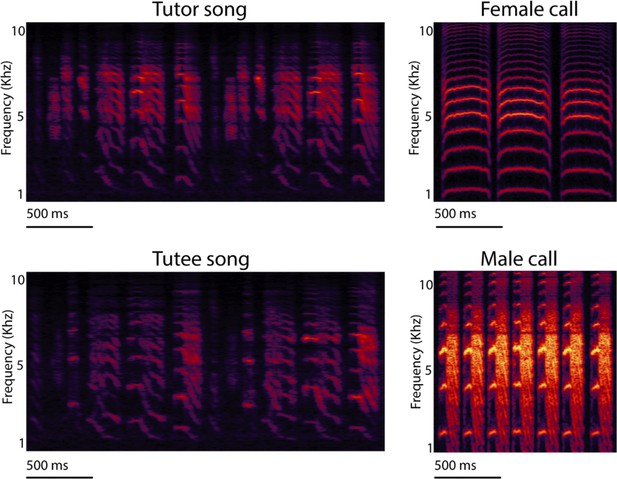
Spectrograms of zebra finch songs and calls.
Spectrogram of tutor and tutee adult male zebra finch songs, and undirected contact calls of adult females and males. Spectrograms represent time (x-axes) and pitch (y-axes) with greater amplitude as increasing brightness. Note the similarity of the tutor (typically social father) and tutee (son) song pair of male zebra finches and the distinct sexual differences of the calls.
However, even in the case of strong social environmental impact upon song learning during the sensitive period, the genetic make-up of individuals may contribute to the resulting song preferences and vocal production patterns through gene-by-environment interactions ( Mets and Brainard, 2019 ). Accordingly, in zebra finches, males preferentially learn to sing from song tutors of the same species over those of another species when given equal access ( Clayton, 1988 ), and both song-naïve and cross-fostered females show greater neuronal spike rates in response to unfamiliar conspecific over an unfamiliar third species’ songs ( Hauber et al., 2013 ). Similarly, the species-specific typical pattern of socially learned song structure can culturally evolve across of just a handful of generations in initially naïve zebra finch populations ( Fehér et al., 2009 ; Diez and MacDougall-Shackleton, 2020 ).
In adulthood, male and female zebra finches can quickly memorize individual vocal characteristics and recognize the identity of others for at least a month without reinforcement ( Yu et al., 2020 ), likely relying on the perception of extremely small differences in calls and songs ( Prior et al., 2018 ). However, experiences with other songs in adulthood do not affect the crystallized songs of males. Given the parallels with language acquisition and speech development in humans, zebra finches have thus long served as an important model for studying the neural mechanisms that control how vocal signals are memorized and copied ( Doupe and Kuhl, 1999 ).
Initial research in the neurobiology of songbirds, primarily with canaries, has revealed the components and plasticity of the neural loops and circuits responsive to learning and producing songs ( Figure 2B ). Over time, studies of the zebra finch (a species that crystallizes its specific song once and does not deviate from it unless experiencing trauma or training) have become increasingly more instructive in the pursuit of identifying where in the forebrain the auditory memories are stored and how this representation directs both vocal learning in males and mate choice preferences in females (reviewed in Hauber et al., 2010 ). Accordingly, following the presentation of tape-recorded songs of conspecifics, the expression level of an immediate-early gene, egr-1 (also known as ZENK), which is associated with neural activation, increases within the zebra finch auditory forebrain, as found in other songbird species ( Mello et al., 1992 ; Louder et al., 2016 ).
Furthermore, neural responses within the NCM, a subregion of the auditory forebrain, are selective for tutor songs ( Yanagihara and Yazaki-Sugiyama, 2016 ) and song-induced expression of neural transcription factors (again, ZENK) also positively correlate with the increased similarity of the bird’s copied song to that of the tutor ( Bolhuis et al., 2000 ), which together suggest that this region may hold the tutor song’s memory. Accordingly, NCM lesions in adult male zebra finches reduce their ability to recognize songs, but not to produce them ( Gobes and Bolhuis, 2007 ). In female zebra finches, on the other hand, behavioral preferences for conspecific versus heterospecific songs can be eliminated by damaging the nearby CMM nucleus (caudomedial mesopallium) ( MacDougall-Shackleton et al., 1998 ).
Overall, the zebra finch remains the best model system to characterize the neural circuitry involved in vocal learning and production, with an often-stated research aim to better understand the capacity of imitative speech learning in humans (e.g. Lipkind et al., 2013 ). Juvenile male zebra finches mimic the tutor song while females only produce non-learned ‘calls’ ( Figure 3 ). In turn, several regions in the zebra finch brain associated with song production are dramatically larger in male zebra finches, a result of neurons in some of these regions atrophying in females while increasing in size and connections in males ( Figure 2B ; Konishi and Akutagawa, 1985 ). Several of these regions selectively respond to the ‘bird’s-own-song’ in anesthetized males ( Doupe and Konishi, 1991 ), which initially suggested a specialized function for this circuit in producing songs; however, the role of such own-song specific auditory responses is no longer clear, as they are gated by behavioral states ( Hessler and Doupe, 1999 ) and much less pronounced in awake birds ( Schmidt and Konishi, 1998 ).
The premotor circuit for song production receives input from auditory nuclei via the HVC, which then projects to the RA, and subsequently connects to the brainstem motor nuclei and syrinx ( Figure 2B ). This ‘motor pathway’ is crucial during the learning process ( Aronov et al., 2008 ) to generate stereotyped adult songs ( Simpson and Vicario, 1990 ). In turn, while singing, neurons in the HVC that connect to the robust nucleus of the arcopallium (RA) perform time-locked bursts of firing, coincident with precise sequences during the song ( Hahnloser et al., 2002 ). HVC neurons also ontogenetically shift their spike rates to become increasingly sparser while producing the male’s song ( Okubo et al., 2015 ), whereas the spike trains of RA neurons lock into the timing of song’s note identity ( Ac and Margoliash, 2008 ). By altering the local temperature of specific brain nuclei, Long and Fee, 2008 demonstrated that the temporal match between HVC, but not RA, and the song‘s timing pattern is a causal link, as cooling the HVC, but not the RA, slows down the song without affecting its frequency content. This demonstrates how and which elements of this forebrain circuit are critical to controlling the temporal structure of male songs and, in the Bengalese finch, their syntax, too ( Zhang et al., 2017 ). By contrast, the anterior forebrain pathway (AFP), homologous to the mammalian basal ganglia–thalamocortical pathway, is required for vocal learning in juvenile male zebra finches, but not the production of stereotyped adult song ( Bottjer et al., 1984 ). In this pathway, Area X and the lateral magnocellular nucleus of the anterior nidopallium (LMAN) are involved in producing song variability in juvenile birds during vocal learning ( Woolley and Kao, 2015 ; Figure 2B ).
Specifically, both theoretical modelling (including in humans) and experimental studies of this pathway (in zebra finches) have pointed to the critical role of vocal motor variability as the substrate upon which trial-and-error learning through reinforcement mechanisms may operate to shape vocal production ontogeny ( Dhawale et al., 2017 ). In turn, the AFP is also involved in auditory-feedback based acoustic correction signaling for the motor pathway, in that inactivation of LMAN in young male zebra finches regresses experimentally induced, recently learned changes in the subjects’ song pitch ( Andalman and Fee, 2009 ). Finally, gene expression patterns, including genes associated with speech in humans such as the transcription factor FOXP2, are highly expressed in the anterior forebrain pathway during sensitive periods for song learning, indicating potential genetic parallels of vocal plasticity in birds and humans ( Haesler, 2004 ; Pfenning et al., 2014 ).
How the memorized tutor song instructs vocal pathways remains unclear. However, research in the zebra finch points to the involvement of nuclei within and outside of the anterior forebrain pathway. Auditory feedback, in which self-uttered and self-heard vocalizations are compared to a memorized song pattern, is necessary for the development of song in juveniles and the maintenance of song in adult zebra finches ( Price, 1979 ; Nordeen and Nordeen, 1992 ; Leonardo and Konishi, 1999 ). Dopaminergic neurons of the ventral tegmental area (VTA) that project to the anterior forebrain pathway through Area X encode perceived errors in song performance from auditory feedback ( Gadagkar et al., 2016 ; Figure 2B ). The VTA receives error signals from auditory feedback through the AIV, which receives connections from the auditory forebrain ( Kearney et al., 2019 ). Furthermore, neurons within the auditory forebrain also demonstrate sensitivity to errors in auditory feedback ( Keller and Hahnloser, 2009 ). Such developments, for example regarding error sensitivity, also illustrate how ongoing research and continued breakthroughs in zebra finch neuroscience hold promise to further identify and understand the neural basis of vocal learning and production in general.
Following the widespread use of immediate early gene studies (see above), some of the research efforts aiming to characterize the genes that regulate zebra finch vocal and auditory behaviors, in particular genes related to vocal production in the brain, were based on utilizing DNA microarrays ( Wada et al., 2006 ). Then, in 2010 an international consortium sequenced, assembled, and annotated the first zebra finch genome ( Warren et al., 2010 ), only the second avian genome presented. This effort revealed the sequences of over 17,000 predicted protein-coding genes, as well as many regulatory regions and non-coding RNAs. More importantly, the annotated genome enhanced the next decade’s analyses into identifying the genes and regulatory networks that are involved in social behavior, including genome-wide investigations into vocal learning, such as auditory-experience induced RNA expression ( Louder et al., 2018 ), microRNA expression ( Gunaratne et al., 2011 ), and epigenetically regulated genes associated with developmental song learning ( Kelly et al., 2018 ). Furthermore, the initial genome helped researchers to identify and map the expression patterns of ~650 candidate genes within the brain of zebra finches, resulting in an online atlas database that provides an opportunity to link behavior, neuroanatomy, and molecular function ( Lovell et al., 2020 ).
A recent high quality, second generation genome of the zebra finch, presented as part of the Vertebrate Genomes Project, improves the accuracy of the reference genome assembly and annotation ( Rhie et al., 2021 ). Leveraging recent technological advances, such as long-read sequencing (up to 100 Kbp) and approaches to detect how DNA interacts across genomic loci (up to 100 Mbp), the latest updated zebra finch genome thus resolves numerous regions with repetitive elements and enhanced gene annotation from the first assembly.
In parallel with genomic advances, a suite of new neurobiological techniques available for zebra finches will only continue to increase the ability to understand the development of vocal learning and behavior. Questions regarding the activity of specific neurons can now be tackled using multi-electrode arrays (e.g. Lim et al., 2016 ; Tanaka et al., 2018 ) or wireless neurotelemetry ( Ma et al., 2020 ) able to simultaneously record the activity of numerous neurons in awake and freely-behaving birds. Imaging the neural connections between distant brain regions is now also possible with tissue clearing and light-sheet microscopy ( Rocha et al., 2019 ).
The experimental regulation of the expression of candidate genes in targeted areas of the zebra finch brain has also recently become available. Existing or new gene constructs can be inserted into neonatal (hatchling) zebra finches via electroporation-based gene construct delivery to study the genetics of vocal learning as songs are memorized, practiced, and first expressed by young males ( Ahmadiantehrani and London, 2017 ). Similarly, genetically modified constructs of nonpathogenic viruses injected in the brain, such as adeno-associated virus (AAV), are able to drive the expression of certain genes.
Viral constructs were developed to control the expression of FOXP2 (e.g. Heston and White, 2015 ; Norton et al., 2019 ), which is expressed in the song control regions within the male zebra finch forebrain and associated with inherited speech and language disorder in humans ( Fisher and Scharff, 2009 ). Viral constructs have also been useful in imaging, such as expressing a genetically encoded calcium indicator (GCaMP6s) for calcium imaging of neuron populations with 2-photon microscopy ( Picardo et al., 2016 ) or the expression of green fluorescent protein (GFP). Recent applications of viral constructs have also enabled researchers to control neurons with light (optogenetics), such as ‘implanting’ artificial song memories into the zebra finch brain ( Zhao et al., 2019 ), or controlling the firing of specific neurons, such as the VTA neurons that project to Area X ( Xiao et al., 2018 ; Kearney et al., 2019 ). Harnessing these new techniques enables us to tackle how genetic pathways are linked to vocal learning and motor control circuits.
However, the utility of the zebra finch as a neurogenetic model laboratory species has been somewhat inhibited by the low success rate in the development of transgenic lines that would enable direct experimental modification of the gene expression patterns in the relevant vocal-production and vocal-perception circuits. This may be due to the unique immune function of oscine birds inhibiting full viral delivery of gene constructs ( London, 2020 ). Nevertheless, the last decade has already seen the successful innovation of lentiviral delivery (e.g. Norton et al., 2019 ) of, for example, human Huntington’s Disease genes into zebra finch lineages, to causally demonstrate reduced vocal imitation and output consistency as a result of the treatment ( Liu et al., 2015 ). However, to date neither a TALEN nor a CRISPR/Cas9 vector-based gene editing approach has taken off in avian (chicken or songbird) lineages ( Woodcock et al., 2017 ; but see Cooper et al., 2018 ). With additional research, the zebra finch could be further explored as to which gene delivery and genomic editing methods will be widely and effectively applicable to this species.
Female zebra finches only slowly and partially assumed a role in some of the earlier behavioral and developmental studies on sexual imprinting (e.g. Collins et al., 1994 ), but now maintain a co-lead position. This is because mate choice is mutual in this species and females participate in the ever-important initial pair-bonding decisions, as well as in all aspects of collaborative biparental care ( Riebel, 2009 ). As such, females make a critical contribution to the phenotype of their offspring through their investments into eggs, and the care of dependent offspring ( Griffith and Buchanan, 2010b ). Still, in studying the neurobiological basis of species and mate recognition, and the relevant funding and publications, female-focused research took a secondary role during the earlier decades when much of the work focused on the developing and adult sensory-motor circuitries of the male zebra finch forebrain.
In the last two decades, however, there has been a definite upsurgence of studies focusing on female zebra finches, both from the perspective of the neurosensory-ontogenetic processes of conspecific ( Theunissen et al., 2004 ; Woolley et al., 2010 ), mate ( Lauay et al., 2004 ; Tokarev et al., 2017 ), and individual recognition ( Vignal et al., 2004 ; D'Amelio et al., 2017 ; Yu et al., 2020 ) by and of females. It is becoming clear that female visual and acoustic displays serve an important role in the development and fine-tuning of male vocalizations during sensitive periods ( Benichov et al., 2016 ; Carouso-Peck and Goldstein, 2019 ) and that male vocal and/or visual displays serve in the activation of auditory forebrain regions in adult females ( Avey et al., 2005 ; Day et al., 2019 ).
For example, the reduced volume of the song control system that exists in the female zebra finch brain is likely not at all vestigial ( Shaughnessy et al., 2019 ) and may be even more functional than previously thought, enabling plasticity in the vocal timing of calls in social interactions ( Benichov et al., 2016 ). In turn, female (and male) parental vocal communication with embryos in ovo in the nest have also been discovered to shape not only the functional neurogenomic responses of the embryos themselves ( Rivera et al., 2019 ) but also the acoustic tutor choice of young male zebra finches ( Katsis et al., 2018 ), as well as adult behavioral phenotypes and reproductive success ( Mariette and Buchanan, 2016 ).
Finally, the behavioral, the neurophysiological and gene-activational bases of perceptual learning of conspecific song features appear to be both species-specific in song-naïve (mother-only parent raised) female zebra finches and dependent on early social experience with con- or cross-fostered heterospecific male songs ( Hauber et al., 2013 ; Louder et al., 2018 ). Some of these latter discoveries in females have been made possible through cross-fostering nestling zebra finches with estrildid finch tutors of other species (e.g. Clayton, 1987 ). Critically, the results from females have now also been both replicated and advanced in cross-fostered males. Specifically, the extent of heterospecific song learning in males can be directly measured by the altered songs that they produce following experimental manipulation of early song exposure, and compared with the extent of neurophysiological response selectivity for conspecific (innate) vs. heterospecific (learned) tutor songs and their contributory bioacoustic features in the brain ( Moore and Woolley, 2019 ). In turn, cross-fostered males singing the foster species’ song famously show an inability to copy the temporal pattern of heterospecific songs, discovered to be due to a lack of ontogenetic flexibility in the neurons that encode heterospecific song-gap (silent period between song bouts) perception again within field L of the auditory forebrain ( Araki et al., 2016 ).
The zebra finch was not originally brought into the laboratory as a model system, nor championed as such by early research pioneers. From the 1950s onwards, the species has been progressively adopted as a useful focus of study in an increasing set of research fields, largely due to its accessibility and the ease with which it can be held and bred in captivity. In contrast, wild passerine birds have long been the focus of ecological and evolutionary research in the northern hemisphere. When studies of free-living study populations were unable to achieve the necessary manipulative rigor, the zebra finch, found commonly in pet shops throughout Europe and North America, became widely adopted as a surrogate captive experimental model. In parallel with its use in early ethological research, the zebra finch became established as an easier model than the canary for studying the neural basis of song, which in turn saw the former species adopted as a model for genomics, neuroscience, and developmental biology.
The zebra finch has provided great insights into diverse fields in biology and has travelled a long path from its natural habitat in arid Australia. It is important to be mindful that the traits that have contributed to its utility and adoption as ‘the’ avian laboratory model species for basic and biomedical research set it aside from most other avian species. The zebra finch evolved in an austral ecological setting that is profoundly different from those in the many geographic regions where most of this laboratory work takes place.
The zebra finch remains almost uniquely suited as a model system for research and the path ahead is likely to be productive and insightful in established and new areas of research. The late Richard Zann’s excellent monograph of the species (1996), whilst already over two decades old, still provides an excellent overview into the natural history of the species, and is never far from our desks, for the insight that it brings. We encourage future adopters of the zebra finch as a research model to use this book to guide their planning and to help interpret their results. The zebra finch is the most widely researched laboratory songbird in the world because of its uniqueness, and not as a result of any advocacy.
No new data were generated in this study.
- Google Scholar
- Margoliash D
- Adkins-Regan E
- Tomaszycki M
- Nottebohm F
- Ahmadiantehrani S
- Andalman AS
- Mariette MM
- Griffith SC
- Antonson ND
- Abolins-Abols M
- Kleindorfer S
- Yazaki-Sugiyama Y
- Phillmore LS
- MacDougall-Shackleton SA
- Balakrishnan CN
- Benichov JI
- Vallentin D
- Globerson E
- Tchernichovski O
- Zijlstra GG
- den Boer-Visser AM
- Van Der Zee EA
- Brainard MS
- Brigande JV
- Campbell DLM
- Carouso-Peck S
- Goldstein MH
- Matheson LE
- Challagulla A
- D'Amelio PB
- Davidson JH
- Shroff-Mehta N
- Ölveczky BP
- Theunissen FE
- Armstrong J
- Faircloth BC
- Andreu-Sánchez S
- Margaryan A
- Brumfield RT
- Christidis L
- Bertelsen MF
- Sicheritz-Ponten T
- Robertson BC
- Claramunt S
- Dumbacher JP
- Jamieson IG
- da Fonseca RR
- Stervander M
- Frandsen PB
- van der Zwan H
- van der Sluis R
- Fitzpatrick JW
- González-Solís J
- Ferrer-Obiol J
- Fleischer RC
- Rutherford KM
- Mouritsen H
- Liedvogel M
- Hoeppner MP
- Fudickar AM
- Ketterson ED
- Parody-Merino ÁM
- Prosdocimi F
- Parchman TL
- Schlinger BA
- Loiselle BA
- Fuxjager MJ
- Camenisch G
- Vasconcelos ATR
- Gilbert MTP
- Forstmeier W
- Segelbacher G
- Kempenaers B
- Schielzeth H
- McCowan LSC
- Baird-Daniel E
- Goldberg JH
- Condliffe D
- Holleley CE
- Alonso-Alvarez C
- Boucaud ICA
- Buchanan KL
- Derégnaucourt S
- Guillette LM
- Holveck M-J
- Tobias Krause E
- Mainwaring MC
- Martin-Wintle MS
- Romero-Haro AA
- Rutkowska J
- Varian-Ramos CW
- Williams TD
- Gunaratne PH
- Tennakoon JB
- Creighton CJ
- Milosavljevic A
- Griffiths-Jones S
- Hahnloser RH
- Kozhevnikov AA
- Woolley SMN
- McDiarmid CS
- Hyland Bruno J
- Immelmann K
- International Chicken Genome Sequencing Consortium
- Stephenson BM
- Ganapathy G
- Zavidovych V
- Subramanian S
- Capella-Gutiérrez S
- Huerta-Cepas J
- Rekepalli B
- Derryberry EP
- Schneider MP
- Samaniego JA
- Vargas Velazquez AM
- Alfaro-Núñez A
- Driskell AC
- Willerslev E
- McCormack J
- Stamatakis A
- Birkhead TR
- Hemmrich-Stanisak G
- Albrechtová J
- Backström N
- Akutagawa E
- Lansverk AL
- Schroeder KM
- Komorowski RW
- Shinn-Cunningham BG
- Miyanohara A
- Carleton JB
- Mackevicius EL
- Mandelblat-Cerf Y
- Denisenko N
- Gopalakrishnan S
- Markowitz AL
- Rajadinakaran G
- Langmore NE
- Pfenning AR
- Mountcastle J
- Thompson JW
- Soderblom EJ
- Bongaarts A
- Hartemink AJ
- Katlowitz KA
- Pnevmatikakis EA
- Wildstein S
- Oberlander S
- Remage-Healey L
- McCarthy SA
- Uliano-Silva M
- Fungtammasan A
- Thibaud-Nissen F
- Robertson B
- Wilkinson M
- Franchini P
- Simbirsky M
- Hannigan BT
- Kronenberg Z
- Friedrich SR
- Secomandi S
- Theofanopoulou C
- Masterson P
- Crawford AJ
- Venkatesh B
- Koepfli K-P
- Marques-Bonet T
- Phillippy AM
- Smallegange IM
- Hildebrand S
- Hahnloser RHR
- Rutstein AN
- Brazill-Boast J
- Shaughnessy DW
- Sannareddy K
- Przeworski M
- Sonnemann P
- Sjölander S
- Sorenson MD
- Terpstra NJ
- Shaevitz SS
- Tomaszycki ML
- Sundberg KA
- Tschirren B
- Verzijden MN
- Servedio MR
- Boughman JW
- Svensson EI
- McConnell P
- Patterson MA
- Sakaguchi H
- Hirozane-Kishikawa T
- Hayashizaki Y
- Kriengwatana B
- Gunaratne P
- Lopez-Otin C
- Chinwalla A
- Woodcock ME
- Idoko-Akoh A
- Yanagihara S
- Wittenbach JD
- Garcia-Oscos F
- Peter Rodgers Senior Editor; eLife, United Kingdom
- Helena Pérez Valle Reviewing Editor; eLife, United Kingdom
- Leslie Phillmore Reviewer; Dalhousie University, Canada
- Mimi Kao Reviewer; Tufts University, United States
In the interests of transparency, eLife publishes the most substantive revision requests and the accompanying author responses.
Thank you for submitting your article "The Natural History of Model Organisms: Neurogenomic insights into behavioral and vocal development of the zebra finch" to eLife for consideration as a Feature Article. Your article has been reviewed by three peer reviewers and the evaluation has been overseen by two editors from the eLife Features Team (Helena Pérez Valle and Peter Rodgers). The following individual involved in review of your submission has agreed to reveal their identity: Leslie Phillmore (Reviewer #3).
The reviewers and editors have discussed the reviews and we have drafted this decision letter to help you prepare a revised submission.
This article reviews the history of the zebra finch as a species of choice to study different aspects of developmental neurobiology and vocal communication. However, the article is lacking references to important works, and should also discuss the roles of the descending motor pathway in relation to the anterior forebrain pathway for song learning and adult song production.
Essential revisions:
1. Please include references to the work from labs that have substantially contributed to the fields of neuroscience and behaviour using zebra finches, including the Fee lab (MIT), the Goldberg lab (Cornell), the Sakata lab (McGill). Also please ensure that the article includes citations to the key works of other labs that are already cited, including the Jarvis lab (Rockefeller), the Long lab (NYU), the Brainard lab (UCSF), the Tchernikovski lab (CUNY) and the Theunissen lab (Berkeley). Please add up to 20 references to address this point. Additionally, please consider the following:
a) Please cite Desmond Morris (1954 and 1957) along with Immelmann when talking about the reproductive behaviour of zebra finches (line 35), and try to find a reference for Immelmann that is not an abstract for a conference.
b) Please cite Katharina Riebel (2000) "Early exposure leads to repeatable preferences for male song in female zebra finches", or any of her later reviews, in addition to Braaten and Reynolds (1999) (line 40).
c) Please check whether Day, 2019 is the correct citation in line 48; and whether work by the White, Mooney, Roberts or Scharff labs would be more appropriate when talking about genome editing.
d) Please check whether there are references missing in line 51 after "illness".
e) Please add references for genetic diversity in line 73 (e.g. "Genetic variation and differentiation in captive and wild zebra finches (Taeniopygia guttata)", from the Forstmeier lab).
f) Please cite reviews on line 140, since the literature is extensive. "Sexual imprinting and evolutionary processes in birds: a reassessment", Ten Cate and Vos (1999), and "The impact of learning on sexual selection and speciation", Verzijden et al. (2012).
g) In line 145, Louder et al. covers auditory but not song production, please find an appropriate reference to cover both.
h) In line 320, please add a reference to Scharff's work on FoxP2
i) In line 334/35, please provide a reference for "the unique immune function of oscine birds inhibiting full viral delivery of gene constructs".
2. Please add between 500 and 1000 words describing the roles of the descending motor pathway vis-à-vis the anterior forebrain pathway for song learning and adult song production; and the use of the zebra finch to study auditory perception of both biologically relevant stimuli (i.e. conspecific vocalizations) and others (e.g. human speech patterns).
3. Figure 2B should include spectrograms of at least a female call (see line 272), perhaps both a male and female call, as well as the male song.
4. The authors' work currently makes up about a third of the references, please check whether these references are all necessary and whether it might be possible to replace some of them with references to other important works in the field.
[Editors' note: further revisions were suggested prior to acceptance, as described below.]
Thank you for resubmitting your work entitled "Natural History of Model Organisms: Neural and genomic insights into behavioral and vocal development of the zebra finch" for further consideration by eLife . Your revised article has been reviewed by a new peer reviewer (Mimi Kao), and the evaluation was overseen by Helena Pérez Valle as the Assistant Features Editor and Peter Rodgers as the Features Editor.
The manuscript has been improved but there are some remaining issues that need to be addressed, as outlined below.
This article reviews the history of the zebra finch as a species of choice to study different aspects of developmental neurobiology and vocal communication, and particularly as a system for investigating sex differences in vocal communication. The revised manuscript is much improved, but it would still benefit of additional revisions to references, and including a discussion of studies of auditory perception using cross-fostered birds to examine innate preferences versus experience-dependent changes in auditory responses.
1. Please make the following modifications to references:
a) In line 37, add references to the work of the Fee and Yazaki-Sugiyama labs. These would be useful for neural mechanisms underlying sensorimotor learning (e.g. Okubo et al., 2015; Mackevicius, Happ, and Fee, 2020; Yanagihara and Yazaki-Sugiyama, 2019).
b) In lines 38-39, the reference to Chen et al., 2017 does not seem to be appropriate, since that study focuses on vocal learning, not female preference. Please remove this reference and replace it with another that examines influences on female song preferences (e.g. Riebel, 2000).
c) In lines 63 and/or 73, please add a reference to Cade et al., 1965, Water economy and metabolism of two estrildine finches, Physiological Zoology.
d) In lines 88-89, please add references to papers by Mets and Brainard (2018, 2019) that investigate the interaction of genes and social and/or auditory experience on vocal
learning and performance. Please add a short discussion about gene-environment interactions that shape song learning based on the findings of these papers in the section "Brains and genes".
e) In line 159 please add a reference to Price, PH (1979) Developmental determinants of structure in zebra finch song. Journal of Comparative and Physiological Psychology.
f) Please check the references in lines 258-260. While several papers have shown that both calls and songs have strong individual signatures that can be used for individual recognition, the references cited did not specifically examine "high levels of coordinated duetting between the male and female".
g) In lines 323-327, please add a reference to Aronov et al. (already cited in the article) in regard to the critical role of HVC for generating stereotyped, learned vocalization in lines 323-327.
h) In lines 330-331, please add references for the idea that the AFP generates song variability in juvenile birds (Olveczky et al., 2005, 2011; or a review article, e.g. Mooney, 2009; Woolley and Kao, 2015; or Dhawale, Smith and Olveczky 2017), and discuss these findings.
i) Please add references to studies showing that LMAN also sends an instructive signal that can bias song and drive systematic changes in mean pitch, mean duration and syllable transition probabilities (Turner and Brainard, 2007; Andalman and Fee, 2009; Ali et al., 2013, Charlesworth et al., 2012), and discuss these findings.
j) In lines 341-344, please add a reference for deafening experiments in juvenile birds, such as Price, 1979.
k) In lines 349-351, it is unclear why the Ma et al., 2020 reference is included, since the paragraph discusses mechanisms by which evaluation of song performance using auditory feedback can drive changes in song. Ma et al., 2020 report activity prior to calls by conspecifics. While predictive activity may be important for learning or maintaining song, please make clear what the activity is predicting (timing of upcoming calls? Expected auditory feedback given the motor commands?) and how it relates to learning the tutor song model.
l) In line 401, please add Xiao and Roberts, Neuron, 2018 as a reference, in addition to Kearney et al., 2019.
m) In lines 441-444, please add a reference to Shaughnessey et al., JCN, 2018 when mentioning the song system in females as that paper argues against the idea that females possess only vestiges of the song control network and suggests that the forebrain network may have a different function in females.
2. Given the manuscript's emphasis on vocal imprinting, please include a brief discussion of studies of auditory perception using cross-fostered birds to examine innate preferences versus experience-dependent changes in auditory responses (e.g. Araki et al., 2017; Moore and Woolley, 2019).
3. In lines 187-189, please modify your description of hair cell regeneration as "a specialized avian feat". Sensory hair cell regeneration after antibiotic treatment has been shown in other vertebrates, including fish (in the inner ear and lateral line; reviewed by Monroe et al., 2015, Front Cell Neurosci), as well as invertebrates (e.g. frogs).
4. Please revise the section "Brains and genes for vocal learning" to either omit mention of selective auditory responses, or expand this section for clarity (lines 319-321). While many studies have shown that neurons in both the motor pathway and the anterior forebrain pathway respond selectively to playback of bird's own song in anesthetized birds, the role of such auditory responses is not entirely clear. Auditory responses in HVC and in LMAN and Area X are gated by behavioral state and are much less pronounced in awake birds (Schmidt and Konishi , 1998; Hessler and Doupe, 1999).
5. Please expand the discussion of the role of HVC in coding the timing of song (lines 323-327). For example, HVC neurons that project to RA fire once per motif while RA neurons may fire multiple bursts at specific times in song (Yu and Margoliash, 1996). Similarly, Long and Fee, 2008 is referenced, but it would help the reader to explain how the cooling experiments help to establish that HVC codes for time in song.
Summary: This article reviews the history of the zebra finch as a species of choice to study different aspects of developmental neurobiology and vocal communication. However, the article is lacking references to important works, and should also discuss the roles of the descending motor pathway in relation to the anterior forebrain pathway for song learning and adult song production.
We have now included a fully-cited section on the role of the motor pathway in mediating juvenile song learning and adult song production.
Essential revisions: 1. Please include references to the work from labs that have substantially contributed to the fields of neuroscience and behaviour using zebra finches, including the Fee lab (MIT), the Goldberg lab (Cornell), the Sakata lab (McGill).
Dozens of previously unmentioned labs’ references and discussion of these works are now added.
Also please ensure that the article includes citations to the key works of other labs that are already cited, including the Jarvis lab (Rockefeller), the Long lab (NYU), the Brainard lab (UCSF), the Tchernikovski lab (CUNY) and the Theunissen lab (Berkeley). Please add up to 20 references to address this point.
Additional references (65 new ones) and discussion of these works are now also added to the manuscript.
Additionally, please consider the following: a) Please cite Desmond Morris (1954 and 1957) along with Immelmann when talking about the reproductive behaviour of zebra finches (line 35), and try to find a reference for Immelmann that is not an abstract for a conference.
Thank you. Done (Morris 1954) but Immelmann 1971 is a full article in the Proceedings of the 15 th IOC, it’s not just a conference abstract.
The Riebel 2000 paper and a Riebel-lab 2003 paper are now included.
Thank you. We have removed the incorrect Day et al. reference here and added additional avian genome editing review instead.
Reference (Han and Park 2018, London 2020) is added.
New Forstmeier lab references are added.
Two review papers are now also added as citations.
We are now citing an additional reference (Mooney 2009) to cover song production.
The Scharff TiG review/reference to FoxP2 is added as well as other Scharff lab references.
Reference of London 2020 is added.
We have now added discussion of both of these themes to the manuscript.
Call spectrograms are also added and turned into a new figure.
We have added 65 new citations to the manuscript, only 59 of which were non-self citations, especially with respect to the neurobiological, developmental, and behavioral contents of the paper and so the proportion of self-citations is now much diminished (25% instead of the earlier 35%). However, we still note that almost exclusively the only field work conducted on wild zebra finches over the past 15 years has been through the lab of Prof. Simon Griffith, our senior author. The main focus of this body of work (of which we have only cited a small fraction) has been to provide a relevant behavioral and ecological context to inform the vast amount of captive work on this species.
Summary: This article reviews the history of the zebra finch as a species of choice to study different aspects of developmental neurobiology and vocal communication, and particularly as a system for investigating sex differences in vocal communication. The revised manuscript is much improved, but it would still benefit of additional revisions to references, and including a discussion of studies of auditory perception using cross-fostered birds to examine innate preferences versus experience-dependent changes in auditory responses.
We now include more discussion of cross-fostering as a method to address innate vs. experience-dependent changes in auditory responses (something that I also did my postdoc research on!)
Essential revisions: 1. Please make the following modifications to references: a) In line 37, add references to the work of the Fee and Yazaki-Sugiyama labs. These would be useful for neural mechanisms underlying sensorimotor learning (e.g. Okubo et al., 2015; Mackevicius, Happ, and Fee, 2020; Yanagihara and Yazaki-Sugiyama, 2019).
We have now added the content and the three references to the text.
This is not correct as Chen et al. 2017 does focus on experience-dependence in females’ song preferences for male song variants. We, therefore, kept this reference here: Chen Y, Clark O, Woolley SC (2017) Courtship song preferences in female zebra finches are shaped by developmental auditory experience. Proceedings of the Royal Society of London B 284: 20170054.
We have now added this reference to the first instance.
d) In lines 88-89, please add references to papers by Mets and Brainard (2018, 2019) that investigate the interaction of genes and social and/or auditory experience on vocal learning and performance. Please add a short discussion about gene-environment interactions that shape song learning based on the findings of these papers in the section "Brains and genes".
Thank you, we agree that G X E interactions are essential in this section and we added the more recent citation, of these two on Bengalese finches, from eLife to support our updated content for the requested new sentences regarding Gene-by-Environment interactions in song learning in zebra finches, too.
We have now incorporated this reference twice at around this section.
Thank you. We have now rephrased this section and included our duetting reference here, too (Elie et al. 2010).
We have now added this reference here, too.
Thank you, we really needed references here. We have now cited two of these reviews and discuss briefly trial-and-error learning specifically in this section.
We have now added to our description and discussion of the role of LMAN and AFP in general, using the Andalman and Fee citation.
We have now added Price 1979 here, too.
We have deleted the Ma et al. 2020 reference and the tangential text associated with it in this paragraph.
We have now added this prior article here, too.
Thank you for this important reference, we now rephrased this sentence to be able to cite this article.
We now contrast female vs. male specific findings using these references in the paragraph just prior to the Conclusions.
Thank you; we have corrected this flaw and have now referred to two additional citations reviewing (including the one suggested) this topic in non-birds, too.
We have now rephrased this section, and adopted the referee’s suggestion for modifying the text and for the two additional citations included.
Thank you for these suggestions; we have now included new text and the new citation (plus one more) regarding HVC, RA, and temperature-dependent effects on song production in this section.
Author details
Mark E Hauber is in the Department of Evolution, Ecology, and Behavior, School of Integrative Biology, University of Illinois at Urbana-Champaign, Urbana-Champaign, United States
Contribution
For correspondence, competing interests.

Matthew IM Louder is in the International Research Center for Neurointelligence, University of Tokyo, Tokyo, Japan and the Department of Biology, Texas A&M University, College Station, United States
Simon C Griffith is in the Department of Biological Sciences, Macquarie University, Sydney, Australia
National Science Foundation (IOS1456524)
The funders had no role in study design, data collection and interpretation, or the decision to submit the work for publication.
Acknowledgements
We thank our many colleagues working with zebra finches for productive discussions, including the editors and the referees of eLife. M Rivera and O Tchernichovski kindly provided song and call files of adult zebra finches. We dedicate our article to the memories of the late Richard Zann and Mark Konishi. FUNDING Financial support was provided by the Harley Jones Van Cleave Professorship and the National Science Foundation IOS # 1456524 (to MIML and MEH).
Publication history
- Received: August 6, 2020
- Accepted: June 8, 2021
- Accepted Manuscript published : June 9, 2021
- Version of Record published : June 28, 2021
© 2021, Hauber et al.
This article is distributed under the terms of the Creative Commons Attribution License , which permits unrestricted use and redistribution provided that the original author and source are credited.
Views, downloads and citations are aggregated across all versions of this paper published by eLife.
Download links
Downloads (link to download the article as pdf).
- Article PDF
Open citations (links to open the citations from this article in various online reference manager services)
Cite this article (links to download the citations from this article in formats compatible with various reference manager tools), categories and tags.
- zebra finch
- recognition systems
- song culture
- natural history of model organisms
- vocal development
- Part of Collection
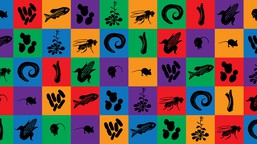
The Natural History of Model Organisms
Further reading.
- Evolutionary Biology
Essays on the wild lives of model organisms, from Arabidopsis to the zebrafish.
Does bumblebee preference of continuous over interrupted strings in string-pulling tasks indicate means-end comprehension?
Bumblebees ( Bombus terrestris ) have been shown to engage in string-pulling behavior to access rewards. The objective of this study was to elucidate whether bumblebees display means-end comprehension in a string-pulling task. We presented bumblebees with two options: one where a string was connected to an artificial flower containing a reward and the other presenting an interrupted string. Bumblebees displayed a consistent preference for pulling connected strings over interrupted ones after training with a stepwise pulling technique. When exposed to novel string colors, bees continued to exhibit a bias towards pulling the connected string. This suggests that bumblebees engage in featural generalization of the visual display of the string connected to the flower in this task. If the view of the string connected to the flower was restricted during the training phase, the proportion of bumblebees choosing the connected strings significantly decreased. Similarly, when the bumblebees were confronted with coiled connected strings during the testing phase, they failed to identify and reject the interrupted strings. This finding underscores the significance of visual consistency in enabling the bumblebees to perform the task successfully. Our results suggest that bumblebees’ ability to distinguish between continuous strings and interrupted strings relies on a combination of image matching and associative learning, rather than means-end understanding. These insights contribute to a deeper understanding of the cognitive processes employed by bumblebees when tackling complex spatial tasks.
Fine-scale tracking reveals visual field use for predator detection and escape in collective foraging of pigeon flocks
During collective vigilance, it is commonly assumed that individual animals compromise their feeding time to be vigilant against predators, benefiting the entire group. One notable issue with this assumption concerns the unclear nature of predator ‘detection’, particularly in terms of vision. It remains uncertain how a vigilant individual utilizes its high-acuity vision (such as the fovea) to detect a predator cue and subsequently guide individual and collective escape responses. Using fine-scale motion-capture technologies, we tracked the head and body orientations of pigeons (hence reconstructed their visual fields and foveal projections) foraging in a flock during simulated predator attacks. Pigeons used their fovea to inspect predator cues. Earlier foveation on a predator cue was linked to preceding behaviors related to vigilance and feeding, such as head-up or down positions, head-scanning, and food-pecking. Moreover, earlier foveation predicted earlier evasion flights at both the individual and collective levels. However, we also found that relatively long delay between their foveation and escape responses in individuals obscured the relationship between these two responses. While our results largely support the existing assumptions about vigilance, they also underscore the importance of considering vision and addressing the disparity between detection and escape responses in future research.
Be the first to read new articles from eLife
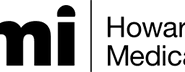
Information
- Author Services
Initiatives
You are accessing a machine-readable page. In order to be human-readable, please install an RSS reader.
All articles published by MDPI are made immediately available worldwide under an open access license. No special permission is required to reuse all or part of the article published by MDPI, including figures and tables. For articles published under an open access Creative Common CC BY license, any part of the article may be reused without permission provided that the original article is clearly cited. For more information, please refer to https://www.mdpi.com/openaccess .
Feature papers represent the most advanced research with significant potential for high impact in the field. A Feature Paper should be a substantial original Article that involves several techniques or approaches, provides an outlook for future research directions and describes possible research applications.
Feature papers are submitted upon individual invitation or recommendation by the scientific editors and must receive positive feedback from the reviewers.
Editor’s Choice articles are based on recommendations by the scientific editors of MDPI journals from around the world. Editors select a small number of articles recently published in the journal that they believe will be particularly interesting to readers, or important in the respective research area. The aim is to provide a snapshot of some of the most exciting work published in the various research areas of the journal.
Original Submission Date Received: .
- Active Journals
- Find a Journal
- Journal Proposal
- Proceedings Series
- For Authors
- For Reviewers
- For Editors
- For Librarians
- For Publishers
- For Societies
- For Conference Organizers
- Open Access Policy
- Institutional Open Access Program
- Special Issues Guidelines
- Editorial Process
- Research and Publication Ethics
- Article Processing Charges
- Testimonials
- Preprints.org
- SciProfiles
- Encyclopedia
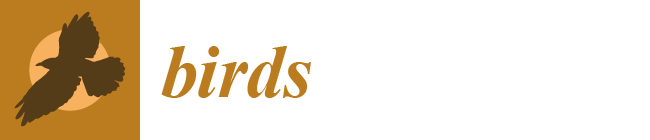
Article Menu
- Subscribe SciFeed
- Recommended Articles
- Google Scholar
- on Google Scholar
- Table of Contents
Find support for a specific problem in the support section of our website.
Please let us know what you think of our products and services.
Visit our dedicated information section to learn more about MDPI.
JSmol Viewer
The ecology of the zebra finch makes it a great laboratory model but an outlier amongst passerine birds.
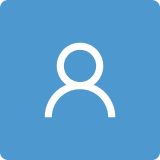
Simple Summary
1. introduction, 1.1. food quality/abundance, reproductive success and development, 1.2. climatic extremes during breeding and development, 1.3. what is the life history of the zebra finch, 2. conclusions, supplementary materials, author contributions, institutional review board statement, conflicts of interest.
- Matthews, B.J.; Vosshall, L.B. How to turn an organism into a model organism in 10 ‘easy’ steps. J. Exp. Biol. 2020 , 223 . [ Google Scholar ] [ CrossRef ] [ PubMed ] [ Green Version ]
- Dietrich, M.R.; Ankeny, R.A.; Crowe, N.; Green, S.; Leonelli, S. How to choose your research organism. Stud. Hist. Philos. Sci. Part C Stud. Hist. Philos. Biol. Biomed. Sci. 2020 , 80 . [ Google Scholar ] [ CrossRef ] [ PubMed ]
- Hedges, S.B. The origin and evolution of model organisms. Nat. Rev. Genet. 2002 , 3 , 838–849. [ Google Scholar ] [ CrossRef ] [ PubMed ]
- Peter, A.K.; Crocini, C.; Leinwand, L.A. Expanding our scientific horizons: Utilization of unique model organisms in biological research. Embo J. 2017 , 36 , 2311–2314. [ Google Scholar ] [ CrossRef ]
- Zann, R. The Zebra Finch: A Synthesis of Field and Laboratory Studies ; Oxford University Press: Oxford, UK, 1996. [ Google Scholar ]
- Griffith, S.C.; Crino, O.L.; Andrew, S.C.; Nomano, F.Y.; Adkins-Regan, E.; Alonso-Alvarez, C.; Bailey, I.E.; Bittner, S.S.; Bolton, P.E.; Boner, W.; et al. Variation in Reproductive Success Across Captive Populations: Methodological Differences, Potential Biases and Opportunities. Ethology 2017 , 123 , 1–29. [ Google Scholar ] [ CrossRef ] [ Green Version ]
- Forstmeier, W.; Segelbacher, G.; Mueller, J.C.; Kempenaers, B. Genetic variation and differentiation in captive and wild zebra finches ( Taeniopygia guttata ). Mol. Ecol. 2007 , 16 , 4039–4050. [ Google Scholar ] [ CrossRef ] [ PubMed ]
- Gasch, A.P.; Payseur, B.A.; Pool, J.E. The Power of Natural Variation for Model Organism Biology. Trends Genet. 2016 , 32 , 147–154. [ Google Scholar ] [ CrossRef ] [ PubMed ] [ Green Version ]
- Brainard, M.S.; Doupe, A.J. What songbirds teach us about learning. Nature 2002 , 417 , 351–358. [ Google Scholar ] [ CrossRef ] [ PubMed ]
- Vallentin, D.; Kosche, G.; Lipkind, D.; Long, M.A. Inhibition protects acquired song segments during vocal learning in zebra finches. Science 2016 , 351 , 267–271. [ Google Scholar ] [ CrossRef ] [ PubMed ] [ Green Version ]
- Riebel, K. Song and Female Mate Choice in Zebra Finches: A Review. Adv. Study Behav. 2009 , 40 , 197–238. [ Google Scholar ] [ CrossRef ]
- Immelmann, K. The influence of early experience upon the development of social behaviour in estrildine finches. Proc. Int. Ornithol. Congr. 1972 , 15 , 316–338. [ Google Scholar ]
- Mello, C.V.; Vicario, D.S.; Clayton, D.F. Song presentation induces gene-expression in the songbird forebrain. Proc. Natl. Acad. Sci. USA 1992 , 89 , 6818–6822. [ Google Scholar ] [ CrossRef ] [ PubMed ] [ Green Version ]
- Ankeny, R.A.; Leonelli, S. What’s so special about model organisms? Stud. Hist. Philos. Sci. 2011 , 42 , 313–323. [ Google Scholar ] [ CrossRef ]
- Paris, O.J.; Swaddle, J.P.; Cristol, D.A. Exposure to Dietary Methyl-Mercury Solely during Embryonic and Juvenile Development Halves Subsequent Reproductive Success in Adult Zebra Finches. Environ. Sci. Technol. 2018 , 52 , 3117–3124. [ Google Scholar ] [ CrossRef ]
- Hofmeister, E.K.; Lund, M.; Shearn-Bochsler, V.; Balakrishnan, C.N. Susceptibility and Antibody Response of the Laboratory Model Zebra Finch ( Taeniopygia guttata ) to West Nile Virus. PLoS ONE 2017 , 12 , e0167876. [ Google Scholar ] [ CrossRef ]
- Hofmeister, E.K.; Balakrishnan, C.N.; Atkinson, C.T. Population Differences in Susceptibility to Plasmodium relictum in Zebra Finches Taeniopygia guttata . Avian Dis. 2018 , 62 , 351–354. [ Google Scholar ] [ CrossRef ]
- Newhouse, D.J.; Balakrishnan, C.N. High major histocompatibility complex class I polymorphism despite bottlenecks in wild and domesticated populations of the zebra finch ( Taeniopygia guttata ). BMC Evol. Biol. 2015 , 15 . [ Google Scholar ] [ CrossRef ] [ Green Version ]
- Phifer-Rixey, M.; Nachman, M.W. Insights into mammalian biology from the wild house mouse Mus musculus. Elife 2015 , 4 . [ Google Scholar ] [ CrossRef ]
- Markow, T.A. The secret lives of Drosophila flies. Elife 2015 , 4 , e06793. [ Google Scholar ] [ CrossRef ]
- Morton, S.R.; Smith, D.M.S.; Dickman, C.R.; Dunkerley, D.L.; Friedel, M.H.; McAllister, R.R.J.; Reid, J.R.W.; Roshier, D.A.; Smith, M.A.; Walsh, F.J.; et al. A fresh framework for the ecology of arid Australia. J. Arid Environ. 2011 , 75 , 313–329. [ Google Scholar ] [ CrossRef ]
- Duursma, D.E.; Gallagher, R.V.; Griffith, S.C. Characterizing opportunistic breeding at a continental scale using all available sources of phenological data: An assessment of 337 species across the Australian continent. Auk 2017 , 134 , 509–519. [ Google Scholar ] [ CrossRef ]
- Zann, R.A.; Morton, S.R.; Jones, K.R.; Burley, N.T. The timing of breeding by zebra finches in relation to rainfall in central Australia. Emu Austral. Ornithol. 1995 , 95 , 208–222. [ Google Scholar ] [ CrossRef ]
- Simmonds, E.G.; Sheldon, B.C.; Coulson, T.; Cole, E.F. Incubation behavior adjustments, driven by ambient temperature variation, improve synchrony between hatch dates and caterpillar peak in a wild bird population. Ecol. Evol. 2017 , 7 , 9415–9425. [ Google Scholar ] [ CrossRef ] [ PubMed ] [ Green Version ]
- Drent, R.H.; Daan, S. The prudent parent: Energetic adjustments in avian breeding. Ardea 1980 , 68 , 225–252. [ Google Scholar ] [ CrossRef ]
- Stephens, P.A.; Boyd, I.L.; McNamara, J.M.; Houston, A.I. Capital breeding and income breeding: Their meaning, measurement, and worth. Ecology 2009 , 90 , 2057–2067. [ Google Scholar ] [ CrossRef ]
- MacKay, A.E.; Forsyth, D.M.; Coulson, G.; Festa-Bianchet, M. Maternal resource allocation adjusts to timing of parturition in an asynchronous breeder. Behav. Ecol. Sociobiol. 2018 , 72 . [ Google Scholar ] [ CrossRef ]
- Monaghan, P.; Metcalfe, N.B.; Houston, D.C. Male finches selectively pair with fecund females. Proc. R. Soc. B Biol. Sci. 1996 , 263 , 1183–1186. [ Google Scholar ] [ CrossRef ]
- Williams, T.D. Variation in reproductive effort in female zebra finches ( Taeniopygia guttata ) in relation to nutrient-specific dietary supplements during egg laying. Physiol. Zool. 1996 , 69 , 1255–1275. [ Google Scholar ] [ CrossRef ]
- Rutkowska, J.; Cichon, M. Maternal investment during egg laying and offspring sex: An experimental study of zebra finches. Anim. Behav. 2002 , 64 , 817–822. [ Google Scholar ] [ CrossRef ]
- Rutstein, A.N.; Slater, P.J.B.; Graves, J.A. Diet quality and resource allocation in the zebra finch. Proc. R. Soc. B Biol. Sci. 2004 , 271 , S286–S289. [ Google Scholar ] [ CrossRef ] [ Green Version ]
- Stearns, S.C. The Evolution of Life Histories ; Oxford University Press: Oxford, UK, 1992. [ Google Scholar ]
- Fisher, M.O.; Nager, R.G.; Monaghan, P. Compensatory growth impairs adult cognitive performance. PLoS Biol. 2006 , 4 , 1462–1466. [ Google Scholar ] [ CrossRef ] [ PubMed ] [ Green Version ]
- Criscuolo, F.; Monaghan, P.; Nasir, L.; Metcalfe, N.B. Early nutrition and phenotypic development: ‘catch-up’ growth leads to elevated metabolic rate in adulthood. Proc. R. Soc. B Biol. Sci. 2008 , 275 , 1565–1570. [ Google Scholar ] [ CrossRef ] [ PubMed ] [ Green Version ]
- Metcalfe, N.B.; Monaghan, P. Compensation for a bad start: Grow now, pay later? Trends Ecol. Evol. 2001 , 16 , 254–260. [ Google Scholar ] [ CrossRef ]
- Naguib, M.; Gil, D. Transgenerational effects on body size caused by early developmental stress in zebra finches. Biol. Lett. 2005 , 1 , 95–97. [ Google Scholar ] [ CrossRef ] [ PubMed ] [ Green Version ]
- Briga, M.; Koetsier, E.; Boonekamp, J.J.; Jimeno, B.; Verhulst, S. Food availability affects adult survival trajectories depending on early developmental conditions. Proc. R. Soc. B Biol. Sci. 2017 , 284 . [ Google Scholar ] [ CrossRef ]
- Pei, Y.F.; Forstmeier, W.; Kempenaers, B. Offspring performance is well buffered against stress experienced by ancestors. Evolution 2020 , 74 , 1525–1539. [ Google Scholar ] [ CrossRef ]
- Griffith, S.C.; Crino, O.L.; Andrew, S.C. Commentary: A Bird in the House: The Challenge of Being Ecologically Relevant in Captivity. Front. Ecol. Evol. 2017 , 5 . [ Google Scholar ] [ CrossRef ] [ Green Version ]
- Griffith, S.; Pryke, S.; Mariette, M. Use of nest-boxes by the Zebra Finch ( Taeniopygia guttata ): Implications for reproductive success and research. EMU 2008 , 108 , 311–319. [ Google Scholar ] [ CrossRef ]
- Janzen, D.H. Why mountain passes are higher in the tropics. Amer Nat. 1967 , 101 , 233–249. [ Google Scholar ] [ CrossRef ]
- Ghalambor, C.K.; Huey, R.B.; Martin, P.R.; Tewksbury, J.J.; Wang, G. Are mountain passes higher in the tropics? Janzen’s hypothesis revisited. Integr. Comp. Biol. 2006 , 46 , 5–17. [ Google Scholar ] [ CrossRef ] [ Green Version ]
- Funk, W.C.; Blouin, M.S.; Corn, P.S.; Maxell, B.A.; Pilliod, D.S.; Amish, S.; Allendorf, F.W. Population structure of Columbia spotted frogs ( Rana luteiventris ) is strongly affected by the landscape. Mol. Ecol. 2005 , 14 , 483–496. [ Google Scholar ] [ CrossRef ] [ Green Version ]
- Cooper, C.E.; Hurley, L.L.; Deviche, P.; Griffith, S.C. Physiological responses of wild zebra finches ( Taeniopygia guttata ) to heatwaves. J. Exp. Biol. 2020 , 223 . [ Google Scholar ] [ CrossRef ]
- Cooper, C.E.; Hurley, L.L.; Griffith, S.C. Effect of acute exposure to high ambient temperature on the thermal, metabolic and hygric physiology of a small desert bird. Comp. Biochem. Physiol. A Mol. Integr. Physiol. 2020 , 244 . [ Google Scholar ] [ CrossRef ] [ PubMed ]
- Khaliq, I.; Hof, C.; Prinzinger, R.; Boehning-Gaese, K.; Pfenninger, M. Global variation in thermal tolerances and vulnerability of endotherms to climate change. Proc. R. Soc. B Biol. Sci. 2014 , 281 . [ Google Scholar ] [ CrossRef ] [ PubMed ] [ Green Version ]
- Andreasson, F.; Nilsson, J.-A.; Nord, A. Avian Reproduction in a Warming World. Front. Ecol. Evol. 2020 , 8 . [ Google Scholar ] [ CrossRef ]
- Nord, A.; Sandell, M.I.; Nilsson, J.A. Female zebra finches compromise clutch temperature in energetically demanding incubation conditions. Funct. Ecol. 2010 , 24 , 1031–1036. [ Google Scholar ] [ CrossRef ]
- Funghi, C.; McCowan, L.S.C.; Schuett, W.; Griffith, S.C. High air temperatures induce temporal, spatial and social changes in the foraging behaviour of wild zebra finches. Anim. Behav. 2019 , 149 , 33–43. [ Google Scholar ] [ CrossRef ] [ Green Version ]
- Cooper, C.E.; Withers, P.C.; Hurley, L.L.; Griffith, S.C. The Field Metabolic Rate, Water Turnover, and Feeding and Drinking Behavior of a Small Avian Desert Granivore During a Summer Heatwave. Front. Physiol. 2019 , 10 , 1405. [ Google Scholar ] [ CrossRef ] [ Green Version ]
- Griffith, S.C.; Mainwaring, M.C.; Sorato, E.; Beckmann, C. High atmospheric temperatures and ‘ambient incubation’ drive embryonic development and lead to earlier hatching in a passerine bird. R. Soc. Open Sci. 2016 , 3 . [ Google Scholar ] [ CrossRef ] [ Green Version ]
- Andrew, S.C.; Hurley, L.L.; Mariette, M.M.; Griffith, S.C. Higher temperatures during development reduce body size in the zebra finch in the laboratory and in the wild. J. Evol. Biol. 2017 , 30 , 2156–2164. [ Google Scholar ] [ CrossRef ] [ Green Version ]
- Crino, O.L.; Driscoll, S.C.; Brandl, H.B.; Buchanan, K.L.; Griffith, S.C. Under the weather: Corticosterone levels in wild nestlings are associated with ambient temperature and wind. Gen. Comp. Endocrinol. 2020 , 285 . [ Google Scholar ] [ CrossRef ] [ PubMed ]
- Sheldon, E.L.; Schrey, A.W.; Hurley, L.L.; Griffith, S.C. Dynamic changes in DNA methylation during postnatal development in zebra finches Taeniopygia guttata exposed to different temperatures. J. Avian Biol. 2020 , 51 . [ Google Scholar ] [ CrossRef ]
- Olson, C.R.; Vleck, C.M.; Adams, D.C. Decoupling morphological development from growth in periodically cooled zebra finch embryos. J. Morphol. 2008 , 269 , 875–883. [ Google Scholar ] [ CrossRef ]
- Wada, H.; Kriengwatana, B.; Allen, N.; Schmidt, K.L.; Soma, K.K.; MacDougall-Shackleton, S.A. Transient and permanent effects of suboptimal incubation temperatures on growth, metabolic rate, immune function and adrenocortical responses in zebra finches. J. Exp. Biol. 2015 , 218 , 2847–2855. [ Google Scholar ] [ CrossRef ] [ PubMed ] [ Green Version ]
- Berntsen, H.H.; Bech, C. Incubation temperature influences survival in a small passerine bird. J. Avian Biol. 2016 , 47 , 141–145. [ Google Scholar ] [ CrossRef ]
- Monaghan, P. Early growth conditions, phenotypic development and environmental change. Philos. Trans. R. Soc. B Biol. Sci. 2008 , 363 , 1635–1645. [ Google Scholar ] [ CrossRef ] [ Green Version ]
- Uller, T.; Nakagawa, S.; English, S. Weak evidence for anticipatory parental effects in plants and animals. J. Evol. Biol. 2013 , 26 , 2161–2170. [ Google Scholar ] [ CrossRef ] [ Green Version ]
- Burgess, S.C.; Marshall, D.J. Adaptive parental effects: The importance of estimating environmental predictability and offspring fitness appropriately. Oikos 2014 , 123 , 769–776. [ Google Scholar ] [ CrossRef ]
- Galloway, L.F.; Etterson, J.R. Transgenerational plasticity is adaptive in the wild. Science 2007 , 318 , 1134–1136. [ Google Scholar ] [ CrossRef ] [ Green Version ]
- Mousseau, T.A.; Dingle, H. Maternal effects in insect life histories. Annu. Rev. Entomol. 1991 , 36 , 511–534. [ Google Scholar ] [ CrossRef ]
- Radersma, R.; Hegg, A.; Noble, D.W.A.; Uller, T. Timing of maternal exposure to toxic cyanobacteria and offspring fitness in Daphnia magna: Implications for the evolution of anticipatory maternal effects. Ecol. Evol. 2018 , 8 , 12727–12736. [ Google Scholar ] [ CrossRef ] [ Green Version ]
- Krause, E.T.; Naguib, M. Effects of parental and own early developmental conditions on the phenotype in zebra finches ( Taeniopygia guttata ). Evol. Ecol. 2014 , 28 , 263–275. [ Google Scholar ] [ CrossRef ]
- De Coster, G.; Verhulst, S.; Koetsier, E.; De Neve, L.; Briga, M.; Lens, L. Effects of early developmental conditions on innate immunity are only evident under favourable adult conditions in zebra finches. Naturwissenschaften 2011 , 98 , 1049–1056. [ Google Scholar ] [ CrossRef ] [ PubMed ]
- Mariette, M.M.; Buchanan, K.L. Prenatal acoustic communication programs offspring for high posthatching temperatures in a songbird. Science 2016 , 353 , 812–814. [ Google Scholar ] [ CrossRef ] [ Green Version ]
- Griffith, S.C.; Buchanan, K.L. Maternal effects in the Zebra Finch: A model mother reviewed. Emu 2010 , 110 , 251–267. [ Google Scholar ] [ CrossRef ]
- Crean, A.J.; Marshall, D.J. Coping with environmental uncertainty: Dynamic bet hedging as a maternal effect. Philos. Trans. R. Soc. B Biol. Sci. 2009 , 364 , 1087–1096. [ Google Scholar ] [ CrossRef ] [ PubMed ]
- McNamara, J.M.; Houston, A.I. Optimal annual routines: Behaviour in the context of physiology and ecology. Philos. Trans. R. Soc. B Biol. Sci. 2008 , 363 , 301–319. [ Google Scholar ] [ CrossRef ] [ PubMed ] [ Green Version ]
- Zann, R.; Runciman, D. Survivorship, dispersal and sex ratios of zebra finches Taeniopygia guttata in southeast Australia. IBIS 1994 , 136 , 136–146. [ Google Scholar ] [ CrossRef ]
- Reichard, M. Evolutionary ecology of aging: Time to reconcile field and laboratory research. Ecol. Evol. 2016 , 6 , 2988–3000. [ Google Scholar ] [ CrossRef ] [ Green Version ]
- Wilbur, H.M.; Rudolf, V.H.W. Life-history evolution in uncertain environments: Bet hedging in time. Am. Nat. 2006 , 168 , 398–411. [ Google Scholar ] [ CrossRef ]
- Martin, T.E.; Ton, R.; Oteyza, J.C. Adaptive influence of extrinsic and intrinsic factors on variation of incubation periods among tropical and temperate passerines. Auk 2018 , 135 , 101–113. [ Google Scholar ] [ CrossRef ]
- Arendt, J.D. Adaptive intrinsic growth rates: An integration across taxa. Q. Rev. Biol. 1997 , 72 , 149–177. [ Google Scholar ] [ CrossRef ]
- Bosque, C.; Bosque, M.T. Nest predation as a selective factor in the evolution of developmental rates in altricial birds. Am. Nat. 1995 , 145 , 234–260. [ Google Scholar ] [ CrossRef ]
- Lloyd, P.; Abadi, F.; Altwegg, R.; Martin, T.E. South temperate birds have higher apparent adult survival than tropical birds in Africa. J. Avian Biol. 2014 , 45 , 493–500. [ Google Scholar ] [ CrossRef ]
- Bech, C.; Chappell, M.A.; Astheimer, L.B.; Londono, G.A.; Buttemer, W.A. A ‘slow pace of life’ in Australian old-endemic passerine birds is not accompanied by low basal metabolic rates. J. Comp. Physiol. B Biochem. Syst. Environ. Physiol. 2016 , 186 , 503–512. [ Google Scholar ] [ CrossRef ] [ PubMed ] [ Green Version ]
- Lloyd, P. Rainfall as a breeding stimulus and clutch size determinant in South African arid-zone birds. IBIS 1999 , 141 , 637–643. [ Google Scholar ] [ CrossRef ]
- Joys, A.C.; Crick, H.Q.P. Breeding periods for selected bird species in England. Bto Res. Rep. 2004 , 352 , 1–45. [ Google Scholar ]
- Mariette, M.M.; Pariser, E.C.; Gilby, A.J.; Magrath, M.J.L.; Pryke, S.R.; Griffith, S.C. Using an electronic monitoring system to link offspring provisioning and foraging behavior of a wild passerine. Auk 2011 , 128 , 26–35. [ Google Scholar ] [ CrossRef ] [ Green Version ]
- Griffith, S.C. Cooperation and coordination in socially monogamous birds: Moving away from a focus on sexual conflict. Front. Ecol. Evol. 2020 , 7 , 455. [ Google Scholar ] [ CrossRef ] [ Green Version ]
- Wang, D.P.; Forstmeier, W.; Kempenaers, B. No mutual mate choice for quality in zebra finches: Time to question a widely held assumption. Evolution 2017 , 71 , 2661–2676. [ Google Scholar ] [ CrossRef ]
- McCowan, L.S.C.; Mariette, M.M.; Griffith, S.C. The size and composition of social groups in the wild zebra finch. Emu 2015 , 115 , 191–198. [ Google Scholar ] [ CrossRef ]
- Adkins-Regan, E.; Tomaszycki, M. Monogamy on the fast track. Biol. Lett. 2007 , 3 , 617–619. [ Google Scholar ] [ CrossRef ] [ PubMed ]
- Dall, S.R.X.; Griffith, S.C. An empiricist guide to animal personality variation in ecology and evolution. Front. Ecol. Evol. 2014 , 2 . [ Google Scholar ] [ CrossRef ] [ Green Version ]
Click here to enlarge figure
English Name | Scientific Name | Papers 2000–2020 |
---|---|---|
Feral/Domestic Pigeon | Columba livia | 4177 |
Great Tit | Parus major | 4035 |
Quail/Japanese Quail | Coturnix coturnix japonica | 3639 |
House Sparrow | Passer domesticus | 2940 |
Zebra Finch | Taeniopygia guttata | 2937 |
Common Starling | Sturnus vulgaris | 2608 |
Blue Tit | Cyanistes caeruleus * | 1970 |
Barn Swallow | Hirundo rustica | 1606 |
Pied Flycatcher | Ficedula hypoleuca | 1354 |
Budgerigar | Melopsittacus undulatus | 908 |
Song Sparrow | Melospiza melodia | 889 |
Chaffinch | Fringilla coelebs | 833 |
Tree Swallow | Tachycineta bicolor | 822 |
Collared Flycatcher | Ficedula albicollis | 646 |
Dark-Eyed Junco | Junco hyemalis | 587 |
Red-Winged Blackbird | Agelaius phoeniceus | 546 |
House Finch | Carpodacus mexicanus | 534 |
Canary | Serinus canaria | 529 |
Red-Legged Partridge | Alectoris rufa | 527 |
Cockatiel | Nymphicus hollandicus | 398 |
Indian Peafowl | Pavo cristatus | 378 |
Research Areas | Records | % of 2937 |
---|---|---|
Behavioural sciences | 1867 | 64 |
Neurosciences neurology | 1542 | 53 |
Biochemistry molecular biology | 1258 | 43 |
Reproductive biology | 1028 | 35 |
Genetics heredity | 984 | 34 |
Environmental sciences ecology | 913 | 31 |
Physiology | 847 | 29 |
Psychology | 781 | 27 |
Evolutionary biology | 661 | 23 |
Endocrinology metabolism | 601 | 20 |
Developmental biology | 582 | 20 |
Anatomy morphology | 465 | 16 |
Nutrition dietetics | 394 | 13 |
Immunology | 347 | 12 |
Pharmacology pharmacy | 334 | 11 |
Communication | 322 | 11 |
Cell biology | 278 | 9 |
Toxicology | 148 | 5 |
Countries/Regions | Records | % of 2937 |
---|---|---|
USA | 1431 | 49 |
UK | 851 | 29 |
Germany | 372 | 13 |
Canada | 279 | 9 |
Australia | 189 | 6 |
Netherlands | 157 | 5 |
France | 153 | 5 |
China | 130 | 4 |
Japan | 112 | 4 |
Sweden | 96 | 3 |
Belgium | 71 | 2 |
Spain | 71 | 2 |
New Zealand | 60 | 2 |
Switzerland | 43 | 1 |
Italy | 33 | 1 |
MDPI stays neutral with regard to jurisdictional claims in published maps and institutional affiliations. |
Share and Cite
Griffith, S.C.; Ton, R.; Hurley, L.L.; McDiarmid, C.S.; Pacheco-Fuentes, H. The Ecology of the Zebra Finch Makes It a Great Laboratory Model but an Outlier amongst Passerine Birds. Birds 2021 , 2 , 60-76. https://doi.org/10.3390/birds2010004
Griffith SC, Ton R, Hurley LL, McDiarmid CS, Pacheco-Fuentes H. The Ecology of the Zebra Finch Makes It a Great Laboratory Model but an Outlier amongst Passerine Birds. Birds . 2021; 2(1):60-76. https://doi.org/10.3390/birds2010004
Griffith, Simon C., Riccardo Ton, Laura L. Hurley, Callum S. McDiarmid, and Hector Pacheco-Fuentes. 2021. "The Ecology of the Zebra Finch Makes It a Great Laboratory Model but an Outlier amongst Passerine Birds" Birds 2, no. 1: 60-76. https://doi.org/10.3390/birds2010004
Article Metrics
Article access statistics, supplementary material.
PDF-Document (PDF, 211 KiB)
Further Information
Mdpi initiatives, follow mdpi.
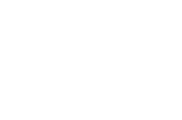
Subscribe to receive issue release notifications and newsletters from MDPI journals

Welfare of zebra finches used in research
- Find this author on Google Scholar
- Find this author on PubMed
- Search for this author on this site
- For correspondence: [email protected]
- Info/History
- Preview PDF
Over the past 50 years, songbirds have become a valuable model organism for scientists studying vocal communication from its behavioral, hormonal, neuronal, and genetic perspectives. Many advances in our understanding of vocal learning result from research using the zebra finch, a close-ended vocal learner. We review some of the manipulations used in zebra finch research, such as isolate housing, transient/irreversible impairment of hearing/vocal organs, implantation of small devices for chronic electrophysiology, head fixation for imaging, aversive song conditioning using sound playback, and mounting of miniature backpacks for behavioral monitoring. We highlight the use of these manipulations in scientific research, and estimate their impact on animal welfare, based on the literature and on data from our past and ongoing work. The assessment of harm-benefits tradeoffs is a legal prerequisite for animal research in Switzerland. We conclude that a diverse set of known stressors reliably lead to suppressed singing rate, and that by contraposition, increased singing rate may be a useful indicator of welfare. We hope that our study can contribute to answering some of the most burning questions about zebra finch welfare in research on vocal behaviors.
View the discussion thread.
Thank you for your interest in spreading the word about bioRxiv.
NOTE: Your email address is requested solely to identify you as the sender of this article.

Citation Manager Formats
- EndNote (tagged)
- EndNote 8 (xml)
- RefWorks Tagged
- Ref Manager
- Tweet Widget
- Facebook Like
- Google Plus One
Subject Area
- Animal Behavior and Cognition
- Animal Behavior and Cognition (5648)
- Biochemistry (12787)
- Bioengineering (9672)
- Bioinformatics (31255)
- Biophysics (16096)
- Cancer Biology (13180)
- Cell Biology (18855)
- Clinical Trials (138)
- Developmental Biology (10202)
- Ecology (15186)
- Epidemiology (2067)
- Evolutionary Biology (19397)
- Genetics (12880)
- Genomics (17773)
- Immunology (12905)
- Microbiology (30206)
- Molecular Biology (12608)
- Neuroscience (65902)
- Paleontology (486)
- Pathology (2041)
- Pharmacology and Toxicology (3518)
- Physiology (5447)
- Plant Biology (11284)
- Scientific Communication and Education (1742)
- Synthetic Biology (3124)
- Systems Biology (7764)
- Zoology (1757)
Prairie Research Institute
Blog navigation, zebra finch study finds mixed impact of early-life stress.
- Share on Facebook

When male zebra finches were stressed early in life, they wound up with duller beaks but sang more complex songs. Illustration by Danielle Ruffatto.

A bout of early-life stress can have lifelong impacts on two key signals that help male zebra finches attract mates: beak color and song complexity. But rather than being uniformly negative, a recent study published in Functional Ecology found that the consequences of stress are mixed. Stressed males wind up with duller, less colorful beaks but sing more complex songs.
“That caught us totally off guard,” said Loren Merrill , a postdoctoral researcher at the Illinois Natural History Survey who was lead author on the study conducted with principal investigator Jennifer Grindstaff, an associate professor at Oklahoma State University. Previous studies found that challenges like infection or lack of nutritious food early in life could lead to poorer outcomes for adult birds, so the team expected that when they activated the young males’ immune systems by treating them with an antigen, those birds would sing less complex songs later in life.
“That’s what we saw with beak coloration,” Merrill said. The males treated with KLH (keyhole limpet hemocyanin, a molecule that stimulates an immune response) “had duller, less colorful beaks, and that impact lasted throughout their lives.”
While beak color can fluctuate throughout a bird’s life based on diet and health, for a KLH-treated bird “the upper limit of his coloration seems to be set in that early-life period. There’s sort of a cap on how ‘sexy’ or red his beak can be,” Merrill said.
“Beak coloration has been linked to attractiveness, but it’s not clear whether females actually prefer males with brighter red bills, or whether that co-varies with other characteristics, like song complexity. Both reflect an individual in good condition,” Merrill said. “Beak coloration may be more important as a signal to other males, as a sign of dominance and competitive ability.” Rather than appealing directly to female zebra finches, beak color may influence the hierarchy among males, and that hierarchy in turn may affect which males the females choose as mates.
Female zebra finches also assess song quality when choosing mates. Each male typically produces one song that is composed of different elements. Each element can be unique, or a male can recycle an element and use it multiple times; they also can combine elements into phrases. Scientists believe that songs with more elements, especially unique elements, and song phrases are more challenging to learn and produce, and that females prefer those songs.
“Song is a cool system in zebra finches, because it’s been used as a model system for understanding human language learning,” Merrill said. “A lot of the ways in which birds learn and process songs are similar to how humans learn and process and produce language.”
In zebra finches, song development occurs in two overlapping stages. From roughly 15 days to 60 days after the birds hatch, they learn songs from older “tutor” males. Then approximately 30 to 90 days after hatching, each bird practices, refines, and crystallizes the song he will sing for life.
Both the volume and connectivity of the brain cells responsible for song learning and production increase around this time in the young bird’s life. The research team hypothesized that stress to the birds’ immune system during this early stage could put those key nuclei at risk, causing the birds to develop less complex songs. That would fit with the concept of selection for “honest signals”—that is, signals that convey information about an individual's underlying genetic, developmental, or epigenetic condition that cannot be cheated.
“In this case, we thought that the region of the brain responsible for this important signal would be sensitive to perturbations because males that experience these challenges in early life probably experience other physiological and somatic changes that make them poorer quality compared to unstressed males,” Merrill said.
However, the team found that male zebra finches who were stressed by KLH early in life grew up to sing more complex songs that contained more elements.
- Bird 198 has seven elements in his song (none of which are repeated) and six song phrases.
- Bird 295 has 12 elements in his song, but three are repeated for a total of nine unique elements.
And when female zebra finches met the KLH-treated males, they sent more signals of interest (zebra finches “flirt” by hopping and rubbing their beaks on something, like a perch or branch).
“This then begs the question: Do our results mean that for these birds song is no longer an honest signal, or is something else at play?” Merrill said.
In addition to the surprising way early-life stress affected song complexity, the team found negative associations between beak color and song complexity in the birds that received KLH. In other words, the beaks of stressed birds became duller, while song complexity increased.
“This is an area I’ve become really interested in—how early-life experiences influence not just individual traits but the relationship between traits.
“It’s going to require additional work to really begin to uncover and understand the mechanisms responsible for the increased song complexity and the changes to the associations between the two signals,” Merrill said. “On top of that, there are lots and lots of moving parts in play here—the specific type of stressor, the stage of development, and the duration of the stressor, for example. All of these components almost certainly play a role in determining what the short- and long-term outcomes are for an individual.”
The paper “Early-life immune activation increases song complexity and alters phenotypic associations between sexual ornaments” is available online. DOI: 10.1111/1365-2435.12916 . Oklahoma State graduate student Madeleine Naylor, University of Illinois graduate student Tara Stewart, and Oklahoma State undergraduate students Merria Dalimonte and Sean McLaughlin also contributed to the research.
This work was supported by a grant from the National Institutes of Health (1R15HD066378-01).
Contact: Loren Merrill, [email protected] , 217-244-8187 or 805-450-1744 (cell)
Written by : Trish Barker, [email protected] , 217-300-2327
Illustration by : Danielle Ruffatto
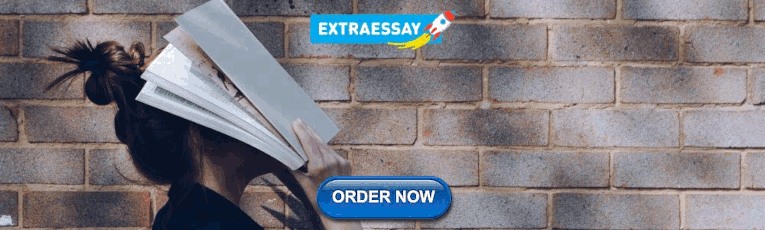
additional actions

An official website of the United States government
The .gov means it’s official. Federal government websites often end in .gov or .mil. Before sharing sensitive information, make sure you’re on a federal government site.
The site is secure. The https:// ensures that you are connecting to the official website and that any information you provide is encrypted and transmitted securely.
- Publications
- Account settings
- My Bibliography
- Collections
- Citation manager
Save citation to file
Email citation, add to collections.
- Create a new collection
- Add to an existing collection
Add to My Bibliography
Your saved search, create a file for external citation management software, your rss feed.
- Search in PubMed
- Search in NLM Catalog
- Add to Search
Spatial memory and the avian hippocampus: research in zebra finches
Affiliations.
- 1 Neuroethology, Bielefeld University, PO Box 100131, 33501 Bielefeld, Germany. Electronic address: [email protected].
- 2 Dept. Psychology, Keio University, Mita 2-15-45, Minato-Ku, Tokyo, Japan. Electronic address: [email protected].
- 3 Neuroethology, Bielefeld University, PO Box 100131, 33501 Bielefeld, Germany. Electronic address: [email protected].
- PMID: 22613455
- DOI: 10.1016/j.jphysparis.2012.05.002
The aim of the present review is to show that spatial learning and memory is not a specialty of just a few avian species, and to describe the role of the avian hippocampus in spatial learning, memory and orientation. Based on our own research in zebra finches, we try to give an (not complete and probably biased) overview of this topic, and we also discuss the question of functional equivalence of hippocampus in birds and in mammals in that we question how far theories developed for mammalian hippocampus can also be applied to the avian hippocampal formation.
Copyright © 2012 Elsevier Ltd. All rights reserved.
PubMed Disclaimer
Similar articles
- Effects of hippocampal lesions on acquisition and retention of spatial learning in zebra finches. Watanabe S, Bischof HJ. Watanabe S, et al. Behav Brain Res. 2004 Nov 5;155(1):147-52. doi: 10.1016/j.bbr.2004.04.007. Behav Brain Res. 2004. PMID: 15325788
- Hippocampal activation of immediate early genes Zenk and c-Fos in zebra finches (Taeniopygia guttata) during learning and recall of a spatial memory task. Mayer U, Watanabe S, Bischof HJ. Mayer U, et al. Neurobiol Learn Mem. 2010 Mar;93(3):322-9. doi: 10.1016/j.nlm.2009.11.006. Epub 2009 Nov 26. Neurobiol Learn Mem. 2010. PMID: 19944176
- Pattern discrimination is affected by entopallial but not by hippocampal lesions in zebra finches. Watanabe S, Mayer U, Bischof HJ. Watanabe S, et al. Behav Brain Res. 2008 Jul 19;190(2):201-5. doi: 10.1016/j.bbr.2008.02.035. Epub 2008 Mar 4. Behav Brain Res. 2008. PMID: 18384892
- [Spatial memory and hippocampus in aves]. Watanabe S. Watanabe S. Shinrigaku Kenkyu. 2000 Jun;71(2):144-56. doi: 10.4992/jjpsy.71.144. Shinrigaku Kenkyu. 2000. PMID: 10998787 Review. Japanese.
- [Spatial learning and the hippocampus]. Santín LJ, Rubio S, Begega A, Miranda R, Arias JL. Santín LJ, et al. Rev Neurol. 2000 Sep 1-15;31(5):455-62. Rev Neurol. 2000. PMID: 11027098 Review. Spanish.
- Dim artificial light at night alters immediate early gene expression throughout the avian brain. Hui CK, Chen N, Chakraborty A, Alaasam V, Pieraut S, Ouyang JQ. Hui CK, et al. Front Neurosci. 2023 Jul 4;17:1194996. doi: 10.3389/fnins.2023.1194996. eCollection 2023. Front Neurosci. 2023. PMID: 37469841 Free PMC article.
- Development of subdomains in the medial pallium of Xenopus laevis and Trachemys scripta : Insights into the anamniote-amniote transition. Jiménez S, Moreno N. Jiménez S, et al. Front Neuroanat. 2022 Nov 3;16:1039081. doi: 10.3389/fnana.2022.1039081. eCollection 2022. Front Neuroanat. 2022. PMID: 36406242 Free PMC article.
- Spatial cognition and the avian hippocampus: Research in domestic chicks. Morandi-Raikova A, Mayer U. Morandi-Raikova A, et al. Front Psychol. 2022 Sep 23;13:1005726. doi: 10.3389/fpsyg.2022.1005726. eCollection 2022. Front Psychol. 2022. PMID: 36211859 Free PMC article. Review.
- Morphology, biochemistry and connectivity of Cluster N and the hippocampal formation in a migratory bird. Heyers D, Musielak I, Haase K, Herold C, Bolte P, Güntürkün O, Mouritsen H. Heyers D, et al. Brain Struct Funct. 2022 Nov;227(8):2731-2749. doi: 10.1007/s00429-022-02566-y. Epub 2022 Sep 17. Brain Struct Funct. 2022. PMID: 36114860 Free PMC article.
- Neural Substrates of Homing Pigeon Spatial Navigation: Results From Electrophysiology Studies. Hough GE. Hough GE. Front Psychol. 2022 Apr 6;13:867939. doi: 10.3389/fpsyg.2022.867939. eCollection 2022. Front Psychol. 2022. PMID: 35465504 Free PMC article. Review.
Publication types
- Search in MeSH
LinkOut - more resources
Full text sources.
- Elsevier Science
Other Literature Sources
- scite Smart Citations
- MedlinePlus Health Information
- Citation Manager
NCBI Literature Resources
MeSH PMC Bookshelf Disclaimer
The PubMed wordmark and PubMed logo are registered trademarks of the U.S. Department of Health and Human Services (HHS). Unauthorized use of these marks is strictly prohibited.
We use cookies to improve your experience with our site. Accept and close | More info .
- Jump to main content
- Jump to navigation
- nature.com homepage
- Publications A-Z index
- Browse by subject
- Submit manuscript
Zebra finch genome
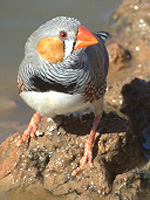
In this focus
Current research, zebra finch genome papers from genome research.
- Zebra finch thematic series in BMC journals
The zebra finch can communicate through learned vocalizations. It is therefore an important model for human neuroscience. Now, genome analysis is revealing that vocalization engages gene-regulatory networks in the zebra finch brain, altering the expression of long non-coding RNAs, microRNAs and transcription factors. In addition, comparative approaches are providing evidence of the rapid molecular evolution of genes regulated during song experience. This web focus celebrates the publication of the zebra finch genome, and showcases recent papers on vocal learning and memory published in Nature or simultaneously in Genome Research and BioMed Central journals.
Image: Simon Griffith, Fowlers Gap, Australia
The genome of a songbird Free access
Warren, W. C. et al.
Nature 464 , 757–762 (1 April 2010) doi :10.1038/nature08819
Abstract | Full Text | PDF | Supplementary Information
Rapid spine stabilization and synaptic enhancement at the onset of behavioural learning Free access -->
Todd F. Roberts, Katherine A. Tschida, Marguerita E. Klein & Richard Mooney
Nature 463 , 948–952 (18 February 2010) doi :10.1038/nature08759
De novo establishment of wild-type song culture in the zebra finch Free access -->
Olga Fehér, Haibin Wang, Sigal Saar, Partha P. Mitra & Ofer Tchernichovski
Nature 459 , 564–568 (28 May 2009) doi :10.1038/nature07994
News and Views
Animal behaviour: Birdsong normalized by culture Free access -->
W. Tecumseh Fitch
Nature 459 , 519–520 (28 May 2009) doi :10.1038/459519a
Full Text | PDF
Sleep and sensorimotor integration during early vocal learning in a songbird Free access -->
Sylvan S. Shank & Daniel Margoliash
Nature 458 , 73–77 (5 March 2009) doi :10.1038/nature07615
Neural processing of auditory feedback during vocal practice in a songbird Free access -->
Georg B. Keller & Richard H. R. Hahnloser
Nature 457 , 187–190 (8 January 2009) doi :10.1038/nature07467
Using temperature to analyse temporal dynamics in the songbird motor pathway Free access -->
Michael A. Long & Michale S. Fee
Nature 456 , 189–194 (13 November 2008) doi :10.1038/nature07448
Neuroscience: Cool songs Free access -->
Chris M. Glaze & Todd Troyer
Nature 456 , 187–188 (13 November 2008) doi :10.1038/456187a
Precise auditory-vocal mirroring in neurons for learned vocal communication Free access -->
J. F. Prather, S. Peters, S. Nowicki & R. Mooney
Nature 451 , 305–310 (17 January 2008) doi :10.1038/nature06492
Behavioural neuroscience: Neurons of imitation Free access -->
Ofer Tchernichovski & Josh Wallman
Nature 451 , 249–250 (17 January 2008) doi :10.1038/451249a
Performance variability enables adaptive plasticity of 'crystallized' adult birdsong Free access -->
Evren C. Tumer & Michael S. Brainard
Nature 450 , 1240–1244 (20 December 2007) doi :10.1038/nature06390
Sex bias and dosage compensation in the zebra finch versus chicken genomes: general and specialized patterns among birds
Itoh, Y. et al.
Genome Res. 20 , 512–518 doi :10.1101/gr.102343.109
Copy number variation, chromosome rearrangement and their association with recombination during avian evolution
Völker, M. et al.
Genome Res. 20 , 503–511 doi :10.1101/gr.103663.109
Pronounced inter and intra-chromosomal variation in linkage disequilibrium across the zebra finch genome
Stapley, J. et al.
Genome Res. 20 , 496–502 doi :10.1101/gr.102095.109
The recombination landscape of the zebra finch Taeniopygia guttata genome
Backström, N. et al.
Genome Res. 20 , 485–495 doi :10.1101/gr.101410.109
Main navigation
Journal content.
- Journal home
- Current issue
- Nature News
- Collections
- Biological sciences
- Earth and environment
Physical sciences
- Science and politics
- Science, art and culture
- News Specials
Journal information
- Contact the journal
- About the editors
- Nature family of journals
- History of the Journal Nature
- For advertisers
- For librarians
- Formatting guide
- Publication policies
- For referees
- Online submission
- Nature Awards
- Nature history
Nature Research Services
- Advertising
- work@ Nature Research
- Reprints & permissions
- Authors & referees
Nature Research Resources
- Gateways & databases
- Nature Reports
- Nature Network
- nature.com blogs
- Nature Conferences
- Scitable Genetics
Nature Research Journals
By subject area.
- Drug discovery
- Biotechnology
- Methods & Protocols
Clinical Practice & Research
- Cardiovascular medicine
- Endocrinology
- Gastroenterology & Hepatology
- Pathology & Pathobiology
Earth & Environment
- Earth sciences
- Evolution & Ecology
Life sciences
- Development
- Medical research
- Microbiology
- Molecular cell biology
- Neuroscience
- Pharmacology
- Systems biology
by A - Z Index
Extra navigation, journal services.
- Sign up for e-alerts
- Recommend to your library
- RSS newsfeeds
- Nature in the news (external link)
nature jobs
Recruitment of faculty and staff for the center for stem cell & ageing of the academy of medical sciences at zhengzhou university.
- The Academy of Medical Sciences of Zhengzhou University
Principal Investigator at IDG / McGovern Institute for Brain Research at Peking University
- Peking University (PKU)
- ISSN : 0028-0836
- EISSN : 1476-4687
- Accessibility statement
- Privacy policy
- Use of cookies
- Legal notice
- Nature jobs
- Nature Asia
- Nature Education
- RSS web feeds
- partner of AGORA, HINARI, OARE, INASP, ORCID, CrossRef, COUNTER and COPE

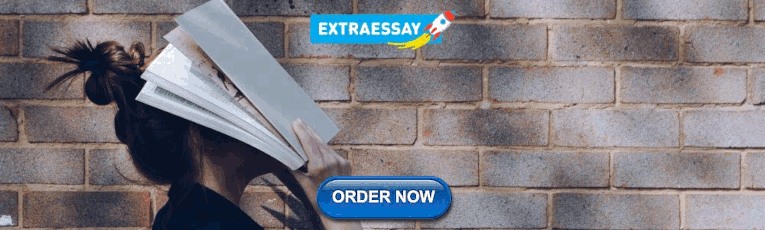
COMMENTS
Temporal trends and general overview of cognitive research in zebra finches. In the abstract screening stage (Fig. 1), we found that 78% of the zebra finch cognitive studies were about song learning, while 19% concerned other learning domains and only 3% studied both at the same time. This shows a clear bias towards song learning among the ...
The zebra finch (Taeniopygia guttata) is a socially monogamous and colonial opportunistic breeder with pronounced sexual differences in singing and plumage coloration.Its natural history has led to it becoming a model species for research into sex differences in vocal communication, as well as behavioral, neural and genomic studies of imitative auditory learning.
The zebra finch (Taeniopygia guttata) is a small songbird from the perching-bird order (Passeriformes) that is used as a model organism for the study of subjects including song production ...
The zebra finch (Taeniopygia guttata) is a socially monogamous and colonial opportunistic breeder with pronounced sexual differences in singing and plumage coloration.Its natural history has led to it becoming a model species for research into sex differences in vocal communication, as well as behavioral, neural and genomic studies of imitative auditory learning.
Neurons regulate their intrinsic physiological properties, which could influence network properties and contribute to behavioral plasticity. Recording from adult zebra finch brain slices we show ...
A detailed analysis of male song structure in zebra finches shows how females use particular features of songs as indicators of male quality in species that learn only one song. ... This research ...
Zebra finches are a widely used model species for neurobehavioral research, in particular in relation to song development and auditory processing. Males learn their songs from a tutor. Females don't sing, but do develop learned song preferences. Regardless of the differences, both sexes exchange calls in social interactions.
The zebra finch (Taeniopygia guttata), a representative oscine songbird species, has been widely studied to investigate behavioral neuroscience, most notably the neurobiological basis of vocal learning, a rare trait shared in only a few animal groups including humans.In 2019, an updated zebra finch genome annotation (bTaeGut1_v1.p) was released from the Ensembl database and is substantially ...
The zebra finch (Taeniopygia guttata), a representative oscine songbird species, has been widely studied to investigate behavioral neuroscience, most notably the neurobiological basis of vocal learning, a rare trait shared in only a few animal groups including humans. In 2019, an updated zebra finch genome annotation (bTaeGut1_v1.p) was ...
The Australian Zebra Finch is widely used by researchers and teachers in many scientific disciplines where it is the preferred subject for investigations ranging from anatomy and physiology to ...
This new research was able to capture variation that previous studies of the brain couldn't, and suggests that the musical mind meanders when zebra finches nod off. For Mindlin, this was huge.
Using in vivo electrophysiology in hummingbirds and zebra finches, Gaede et al. reveal 3-dimensional maps of the body within two discrete nuclei of the forebrain. These new data also show that feathers of the leading edge of the wing and from the skin of the hindlimb are acutely sensitive to force, including air puffs associated with flight.
The origin of the published research focused on the Zebra Finch from 2000 to 2020. From Web of Science (Clarivate Analytics), accessed on the topic search "Taeniopygia guttata" on 18 December 2020 ...
The zebra finch is a popular model organism among neurobiologists interested in vocal learning. It's an ability that zebra finches and a few other birds share with primates, including humans ...
The zebra finch (Taeniopygia guttata) is a socially monogamous and colonial opportunistic breeder with pronounced sexual differences in singing and plumage coloration.Its natural history has led to it becoming a model species for research into sex differences in vocal communication, as well as behavioral, neural and genomic studies of imitative auditory learning.
Zebra Finches are logistically amenable to this kind of study as environmental conditions of parents and offspring can be experimentally manipulated in captivity, and there has been research on Zebra Finches into the adaptive role of parental effects resulting from nutrition [64,65] and temperature , as well as much discussion about the ...
The zebra finch, Taeniopygia guttata, is an estrildid finch common in Central Australia, which occurs in most of the Australian continent and up through parts of Indonesia. ... 2013), and detailed, current protocols for using zebra finches in research can be accessed online (Cold Spring Harbor Press, 2014).
Over the past 50 years, songbirds have become a valuable model organism for scientists studying vocal communication from its behavioral, hormonal, neuronal, and genetic perspectives. Many advances in our understanding of vocal learning result from research using the zebra finch, a close-ended vocal learner. We review some of the manipulations used in zebra finch research, such as isolate ...
In zebra finches, song development occurs in two overlapping stages. From roughly 15 days to 60 days after the birds hatch, they learn songs from older "tutor" males. ... The research team hypothesized that stress to the birds' immune system during this early stage could put those key nuclei at risk, causing the birds to develop less ...
The Australian zebra finch is used worldwide in several research fields (e.g. neurobiology, physiology, behaviour, ecology and evolution) as individuals are easy to maintain and breed in captivity. [12] Zebra finches are more social than many migratory birds, generally traveling in small bands and sometimes gathering in larger groups. [13]
The aim of the present review is to show that spatial learning and memory is not a specialty of just a few avian species, and to describe the role of the avian hippocampus in spatial learning, memory and orientation. Based on our own research in zebra finches, we try to give an (not complete and probably biased) overview of this topic, and we ...
Zebra finch thematic series in BMC journals. The zebra finch can communicate through learned vocalizations. It is therefore an important model for human neuroscience. Now, genome analysis is ...
Chapter 23 - Zebra Finches in Biomedical Research. Zebra finches (Taenopygia guttata, formerly Poephila guttata) are small, colorful songbirds that have been favored by bird fanciers since the nineteenth century. In captivity, zebra finches are prolific breeders and robust 'easy keepers'; these characteristics, along with a diurnal activity ...
"It appears that the declarations of 2020, 2021 and 2022 authorising the trapping of finches of the species concerned during the 'research periods' of 2020, 2021 and 2022 do not contain any ...