Thank you for visiting nature.com. You are using a browser version with limited support for CSS. To obtain the best experience, we recommend you use a more up to date browser (or turn off compatibility mode in Internet Explorer). In the meantime, to ensure continued support, we are displaying the site without styles and JavaScript.
- View all journals
- Explore content
- About the journal
- Publish with us
- Sign up for alerts
- Open access
- Published: 28 August 2024
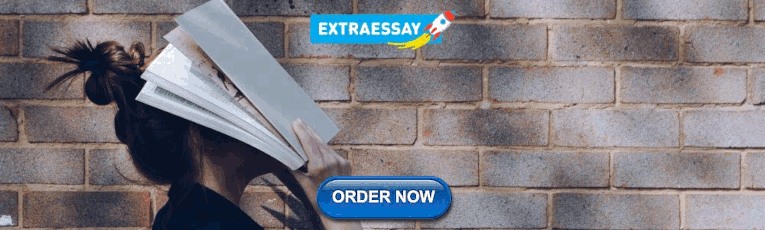
A systematic review and Bayesian meta-analysis provide evidence for an effect of acute physical activity on cognition in young adults
- Jordan Garrett ORCID: orcid.org/0000-0002-5893-5904 1 , 2 ,
- Carly Chak 1 , 2 ,
- Tom Bullock 1 , 2 &
- Barry Giesbrecht ORCID: orcid.org/0000-0002-1976-1251 1 , 2
Communications Psychology volume 2 , Article number: 82 ( 2024 ) Cite this article
10 Altmetric
Metrics details
- Cognitive neuroscience
- Human behaviour
Physical exercise is a potential intervention for enhancing cognitive function across the lifespan. However, while studies employing long-term exercise interventions consistently show positive effects on cognition, studies using single acute bouts have produced mixed results. Here, a systematic review and meta-analysis was conducted to determine the impact of acute exercise on cognitive task performance in healthy young adults. A Bayesian hierarchical model quantified probabilistic evidence for a modulatory relationship by synthesizing 651 effect sizes from 113 studies from PsychInfo and Google Scholar representing 4,390 participants. Publication bias was mitigated using the trim-and-fill method. Acute exercise was found to have a small beneficial effect on cognition ( g = 0.13 ± 0.04; BF = 3.67) and decrease reaction time. A meta-analysis restricted to executive function tasks revealed improvements in working memory and inhibition. Meta-analytic estimates were consistent across multiple priors and likelihood functions. Physical activities were categorized based on exercise type (e.g., cycling) because many activities have aerobic and anaerobic components, but this approach may limit comparison to studies that categorize activities based on metabolic demands. The current study provides an updated synthesis of the existing literature and insights into the robustness of acute exercise-induced effects on cognition. Funding provided by the United States Army Research Office.
Similar content being viewed by others
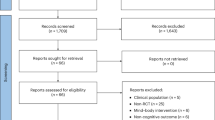
An umbrella review of randomized control trials on the effects of physical exercise on cognition
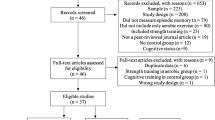
Aerobic exercise improves episodic memory in late adulthood: a systematic review and meta-analysis

Systematic review and meta-analysis investigating moderators of long-term effects of exercise on cognition in healthy individuals
Introduction.
A single bout of exercise induces a cascade of neuromodulatory changes that influence multiple brain systems 1 , 2 . This includes an increase in the synthesis of neurotransmitters (e.g., acetylcholine, dopamine, GABA, glutamate) and neurotrophic factors (e.g., BDNF), which can occur in a brain-region-specific manner (see ref. 1 for review). Given these impacts on the brain, it would be reasonable to hypothesize that single brief bouts of exercise are associated with changes in performance across a range of cognitive domains. Consistent with this hypothesis, there is abundant evidence that attention 3 , 4 , 5 , 6 , working memory 7 , 8 , 9 , 10 , 11 , decision making 12 , 13 , and cognitive control 14 , 15 are facilitated by brief bouts of physical exercise. However, there is also evidence suggesting that exercise has little or no effect on cognitive task performance. For instance, Komiyama et al. 16 observed no difference in accuracy on a spatial delayed response task between exercise and rest conditions. Further, working memory performance has been shown to remain unchanged either during or after a single bout of exercise 6 . The discrepant pattern of results in the literature investigating the link between exercise and performance on cognitive tasks is surprising given the consistent and robust physiological effects of even brief bouts of physical activity. However, it is unclear whether this limited impact of exercise on performance reflects the true state of affairs or whether the apparent lack of robust influence is due to vast empirical discrepancies across studies in the literature. Studying the impact of single exercise sessions on cognition can provide insight into how changes in our body’s physiological state impact behavior. This understanding can then guide the creation of more effective longer-term exercise interventions, which essentially involve regularly repeating brief exercise sessions over an extended period.
Meta-analytic techniques are a set of powerful tools that can expose dominant trends within a methodologically heterogeneous literature. There is a consensus amongst narrative reviews and previous meta-analyses that an acute bout of exercise has a small positive influence on behavioral performance 1 , 17 , 18 , 19 , 20 , 21 , 22 , 23 . The nature of this effect is moderated by exercise protocol, cognitive tasks, and participant characteristics. For instance, Lambourne & Tomporowski 20 observed that task performance during exercise was dependent on exercise modality, the type of cognitive task, and when it was completed relative to exercise onset. Similarly, post-exercise performance was moderated by exercise modality and the type of cognitive task. Chang et al. 18 reported that post-exercise cognitive performance was influenced by exercise intensity, duration, and the time of cognitive test relative to exercise cessation. Interestingly, the authors found that study sample age was a significant moderator, where larger positive effects were found for high school (14–17 years), adult (31–60 years), and older adult (>60 years) samples compared to elementary (6–13 years) and young adult (18–30 years) samples. Multiple meta-analyses have observed that the effect of exercise is dependent on cognitive domain, with measures of executive function, attention, crystallized intelligence, and information processing speed showing the largest gains 18 , 19 , 24 , 25 , 26 . Further, there is evidence that exercise has a differential influence on the speed and accuracy of cognitive processes. McMorris et al. 21 observed that acute, intermediate exercise facilitated response times on working memory tasks, while accuracy was compromised. In contrast, exercise has been shown to boost both the accuracy and speed of cognitive control 23 . Altogether, it is important to consider cognitive task, participant, and physical activity characteristics to develop a holistic model of the relationship between exercise and cognition.
While these earlier meta-analyses have provided unique insights into understanding the relationship between acute exercise and cognition, they have two major limitations. First, the most recent holistic quantitative synthesis of the extant literature was published over a decade ago 18 . Meanwhile, the exercise and cognition literature has grown drastically. According to the electronic database Web of Science, almost 6,000 articles associated with the search term “exercise and cognition” have been published since this last holistic meta-analysis. In addition, more recent meta-analyses have primarily focused on executive processes 19 , 22 , 26 , 27 . Thus, previous models may provide an outdated and limited account of exercise-induced influences on other aspects of cognition, such as perception, long-term memory, and learning. Second, previous meta-analytic approaches employed frequentist statistical methods, which are based on a decision threshold rather than a characterization of the relevant evidence. As a result, it is possible that acute exercise and moderator variables are deemed to have a significant influence on task performance despite the fact that there may only be a small degree of probabilistic evidence in favor of this notion. In addition, relying on a decision threshold prevents these models from conveying the likelihood that an exercise protocol elicits a change in cognitive task performance. Past frequentist meta-analytic models also treated heterogeneity parameters as a fixed quantity and utilize only a point estimate, which can lead to an underestimation of the variability either between or within studies 28 , 29 , 30 , 31 . This is especially true when the number of modeled studies is low 32 , 33 , 34 . When considered together, there is a clear need for an updated meta-analysis using an approach that addresses these limitations.
The current study addressed these limitations in two ways. First, a comprehensive literature search was conducted spanning the years 1995–2023. To quantify the influence of exercise on cognition in young healthy adults, the search was limited to non-clinical studies whose subjects were between 18–45 years old. The analysis focused on subjects within this age range since exercise research has predominantly been dedicated toward studying the effects in children and older adults 35 , 36 . Studies were required to be experimental in nature, and consist of both an acute exercise manipulation and cognitive task measurements. A broad range of cognitive domains encompassing tasks probing perception to executive function were included in the meta-analysis. Similarly, a wide range of exercise types and testing contexts were included. For example, traditional laboratory exposures to exercise (e.g., cycling, running) and sport activities in real-world settings were viable candidates for analysis. By casting a wide net, the current study provides a large scope and updated summary of the current state of the exercise and cognition literature.
Second, the current study uses a Bayesian meta-analytic approach to synthesize studies across the acute exercise and cognition literature. The Bayesian approach affords a flexible modeling framework that uses reported effect sizes to characterize the relative evidence in favor of a modulatory account. Inherently, a random effects meta-analytic model is hierarchical in nature, making it well suited for Bayesian methods. When utilized within this statistical framework, priors are placed on parameters at the highest level of the model such as the estimated pooled effect size and measures of heterogeneity. This approach has several advantages compared to its frequentist counterpart. First, the use of priors on heterogeneity parameters can attenuate the underestimation of variation both between and within studies 37 , 38 , leading to a clearer understanding of sources of heterogeneity and an increased precision when estimating the pooled effect size 39 . Furthermore, priors provide additional constraints on low-level parameter estimates and a greater degree of “shrinkage” of outliers towards the overall pooled effect size or mode(s) of grouping variables 39 , 40 . Therefore, a Bayesian meta-analysis is more robust to outliers and can be more conservative when proper priors are employed. Second, the method yields a posterior distribution for all parameter estimates. This grants the capability of directly modeling the degree of uncertainty in heterogeneity estimates 37 . Posterior distributions can be used to compute the probability that an exercise protocol elicits a change in task performance of a given magnitude (e.g., large effect size). Compared to the approximation of p -values and confidence intervals, which require additional assumptions for hierarchical models, calculating the high-density interval (HDI), which indicates the most credible outcomes in the posterior distribution, for complex hierarchical models is seamless 39 . Third, it is possible to incorporate knowledge from previous meta-analyses when constructing prior distributions. This affords the ability to quantitatively compare the observed data to the predictions of previous models.
Considering the results of past meta-analyses, exercise was expected to have a small positive influence on cognition. Cognitive task and exercise characteristics were anticipated to moderate this relationship, as evidenced by nonzero parameter estimates, reflecting the selective nature of exercise-induced effects. Model comparisons were conducted to evaluate how moderator inclusion improved predictive performance, and robustness of parameter estimates were determined by employing multiple priors and likelihood functions.
Literature search
Studies investigating the impact of an acute bout of exercise on cognition were obtained through searches of the electronic databases PsychInfo and Google Scholar according to the PRISMA guidelines 41 . On 09 September 2023, databases were queried using a search string that combined the following physical activity and cognitive domain keywords: [“exercise” OR “physical activity” OR “physical exertion” OR “physical fatigue”] AND [“perception” OR “attention” OR “working memory” OR “executive function” OR “memory” OR “decision making” OR “motor skill” OR “skill acquisition” OR “language” OR “reasoning”]. For the PsychInfo search, the filters “journal article”, “English”, “empirical study”, “human”, and “peer reviewed” were applied. Search results were limited to studies published between 1995 and 2023 and whose subjects were between 18 and 45 years of age. Note, this literature search and analysis were not preregistered, nor was a review protocol prepared prior to the literature search.
Eligibility criteria
Studies were deemed eligible for inclusion in the meta-analysis if they met all of the following criteria: assessed the influence of an acute bout of exercise on cognition, compared the effects of exercise with an active and/or passive control group(s), utilized cognitive tasks that measured reaction time (RT) and/or accuracy, tested cognition either during, pre-, or post-exercise and consisted of cognitively normal subjects. Note, an acute bout was defined as an instance of physical activity that occurred within a single 24-hour period 18 . Two researchers independently screened records based on their title, abstract, and full text. In the case of discrepancies, a third researcher resolved them by reading the full-text.
Data extraction and coding
Information concerning experimental design and procedures, exercise details (i.e., type, intensity, duration), and sample characteristics were extracted from the final list of studies by a single researcher. Means and standard deviations of accuracy and/or RT measures on all cognitive tasks were inserted into an electronic spreadsheet for the calculation of effect sizes. The primary outcome measures for each domain were inserted separately if a task assessed multiple cognitive domains. Regarding studies that probed cognition at multiple time points during or post-exercise, measures for each time point were also recorded separately. If the statistics necessary for calculating effect sizes were not reported in the full-text of the article, the authors were contacted and asked to provide them.
All effect sizes were categorized into one of seven cognitive domains that were generally based on the DSM-5 42 : executive function, information processing, perception, attention, learning, motor skills, and memory. The classification criteria used for categorizing a cognitive task into a domain is provided in the Supplementary Table 1 . To account for variability in the metric used to measure exercise intensity across studies (e.g., ventilatory threshold, heart rate), each intensity was labeled as either light, moderate, or vigorous according to the American College of Sports Medicine guidelines 43 . Exercise durations were grouped into one of five time bins: ≤16 minutes, 20–27 minutes, 30–35 minutes, 40–45 minutes, ≥60 minutes. In the event that a study did not provide the exercise duration, its time bin was labeled as “not provided”. Exercise types were based on the modality reported in each study, yielding the following categorizations: cycling, high intensity interval training (HIIT), running, walking, circuit training, resistance exercise, and sports activity. The latter category encompassed studies that used sports-related exercises that did not fit into the other labels, such as rock climbing or soccer. The time at which cognitive task performance was evaluated relative to exercise was categorized as either during exercise or 0, 15, 20–75, and ≥180 minutes after cessation. Lastly, effect sizes were also coded according to task performance dependent measures (i.e., RT vs accuracy). Note, the levels of each categorical moderator were chosen with the intention of achieving a balance between specificity and statistical power to yield reliable estimates that can inform the design of future exercise studies.
Calculating effect sizes
Cohen’s d effect sizes were calculated for studies that tested cognition pre-/post-exercise without a control condition by dividing the mean change in performance by the standard deviation of the pre-test. If the study included a control group (e.g., rest), the mean change of the control condition was subtracted from the mean change of the exercise condition and divided by the pooled standard deviation of pretest scores 20 , 44 . For studies that tested cognition during, or only after exercise, the mean of the control condition was subtracted from the mean of the exercise condition and divided by the standard deviation of the control condition 21 . All effect sizes were converted into the bias-corrected standardized mean difference, Hedge’s g , by multiplying them by the correction factor \({{\rm{J}}}=1-\frac{3}{4{df}-1}\) where df is the degrees of freedom 45 . The sign of effect sizes for RT and error were reversed to reflect a positive influence of exercise on cognitive task performance. Once effect sizes were extracted from each study, inspection of a funnel plot and Egger’s regression test were conducted to assess the risk of publication bias.
Bayesian hierarchical modeling
The overall effect of exercise on cognition was assessed using a Bayesian hierarchical model 46 , 47 , which was implemented through the R package brms 48 . In the first level of the model, a study’s observed effect size(s) \({\hat{{{\rm{\theta }}}}}_{{ik}}\) was assumed to be an estimate of the true effect size \({{{\rm{\theta }}}}_{k}\) . The observed effect(s) \({\hat{\theta }}_{{ik}}\) were modeled as being sampled from a normally distributed population underlying study k with a mean equivalent to the true effect and a variance of \({{{\rm{\sigma }}}}_{k}^{2}\) . In the second level of the model, the true effect size \({\theta }_{k}\) was assumed to have been drawn from an overarching distribution whose mean represented the overall pooled effect \({{\rm{\mu }}}\) , and whose variance depicted the degree of between-study heterogeneity \({\tau }^{2}\) . The final level of the model contained weakly informative priors. A standard normal prior was used for the pooled effect, while the prior for \({\tau }^{2}\) was a Half-Cauchy distribution with location and scale parameters set to 0 and 0.5, respectively.
Following the main meta-analysis, subgroup analyses were conducted to determine potential moderators of the relationship between exercise and cognitive task performance. More specifically, we analyzed the influence of the following primary moderators: cognitive domain, time of cognitive test relative to exercise, task outcome measure, exercise intensity, duration, and type. The following secondary moderators were also analyzed to determine the influence of study and participant characteristics on the overall pooled effect size: average sample age, body mass index (BMI kg/m 2 ), height (cm), weight (kg), VO2 max (ml/kg/min), percentage of female participants, within- vs between-study design, and publication year. With the exception of publication year and the percentage of female participants, all secondary moderators were mean centered for interpretability. A standard normal distribution was used as a weakly informative prior for the difference in effect sizes between subgroups. When reporting model parameter estimates, we use the [mode ± standard deviation] and the 89% HDI of posterior distribution.
Statistical inference
For all estimated effect sizes, Bayes Factors (BFs) were used to determine the degree of evidence in favor of a difference from zero. BFs were approximated using the reciprocal of the Savage-Dickey density ratio, which was implemented using the function bayesfactor_parameters from the bayestestR package 49 . This method involves dividing the height of the prior distribution for the null value by the height of the posterior distribution at the same value, and represents the credibility of the null value for a parameter once the data has been taken into consideration. BFs were also used to ascertain the predictive performance of subgroup models. After each model was compared to a null counterpart (i.e., moderator excluded) using the function bayesfactor_models , an inclusion BF ( bayesfactor_inclusion ) was estimated to determine if including a moderator improved predictive power 50 . To estimate stable BFs, a large number of sampling iterations (10,000) and warmup samples (2000) were used for each of four chains when estimating model parameters 51 . BFs were interpreted following the guidelines proposed by Jeffreys 52 . A BF between 1 and 3 indicates “anecdotal” evidence for the alternative hypothesis, between 3 and 10 indicates “moderate” evidence, between 10 and 30 indicates “strong” evidence, and greater than 30 indicates “very strong” evidence 39 , 53 , 54 , 55 . The reciprocal of these ranges signifies evidence in favor of the null hypothesis (e.g., 0.33-1 = anecdotal evidence). When conducting subgroup analyses with more than two factors, orthonormal coding was employed to ensure that an identical prior was used for each factor level and that estimated BFs were accurate 56 . Parameter estimates were extracted from all models using the R package emmeans .
Sensitivity analysis
A popular criticism of the Bayesian approach is that priors are chosen subjectively, which in turn can bias parameter estimates and their corresponding BFs 40 , 57 . Although utilizing weakly informative priors mitigates bias, a sensitivity analysis that evaluates the contribution of both priors and the likelihood function must be conducted to determine if the model results are robust 38 , 58 , 59 , 60 . Thus, we replicated the previously described modeling approach with the exception of using two different priors for the overall pooled effect size. The first was a normal distribution with a mean of zero and standard deviation of ½. Since this prior adds greater weight to the probability that exercise has no influence on task performance, we denoted it as the no effect (NE) prior. The second prior was constructed by synthesizing estimates from previous meta-analyses on acute exercise and cognition 18 , 20 , 21 , 22 , 23 , resulting in a normal distribution with a mean of 0.24 and standard deviation of 0.57. This prior was denoted as the positive effect (PE) prior.
The influence of the likelihood function was assessed by modeling study effect sizes as being sampled from a t -distribution. An advantage of using this likelihood function, compared to a normal distribution, is that model parameter estimates are influenced less by outliers 40 . The Half-Cauchy prior was used for the scale of the distribution, while a standard normal prior was used for its mean. For its shape (i.e., degree of freedom) an exponential distribution with a rate equal to 1/29 served as a prior. To determine if meta-analytic estimates were robust across the alternative priors and likelihood function, we visually compared the posterior distributions across models for large deviations 58 .
Description of studies
The literature search yielded 15,900 peer reviewed journal articles, and after removing duplicates 8295 remained. Subsequent an initial screening based off the titles and abstracts, 805 studies were identified as potential candidates for modeling. 113 of these studies were deemed eligible for inclusion in the meta-analysis according to their full-text contents (Fig. 1 ). In total, 642 effect sizes were extracted from these studies, representing data from 4390 subjects. A majority of the effects measured the influence of exercise on executive function ( k = 434) and attention ( k = 109). Fewer effects were measured during exercise ( k = 82) relative to after the cessation of exercise ( k = 560). Visual inspection of a funnel plot suggested that the effect sizes were distributed symmetrically (Fig. 2a ), however there was very strong evidence for asymmetry according to Egger’s regression intercept ( \({{\rm{\beta }}}\) = 1.18 ± 0.25; HDI = [0.78, 1.58]; BF = 253.24) suggesting the presence of publication bias. This was addressed by employing the trim and fill approach, which imputes low-precision effect sizes until the funnel plot is symmetrical 61 .
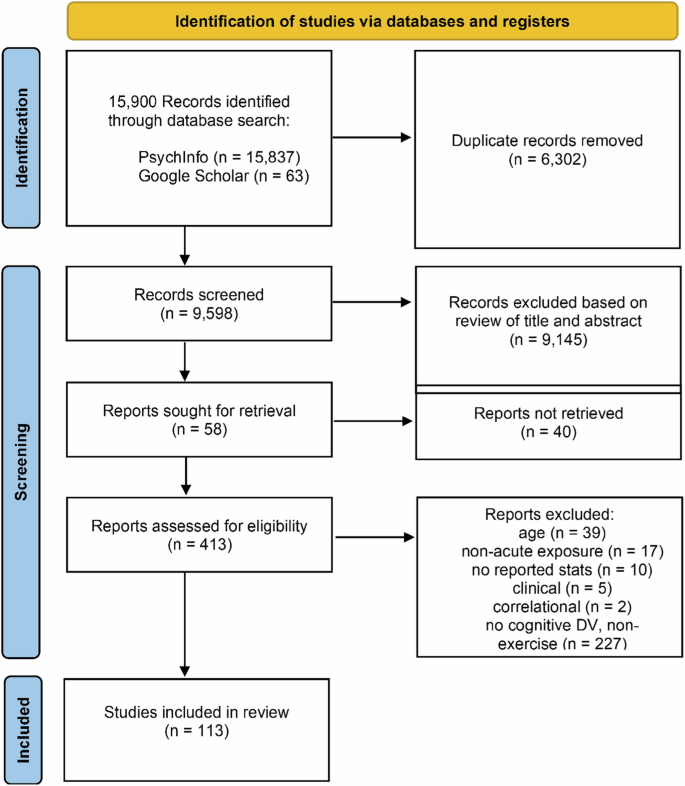
A total of 113 studies were deemed eligible for meta-analytic modeling.

a Funnel plot of 642 study effect sizes (black circles). Imputed effect sizes after using the trim and fill method are represented by the unfilled circles ( \(n=9\) ). Vertical blue line indicates the estimated pooled effect sizes, while dashed black lines represent a pseudo 95% confidence limits. b Posterior distribution of estimated pooled effect. Horizontal black line indicates bounds of 89% HDI derived using \(n=651\) effect sizes. c Empirical cumulative density function of distribution in b , where the dashed black line indicates the pooled effect. d Representation of using the Savage-Dickey ratio to calculate BFs. The density of the null value in the prior distribution (red) is divided by its density in the posterior distribution (blue) to yield probabilistic evidence in favor of the alternative hypothesis. e Posterior distributions of between and within study heterogeneity.
Overall effect
The meta-analysis indicated that there was moderate evidence for an acute bout of exercise to have a small positive influence on overall performance across cognitive domains ( g = 0.13 ± 0.04; HDI = [0.06, 0.20]; BF = 3.67) (Fig. 2 b, d ) . According to the posterior distribution, there was a low probability that the estimated pooled effect was less than or equal to zero ( p = 0.01) and an 80% chance that the effect size fell between the range of 0 to 0.2 (Fig. 2c ). There was a large amount of heterogeneity within ( \({{{\rm{\tau }}}}_{{within}}\,\) = 0.65 ± 0.03; HDI = [0.60, 0.70]; \({I}_{{within}}^{2}\,\) = 81.19%) and moderate amount between ( \({{{\rm{\tau }}}}_{{between}}\,\) = 0.29 ± 0.05; HDI = [0.20, 0.38]; \({I}_{{between}}^{2}\) = 15.9%) studies (Fig. 2e ). Effect size estimates for each individual study are presented in Table 1 .
Subgroup analyses
Primary subgroup analyses revealed that acute exercise reduced RT on cognitive tasks ( g = 0.27; HDI = [0.18, 0.36]; BF \(=6.71\times {10}^{3}\) ), but had no impact on accuracy ( g = 0.04; HDI = [−0.04, 0.12]; BF \(=6.15\times {10}^{-2}\) ) (Table 2 ) (Fig. 3a ). Engaging in either cycling ( g = 0.21; HDI = [0.11, 0.32]; BF = \(14.74\) ) or HIIT ( g = 0.73; HDI = [0.40, 1.09]; BF \(=26.05\) ) was found to have an enhancing effect on performance in cognitive tasks (Fig. 3b ). In regard to cognitive domain, there was evidence that acute exercise has a positive influence on executive processes ( g = 0.18; HDI = [0.10, 0.27]; BF \(=36.97\) ). Furthermore, behavioral performance was found to improve immediately after exercise cessation ( g = 0.16; HDI = [0.11, 0.30]; BF \(=4.03\) ) and in response to vigorous intensity exercises ( g = 0.19; HDI = [0.09, 0.28]; BF \(=5.03\) ). Lastly, at least moderate evidence in favor of non-zero parameter estimates were observed for the secondary moderators publication year, within-subjects design, age, percentage of female participants, and weight (Table 3 ).
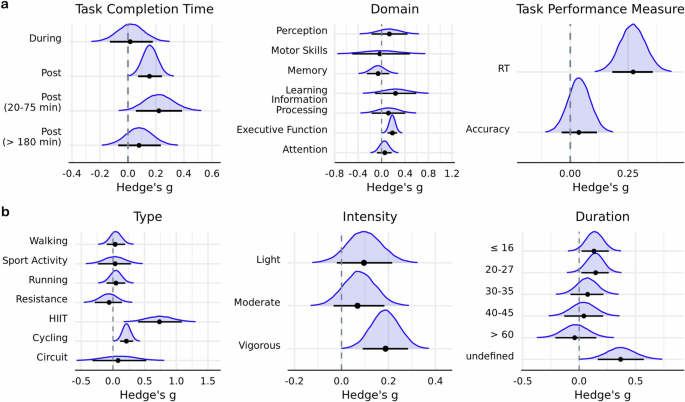
Posterior distributions of a cognitive and b exercise moderators. Horizontal black line indicates the 89% HDI interval, while the black dot represents the mode of the posterior distribution. Intervals derived using \({{\rm{n}}}=651\) effect sizes.
To test for the possible contribution of a learning effect to the estimated overall pooled effect size, a separate meta-analysis was conducted on effects from studies employing a pre-/post-test design ( N effect sizes = 298). Despite the estimated pooled effect size for this subset of data being nominally similar to the estimate for the entire dataset, there was anecdotal evidence in favor of the null hypothesis ( g = 0.15 ± 0.06; HDI = [0.04, 0.24]; BF \(=0.95\) ). Moderator analyses indicated that there was no credible evidence for a difference in this estimated pooled effect size as a function of whether or not a control group was included in the study \(({{{\rm{BF}}}}_{{{\rm{Inclusion}}}}=0.12;\,{\mbox{w}}/{\mbox{control}}:g\,=0.18\,\pm \,0.10;\,{\mbox{HDI}}=[0.03,\,0.33];{\mbox{BF}}=0.51;{\mbox{w}}/{{\rm{o}}} {{\rm{control}}}:g=0.11\pm 0.13;{\mbox{HDI}}=\left[-0.03,\,0.26\right]{\mbox{;BF}}=0.18)\) , suggesting that the estimated influence of exercise on general cognitive performance is not driven by a learning effect.
Model comparisons
Model comparisons were performed to determine if including a moderator improved predictive performance. Only a model that included task performance measure as a moderator was more likely when compared to a null counterpart (BF Inclusion \(=357.10\) ) (Table 4 ). This is likely due to a number of factors. First, acute exercise had a negligible impact on a majority of the levels in each subgroup. Second, there was a high degree of uncertainty in estimated model coefficients, as evidenced by their wide HDI intervals. Third, Bayesian inference automatically penalizes model complexity and favors more parsimonious models. If a model has many parameters, but a majority of them are nonzero, then a simpler counterpart will be favored.
Interactions between moderators
An exploratory analysis was conducted to determine if the influence of moderator variables was contingent on one another. Due to the computationally intensive nature of Bayesian modeling, analyses were limited to the following pairs of moderators: (1) exercise intensity and type, (2) exercise intensity and duration, (3) exercise type and duration, (4) cognitive domain and exercise type, (5) cognitive domain and exercise intensity, (6) cognitive domain and task performance measure, (7) exercise type and task performance measure. Although none of the pairs of interaction models had more predictive power compared to a null counterpart ( \({{{\rm{BF}}}}_{{{\rm{Inclusion}}}}\) : Model 1 = \(3.86\times {10}^{-6}\) ; Model \(2=1.66\times {10}^{-8}\) ; Model \(3=1.84\times {10}^{-3}\) ; Model 4 = 1.31 × 10 −4 ; Model 5 = \(3.91\times {10}^{-5}\) ; Model 6 = \(7.1\times {10}^{-3}\) ; Model 7 = \(7.05\times {10}^{-4}\) ), there were two that had nonzero parameter estimates.
The first model included an interaction between cognitive domain and exercise type. There was evidence in favor of cycling improving performance on tasks that probed attention ( g = 0.34; HDI = [0.14, 0.56]; BF \(=3.05\) ) and executive function ( g = 0.28; HDI = [0.14, 0.40]; BF = \(17.83\) ). HIIT exercises were found to bolster executive function ( g = 1.01; HDI = [0.61, 1.43]; BF \(=155.33\) ), while resistance exercises had an aversive impact on attentional performance ( g = −0.76; HDI = [−1.20, −0.38]; BF \(=18.07\) ) (Fig. 4a ). The second model included an interaction between cognitive domain and task performance measure and indicated that time-dependent measures of executive function are improved ( g = 0.30; HDI = [0.19, 0.39]; BF \(=1.10\times {10}^{3}\) ) (Fig. 4b ).
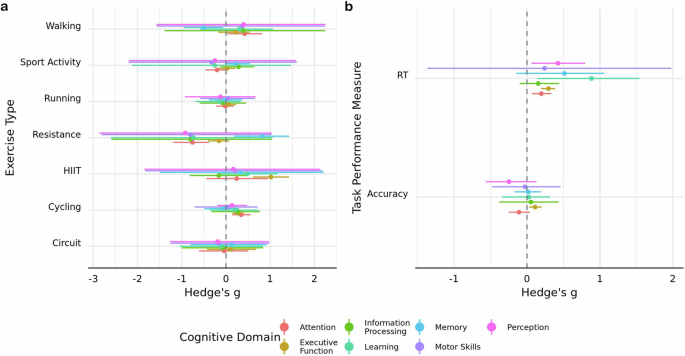
Posterior mode estimates of models including interactions between cognitive domain and a exercise type and b task outcome measure. Width of line represents 89% HDI derived using \({{\rm{n}}}=651\) effect sizes.
Sensitivity analyses
The estimated overall effect of acute exercise on cognition was consistent across the NE prior ( g = 0.13 ± 0.04; HDI = [0.06, 0.20]; BF = 6.52), PE prior ( g = 0.12 ± 0.04; HDI = [0.06, 0.19]; BF \(=6.51\) ), and t likelihood function ( g = 0.12 ± 0.04; HDI = [0.06, 0.18]; BF \(=8.77\) ) (Fig. 5a ). Interestingly, there was anecdotal-to-moderate evidence in favor of the synthesized estimate from previous meta-analyses (i.e., g = 0.24) across the PE (BF \(=3.19\) ), NE (BF \(=2.78\) ), and standard normal (BF \(=5.27\) ) priors. Estimates of between-study heterogeneity were also robust across the NE prior ( \({{{\rm{\tau }}}}_{{between}}\,\) = 0.29 ± 0.05; HDI = [0.20, 0.37]), the PE prior ( \({{{\rm{\tau }}}}_{{between}}\,\) = 0.29 ± 0.05; HDI = [0.21, 0.37]), and t likelihood function ( \({{{\rm{\tau }}}}_{{between}}\,\) = 0.31 ± 0.03; HDI = [0.26, 0.38]) (Fig. 5b ). In contrast, within study heterogeneity was estimated to be lower when using the t likelihood function ( \({{{\rm{\tau }}}}_{{within}}\,\) = 0.17 ± 0.02; HDI = [0.13, 0.19]) relative to the NE ( \({{{\rm{\tau }}}}_{{within}}\,\) = 0.65 ± 0.03; HDI = [0.61, 0.70]) and PE ( \({{{\rm{\tau }}}}_{{within}}\,\) = 0.65 ± 0.02; HDI = [0.60, 0.70]) priors (Fig. 5c ). Note, this reduction reflects the diminished influence of outliers on variance estimates by the inclusion of the shape parameter for the t distribution ( v = 1.52 ± 0.14; HDI = [1.30; 1.73]). In addition to testing the robustness of parameter estimates, a model comparison was conducted to determine if either the null or positive effect prior was more probable given the data. The t -likelihood function was not included in this comparison since it would only indicate if effect sizes were more likely to have been drawn from either a normal or t -distribution. When compared to a standard normal prior, there was anecdotal evidence in favor of both the PE (BF \(=2.56\) ) and NE (BF \(=1.48\) ) priors. Relative to the PE prior, there was anecdotal evidence against the NE prior (BF \(=0.73\) ). Altogether, parameter estimates were not biased by the prior or likelihood function.

Estimates for the a overall pooled effect size, b between- and c within-study heterogeneity parameters across the t-likelihood function (TL), weakly informed, null effect (NE), and positive effect (PE) priors. Color dots represent mode of posterior distributions, while color horizontal line depicts the 89% HDI derived using \(n=651\) effect sizes.
Executive function meta-analysis
Considering that the majority of the effect sizes were from tasks that probed executive function, and that this cognitive domain encompasses multiple sub-domains, a separate meta- analysis and set of meta-regressions were conducted on this subset of data. Categorization criteria from previous meta-analyses and systematic reviews 17 , 24 , 26 were used to classify effect sizes into the following sub-domains of executive function: working memory, cognitive control, decision making, planning, and inhibition. For completeness, the primary moderators used in the main meta-analysis were also tested.
The results were similar to the main meta-analysis. There was very strong evidence in favor of exercise having a small positive influence on overall task performance ( \(g\) = 0.20 ± 0.06; HDI = [0.12, 0.30]; BF \(=29.57\) ), and a moderate degree of heterogeneity both within ( \({{{\rm{\tau }}}}_{{within}}\,\) = 0.51 ± 0.03; HDI = [0.47, 0.57]) and between studies ( \({{{\rm{\tau }}}}_{{between}}\,\) = 0.40 ± 0.06; HDI = [0.30, 0.48]). Subgroup analyses indicated that a model including the moderator task outcome measure had more predictive power relative to a null counterpart (BF Inclusion \(=48.43\) ). Paralleling the main meta-analysis, there was very strong evidence that acute exercise improved RT on executive function tasks ( g = 0.32; HDI = [0.21, 0.42]; BF \(=748.18\) ), but no credible evidence was observed for an effect on accuracy ( g = 0.13; HDI = [0.04, 0.23]; BF \(=0.63\) ) (Table 5 ). Furthermore, there was moderate evidence in favor of a positive impact of exercise on inhibition ( g = 0.21; HDI = [0.09, 0.33]; BF \(=3.14\) ) and working memory ( g = 0.22; HDI = [0.11, 0.34]; BF \(=6.89\) ) (Fig. 6 ). Yet, a model including executive function sub-domain as a moderator did not improve model performance (BF Inclusion \(=7.52\times {10}^{-4}\) ), nor did models including interactions between moderators.
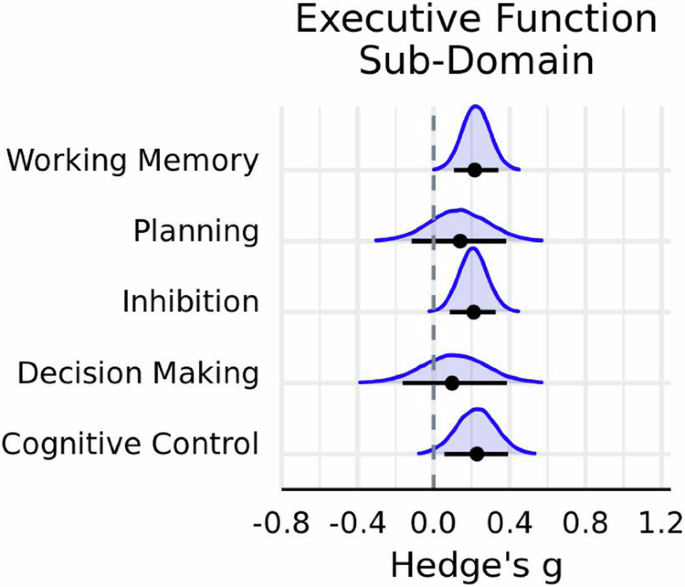
Posterior distributions for executive function sub-domain. Horizontal black line indicates the 89% HDI interval, while the black dot represents the mode of the posterior distribution, which was derived using \(n=433\) effect sizes.
A large corpus of empirical work has examined how a single bout of acute exercise modulates activity within multiple brain systems that underly cognition. Despite inconsistencies in results across empirical studies, there is consensus amongst previous reviews and meta-analyses that acute exercise impacts behavioral performance 18 , 20 , 22 and that this relationship is moderated by both exercise protocol and behavioral task characteristics. The goal of the present work was to address two key limitations of previous meta-analyses. First, recent meta-analyses have a narrower focus, often limited to a single cognitive domain or a specific subset of domains. In contrast, the current meta-analysis presents an updated synthesis of the literature spanning a much wider range of cognitive domains. Second, in contrast to previous frequentist approaches, a Bayesian framework was adopted allowing for the quantification of the degree of evidence in favor of the hypothesis that acute exercise influences cognition in young healthy adults. The current meta-analysis observed that acute exercise has a small positive influence on overall cognitive task performance, and sensitivity analyses indicated that the alternative hypothesis was 6.51–8.77 times more likely than the null across multiple priors and likelihood functions. The magnitude and directionality of this effect were consistent with the results of previous meta-analyses on acute exercise and cognition 18 , 21 , 22 , 62 . Subgroup analyses suggested that this relationship is moderated by task performance measure, cognitive domain, exercise type and intensity, and the time of task completion relative to exercise cessation. Model comparison results indicated that accounting for variations amongst moderator levels did not improve predictive performance. Given our eligibility criteria, these results are limited to healthy individuals between the ages of 18–45 years old.
Similar to McMorris et al. 63 , acute exercise was found to improve RT but no credible evidence was observed for an influence on accuracy. A possible explanation for this differential impact on task outcome measures is that exercise modulates primary motor cortex (M1) excitability 64 . There is accumulating evidence that acute exercise increases M1 intracortical facilitation 65 , 66 , 67 , 68 and inhibition 69 , 70 . Yamazaki et al. 68 observed that the intracortical circuits of both exercised (i.e., legs) and non-exercised (i.e., hand) effectors are modulated by an acute bout of low intensity pedaling. Thus, alterations in the activity of excitatory or inhibitory circuits of non-exercised cortical representations may promote faster RT on cognitive tasks. However, the lack of concurrent changes in corticospinal excitability or motor-evoked potentials suggests that this explanation is not a viable account of a mechanism that engenders faster RTs. An alternative explanation is that exercise increases peripheral and central concentrations of catecholamines, such as norepinephrine, epinephrine, and dopamine, which in turn improves the speed of cognition 1 , 71 , 72 . Indeed, acute exercise has been found to improve response time on choice RT, decision-making, and interference tasks 18 , 73 , 74 . Yet, it is unclear as to why changes in neurochemical levels would facilitate RT but have no impact on accuracy. Considering that physical activity modulates population-level tuning in the sensory areas of nonhuman animals and invertebrates 75 , 76 , 77 , 78 , 79 , 80 , 81 , along with sensory responses in humans 82 , 83 , 84 , it stands to reason that the fidelity of stimulus representations would also be impacted, resulting in changes in accuracy. Changes in the fidelity of feature selective stimulus representations can be determined by applying encoding models to recorded neural activity 83 , 85 , 86 , 87 , 88 , 89 , 90 . For instance, Garrett et al. 91 applied an inverted encoding model to topographical patterns of alpha band activity, recorded at the scalp, while subjects completed a spatial working memory task both at rest and during a bout of moderate intensity cycling. Notably, it was possible to reconstruct spatially selective responses during exercise, and the selectivity of these responses decreased during exercise relative to rest. Therefore, encoding models can be a powerful tool for future research to demystify how the precision of task-relevant representations is influenced by exercise. It is also important to keep in mind that many psychological tasks are relatively simple to do, which can lead to ceiling effects that may mask the influence of exercise on accuracy measures. Lastly, the differential impact of exercise on accuracy and RT may be due to the relative sensitivities of these dependent measures to modulations of different stages of information processing. For example, there is evidence that in near-threshold tasks accuracy is sensitive to perceptual manipulations, whereas in supra-threshold (i.e., perceptually easy tasks, including many of those used in the studies in this meta-analysis) RT is sensitive to modulations in both perceptual and post-perceptual processes 92 , 93 . Indeed, Davranche et al. 73 utilized a drift diffusion model to determine which aspects of decision-making are modulated by HIIT. Importantly, drift rate and decision response boundary size increased significantly after exercise relative to before, while non-decision time decreased. This suggests there was an improvement in perceptual discrimination, the efficiency of non-decisional processes (e.g., motor execution), and the adoption of a more conservative criterion. Future research employing computational models of response time and representational fidelity is needed to develop a comprehensive understanding of the selective influence exercise on information processing speed and accuracy.
Parameter estimates of a model including exercise modality as a moderator suggested that engaging in cycling or HIIT may beneficially impact cognition, especially on attentional and executive processes. Cycling is a commonly used modality in exercise and cognition research. Numerous empirical studies have found that a bout of cycling benefits inhibition, as measured using either the Stroop or Eriksen Flanker task 15 , 94 , 95 , 96 , 97 , 98 , 99 . Improvements in planning 94 , 100 , task-switching 27 , 101 , 102 , and the speed of decision making 103 have also been reported. In contrast to the ubiquity of cycling, the use of HIIT workouts in exercise and cognition research is a relatively recent practice, hence the small number of effect sizes from studies using this modality compared to other types of exercise. The number of effect sizes is important because low-level parameters in a hierarchical model are influenced both by the subset of data directly dependent on the low-level parameter, and by high-level parameter estimates that rely on all of the data. This makes low-level parameter estimates indirectly dependent on the entire dataset, and causes shrinkage in estimates at all levels of the model. In other words, the estimated relationship between HIIT and behavioral performance is derived directly from the few representative effect sizes and indirectly from the rest of the data. The observed positive effect of HIIT on cognition corroborates previous findings. For example, Alves et al. 3 observed that the time to complete a Stroop Task decreased after ten 1-minute bouts of exercising at 80% heart rate reserve relative to a control condition. Improvements in time-dependent measures on interference tasks (i.e., Stroop and flanker) have been correlated with an increase in left dorsolateral prefrontal cortex activity, as measured with functional near infrared spectroscopy (fNIRS), and a decrease in P3 latency measured with EEG 104 . Furthermore, enhancements have also been shown to coincide with an increase in peripheral levels of neural growth factors and lactate 105 . Lastly, a recent meta-analysis on elite athletes observed that HIIT team-based sports had a positive impact on cognitive task performance 25 . Interestingly, because of the small number of published studies in the literature, it is currently unclear if the type of exercise modality used for HIIT workouts (e.g., cycling, sprinting, resistance) differentially impacts cognition.
Behavioral task performance was found to be improved by engaging in vigorous intensity exercise. These results are surprising, considering that exercise intensity is believed to have an inverted-U relationship with performance; where moderate intensity exercise elicits the greatest enhancements while more intense, fatiguing exercise imposes decrements 18 , 62 , 63 , 71 , 106 , 107 . This effect could be driven by HIIT workouts, but may also depend on multiple cognitive task and exercise protocol characteristics. For instance, Chang et al. 18 observed that exercise intensity was only a significant moderator when cognition was tested post-exercise. Similarly, Oberste et al. 23 found that exercise intensity influenced time-dependent measures of interference control but not accuracy. When considering these results, one must also consider that both aforementioned meta-analyses included studies whose subjects were children, adolescents, and older adults. In contrast, the current study was limited to young adults, and there is evidence that the effect of exercise on cognition is comparatively smaller in this age group 18 , 23 . Thus, a model containing an interaction between cognitive domain, task outcome measure, and age groups across the lifespan may be required to observe evidence for an effect of intensity. In addition, there was evidence for the enhancing effects of exercise post-cessation, corroborating previous research 1 , 18 , 94 . Interestingly, in the current meta-analysis cognition was not found to be impacted during exercise. Prior meta-analytic findings on cognition during exercise are mixed, with some reporting that it is exacerbated 20 , while others that find evidence for an enhancement 18 .
Given that the majority of the effect sizes were from tasks that probed executive function, a separate meta-analysis was conducted on this subset of data. This analysis revealed that exercise has a small positive impact on RT measures of executive processes. When looking at model parameters, there was evidence in favor of exercise-enhancing inhibition and working memory. Behavioral research has shown that both the accuracy 9 and speed of working memory 108 , 109 are facilitated by an instance of physical activity. What remains to be determined is the neural mechanisms that engender these behavioral effects. Kao et al. 108 observed that a reduction in RT on the Sternberg task post-HIIT corresponded to an increase in frontal alpha desynchronization during encoding, maintenance, and retrieval periods when working memory load is high. Neuroimaging studies have also found evidence for changes in the activation levels of frontal areas 110 and their connectivity with the intraparietal sulcus post-exercise 111 . These changes in neural activity were not accompanied by a change in behavior, suggesting that more research is needed to demystify the neuromodulatory effect of acute exercise on working memory.
Engaging in repeated bouts of acute exercise over a long period of time can have lasting changes on baseline neurochemical levels, cortical volume, and structural/functional connectivity, which can alter cognitive task performance 1 , 112 , 113 , 114 , 115 . Research investigating the influence of these long-term interventions on cognition has primarily focused on children or older adults. Systematic reviews and meta-analyses suggest that exercise has a small to moderate beneficial impact on general task performance for both of these age groups, with the largest effect sizes observed for measures of executive function, attention, and academic performance 35 . Despite the relative paucity of meta-analyses on how exercise interventions impact cognition in healthy young adults, recent work suggests that it may have a similar beneficial effect. Indeed, a recent meta-analysis, conducted by Ludyga et al. 116 , indicated that long-term exercise interventions have a small positive influence on general cognition regardless of age. The magnitude of this effect was dependent on the interaction between intervention length and exercise duration, with longer interventions and sessions producing greater benefits. Integrating these findings with the current meta-analysis, there is support for the notion that the beneficial impact of long-term interventions on cognition may be a product of repeated exposure to acute exercise induced effects.
There are a number of possible explanations as to why exercise induced effects are small. One possibility is that cognitive function is at its peak during young adulthood, leaving little room for improvements in task performance. Indeed, previous reviews and meta-analyses have observed that the effect of exercise is moderated by age 35 , with the greatest benefits observed for preadolescent children and older adults 18 , 23 , 26 . Contrary to this account, though, the largest exercise induced effects were observed for executive processes, which are believed to be at peak efficiency during this period in the lifespan 117 , 118 . Furthermore, there was moderate evidence that the impact of exercise increased as the average age of sampled young adults also increased. Another explanation may be that cognition is resilient to slight or modest perturbations in overall global state. For example, Bullock et al. 119 demonstrated there was no change in accuracy or RT on a target detection task during experimentally induced hypoxia, hypercapnia, hypocapnia, and normoxia. Meta-analytic modeling of the influence of acute stress on executive function revealed that stress has a small negative impact on working memory and cognitive flexibility, but no impact on inhibition 120 . This suggests that cognition is able to selectively adapt to changes in physiological state caused by various types of stressors, including exercise. A final more intriguing and functional explanation for exercise having a small impact on cognition is that experimental protocols do not typically require the engagement of the body to execute the cognitive task, but rather have people engage in a cognitive task while exercising (or shortly thereafter). This experimental design contrasts real-world tasks that require engagement of the body in the service of the cognitive task. When components of the exercise are incorporated into task goals, then larger changes in performance may be observed. Empirical research investigating how exercise influences task performance in embodied settings versus classic laboratory settings (see 121 for review) is necessary to test the plausibility of this explanation. In addition, the notion that the integrated action of the body and the mind are required to produce the largest effects of exercise on cognition is consistent with a recent evolutionary account of the link between cognition and exercise 122 .
The discrepancy in moderator results between the current meta-analysis and previous meta-analyses could be due to differences in the statistical approach. Frequentist methods typically conduct an omnibus test to determine if levels of a moderator are significantly different from one another and as a measure of a model’s goodness of fit. In contrast, the Bayesian approach determines how likely the observed effect sizes are under a model that includes a moderator and if predictive power is increased. There are a few key advantages to using the Bayesian approach compared to classical frequentist methods. First, it models the uncertainty involved in estimates of between- and within-study heterogeneity and returns a full posterior distribution for both parameters 123 . With these posterior distributions, one can simulate possible pooled effect sizes across credible levels of heterogeneity and develop an informed hypothesis for a subsequent meta-analysis. Similarly, the posterior distributions of effect size estimates can be used as well-informed prior distributions for new data. Importantly, this facilitates the updating of meta-analyses as new research is published. It should be mentioned that the degree of between-study heterogeneity was numerically similar to previous meta-analyses 18 , 22 , implying that they did not suffer from an issue of underestimation by assuming heterogeneity to be a fixed quantity. Second, the Bayesian approach permits the inclusion of prior knowledge. Across all tested priors, there was evidence in favor of a pooled effect derived from averaging the reported estimates of previous meta-analyses. When comparing a prior distribution based on this knowledge to a null effect prior, the former was found to be more probable. Lastly, the posterior distribution of parameter estimates can be used to ascertain the likelihood that one will observe an effect size of a given magnitude for an exercise protocol and cognitive task combination. For example, a researcher could compute the probability that the influence of a bout of cycling on cognitive control will fall within the range of large effect sizes, even if that range does not encompass the maximum a posteriori probability estimate. In contrast, the frequentist approach only produces the maximum likelihood estimate and an interval around it based on fictitious repeats of the meta-analysis. Therefore, the Bayesian approach provides more information for designing future exercise and cognition studies.
Limitations
A potential limitation in the current meta-analysis is the categorization of exercise type using the activity reported in each study. An alternative approach is to categorize exercise based on the theoretical and physiological distinctions between aerobic and anaerobic exercise. We did not adopt this approach here because many activities used in the literature typically include aerobic and anaerobic components, and basing their classification on what authors reported provides insights into the exercise modalities that have been predominantly used in the literature. Another limitation is the schema used to categorize exercise duration. In the event that a study did not report how long participants engaged in exercise, these effects were classified as “not provided”, rendering them as uninterpretable. Lastly, sensitivity analyses were not conducted for moderator parameter estimates due to the high degree of computational demands. However, considering that the pooled effect size estimate was robust across multiple priors and likelihood functions, it is likely that moderator parameter estimates are also consistent.
Conclusions
In summary, the current meta-analytic examination has shown that there is moderate evidence for an acute bout of aerobic exercise inducing a small enhancement in overall performance on cognitive tasks, especially on those that probe executive function and measure response time. Incorporating computational models of decision-making processes, such as drift-diffusion or signal detection models, into exercise research may provide useful insights into the nature of speeded executive processes. Furthermore, testing performance in a real-world setting where individuals typically engage in physical activity may amplify exercise-induced effects.
Data availability
Data have been made publicly available at https://github.com/jggarrett23/PACMAn .
Code availability
Code has been made publicly available at https://github.com/jggarrett23/PACMAn .
Basso, J. C. & Suzuki, W. A. The effects of acute exercise on mood, cognition, neurophysiology, and neurochemical pathways: a review. Brain Plast. 2 , 127–152 (2017).
Article PubMed PubMed Central Google Scholar
Hillman, C. H., Erickson, K. I. & Kramer, A. F. Be smart, exercise your heart: exercise effects on brain and cognition. Nat. Rev. Neurosci. 9 , 58–65 (2008).
Article PubMed Google Scholar
Alves, C. R. R. et al. Influence of acute high-intensity aerobic interval exercise bout on selective attention and short-term memory tasks. Percept. Mot. Skills 118 , 63–72 (2014).
Chang, Y. K., Pesce, C., Chiang, Y. T., Kuo, C. Y. & Fong, D. Y. Antecedent acute cycling exercise affects attention control: an ERP study using attention network test. Front. Hum. Neurosci. 9 , 156 (2015).
Davranche, K. & Audiffren, M. Facilitating effects of exercise on information processing. J. Sports Sci. 22 , 419–428 (2004).
Lambourne, K., Audiffren, M. & Tomporowski, P. D. Effects of acute exercise on sensory and executive processing tasks. Med. Sci. Sports Exerc 42 , 0–00 (2010).
Article Google Scholar
Chen, A. G., Zhu, L. N., Yan, J. & Yin, H. C. Neural basis of working memory enhancement after acute aerobic exercise: FMRI study of preadolescent children. Front. Psychol. 7 , 1804 (2016).
Kao, S. C., Wang, C. H. & Hillman, C. H. Acute effects of aerobic exercise on response variability and neuroelectric indices during a serial n-back task. Brain Cogn. 138 , 105508 (2020).
Quelhas Martins, A., Kavussanu, M., Willoughby, A. & Ring, C. Moderate intensity exercise facilitates working memory. Psychol. Sport Exerc. 14 , 323–328 (2013).
Roig, M., Nordbrandt, S., Geertsen, S. S. & Nielsen, J. B. The effects of cardiovascular exercise on human memory: a review with meta-analysis. Neurosci. Biobehav. Rev. 37 , 1645–1666 (2013).
Schaefer, S., Lövdén, M., Wieckhorst, B. & Lindenberger, U. Cognitive performance is improved while walking: differences in cognitive–sensorimotor couplings between children and young adults. Eur. J. Dev. Psychol. 7 , 371–389 (2010).
Kamijo, K. et al. Differential influences of exercise intensity on information processing in the central nervous system. Eur. J. Appl. Physiol. 92 , 305–311 (2004).
Kamijo, K. & Takeda, Y. General physical activity levels influence positive and negative priming effects in young adults. Clin. Neurophysiol. 120 , 511–519 (2009).
Hillman, C. H. et al. The effect of acute treadmill walking on cognitive control and academic achievement in preadolescent children. Neuroscience 159 , 1044–1054 (2009).
Kamijo, K., Nishihira, Y., Higashiura, T. & Kuroiwa, K. The interactive effect of exercise intensity and task difficulty on human cognitive processing. Int. J. Psychophysiol. 65 , 114–121 (2007).
Komiyama, T. et al. Cognitive function at rest and during exercise following breakfast omission. Physiol. Behav. 157 , 178–184 (2016).
Cantelon, J. A. & Giles, G. E. A review of cognitive changes during acute aerobic exercise. Front. Psychol. 12 , 653158 (2021).
Chang, Y. K., Labban, J. D., Gapin, J. I. & Etnier, J. L. The effects of acute exercise on cognitive performance: a meta-analysis. Brain Res. 1453 , 87–101 (2012).
Haverkamp, B. F. et al. Effects of physical activity interventions on cognitive outcomes and academic performance in adolescents and young adults: a meta-analysis. J. Sports Sci. 38 , 2637–2660 (2020).
Lambourne, K. & Tomporowski, P. The effect of exercise-induced arousal on cognitive task performance: a meta-regression analysis. Brain Res. 1341 , 12–24 (2010).
McMorris, T., Sproule, J., Turner, A. & Hale, B. J. Acute, intermediate intensity exercise, and speed and accuracy in working memory tasks: a meta-analytical comparison of effects. Physiol. Behav. 102 , 421–428 (2011).
Moreau, D. & Chou, E. The acute effect of high-intensity exercise on executive function: a meta-analysis. Perspect. Psychol. Sci. 14 , 734–764 (2019).
Oberste, M. et al. Effects and moderators of acute aerobic exercise on subsequent interference control: a systematic review and meta-analysis. Front. Psychol. 10 , 2616 (2019).
Ishihara, T., Drollette, E. S., Ludyga, S., Hillman, C. H. & Kamijo, K. The effects of acute aerobic exercise on executive function: a systematic review and meta-analysis of individual participant data. Neurosci. Biobehav. Rev. 128 , 258–269 (2021).
Logan, N. E., Henry, D. A., Hillman, C. H. & Kramer, A. F. Trained athletes and cognitive function: a systematic review and meta-analysis. Int. J. Sport Exerc. Psychol. 21 , 725–749 (2023).
Ludyga, S., Gerber, M., Brand, S., Holsboer-Trachsler, E. & Pühse, U. Acute effects of moderate aerobic exercise on specific aspects of executive function in different age and fitness groups: a meta-analysis. Psychophysiology 53 , 1611–1626 (2016).
Oberste, M., Sharma, S., Bloch, W. & Zimmer, P. Acute exercise-induced set shifting benefits in healthy adults and its moderators: a systematic review and meta-analysis. Front. Psychol. 12 , 2 (2021).
Borenstein, M., Hedges, L. V., Higgins, J. P. T. & Rothstein, H. R. A basic introduction to fixed-effect and random-effects models for meta-analysis. Res. Synth. Methods 1 , 97–111 (2010).
Hackenberger, B. K. Bayesian meta-analysis now – let’s do it. Croat. Med. J. 61 , 564 (2020).
Sutton, A. J. et al. Methods for meta-analysis in medical research methods for meta-analysis in medical research contents preface acknowledgements part a: meta-analysis methodology: the basics. https://www.researchgate.net/publication/236905532 (2000).
Sutton, A. J. & Abrams, K. R. Bayesian methods in meta-analysis and evidence synthesis. Stat. Methods Med. Res. 10 , 277–303 (2001).
Chung, Y., Rabe-Hesketh, S. & Choi, I. H. Avoiding zero between-study variance estimates in random-effects meta-analysis. Stat. Med. 32 , 4071–4089 (2013).
Kontopantelis, E., Springate, D. A. & Reeves, D. A re-analysis of the Cochrane Library data: the dangers of unobserved heterogeneity in meta-analyses. PloS one 8 , e69930 (2013).
Sidik, K. & Jonkman, J. N. A comparison of heterogeneity variance estimators in combining results of studies. Stat. Med. 26 , 1964–1981 (2007).
Erickson, K. I. et al. Physical activity, cognition, and brain outcomes: a review of the 2018 physical activity guidelines. Med Sci. Sports Exerc. 51 , 1242–1251 (2019).
Stillman, C. M., Esteban-Cornejo, I., Brown, B., Bender, C. M. & Erickson, K. I. Effects of exercise on brain and cognition across age groups and health states. Trends Neurosci. 43 , 533–543 (2020).
Thompson, S. G. & Sharp, S. J. Explaining heterogeneity in meta-analysis: a comparison of methods. Stat. Med. 18 , 2693–2708 (1999).
Williams, D. R., Rast, P. & Bürkner, P.-C. Bayesian meta-analysis with weakly informative prior distributions. https://doi.org/10.31234/OSF.IO/7TBRM (2018).
Kruschke, J. K. & Liddell, T. M. The Bayesian new statistics: hypothesis testing, estimation, meta-analysis, and power analysis from a bayesian perspective. Psychon. Bull. Rev. 25 , 178–206 (2018).
Kruschke, J. K. Doing Bayesian data analysis: a tutorial with R, JAGS, and Stan, 2nd Edn. https://doi.org/10.1016/B978-0-12-405888-0.09999-2 (Elsevier Science, 2014).
Moher, D. et al. Preferred reporting items for systematic review and meta-analysis protocols (PRISMA-P) 2015 statement. Syst. Rev. 20 , 148–160 (2015).
Google Scholar
Sachdev, P. S. et al. Classifying neurocognitive disorders: the DSM-5 approach. Nat. Rev. Neurol. 10 , 634–642 (2014).
Garber, C. E. et al. American College of Sports Medicine position stand. Quantity and quality of exercise for developing and maintaining cardiorespiratory. Med. Sci. Sports Exerc. 43 , 1334–1359 (2011).
Hedges, L. V. & Olkin, I. Statistical methods for meta-analysis. https://doi.org/10.1016/c2009-0-03396-0 (Elsevier, 1985).
Borenstein, M., Hedges, L. V., Higgins, J. P. T. & Rothstein, H. Introduction to meta-analysis. (Wiley, 2021).
Higgins, J. P. T., Thompson, S. G. & Spiegelhalter, D. J. A re-evaluation of random-effects meta-analysis. J. R. Stat. Soc. Ser. A, (Stat. Soc.) 172 , 137 (2009).
Röver, C. Bayesian random-effects meta-analysis using the Bayesmeta R package. J. Stat. Softw. 93 , 1–51 (2020).
Bürkner, P. C. Advanced Bayesian multilevel modeling with the R package brms. R. J. 10 , 395–411 (2018).
Makowski, D., Ben-Shachar, M. & Lüdecke, D. bayestestR: describing effects and their uncertainty, existence and significance within the bayesian framework. J. Open Source Softw. 4 , 1541 (2019).
Hinne, M., Gronau, Q. F., van den Bergh, D. & Wagenmakers, E.-J. A conceptual introduction to bayesian model averaging. Adv. Methods Pract. Psychol. Sci. 3 , 200–215 (2020).
Schad, D. J., Betancourt, M. & Vasishth, S. Toward a principled Bayesian workflow in cognitive science. Psychol. Methods 26 , 103–126 (2021).
Jeffreys, H. Theory of probability. 3rd Ed., Clarendon Press, Oxford. (Oxford University Press, 1961).
Dienes, Z. How Bayes factors change scientific practice. J. Math. Psychol. https://doi.org/10.1016/j.jmp.2015.10.003 (2016).
Kass, R. E. & Raftery, A. E. Bayes factors. J. Am. Stat. Assoc. https://doi.org/10.1080/01621459.1995.10476572 (1995).
Wetzels, R. et al. Statistical evidence in experimental psychology: an empirical comparison using 855 t tests. Perspect. Psychol. Sci. 6 , 291–298 (2011)
Rouder, J. N., Morey, R. D., Speckman, P. L. & Province, J. M. Default Bayes factors for ANOVA designs. J. Math. Psychol. 56 , 356–374 (2012).
Goldstein, M. Subjective bayesian analysis: principles and practice. Bayesian Anal . 1 , 403–420 (2006).
Depaoli, S., Winter, S. D. & Visser, M. The importance of prior sensitivity analysis in bayesian statistics: demonstrations using an interactive shiny app. Front. Psychol. 11 , 3271 (2020).
Lambert, P. C., Sutton, A. J., Burton, P. R., Abrams, K. R. & Jones, D. R. How vague is vague? A simulation study of the impact of the use of vague prior distributions in MCMC using WinBUGS. Stat. Med. 24 , 2401–2428 (2005).
McElreath, R. Statistical rethinking: a Bayesian course with examples in R and Stan. https://doi.org/10.1201/9781315372495 (2018).
Duval, S. & Tweedie, R. Trim and fill: a simple funnel-plot-based method of testing and adjusting for publication bias in meta-analysis. Biometrics 56 , 455–463 (2000).
Etnier, J. et al. The influence of physical fitness and exercise upon cognitive functioning: a meta-analysis. J. Sport Exerc. Psychol. 19 , 249–277 (1997).
McMorris, T. & Hale, B. J. Differential effects of differing intensities of acute exercise on speed and accuracy of cognition: a meta-analytical investigation. Brain Cogn. 80 , 338–351 (2012).
Neva, J. L. et al. Acute exercise modulates the excitability of specific interneurons in human motor cortex. Neuroscience 475 , 103–116 (2021).
Lulic, T., El-Sayes, J., Fassett, H. J. & Nelson, A. J. Physical activity levels determine exercise-induced changes in brain excitability. PLoS One 12 , e0173672 (2017).
Neva, J. L., Brown, K. E., Mang, C. S., Francisco, B. A. & Boyd, L. A. An acute bout of exercise modulates both intracortical and interhemispheric excitability. Eur. J. Neurosci. 45 , 1343–1355 (2017).
Singh, A. M., Duncan, R. E., Neva, J. L. & Staines, W. R. Aerobic exercise modulates intracortical inhibition and facilitation in a nonexercised upper limb muscle. BMC Sports Sci. Med. Rehabil. 6 , 23 (2014).
Yamazaki, Y. et al. Acute low-intensity aerobic exercise modulates intracortical inhibitory and excitatory circuits in an exercised and a non-exercised muscle in the primary motor cortex. Front. Physiol. 10 , 1361 (2019).
Mooney, R. A. et al. Acute aerobic exercise modulates primary motor cortex inhibition. Exp. Brain Res. 234 , 3669–3676 (2016).
Smith, A. E., Goldsworthy, M. R., Garside, T., Wood, F. M. & Ridding, M. C. The influence of a single bout of aerobic exercise on short-interval intracortical excitability. Exp. Brain Res. 232 , 1875–1882 (2014).
McMorris, T., Tomporowski, P. D. & Audiffren, M. Exercise and cognitive function. Wiley Blackwell , 1–377 https://doi.org/10.1002/9780470740668 (2009)
McMorris, T. & Hale, B. J. Is there an acute exercise-induced physiological/biochemical threshold which triggers increased speed of cognitive functioning? A meta-analytic investigation. J. Sport Health Sci. 4 , 4–13 (2015).
Davranche K., Giraud D., Hays A., Gajdos T. Exploring Decision-making Processes: The Impact of Acute High-Intensity Aerobic Exercise. bioRxiv; https://doi.org/10.1101/2023.02.14.528466 (2023).
Tomporowski, P. D. Effects of acute bouts of exercise on cognition. Acta Psychol. 112 , 297–324 (2003).
Ayaz, A., Saleem, A. B., Schölvinck, M. L. & Carandini, M. Locomotion controls spatial integration in mouse visual cortex. Curr. Biol. 23 , 890–894 (2013).
Fu, Y. et al. A cortical circuit for gain control by behavioral state. Cell 156 , 1139–1152 (2014).
Kaneko, M., Fu, Y. & Stryker, M. P. Locomotion induces stimulus-specific response enhancement in adult visual cortex. J. Neurosci. 37 , 3532–3543 (2017).
Keller, G. B., Bonhoeffer, T. & Hübener, M. Sensorimotor mismatch signals in primary visual cortex of the behaving mouse. Neuron https://doi.org/10.1016/j.neuron.2012.03.040 (2012)
Maimon, G., Straw, A. D. & Dickinson, M. H. Active flight increases the gain of visual motion processing in Drosophila. Nat. Neurosci . 13 , 393–399 (2010).
Niell, C. M. & Stryker, M. P. Modulation of visual responses by behavioral state in mouse visual cortex. Neuron https://doi.org/10.1016/j.neuron.2010.01.033 (2010)
Polack, P.-O., Friedman, J. & Golshani, P. Cellular mechanisms of brain state-dependent gain modulation in visual cortex. Nat. Neurosci. 16 , 1331–1339 (2013).
Bullock, T., Cecotti, H. & Giesbrecht, B. Multiple stages of information processing are modulated during acute bouts of exercise. Neuroscience 307 , 138–150 (2015).
Bullock, T., Elliott, J. C., Serences, J. T. & Giesbrecht, B. Acute exercise modulates feature-selective responses in human cortex. J. Cogn. Neurosci. 29 , 605–618 (2017).
Cao, L. & Händel, B. Walking enhances peripheral visual processing in humans. PLOS Biol. 17 , e3000511 (2019).
Brouwer, G. J. & Heeger, D. J. Decoding and reconstructing color from responses in human visual cortex. J. Neurosci. 29 , 13992–14003 (2009).
Bullock, T. et al. Eye movements disrupt EEG alpha-band coding of behaviorally relevant and irrelevant spatial locations held in working memory. J. Neurophysiol. 129 , 1191–1211 (2023).
Foster, J. J., Sutterer, D. W., Serences, J. T., Vogel, E. K. & Awh, E. The topography of alpha-band activity tracks the content of spatial working memory. J. Neurophysiol. 115 , 168–177 (2016).
MacLean, M. H., Bullock, T. & Giesbrecht, B. Dual process coding of recalled locations in human oscillatory brain activity. J. Neurosci. 39 , 6737–6750 (2019).
Samaha, J., Sprague, T. C. & Postle, B. R. Decoding and reconstructing the focus of spatial attention from the topography of alpha-band oscillations. J. Cogn. Neurosci. 28 , 1090–1097 (2016).
Sprague, T. C., Saproo, S. & Serences, J. T. Visual attention mitigates information loss in small- and large-scale neural codes. Trends Cogn. Sci. 19 , 215–226 (2015).
Garrett, J., Bullock, T. & Giesbrecht, B. Tracking the contents of spatial working memory during an acute bout of aerobic exercise. J. Cogn. Neurosci. 33 , 1271–1286 (2021).
Mordkoff, J. T. & Egeth, H. E. Response time and accuracy revisited: converging support for the interactive race model. J. Exp. Psychol. Hum. Percept. Perform. 19 , 981–991 (1993).
Santee, J. L. & Egeth, H. E. Do reaction time and accuracy measure the same aspects of letter recognition? J. Exp. Psychol. Hum. Percept. Perform. 8 , 489–501 (1982).
Basso, J. C., Shang, A., Elman, M., Karmouta, R. & Suzuki, W. A. Acute exercise improves prefrontal cortex but not hippocampal function in healthy adults. J. Int. Neuropsychol. Soc. 21 , 791–801 (2015).
Chang, Y. K. et al. Effect of acute aerobic exercise on cognitive performance: role of cardiovascular fitness. Psychol. Sport Exerc. 15 , 464–470 (2014).
Davranche, K., Hall, B. & McMorris, T. Effect of acute exercise on cognitive control required during an Eriksen Flanker task. J. Sport Exerc. Psychol. 31 , 628–639 (2009).
Faulkner, J., Lambrick, D., Kaufmann, S. & Stoner, L. Effects of upright and recumbent cycling on executive function and prefrontal cortex oxygenation in young healthy men. J. Phys. Act. health 13 , 882–887 (2016).
Kunzler, M. R. & Carpes, F. P. Intense cycling exercise improves acute cognitive responses. Int. J. Sports Med. 41 , 879–884 (2020).
Yanagisawa, H. et al. Acute moderate exercise elicits increased dorsolateral prefrontal activation and improves cognitive performance with Stroop test. NeuroImage 50 , 1702–1710 (2010).
Hung, T. M., Tsai, C. L., Chen, F. T., Wang, C. C. & Chang, Y. K. The immediate and sustained effects of acute exercise on planning aspect of executive function. Psychol. Sport Exerc. 14 , 728–736 (2013).
Bae, S. & Masaki, H. Effects of acute aerobic exercise on cognitive flexibility required during task-switching paradigm. Front. Hum. Neurosci. 13 , 1–9 (2019).
Pesce, C. & Audiffren, M. Does acute exercise switch off switch costs? A study with younger and older athletes. J. Sport Exerc. Psychol. 33 , 609–626 (2011).
McMorris, T. Exercise and decision-making in team games. Exercise and cognitive function 179–192 https://doi.org/10.1002/9780470740668.CH9 (2009)
Kao, S. C., Drollette, E. S., Ritondale, J. P., Khan, N. & Hillman, C. H. The acute effects of high-intensity interval training and moderate-intensity continuous exercise on declarative memory and inhibitory control. Psychol. Sport Exerc. 38 , 90–99 (2018).
Kujach, S. et al. Acute sprint interval exercise increases both cognitive functions and peripheral neurotrophic factors in humans: the possible involvement of lactate. Front. Neurosci. 13 , 1455 (2020).
Dietrich, A. & Audiffren, M. The reticular-activating hypofrontality (RAH) model of acute exercise. Neurosci. Biobehav. Rev. 35 , 1305–1325 (2011).
Mehren, A. et al. Intensity-dependent effects of acute exercise on executive function. Neural Plast. 2019 , 1–17 (2019).
Kao, S.-C., Wang, C.-H., Kamijo, K., Khan, N. & Hillman, C. Acute effects of highly intense interval and moderate continuous exercise on the modulation of neural oscillation during working memory. Int. J. Psychophysiol. 160 , 10–17 (2021).
Rattray, B. & Smee, D. J. The effect of high and low exercise intensity periods on a simple memory recognition test. J. Sport Health Sci. 5 , 342 (2016).
Li, L. et al. Acute aerobic exercise increases cortical activity during working memory: a functional MRI study in female college students. PLoS One 9 , e99222 (2014).
Weng, T. B. et al. The acute effects of aerobic exercise on the functional connectivity of human brain networks. Brain Plast. 2 , 171–190 (2017).
Baniqued, P. L. et al. Brain network modularity predicts exercise-related executive function gains in older adults. Front. Aging Neurosci. 9 , 426 (2018).
Firth, J. et al. Effect of aerobic exercise on hippocampal volume in humans: a systematic review and meta-analysis. NeuroImage 166 , 230–238 (2018).
Voss, M. W., Nagamatsu, L. S., Liu-Ambrose, T. & Kramer, A. F. Exercise, brain, and cognition across the life span. J. Appl. Physiol. 111 , 1505–1513 (2011).
Voss, M. W. et al. Neurobiological markers of exercise-related brain plasticity in older adults. Brain Behav. Immun. 28 , 90–99 (2013).
Ludyga, S., Gerber, M., Pühse, U., Looser, V. N. & Kamijo, K. Systematic review and meta-analysis investigating moderators of long-term effects of exercise on cognition in healthy individuals. Nat. Hum. Behav. 4 , 603–612 (2020)
Ferguson, H. J., Brunsdon, V. E. A. & Bradford, E. E. F. The developmental trajectories of executive function from adolescence to old age. Sci. Rep. 11 , 1382 (2021).
Hartshorne, J. K. & Germine, L. T. When does cognitive functioning peak? The asynchronous rise and fall of different cognitive abilities across the lifespan. Psychol. Sci. 26 , 433–443 (2015).
Bullock, T., Giesbrecht, B., Beaudin, A. E., Goodyear, B. G. & Poulin, M. J. Effects of changes in end-tidal PO2 and PCO2 on neural responses during rest and sustained attention. Physiol. Rep. 9 , e15106 (2021).
Shields, G. S., Sazma, M. A. & Yonelinas, A. P. The effects of acute stress on core executive functions: a meta-analysis and comparison with cortisol. Neurosci. Biobehav Rev. 68 , 651–668 (2016).
Gordon, J. et al. The road towards understanding embodied decisions. Neurosci. Biobehav. Rev. 131 , 722–736 (2021).
Raichlen, D. A. & Alexander, G. E. Adaptive capacity: an evolutionary-neuroscience model linking exercise, cognition, and brain health. Trends Neurosci. 40 , 408–421 (2017).
Raudenbush, S. W. & Bryk, A. S. Hierarchical linear models: applications and data analysis methods, 485 (2002).
Hogervorst, E., Riedel, W., Jeukendrup, A. & Jolles, J. Cognitive performance after strenuous physical exercise. Percept. Mot. Skills 83 , 479–488 (1996).
Pesce, C., Capranica, L., Tessitore, A. & Figura, F. Focusing of visual attention under submaximal physical load. Int. J. Sport Exerc. Psychol. 1 , 275–292 (2003).
Pesce, C., Tessitore, A., Casella, R., Pirritano, M. & Capranica, L. Focusing of visual attention at rest and during physical exercise in soccer players. J. Sports Sci. 25 , 1259–1270 (2007).
Vickers, J. N. & Williams, A. M. Performing under pressure: the effects of physiological arousal, cognitive anxiety, and gaze control in biathlon. J. Mot. Behav. 39 , 381–394 (2007).
Lo Bue-Estes, C. et al. Short-term exercise to exhaustion and its effects on cognitive function in young women. Percept. Mot. Skills 107 , 933–945 (2008).
Coles, K. & Tomporowski, P. D. Effects of acute exercise on executive processing, short-term and long-term memory. J. Sports Sci. 26 , 333–344 (2008).
Fontana, F. E., Mazzardo, O., Mokgothu, C., Furtado, O. & Gallagher, J. D. Influence of exercise intensity on the decision-making performance of experienced and inexperienced soccer players. J. Sport Exerc Psychol. 31 , 135–151 (2009).
Luft, C. D. B., Takase, E. & Darby, D. Heart rate variability and cognitive function: effects of physical effort. Biol. Psychol. 82 , 186–191 (2009).
Pontifex, M. B., Hillman, C. H., Fernhall, B., Thompson, K. M. & Valentini, T. A. The effect of acute aerobic and resistance exercise on working memory. Med. Sci. Sports Exerc. 41 , 927–934 (2009).
Srygley, J. M., Mirelman, A., Herman, T., Giladi, N. & Hausdorff, J. M. When does walking alter thinking? Age and task associated findings. Brain Res. 1253 , 92–99 (2009).
Thomson, K., Watt, A. P. & Liukkonen, J. Differences in ball sports athletes speed discrimination skills before and after exercise induced fatigue. J. Sports Sci. Med. 8 , 259–264 (2009).
PubMed PubMed Central Google Scholar
Norling, J. C., Sibthorp, J., Suchy, Y., Hannon, J. C. & Ruddell, E. The benefit of recreational physical activity to restore attentional fatigue: the effects of running intensity level on attention scores. J. Leis. Res. 42 , 135–152 (2010).
Young, W. et al. Acute effect of exercise on kicking accuracy in elite Australian football players. J. Sci. Med. Sport 13 , 85–89 (2010).
Chang, Y.-K. et al. Effects of acute exercise on executive function: a study with a tower of London task. J. Sport Exerc. Psychol. 33 , 847–865 (2011).
Green, A. L. & Helton, W. S. Dual-task performance during a climbing traverse. Exp. Brain Res. 215 , 307–313 (2011).
Ohlinger, C. M., Horn, T. S., Berg, W. P. & Cox, R. H. The effect of active workstation use on measures of cognition, attention, and motor skill. J. Phys. Act. Health 8 , 119–125 (2011).
Hope, L., Lewinski, W., Dixon, J., Blocksidge, D. & Gabbert, F. Witnesses in action: the effect of physical exertion on recall and recognition. Psychol. Sci. 23 , 386–390 (2012).
Lambourne, K. The effects of acute exercise on temporal generalization. Q J. Exp. Psychol. 65 , 526–540 (2012).
Moore, R. D., Romine, M. W., O’connor, P. J. & Tomporowski, P. D. The influence of exercise-induced fatigue on cognitive function. J. Sports Sci. 30 , 841–850 (2012).
Roberts, A. P. J. & Cole, J. C. The effects of exercise and body armor on cognitive function in healthy volunteers. Mil. Med. 178 , 479–486 (2013).
Bullock, T. & Giesbrecht, B. Acute exercise and aerobic fitness influence selective attention during visual search. Front. Psychol. 5 , 1290 (2014).
Byun, K. et al. Positive effect of acute mild exercise on executive function via arousal-related prefrontal activations: an fNIRS study. NeuroImage 98 , 336–345 (2014).
Darling, K. A. & Helton, W. S. Dual-task interference between climbing and a simulated communication task. Exp. Brain Res. 232 , 1367–1377 (2014).
Nibbeling, N., Oudejans, R. R. D., Ubink, E. M. & Daanen, H. A. M. The effects of anxiety and exercise-induced fatigue on shooting accuracy and cognitive performance in infantry soldiers. Ergonomics 57 , 1366–1379 (2014).
Pontifex, M. B., Parks, A. C., Henning, D. A. & Kamijo, K. Single bouts of exercise selectively sustain attentional processes. Psychophysiology 52 , 618–625 (2015).
Schmidt-Kassow, M. et al. Treadmill walking during vocabulary encoding improves verbal long-term memory. Behav. Brain Funct. 10 , 24 (2014).
Tsai, C. L. et al. Executive function and endocrinological responses to acute resistance exercise. Front. Behav. Neurosci. 8 , 1–12 (2014).
Bantoft, C. et al. Effect of standing or walking at a workstation on cognitive function: a randomized counterbalanced trial. Hum. Factors 58 , 140–149 (2016).
Larson, M. J. et al. Slow walking on a treadmill desk does not negatively affect executive abilities: an examination of cognitive control, conflict adaptation, response inhibition, and post-error slowing. Front. Psychol. 6 , 723 (2015).
Osgood, J. M. Acute cardiovascular exercise counteracts the effect of ego-depletion on attention: how ego-depletion increases boredom and compromises directed attention. Int. J. Psychol. Stud. 7 , 85–96 (2015).
Perciavalle, V., Maci, T., Perciavalle, V., Massimino, S. & Coco, M. Working memory and blood lactate levels. Neurol. Sci. 36 , 2129–2136 (2015).
Shia, R. M. et al. Individual differences in biophysiological toughness: sustaining working memory during physical exhaustion. Mil. Med. 180 , 230–236 (2015).
Stevens, D. J., Arciuli, J. & Anderson, D. I. Concurrent movement impairs incidental but not intentional statistical learning. Cogn. Sci. 39 , 1081–1098 (2015).
Weng, T. B., Pierce, G. L., Darling, W. G. & Voss, M. W. Differential effects of acute exercise on distinct aspects of executive function. Med. Sci. Sports Exerc. 47 , 1460–1469 (2015).
Alloway, R. G., Alloway, T. P., Magyari, P. M. & Floyd, S. An exploratory study investigating the effects of barefoot running on working memory. Percept. Mot. Skills 122 , 432–443 (2016).
Ando, S. et al. Effects of strenuous exercise on visual perception are independent of visual resolution. Physiol. Behav. 106 , 117–121 (2012).
Brush, C. J. et al. Dose–response and time course effects of acute resistance exercise on executive function. J. Sport Exerc. Psychol. 38 , 396–408 (2016).
Connell, C. J. W., Thompson, B., Kuhn, G. & Gant, N. Exercise-induced fatigue and caffeine supplementation affect psychomotor performance but not covert visuo-spatial attention. PLoS One 11 , e0165318 (2016)
Hsieh, S.-S., Chang, Y.-K., Hung, T.-M. & Fang, C.-L. The effects of acute resistance exercise on young and older males’ working memory. Psychol. Sport Exerc. 22 , 286–293 (2016).
Hsieh, S.-S. Acute resistance exercise facilitates attention control in adult males without an age-moderating effect. J. Sport Exerc. Psychol. 38 , 247–254 (2016).
Lowe, C. J., Kolev, D. & Hall, P. A. An exploration of exercise-induced cognitive enhancement and transfer effects to dietary self-control. Brain Cogn. 110 , 102–111 (2016).
Torbeyns, T. et al. Cycling on a bike desk positively influences cognitive performance. PloS One https://doi.org/10.1371/journal.pone.0165510 (2016)
Tsukamoto, H. et al. Greater impact of acute high-intensity interval exercise on post-exercise executive function compared to moderate-intensity continuous exercise. Physiol. Behav. 155 , 224–230 (2016).
Zach, S. & Shalom, E. The influence of acute physical activity on working memory. Percept. Mot. Skills 122 , 365–374 (2016).
Chang, Y.-K. et al. Acute exercise has a general facilitative effect on cognitive function: a combined ERP temporal dynamics and BDNF study. Psychophysiology 54 , 289–300 (2017).
Crush, E. A. & Loprinzi, P. D. Dose-response effects of exercise duration and recovery on cognitive functioning. Percept. Mot. Skills 124 , 1164–1193 (2017).
González Fernández, F. T., Etnier, J., Zabala, M. & Sanabria, D. Vigilance performance during acute exercise. Int. J. Sport Psychol. 48 , (2017).
Lindheimer, J. B., OʼConnor, P. J., McCully, K. K. & Dishman, R. K. The effect of light-intensity cycling on mood and working memory in response to a randomized, placebo-controlled design. Psychosom. Med. 79 , 243–253 (2017).
Lowe, C. J., Staines, W. R. & Hall, P. A. Effects of moderate exercise on cortical resilience: a transcranial magnetic stimulation study targeting the dorsolateral prefrontal cortex. Psychosom. Med. 79 , 143–152 (2017).
Luu, K. & Hall, P. A. Examining the acute effects of hatha yoga and mindfulness meditation on executive function and mood. Mindfulness 8 , 873–880 (2017).
Randolph, D. D. & O’Connor, P. J. Stair walking is more energizing than low dose caffeine in sleep deprived young women. Physiol. Behav. 174 , 128–135 (2017).
Slutsky, A. B. et al. The effects of low-intensity cycling on cognitive performance following sleep deprivation. Physiol. Behav. 180 , 25–30 (2017).
Sudo, M. et al. Executive function after exhaustive exercise. Eur. J. Appl Physiol. 117 , 2029–2038 (2017).
Cuttler, C., Connolly, C. P., LaFrance, E. M. & Lowry, T. M. Resist forgetting: effects of aerobic and resistance exercise on prospective and retrospective memory. Sport Exerc. Perform. Psychol. 7 , 205 (2017).
Daikoku, T., Takahashi, Y., Tarumoto, N. & Yasuda, H. Auditory statistical learning during concurrent physical exercise and the tolerance for pitch, tempo, and rhythm changes. Mot. Control 22 , 233–244 (2018).
Elkana, O. et al. Conscientiousness is associated with improvement in visuospatial working memory and mood following acute physical exercise: a randomized controlled trial. Pers. Individ. Differ. 132 , 126–132 (2018).
Fenesi, B., Lucibello, K., Kim, J. A. & Heisz, J. J. Sweat so you don’t forget: exercise breaks during a university lecture increase on-task attention and learning. J. Appl. Res. Mem. Cogn. 7 , 261–269 (2018).
Kendall, B. The effects of acute exercise on postural control, information processing, motor skill acquisition, and executive function. Wayne State University Dissertations (2018).
Legrand, F. D. et al. Brief aerobic exercise immediately enhances visual attentional control and perceptual speed. Testing the mediating role of feelings of energy. Acta Psychol. (Amst.) 191 , 25–31 (2018).
Samani, A. & Heath, M. Executive-related oculomotor control is improved following a 10-min single-bout of aerobic exercise: evidence from the antisaccade task. Neuropsychologia 108 , 73–81 (2018).
Siddiqui, A. & Loprinzi, P. D. Experimental investigation of the time course effects of acute exercise on false episodic memory. J. Clin. Med. 7 , 157 (2018).
Sng, E., Frith, E. & Loprinzi, P. D. Temporal effects of acute walking exercise on learning and memory function. Am. J. Health Promot. 32 , 1518–1525 (2018).
Wade, B. & Loprinzi, P. D. The experimental effects of acute exercise on long-term emotional memory. J. Clin. Med. 7 , 486 (2018).
Yamazaki, Y. et al. Inter-individual differences in working memory improvement after acute mild and moderate aerobic exercise. PLoS One 13 , e0210053 (2018).
Baker, R., Coenen, P., Howie, E., Williamson, A. & Straker, L. The musculoskeletal and cognitive effects of under-desk cycling compared to sitting for office workers. Appl. Ergon. 79 , 76–85 (2019).
Du Rietz, E. et al. Beneficial effects of acute high-intensity exercise on electrophysiological indices of attention processes in young adult men. Behav. Brain Res. 359 , 474–484 (2019).
Engeroff, T., Niederer, D., Vogt, L. & Banzer, W. Intensity and workload related dose-response effects of acute resistance exercise on domain-specific cognitive function and affective response – a four-armed randomized controlled crossover trial. Psychol. Sport Exerc. 43 , 55–63 (2019).
Haynes, J. T., Frith, E., Sng, E. & Loprinzi, P. D. Experimental effects of acute exercise on episodic memory function: considerations for the timing of exercise. Psychol. Rep. 122 , 1744–1754 (2019).
Johnson, L. & Loprinzi, P. D. The effects of acute exercise on episodic memory function among young university students: moderation considerations by biological sex. Health Promot. Perspect. 9 , 99–104 (2019).
McGowan, A. L., Chandler, M. C. & Brascamp, J. W. & Pontifex, M. B. Pupillometric indices of locus-coeruleus activation are not modulated following single bouts of exercise. Int. J. Psychophysiol. 140 , 41–52 (2019).
Schmidt, S. L. et al. Decrease in attentional performance after repeated bouts of high intensity exercise in association-football referees and assistant referees. Front. Psychol. 10 , 2014 (2019).
Stenling, A., Moylan, A., Fulton, E. & Machado, L. Effects of a brief stair-climbing intervention on cognitive performance and mood states in healthy young adults. Front. Psychol. 10 , 2300 (2019).
Wu, C.-H. et al. Effects of acute aerobic and resistance exercise on executive function: an ERP study. J. Sci. Med. Sport 22 , 1367–1372 (2019).
Zhou, F. & Qin, C. Acute moderate-intensity exercise generally enhances attentional resources related to perceptual processing. Front. Psychol. 10 , 2547 (2019).
Aly, M. & Kojima, H. Acute moderate-intensity exercise generally enhances neural resources related to perceptual and cognitive processes: a randomized controlled ERP study. Ment. Health Phys. Act. 19 , 100363 (2020).
Chacko, S. C. et al. A single bout of vigorous-intensity aerobic exercise affects reactive, but not proactive cognitive brain functions. Int. J. Psychophysiol. 147 , 233–243 (2020).
Morris, T. P. et al. Light aerobic exercise modulates executive function and cortical excitability. Eur. J. Neurosci. 51 , 1723–1734 (2020).
Walsh, J. J. et al. Acute aerobic exercise impairs aspects of cognitive function at high altitude. Physiol. Behav. 223 , 112979 (2020).
Kim, H. J., Bae, S., Huh, J. H., Lee, J. W. & Han, D. H. Hemodynamic changes in response to aerobic exercise: near-infrared spectroscopy study. Int. J. Sports Med. 42 , 377–385 (2021).
Klatt, S. & Smeeton, N. J. Attentional and perceptual capabilities are affected by high physical load in a simulated soccer decision-making task. Sport Exerc. Perform. Psychol. 10 , 205–216 (2021).
Kuhne, L. A. et al. The effects of acute cardiovascular exercise on memory and its associations with exercise-induced increases in neurotrophic factors. Front. Aging Neurosci. 13 , 750401 (2021).
Manocchio, F. & Lowe, C. J. Investigating cortical buffering effects of acute moderate intensity exercise: a cTBS study targeting the left dorsolateral prefrontal cortex. Front. Hum. Neurosci. 15 , 645326 (2021).
Miyashiro, S. et al. Activation of the orbitofrontal cortex by both meditation and exercise: a near-infrared spectroscopy study. PLoS One 16 , e0247685 (2021).
Trammell, J. P. & Aguilar, S. C. Natural is not always better: the varied effects of a natural environment and exercise on affect and cognition. Front. Psychol. 11 , 575245 (2020).
Zhu, Y., Sun, F., Chiu, M. M. & Siu, A. Y.-S. Effects of high-intensity interval exercise and moderate-intensity continuous exercise on executive function of healthy young males. Physiol. Behav. 239 , 113505 (2021).
Aguirre-Loaiza, H. et al. Effect of acute physical exercise on inhibitory control in young adults: high-intensity indoor cycling session. Physiol. Behav. 254 , 113902 (2022).
Drollette, E. S. & Meadows, C. C. The effects of acute high-intensity interval exercise on the temporal dynamics of working memory and contralateral delay activity. Psychophysiology 59 , e14112 (2022).
Engeroff, T., Banzer, W. & Niederer, D. The impact of regular activity and exercise intensity on the acute effects of resistance exercise on cognitive function. Scand. J. Med. Sci. Sports 32 , 94–105 (2022).
Frith, E., Miller, S. E. & Loprinzi, P. D. Effects of verbal priming with acute exercise on convergent creativity. Psychol. Rep. 125 , 375–397 (2022).
Kao, S.-C. et al. Acute effects of aerobic exercise on conflict suppression, response inhibition, and processing efficiency underlying inhibitory control processes: an ERP and SFT study. Psychophysiology 59 , e14032 (2022).
LaCount, P. A. et al. Acute effects of physical exercise on cognitive and psychological functioning in college students with attention-deficit/hyperactivity disorder. Ment. Health Phys. Act. 22 , 100443 (2022).
Loprinzi, P. D. & Storm, B. C. Acute exercise on memory: application of the retrieval-induced forgetting paradigm. Psychol. Res. 87 , 1417–1428 (2023).
Shirzad, M. et al. Passive exercise increases cerebral blood flow velocity and supports a postexercise executive function benefit. Psychophysiology 59 , e14132 (2022).
Zheng, K. et al. Changes in working memory performance and cortical activity during acute aerobic exercise in young adults. Front. Behav. Neurosci. 16 , 884490 (2022).
Chueh, T.-Y., Hung, C.-L., Chang, Y.-K., Huang, C.-J. & Hung, T.-M. Effects of cognitive demand during acute exercise on inhibitory control and its electrophysiological indices: a randomized crossover study. Physiol. Behav. 265 , 114148 (2023).
Yamada, Y. et al. Improved interference control after exercise with blood flow restriction and cooling is associated with but not mediated by increased lactate. Physiol. Behav. 270 , 114291 (2023).
Zhang, B., Meng, X., Yu, Y., Han, Y. & Liu, Y. Effects of moderate-to-vigorous acute exercise on conscious perception and visual awareness. J. Mot. Behav. 55 , 262–268 (2023).
Zhang, D., Jin, X., Wang, L. & Jin, Y. Form and color visual perception in green exercise: positive effects on attention, mood, and self-esteem. J. Environ. Psychol. 88 , 102028 (2023).
Download references
Acknowledgements
This work was funded by the U.S. Army Combat Capabilities Development Command Soldier Center Measuring and Advancing Soldier Tactical Readiness and Effectiveness (MASTR-E) program through award W911NF-19F-0018 under US Army Research Office contract W911NF-19-D-0001 for the Institute for Collaborative Biotechnologies. The views expressed in this article are solely those of the authors and do not reflect the official policies or positions of the Department of Army, the Department of Defense, or any other department or agency of the U.S. government. The funders had no role in study design, data collection, and analysis, the decision to publish, or preparation of the manuscript. Thank you to Grace Giles, Ph.D., Julie Cantelon, Ph.D., and Neil Dundon, Ph.D., for providing guidance in conducting analyses and interpreting results. Lastly, thank you to Emily Machniak and Riddhima Chandra for assisting in data collection efforts.
Author information
Authors and affiliations.
Department of Psychological & Brain Sciences, University of California, Santa Barbara, CA, USA
Jordan Garrett, Carly Chak, Tom Bullock & Barry Giesbrecht
Institute for Collaborative Biotechnologies, University of California, Santa Barbara, CA, USA
You can also search for this author in PubMed Google Scholar
Contributions
J.G., C.C., T.B., and B.G., designed the study, created the literature search criterion, and performed initial article screenings. J.G., C.C., and B.G. performed screenings of articles based on their full text. J.G., extracted effect sizes, conducted statistical analyses, and wrote the initial draft of the manuscript. B.G., T.B., and C.C. provided edits to the manuscript.
Corresponding authors
Correspondence to Jordan Garrett or Barry Giesbrecht .
Ethics declarations
Competing interests.
The authors declare no competing interests.
Peer review
Peer review information.
Communications Psychology thanks Sebastian Ludyga and Nicole Logan for their contribution to the peer review of this work. Primary Handling Editors: Daniel Quintana, Antonia Eisenkoeck and Jennifer Bellingtier. A peer review file is available.
Additional information
Publisher’s note Springer Nature remains neutral with regard to jurisdictional claims in published maps and institutional affiliations.
Supplementary information
Peer review file, supplementary material, rights and permissions.
Open Access This article is licensed under a Creative Commons Attribution 4.0 International License, which permits use, sharing, adaptation, distribution and reproduction in any medium or format, as long as you give appropriate credit to the original author(s) and the source, provide a link to the Creative Commons licence, and indicate if changes were made. The images or other third party material in this article are included in the article’s Creative Commons licence, unless indicated otherwise in a credit line to the material. If material is not included in the article’s Creative Commons licence and your intended use is not permitted by statutory regulation or exceeds the permitted use, you will need to obtain permission directly from the copyright holder. To view a copy of this licence, visit http://creativecommons.org/licenses/by/4.0/ .
Reprints and permissions
About this article
Cite this article.
Garrett, J., Chak, C., Bullock, T. et al. A systematic review and Bayesian meta-analysis provide evidence for an effect of acute physical activity on cognition in young adults. Commun Psychol 2 , 82 (2024). https://doi.org/10.1038/s44271-024-00124-2
Download citation
Received : 12 June 2023
Accepted : 31 July 2024
Published : 28 August 2024
DOI : https://doi.org/10.1038/s44271-024-00124-2
Share this article
Anyone you share the following link with will be able to read this content:
Sorry, a shareable link is not currently available for this article.
Provided by the Springer Nature SharedIt content-sharing initiative
Quick links
- Explore articles by subject
- Guide to authors
- Editorial policies
Sign up for the Nature Briefing newsletter — what matters in science, free to your inbox daily.

- Open access
- Published: 08 February 2018
A systematic literature review of reviews on techniques for physical activity measurement in adults: a DEDIPAC study
- Kieran P. Dowd 1 ,
- Robert Szeklicki 2 ,
- Marco Alessandro Minetto 3 ,
- Marie H. Murphy 4 ,
- Angela Polito 5 ,
- Ezio Ghigo 3 ,
- Hidde van der Ploeg 6 , 7 ,
- Ulf Ekelund 8 , 9 ,
- Janusz Maciaszek 2 ,
- Rafal Stemplewski 2 ,
- Maciej Tomczak 2 &
- Alan E. Donnelly ORCID: orcid.org/0000-0002-6874-0991 10
International Journal of Behavioral Nutrition and Physical Activity volume 15 , Article number: 15 ( 2018 ) Cite this article
36k Accesses
225 Citations
90 Altmetric
Metrics details
The links between increased participation in Physical Activity (PA) and improvements in health are well established. As this body of evidence has grown, so too has the search for measures of PA with high levels of methodological effectiveness (i.e. validity, reliability and responsiveness to change). The aim of this “review of reviews” was to provide a comprehensive overview of the methodological effectiveness of currently employed measures of PA, to aid researchers in their selection of an appropriate tool. A total of 63 review articles were included in this review, and the original articles cited by these reviews were included in order to extract detailed information on methodological effectiveness.
Self-report measures of PA have been most frequently examined for methodological effectiveness, with highly variable findings identified across a broad range of behaviours. The evidence-base for the methodological effectiveness of objective monitors, particularly accelerometers/activity monitors, is increasing, with lower levels of variability observed for validity and reliability when compared to subjective measures. Unfortunately, responsiveness to change across all measures and behaviours remains under-researched, with limited information available.
Other criteria beyond methodological effectiveness often influence tool selection, including cost and feasibility. However, researchers must be aware of the methodological effectiveness of any measure selected for use when examining PA. Although no “perfect” tool for the examination of PA in adults exists, it is suggested that researchers aim to incorporate appropriate objective measures, specific to the behaviours of interests, when examining PA in free-living environments.
Physical inactivity is the fourth leading cause of death worldwide [ 1 ]. Despite this, PA levels of adults across developed nations remain low and the promotion of regular participation in PA is a key public health priority [ 2 ]. Population level PA surveillance relies upon having tools to accurately measure activity across all population sub-groups. In addition to surveillance, it is essential that valid, reliable and sensitive measures of PA are available to practitioners, researchers and clinicians in order to examine the effectiveness of interventions and public health initiatives. The accurate measurement of PA in adults has relevance not only for refining our understanding of PA-related disorders [ 3 ], but also for defining the dose-response relationship between the volume, duration, intensity and pattern of PA and the associated health benefits.
A number of methods are available for the assessment of PA [ 4 ]. When selecting a measurement technique, researchers and practitioners need to consider not only feasibility and practicality of the measure, but also the methodological effectiveness, such as the validity, reliability and sensitivity. Validity refers to the degree to which a test measures what it is intended to measure, and is most often investigated by comparing the observed PA variables determined by the proposed measure with another comparable measure [ 5 ]. Criterion validity is when a measure is validated against the ‘gold standard’ measure. Good agreement between the proposed method and the gold standard provides some assurance that the results are an accurate reflection of PA behaviour. Other frequently examined forms of validity are concurrent validity (when two measures that give a result that is supposed to be equal are compared) and construct validity (when two measures that are in the same construct are compared). Reliability refers to the degree to which a test can produce consistent results on different occasions, when there is no evidence of change, while sensitivity is the ability of the test to detect changes over time [ 5 ].
In addition to methodological effectiveness, other factors need to be considered when selecting a method for assessing PA and interpreting the findings derived from these methods. Feasibility often drives the selection of the study measures. Some measures are more feasible than others depending on the setting, number of participants and cost. For example, the use of activity monitors to estimate PA may be less feasible in epidemiological studies where large numbers of individuals are being assessed. Reactivity may mean that the act of measuring PA may change a person’s behaviour: for example, being observed for direct observation [ 6 ] or wearing an activity monitor may cause the participant to alter their habitual PA behaviour [ 7 ]. When using self-report measures, social desirability may result in over-reporting of PA among participants keen to comply with the intervention aims [ 8 ]. These factors require careful consideration when selecting methods for assessing PA.
Although methods for the measurement of PA have been extensively examined, reviews to date have focused on specific categories of methods (i.e. self-report questionnaires [ 9 , 10 , 11 ], specific techniques i.e. Doubly Labelled Water (DLW) [ 12 ], smart phone technology [ 13 ], motion sensors and heart rate monitors (HRM) [ 14 ], pedometers [ 15 ] or a comparison of two or more methods [ 16 ]). Some reviews looked exclusively at specific PA behaviours (e.g. walking) [ 17 ] or focused solely on validity and/or reliability issues [ 18 , 19 , 20 ]. Other reviews have concentrated on methods for assessing PA in population subgroups (e.g. individuals with obesity [ 21 ] or older adults) [ 22 , 23 , 24 , 25 , 26 , 27 , 28 , 29 , 30 ]. Due to the level of variability in how information on measurement properties has been presented, and due to the wide range of different measures examined in existing reviews, it is extraordinarily difficult for researchers to compare and contrast measures of PA in adult populations.
The purpose of this article is to review existing reviews (a review of reviews ) that have examined the methodological effectiveness of measures of PA. To aid in the comparison of measurement properties between different PA measures, original papers referred to within each review article were sourced, and additional analysis of these references was completed to enable better comparison and interpretation of findings. This review of reviews (as it will be referred to for the remainder of this article) is intended to provide a concise summary of PA measurement in adults. This work was completed as a component of the European DEDIPAC (DEterminants of DIet and Physical ACtivity) collaboration.
Literature search and search strategy
A systematic search of the electronic databases PubMed, ISI Web of Science, CINAHL, PsycINFO, SPORTDiscus and EMBASE took place in April 2014. The search strategy was developed by two of the authors from examining existing literature reviews, whereby common terminology utilised by published systematic reviews of specific methodologies or narrative reviews of all methodologies were included [ 4 , 5 , 31 , 32 , 33 , 34 , 35 ]. The developed search strategy was reviewed and agreed on by all members of the review team. The electronic databases were searched for the terms “Physical Activity” AND “Review OR Meta-Analysis” AND “Self-report” OR “Logs” OR “Diaries” OR “Questionnaire” OR “Recall” OR “Objective” OR “Acceleromet * ” OR “Activity Monitor * ” OR “Motion Sensor * ” OR “Pedom * ” OR “Heart Rate Monitor*” or “Direct Observation” AND “Valid * ” OR “Reliab * ” OR “Reproducib * ” OR “Sensitiv * ” OR “Responsiv * ”. The search terms and criteria were tailored for each specific electronic database to ensure consistency of systematic searching. Only articles that were published in peer reviewed journals in the English language and were included in this review.
Eligibility for inclusion
Although DLW is suggested as the gold standard measure of energy expenditure [ 36 ], it has not been included in the search strategy, as its feasibility for use in population surveillance research is limited due to its high cost and participant invasiveness [ 34 ]. Due to similar limitations, indirect calorimetry has also not been included in this search strategy. However, reviews that discuss studies which have examined the validity of PA measures against DLW and indirect calorimetry were included. The term Global Positioning System (GPS) was not included as it was felt that the limitations associated with GPS used alone [ 37 ] deemed it an inappropriate measure of PA for population surveillance in its current form.
Review articles that focused solely on the methodological effectiveness of measures of PA in clinical populations and in children/adolescents were not included in this review. Reviews identified in this study which described the methodological effectiveness of measures of PA in both adult and youths were included, but only the adult data extracted and included.
Article selection
A single reviewer screened all article titles, with only articles that were clearly unrelated to the review of reviews removed at this level. Two independent reviewers examined the article abstracts. Results were collated and reported to a third reviewer, who made the final decision in the case of conflicting results. The full texts of included articles were reviewed by two reviewers using the same protocol for handling conflicting results. Reference lists of identified articles were reviewed to ensure that no relevant articles were overlooked. The collated list of accepted reviews was examined by three leading PA measurement experts, who identified key reviews they felt were not included. The full screening protocol was repeated for all supplementary articles identified (Fig. 1 ).
PRISMA flow diagram for search and inclusion process for identification of review articles
Quality assessment
The methodological quality of the systematic reviews was evaluated using the Assessment of Multiple Systematic Reviews (AMSTAR) quality assessment tool [ 38 ]. No similar quality assessment tool exists for narrative reviews. The AMSTAR protocol was applied to each article by two researchers with any conflicting results resolved by a third reviewer.
Data extraction
Initially, the full text and the reference list of each review article meeting the inclusion criteria was screened by a single reviewer for all references to methodological effectiveness, and each methods paper was sourced, screened and all relevant data extracted. The extracted data included general information about the article, the specific measure of PA examined and the demographic characteristics, including the sample population age, size and gender.
Finally, all relevant information relating to properties of methodological effectiveness (i.e. reliability, validity and sensitivity) was recorded. This included the key methodological details of the study and all relevant statistics used to examine measures of methodological effectiveness.
Data synthesis
Data synthesis was conducted separately for each of the PA measurement methods, including general recommendations of the method and its effectiveness indicators. The results extracted from the methods papers were presented in the following order: Validity data is presented as mean percentage difference (MPD) in modified forest plots. Similar to Prince and colleagues (2008), where possible, the MPD was extracted or calculated from the original articles as (((Comparison Measure – Criterion Measure)/Criterion Measure)*100) [ 16 ]. Data points positioned around the 0 mark suggest high levels of validity compared to the reference measure. Data points positioned to the left of the 0 mark suggest an underestimation of the variable in comparison to the reference measure. Data points positioned to the right of the 0 mark suggest an overestimation of the variable in comparison to the reference measure. The further away from the 0 mark the point is positioned, the greater the under/overestimation. Data points 250% greater than or less than the reference measure were capped at 250%, and are marked with an asterisk. Due to the lack of reporting of variance results, and the use of differing and incompatible measurement units, confidence intervals are not reported.
Study selection
The literature search produced 260 potentially relevant abstracts for screening, of which 58 were included in the review following abstract and full text review. After consultation from three international PA experts, and from bibliography review, a further 5 articles were identified for inclusion, providing a total of 63 articles for data extraction (Fig. 1 ) [ 4 , 5 , 6 , 7 , 9 , 10 , 11 , 13 , 14 , 15 , 16 , 17 , 18 , 19 , 21 , 22 , 23 , 24 , 25 , 26 , 27 , 28 , 29 , 30 , 31 , 32 , 33 , 34 , 35 , 39 , 40 , 41 , 42 , 43 , 44 , 45 , 46 , 47 , 48 , 49 , 50 , 51 , 52 , 53 , 54 , 55 , 56 , 57 , 58 , 59 , 60 , 61 , 62 , 63 , 64 , 65 , 66 , 67 , 68 , 69 , 70 , 71 , 72 ].
For this article, reviews were categorised as either “Narrative Reviews” or “Systematic Reviews”. A systematic review was defined as a review which described a search strategy for identification of relevant literature. Of the 63 articles, 41 were categorised as narrative reviews, while 22 were identified as systematic reviews. Findings of the AMSTAR quality assessment and review are described in Table 1 . The mean AMSTAR score across the 22 articles was 5.4 (out of a possible score of 11), with three articles achieving a score of 3, four articles scoring 4, six articles scoring 5, four articles scoring 6, two articles scoring 7, two articles scoring 8 and one article achieving a score of 9 (Table 1 ). Based on AMSTAR categorisation, three reviews were considered low quality, 16 reviews were of medium quality and three reviews were considered high quality. The predominant measures examined/discussed in the identified review articles were activity monitors (n=44; 70%), self-report measures (n=28; 44%), pedometers (n=23; 37%) and HRM (n=18; 29%). Other measures included combined physiologic and motion sensors, multi-physiologic measures, multiphasic devices and foot pressure sensors. These measures were incorporated under the combined sensors heading.
Self-report measures
Criterion validity : A total of 35 articles examined the criterion validity of self-reported measures by comparison to DLW determined energy expenditure [ 73 , 74 , 75 , 76 , 77 , 78 , 79 , 80 , 81 , 82 , 83 , 84 , 85 , 86 , 87 , 88 , 89 , 90 , 91 , 92 , 93 , 94 , 95 , 96 , 97 , 98 , 99 , 100 , 101 , 102 , 103 , 104 , 105 , 106 , 107 ]. Self-reported measures of PA included 7 day recall questionnaires, past year recall questionnaires, typical week questionnaires and PA logs/diaries were validated against 8-15 days of DLW measurement (Additional file 1 : Table S1).
The mean values for self-reported and criterion determined PA energy expenditure were available for the calculation of MPD in 27 articles [ 73 , 74 , 75 , 76 , 77 , 78 , 79 , 80 , 81 , 82 , 83 , 84 , 85 , 86 , 87 , 91 , 92 , 93 , 95 , 97 , 99 , 100 , 102 , 104 , 105 , 106 , 107 ]. Energy expenditure was calculated from a range of behaviours, including leisure time PA, work based PA and PA frequency. The MPD between self-reported PA energy expenditure (time spent in PA normally converted to energy expenditure using a compendium of PA) is presented in Fig. 2 . The MPDs observed in studies that examined the validity of PA diaries ranged from -12.9% to 20.8%. MPD for self-reported PA energy expenditure recalled from the previous 7 days (or typical week) were larger, ranging from -59.5% to 62.1%. MPDs from self-reported PA energy expenditure for the previous month compared to DLW determined energy expenditure ranged from -13.3% to 11.4%, while the difference between self-reported PA from the previous twelve months and DLW determined energy expenditure ranged from -77.6% to 112.5%.
Forest plot of percentage mean difference between self-reported energy expenditure (TEE, PAEE, PAL) compared to criterion measure of energy expenditure (doubly labelled water)
Concurrent validity: A total of 89 articles reported on concurrent validity of self-reported measures [ 75 , 80 , 83 , 84 , 97 , 102 , 108 , 109 , 110 , 111 , 112 , 113 , 114 , 115 , 116 , 117 , 118 , 119 , 120 , 121 , 122 , 123 , 124 , 125 , 126 , 127 , 128 , 129 , 130 , 131 , 132 , 133 , 134 , 135 , 136 , 137 , 138 , 139 , 140 , 141 , 142 , 143 , 144 , 145 , 146 , 147 , 148 , 149 , 150 , 151 , 152 , 153 , 154 , 155 , 156 , 157 , 158 , 159 , 160 , 161 , 162 , 163 , 164 , 165 , 166 , 167 , 168 , 169 , 170 , 171 , 172 , 173 , 174 , 175 , 176 , 177 , 178 , 179 , 180 , 181 , 182 , 183 , 184 , 185 , 186 , 187 , 188 , 189 , 190 ]. Articles were collated based on the types of referent measures used (Additional file 1 : Table S2). The MPD between self-reported energy expenditure and energy expenditure from PA log/diaries for 12 studies ranged from -67.6% to 23.8% (Additional file 1 : figure S2a) [ 80 , 108 , 110 , 111 , 128 , 145 , 152 , 157 , 159 , 160 , 169 , 175 ]. These findings suggest that self-report underestimates energy expenditure compared to activity logs/diaries. Seven studies compared self-reported time spent in specific activity intensities with PA intensities from logs/diaries (Additional file 1 : figure S2a) [ 109 , 120 , 121 , 146 , 152 , 182 , 187 ]. A wider MPD range (-69.0% to 438.5%) was evident, with the greatest differences occurring for moderate intensity physical activity (MPA) and vigorous intensity physical activity (VPA) [ 109 , 120 , 121 ].
Eight studies compared two different self-reported measures of PA energy expenditure [ 80 , 83 , 97 , 152 , 158 , 162 , 175 , 190 ], and and 6 studies compared two different self-reported measures of time spent in PA [ 112 , 135 , 136 , 146 , 152 , 153 , 158 ] (Additional file 1 : figure S2b). Additional file 1 : figure S2c presents 15 studies that compared self-reported PA energy expenditure with PA energy expenditure from activity monitors [ 80 , 132 , 142 , 143 , 144 , 150 , 159 , 168 , 170 , 172 , 174 , 178 , 183 , 185 , 191 ]. The MPD ranged from -74.7% to 82.8%, with self-reported measures tending to overestimate energy expenditure.
Self-reported time spent in light intensity physical activity (LIPA) (n=6) [ 75 , 119 , 131 , 146 , 179 , 189 ], MPA (n=17) [ 75 , 115 , 119 , 130 , 131 , 133 , 134 , 139 , 140 , 141 , 146 , 147 , 161 , 163 , 176 , 177 , 187 , 189 ] and moderate-to-vigorous intensity physical activity (MVPA) (n=7) [ 115 , 116 , 127 , 145 , 149 , 153 , 179 , 192 ] was validated against activity monitors that mainly employed count-to-activity thresholds to determine PA intensity (Additional file 1 : figure S2d), with the MPD for LIPA ranging from -70.1% to 129.2%, MPA ranging from -78.9% to 1007.6% and MVPA ranging from -34.9% to 217.1%. The MPD for VPA was also validated against activity monitors (Additional file 1 : figure S2e) [ 75 , 115 , 119 , 130 , 131 , 133 , 134 , 140 , 141 , 146 , 147 , 161 , 163 , 177 , 187 , 189 ], with all studies identifying an overestimation of self-reported VPA (Additional file 1 : figure S2e).
The concurrent validity of additional self-reported variables, including total PA [ 163 , 181 , 184 , 193 ], frequency of MVPA [ 149 ], active time [ 151 , 161 ], time standing [ 192 ] and time stepping [ 192 ] were also compared to activity monitor determined variables (Additional file 1 : figure S2e).
The MPD between self reported energy expenditure and both pedometer and HRM determined energy expenditure [ 80 , 102 , 123 , 142 , 194 ]; and self-reported time spent in PA intensities and HRM determined time spent in PA intensities [ 118 , 129 , 146 , 154 , 174 , 195 ] are presented in Additional file 1 : figure S2f. Self-reported energy expenditure overestimated pedometer determined energy expenditure (range=17.1% to 86.5%). Self-reported measures notably overestimated time spent in PA intensities when compared to HRM. Although self-reported energy expenditure underestimated HRM determined energy expenditure, this underestimation was small compared to other measures (-17.7% to -1.3%).
Reliability
Intra-instrument reliability: One article examined the intra-instrument reliability of a self-reported measure of PA [ 196 ]. A self-reported instrument examining the previous 14 days of PA was administered [ 196 ]. After 3 days, the instrument examined the PA of the same 14 day period. The findings identified high levels of intra-instrument reliability for total activity (ICC=0.90; 95% CI=0.86-0.93), MPA (ICC=0.77; 95% CI=0.69-0.84), VPA (ICC=0.90; 95% CI=0.86-0.93), walking (ICC=0.89; 95% CI=0.85-0.93) and energy expenditure (ICC=0.86; 95% CI=0.80-0.90) (Additional file 1 : Table S3).
Test-retest reliability: The test-retest reliability of self-reported measures was examined in 116 studies [ 75 , 77 , 83 , 110 , 116 , 117 , 122 , 125 , 126 , 127 , 129 , 131 , 132 , 135 , 137 , 140 , 144 , 145 , 147 , 149 , 152 , 153 , 155 , 157 , 159 , 161 , 162 , 167 , 168 , 169 , 172 , 175 , 176 , 178 , 179 , 180 , 181 , 184 , 187 , 188 , 190 , 191 , 196 , 197 , 198 , 199 , 200 , 201 , 202 , 203 , 204 , 205 , 206 , 207 , 208 , 209 , 210 , 211 , 212 , 213 , 214 , 215 , 216 , 217 , 218 , 219 , 220 , 221 , 222 , 223 , 224 , 225 , 226 , 227 , 228 , 229 , 230 , 231 , 232 , 233 , 234 , 235 , 236 , 237 , 238 , 239 , 240 , 241 , 242 , 243 , 244 , 245 , 246 , 247 , 248 , 249 , 250 , 251 , 252 , 253 , 254 , 255 , 256 , 257 , 258 , 259 , 260 , 261 , 262 , 263 , 264 , 265 , 266 , 267 , 268 , 269 ]. Due to the wide test-retest periods, articles were allocated to one of 5 periods, ≤1 week (Additional file 1 : Table S4a), >1 - <4 weeks (Additional file 1 : Table S4b), >4 - <8 weeks (Additional file 1 : Table S4c), >8 weeks - <1 year (Additional file 1 : Table S4d) and >1 year (Additional file 1 : Table S4e). Test-retest statistics employed were extracted and are presented in Table 2 . An overview of all identified studies examining the test-retest reliability of PA/energy expenditure measured by self-report, along with all test-retest statistics is provided in Additional file 1 : Table S4a-e.
Sensitivity : Two studies examined the sensitivity of self-reported measures to detect change in PA behaviours over time [ 256 , 270 ]. Both studies identified small to moderate effect sizes for specific PA behaviours over a six month period in older adults (Additional file 1 : Table S5).
Activity monitors
Criterion validity : Fifty-eight articles examined the criterion validity of activity monitor determined PA variables [ 73 , 77 , 80 , 96 , 105 , 119 , 271 , 272 , 273 , 274 , 275 , 276 , 277 , 278 , 279 , 280 , 281 , 282 , 283 , 284 , 285 , 286 , 287 , 288 , 289 , 290 , 291 , 292 , 293 , 294 , 295 , 296 , 297 , 298 , 299 , 300 , 301 , 302 , 303 , 304 , 305 , 306 , 307 , 308 , 309 , 310 , 311 , 312 , 313 , 314 , 315 , 316 , 317 , 318 , 319 , 320 , 321 , 322 , 323 ]. The majority of articles compared activity monitor determined energy expenditure with DLW [ 73 , 77 , 80 , 96 , 105 , 274 , 275 , 277 , 278 , 280 , 281 , 285 , 292 , 293 , 295 , 296 , 300 , 303 , 304 , 305 , 311 , 313 , 317 , 323 ], while activity monitor determined steps [ 119 , 271 , 283 , 287 , 289 , 298 , 299 , 306 , 307 , 314 , 315 , 318 ], distance travelled [ 282 ] and activity type [ 272 , 273 , 276 , 279 , 284 , 286 , 288 , 290 , 291 , 294 , 297 , 301 , 302 , 308 , 309 , 310 , 312 , 316 , 319 , 320 , 321 , 322 ] were also compared to direct observation (Additional file 1 : Table S6).
The range of MPD observed in studies that examined the criterion validity of activity monitor determined energy expenditre ranged from -56.59% to 96.84% (Fig. 3a ). However, a trend was apparent for activity monitor determined energy expenditure to underestimate the criterion measure. The range of MPD between activity monitor and direct observation determined steps was smaller, with values ranging from -48.52% to 7.47%, with 96% of studies having a MPD between -10% to 10% (Fig. 3b ). Activity monitors overestimated distance walked/run (0.88% to 27.5%). Activity monitors also tended to underestimate activity classification, with MPD varying between -36.67% to 2.00%.
a Forest plot of percentage mean difference between accelerometer determined energy expenditure (TEE, PAEE, PAL) compared to criterion measure of energy expenditure (doubly labelled water). b Forest plot of percentage mean difference between accelerometer determined steps, distance walked and activity type compared to criterion measure of direct observation
Concurrent validity: A total of 103 articles examined the concurrent validity of activity monitor measures of PA [ 73 , 77 , 80 , 119 , 146 , 151 , 174 , 192 , 194 , 195 , 262 , 271 , 282 , 295 , 305 , 316 , 324 , 325 , 326 , 327 , 328 , 329 , 330 , 331 , 332 , 333 , 334 , 335 , 336 , 337 , 338 , 339 , 340 , 341 , 342 , 343 , 344 , 345 , 346 , 347 , 348 , 349 , 350 , 351 , 352 , 353 , 354 , 355 , 356 , 357 , 358 , 359 , 360 , 361 , 362 , 363 , 364 , 365 , 366 , 367 , 368 , 369 , 370 , 371 , 372 , 373 , 374 , 375 , 376 , 377 , 378 , 379 , 380 , 381 , 382 , 383 , 384 , 385 , 386 , 387 , 388 , 389 , 390 , 391 , 392 , 393 , 394 , 395 , 396 , 397 , 398 , 399 , 400 , 401 , 402 , 403 , 404 , 405 , 406 , 407 , 408 , 409 ]. Data extractions were grouped by the types of measures used (Additional file 1 : Table S7).
The MPD of activity counts from two different activity monitors ranged from -40.6% to 13.2% [ 262 , 327 , 351 , 389 , 392 , 405 ]. The MPD for a wide range of activity behaviours from two different activity monitors were examined; LIPA (-12.5% - 13.7%) [ 146 , 340 , 392 , 405 ], MPA (-10.9% - 3.1%) [ 146 , 340 ], VPA (-9.7% - 20.3%) [ 146 , 352 ], MVPA (-57.5% - 3.3%) [ 344 , 392 , 405 ], total PA (1.1%) [ 146 ]. Stepping [ 151 , 192 ] and step counts [ 77 , 119 , 340 , 405 ] were compared between 2 activity monitor devices (MPD ranged from -21.7% - 0% for step counts and -57.1% - 56% for stepping). Energy expenditure estimated by two activity monitors were compared [ 372 , 404 , 408 ], with MPD ranging from -21.1% - 61% (Additional file 1 : figure S3c).
Energy expenditure at different PA intensities from activity monitors were compared against estimates from indirect calorimetry and whole room calorimetry. For LIPA, the MPD ranged from -79.8% - 429.1% [ 349 , 394 ]. For MPA, MPD ranged from -50.4% - 454.1% [ 349 , 395 ], while estimates for VPA ranged from -100% - 163.6%. Energy expenditure estimates from activity monitoring devices for total PA were compared against indirect calorimetry estimates [ 368 , 394 , 396 , 398 , 404 ], where MPD ranged from -41.4% to 115.7%. The MPD range for activity monitor determined total energy expenditure compared with whole-room calorimetry were narrower (-16.7% to -15.7%) [ 343 , 364 ] (Additional file 1 : figure S3d).
Activity monitor estimates of energy expendture were compared to HRM estimates of energy expenditure for total PA (-10.4% - 22.2%) [ 80 , 402 ], for LIPA (-75.4% - 72.8%) [ 146 ], for MPA (49.2% - 677.7%), VPA (-46.2% - 46.2%) [ 146 , 361 ] and for total time spent in PA (-16.1% - 34.9%) [ 146 , 174 ]. Self-reported measures were used to examine the concurrent validity of activity monitors for energy expenditure [ 80 ] and total time spent in PA [ 174 ], with MPD ranging from -6.0% - 32.1% (Additional file 1 : figure S3e).
Estimated energy expenditure was compared between activity monitors and indirect calorimetry (kcal over specified durations; Additional file 1 : figure S3f (-68.5% - 81.1%)) [ 282 , 328 , 341 , 358 , 367 , 369 , 370 , 375 , 376 , 380 , 382 , 383 , 385 , 387 ]; (METs over specified durations; Additional file 1 : figure S3g (-67.3% -- 48.4%)) [ 195 , 325 , 345 , 346 , 347 , 349 , 350 , 353 , 357 , 362 , 384 , 397 , 400 , 407 , 409 ]. A single study compared the estimated energy expenditure from 5 different activity monitors and indirect calorimetry at incremental speeds (54, 80, 107, 134, 161, 188 and 214 m.min -1 ) in both men and women (MPD ranged from -60.4% - 90.8%) (Additional file 1 : figure S3h) [ 374 ].
Inter-instrument reliability : The inter-instrument reliability of activity monitoring devices (e.g. the reliability of the same device worn by the same participant over the same time period) was examined in 18 studies [ 301 , 315 , 337 , 344 , 370 , 385 , 387 , 406 , 409 , 410 , 411 , 412 , 413 , 414 , 415 , 416 , 417 , 418 ]. Study methodologies included the wearing of devices over the left and right hip [ 337 , 370 , 385 , 387 , 406 , 413 , 415 , 417 ], over the hip and lower back [ 409 ], the wearing of devices side by side at the same location on the hip [ 301 , 344 , 411 , 414 , 416 , 417 , 418 ], devices worn at 3 rd intercostal space and just below the apex of the sternum [ 410 ], device worn on both wrists [ 412 ], worn on both legs [ 315 ] and worn side by side on the same leg [ 315 ]. Coefficients of variations ranged from 3% to 10.5% for the ActiGraph device [ 418 ] and from <6% to 35% for the RT3 accelerometer [ 387 , 416 ]. All reported correlation coefficients were significant and greater than 0.56 [ 370 , 385 , 387 , 406 , 409 , 412 , 415 , 417 ]. ICC values for the majority of devices were >0.90 [ 301 , 315 , 337 , 344 , 411 , 413 ], excluding those observed for the RT3 accelerometer (0.72-0.95) [ 417 ], Actitrac (0.40 -0.87) and Biotrainer devices (0.60–0.71) [ 406 ] (Additional file 1 : Table S8).
Test-retest reliability: Test-retest reliability of activity monitoring devices was examined in 26 studies [ 153 , 155 , 228 , 234 , 262 , 282 , 297 , 314 , 358 , 385 , 407 , 411 , 414 , 416 , 419 , 420 , 421 , 422 , 423 , 424 , 425 , 426 , 427 , 428 , 430 ]. For the laboratory-based studies, variables examined included distance walked [ 282 ], steps at different speeds [ 314 , 420 ], resting periods [ 358 ], accelerometer counts [ 385 , 407 , 411 , 414 , 416 , 425 , 429 , 430 ], energy expenditure [ 426 ] and postural position [ 297 , 429 ]. For the free-living analyses, behaviours examined included activity behaviours [ 155 , 419 ], accelerometer counts [ 262 , 421 , 422 ], step count [ 422 ], energy expenditure [ 228 , 234 ] and the number of people achieving the recommended amount of PA [ 153 ] (Additional file 1 : Table S9).
As the examination of PA over a number of days can be considered a measure of test-retest reliability, researchers have used statistical processes (i.e. generalizability theory or the Spearman Brown Prophecy formula) to determine the minimum number of days required to provide a reliability estimate of PA behaviours [ 431 ]. Studies reported that a minimum of three days of ActiGraph data are required to provide a reliable estimate of total PA [ 423 ] and time spent in MVPA [ 424 ], while a minimum of 2 days is required to provide a reliable estimate of ActiGraph determined steps per day, accelerometer counts per day and intermittent MVPA per day [ 427 ]. However, for the examination of continuous 10 minute bouts of MVPA (as suggested in the majority of international PA recommendations), a minimum of 6 days of measurement is required [ 427 ].
Sensitivity : The only study of responsiveness to change in activity monitors, using the ActiWatch, identified that this device was able to detect significant differences in activity counts accumulated between young adults and sedentary older adults and between active older adults and sedentary older adults [ 421 ]. However, no differences could be detected between the young adults and active older adults (Additional file 1 : Table S10).
Criterion validity: A total of 30 studies were sourced that examined the criterion validity of step count in pedometer devices [ 283 , 289 , 298 , 306 , 307 , 314 , 318 , 365 , 391 , 432 , 433 , 434 , 435 , 436 , 437 , 438 , 439 , 440 , 441 , 442 , 443 , 444 , 445 , 446 , 447 , 448 , 449 , 450 , 451 , 452 ], while 3 studies examined the criterion validity of pedometer determined energy expenditure compared to DLW [ 93 , 453 , 454 ]. Of the laboratory based studies assessing criterion validity, 30% used over ground walking protocols [ 307 , 318 , 365 , 391 , 442 , 445 , 446 , 447 , 450 , 451 ] and the remaining treadmill-based protocols [ 283 , 289 , 298 , 306 , 314 , 432 , 433 , 434 , 435 , 436 , 437 , 438 , 439 , 440 , 441 , 443 , 444 , 448 , 449 ] or a combination of the two [ 452 ]. In free-living studies which examined the criterion validity of pedometer determined energy expenditure, pedometers were worn for 2 [ 454 ], 7 [ 93 ] and 8 days [ 453 ] (Fig. 4 ; (-62.3% - 0.8%)). Pedometer determined step count was generally lower when compared to direct observation (-58.4% - 6.9%). Some studies also examined the effect of speed on pedometer output. Pedometers had relatively high levels of accuracy across all speeds, but appear to be more accurate at determining step-count at higher walking speeds compared to lower walking speeds (Additional file 1 : Table S11) [ 306 , 436 , 438 , 439 ].
Forest plot of mean percentage difference between pedometer determined step count/energy expenditure compared to criterion measure (direct observation/doubly labelled water respectively). * denotes multiple devices compared in the same study
Concurrent validity: The concurrent validity of pedometers was examined in a total of 22 articles [ 77 , 194 , 298 , 376 , 391 , 399 , 404 , 422 , 432 , 433 , 434 , 441 , 444 , 448 , 449 , 451 , 452 , 455 , 456 , 457 , 458 , 459 ]. Various approaches were used to examine the concurrent validity of pedometers, with 14 studies comparing pedometer step count with steps determined from other pedometers [ 432 , 451 , 458 ] and activity monitors [ 77 , 298 , 391 , 422 , 433 , 434 , 444 , 455 , 456 , 457 , 459 ] and 4 studies comparing pedometer determined energy expenditure with energy expenditure determined from indirect calorimetry [ 376 , 399 , 404 , 441 , 448 , 451 ] and/or energy expenditure determined from other activity monitors [ 451 ]. One study compared pedometer determined distance travelled with treadmill determined distance travelled [ 449 ], while one study compared pedometer determined MVPA with activity monitors determined MVPA [ 452 ] (Additional file 1 : figure S4a). Pedometers appear to underestimate time spent in MVPA and estimated energy expenditure when compared to other measures. The findings are less clear for step count determined from pedometers when compared to other pedometers or activity monitors, with devices appearing to both over and underestimate step count (Additional file 1 : Table S12).
Inter-instrument reliability: A total of 6 articles examined the inter-instrument reliability of pedometer output obtained from 18 different devices [ 314 , 315 , 447 , 449 , 451 , 457 ]. Many included articles examined the inter-instrument reliability of multiple devices in the same study (e.g. 2 pedometers [ 315 ], 5 pedometers [ 451 ], 10 pedometers [ 446 , 449 ]). Inter-instrument reliability was examined by comparing pedometer outputs from two of the same model devices worn on the left and right hip [ 315 , 449 , 451 , 457 ], on the left hip, right hip and middle back [ 447 ] and on the left and right hip and repeated with two further devices of the same model [ 446 ].
Three studies (1 examining the inter-instrument reliability of a single pedometer and 2 examining the inter-instrument reliability of multiple pedometers), identified that the majority of devices had acceptable levels of inter-instrument reliability (ICC ≥ 080) [ 446 , 449 , 457 ]. In the studies which examined the inter-instrument reliability of multiple devices, 8/10 pedometers [ 449 ] and 9/10 pedometers [ 446 ] achieved ICC ≥ 0.80. Using planned contrasts, Bassett and colleagues highlight that no significant differences were observed between devices worn on the left and right hip [ 451 ]. Two studies investigated the effect of walking speed on inter-instrument reliability, highlighting that ICC values increased as speed increased [ 315 , 447 ] (Additional file 1 : Table S13).
Test-retest reliability: A single laboratory-based test-retest reliability study in a laboratory-based treadmill protocol identified that the Yamax Digiwalker SW-200 (Tokyo, Japan) had appropriate test-retest reliability (ICC > 0.80 and significant) at 7 out of 11 treadmill speeds (non-significant speeds = 4, 20, 22 and 26 km.h -1 ) [ 314 ].
A total of 6 articles examined the reliability of pedometer steps obtained over a specified measurement period [ 423 , 427 , 460 , 461 , 462 , 463 ], presenting the minimum number of days of pedometer measurement to reliably estimate PA behaviours. The minimum number of days of measurement required for a reliable estimate (i.e. ICC >0.8) of pedometer steps was 2-4 days (Additional file 1 : Table S14) [ 423 , 427 , 460 , 461 , 462 , 463 ].
Sensitivity : In the only study of pedometer responsiveness to change, effect size was used to examine the meaningfulness of difference between means [ 464 ]. A large effect size (>0.8) was observed, suggesting that pedometers, in this study, were sensitive to change (Additional file 1 : Table S15).
- Heart rate monitors
Criterion validity: All 12 studies that examined the criterion validity of HRMs were unstructured, free-living protocols [ 80 , 85 , 87 , 96 , 99 , 100 , 102 , 123 , 304 , 371 , 465 , 466 ]. The duration of monitoring for HRM ranged from 24 hours [ 102 , 465 ] to 14 days [ 96 , 371 ]. Two studies examined the validity of HRM determined physical activity levels (PAL) compared to DLW determined PAL. All remaining articles compared estimates of energy expenditure determined by HRM techniques with DLW determined energy expenditure. The flex heart rate methodology, which distinguishes between activity intensities based on heart rate versus VO 2 calibration curves, were utilised in all studies using individual calibration curves. MPDs between HRM determined energy expenditure and DLW determined energy expenditure ranged from -60.8% - 19.7% across identified studies (Fig. 5 ). No clear trend for over/under estimation was apparent (MPDs for energy expenditure ranging between -60.8% - 19.7%). For PAL, a slight trend in underestimation was apparent (-11.1 to -7.6) (Additional file 1 : Table S16).
Forest plot of percentage mean difference between heart rate monitor determined energy expenditure/physical activity level compared to criterion measure (doubly labelled water)
Concurrent validity: The concurrent validity of HRM determined energy expenditure [ 80 , 467 , 468 , 469 , 470 ], PAL [ 80 ] and PA intensity [ 146 , 174 ] was examined using a range of measures, including direct/indirect calorimetry [ 467 , 469 , 470 ], activity monitoring [ 80 , 146 , 174 , 401 ] and measures of self-reported PA [ 80 , 174 , 468 ] (Additional file 1 : Table S17). A slight trend in overestimation of energy expenditure and PAL was observed (Additional file 1 : figure S5a). For PA intensities, MPDs were larger and more variable, with MPA underestimated and VPA overestimated. The MPD between HRM determined LIPA and LIPA determined by the Tritrac and MTI activity monitors fell outside the range for the presented forest plot, with values of +306.4% and +367.2%, respectively [ 146 ] (Additional file 1 : figure S5a). No articles sourced through the data extraction reported on the reliability or responsiveness to change of HRM.
Combined sensors
Criterion validity: A total of 8 articles were identified that examined the criterion validity of multiple accelerometers [ 471 , 472 , 473 , 474 ] or accelerometers combined with gyroscopes [ 475 ] or HRMs [ 371 , 476 , 477 ]. The included studies had relatively small sample sizes, ranging from 3-31 participants. Studies primarily examined the effectiveness of data synthesis methodologies (i.e. Decision Tree Classification, Artificial Neural Networks, Support Vector Machine learning etc.) to identify specific postures/activities [ 471 , 472 , 473 , 474 , 475 , 476 , 477 ] or energy expenditure [ 371 , 477 ]. Time spent in specific body postures/activity types tended to be underestimated from combined sensors when compared to direct observation (-33.3% to -3.2%; Fig. 6 ). In contrast, energy expenditure was overestimated by combined sensors when compared to DLW in free-living settings (13.0% to 26.8%) (Additional file 1 : Table S18) [ 371 ].
Forest plot of percentage mean difference between energy expenditure/body posture determined by combined sensors compared to criterion measure (doubly labelled water/direct observation)
Concurrent validity: Eleven studies examined the validity of combined accelerometry and HRM determined energy expenditure compared to whole room calorimetry [ 478 , 479 , 480 ] or indirect calorimetry [ 400 , 477 , 481 , 482 , 483 , 484 , 485 , 486 ] determined energy expenditure. No clear trend for under/overestimation was apparent, with combined sensors appearing to be relatively accurate in estimating energy expenditure when compared to indirect calorimetry in both a structured (-13.8% - 31.1%) and unstructured (0.13%) [ 485 ] settings (Additional file 1 : Table S19). No articles sourced through the data extraction reported on the reliability or responsiveness to change of combined sensors.
To the authors’ knowledge, this is the first systematic literature review of reviews to simultaneously examine the methodological effectiveness of the majority of PA measures. The greatest quantity of information was available for self-reported measures of PA (198 data extraction points), followed by activity monitors (179 data extraction points), pedometers (52 data extraction points), HRMs (19 data extraction points) and combined sensors (18 data extraction points).
The criterion validity of measures was determined through the examination of energy expenditure via DLW and by direct observation of steps and PA behaviours. For accelerometry, although variability was lower, a substantial proportion of studies (44/54) underestimated energy expenditure compared to DLW when proprietary algorithms or count-to-activity thresholds were employed. Based on the amended forest plots for the criterion validity of measures of PA, a greater level of variability was apparent for self-reported measures compared to objective measures (Figs. 2 – 6 ). Limited data on the criterion validity of HRM and combined sensors determined energy expenditure was available. HRMs tended to underestimate DLW determined energy expenditure, while combined sensors often overestimated energy expenditure. Unfortunately, due to the lack of measures of variability, resulting in the absence of meta-analysis, it was not possible to describe the extent of differences between measures statistically. For step counts, both activity monitors and pedometers achieved high levels of criterion validity. When comparing the two, pedometers appeared to be less accurate than activity monitors at estimating step count, tending to underestimate steps when compared to direct observation. Activity monitors tended to slightly overestimate distance travelled, while time spent in each activity type (or posture) determined by both activity monitors and combined sensors was slightly underestimated when compared to direct observation (Fig. 3a and Fig. 6 ). For concurrent validity of all measure of PA, high levels of variability were observed across a wide range of activity behaviours. In particular, high levels of variability were apparent in the estimation of PA intensities, with VPA substantially overestimated in the majority of concurrent validations across all measures. In summary, objective measures are less variable than recall based measures across all behaviours, but high levels of variability across behaviours are still apparent.
For activity monitors and pedometers, acceptable inter-instrument reliability was observed in the majority of studies. Variability for inter-instrument reliability across different activity monitors and pedometers was apparent, with some instruments demonstrating better reliability compared to others. However, a detailed examination of study methodology, device wear locations and activities performed is necessary when interpreting the inter-instrument reliability of pedometers and activity monitors.
A wide range of values were reported for the test-retest reliability of self-reported measures, with apparent trends for reduced levels of test-retest reliability as the duration of recall increased. Researchers must be cognisant of potential differences in test-retest reliability due to duration between administrations and between PA behaviours assessed within each tool when selecting a self-reported measure of PA. Moderate to strong test-retest reliability was observed for activity monitors in free-living environments. However, the reliability of activity monitors attenuated as the duration between measurements increased. As expected, the test-retest reliability of different devices varied, while intensity of activity often had a significant effect. The test-retest reliability of pedometer determined steps in a laboratory setting was high across the majority of speeds, but the reliability appeared to weaken at higher speeds (e.g. 20, 22 and 26 km·h -1 ). Although moderate to strong test-retest reliability of both pedometers and activity monitors were apparent, researchers should be aware of differences between models and devices when selecting a measure for use. Furthermore consideration should be given to the duration between test and retest and the behaviour being assessed when considering test-retest reliability, as although a measure may be reliable for one output, it may not be reliable for all outcomes.
When examining PA in free-living environments, it is essential that sufficient data is gathered to ensure a reliable estimate is obtained [ 7 , 431 ]. By determining the inter- and intra-individual variability across days of measurement, researchers can define the number of days of monitoring required to reliably estimate such behaviours. For activity monitors and pedometers, analysis has been conducted to estimate the minimum number of days of measurement required to provide a reliable estimate of PA behaviors. For activity monitors, two days of measurement are recommended for a reliable estimate of steps per day, accelerometer counts per day and intermittent MVPA per day measured, 3 days for a reliable estimate of total PA and time spent in MVPA and 6 days are required for a reliable estimate of continuous 10 minute bouts of MVPA. For pedometers, a minimum of 2-4 days of measurement was required to provide a reliable estimate of steps in older adults, while 2-5 days of measurement was required in adults. These findings highlight the importance of knowing what behaviours are to be examined prior to collecting objective data from free-living environments, to ensure that sufficient information is recorded to provide reliable estimates of the behaviours of interest.
The responsiveness of measures to detect change over time was the least reported property of measures of PA. When evaluating interventions, or indeed evaluating changes in PA behaviours in longitudinal research, it is critical to utilise measures that can detect such changes. Although validity and reliability are requirements for sensitivity/responsiveness to change [ 5 ], this does not imply that a measure is responsive to change simply because it is valid and reliable. Responsiveness to change must be evaluated, and not assumed. Currently, the research on the responsiveness to change for all types of PA measurement is at best limited. Substantial investigation into the responsiveness of PA measures to detect change is required to ensure that measures employed in future intervention and longitudinal research can detect meaningful change.
Although the validity, reliability and responsiveness to change are key when selecting a measure of PA and energy expenditure, other factors including feasibility and cost should be considered. For example, wearing several sensors around the body for a short period in a laboratory setting is often quite feasible, but prolonging the wear period for several days may be uncomfortable for participants, while reattachment of sensors may require specific and detailed training. The appropriateness of the measure for use in specific populations is critical. Activity monitors or HRMs may need to be attached to body locations that are visible and may be considered “embarrassing” for certain populations in free-living environments, likely resulting in lower compliance to wear protocols. Finally, while the cost of objective measures have reduced significantly and are now feasible for inclusion in large scale data collections (i.e. UK Biobank study, HELENA study), worn devices can be expensive to use in large populations, especially if recording needs to be concurrent, requiring 100’s or 1000’s of devices. Although these issues are often the dominant determinant for researchers when selecting a measure of PA, it is critical that researchers consider selecting the measure with the best validity, reliability and responsiveness to change available to them; a larger dataset with less valid measures may not always be superior to a smaller dataset.
The findings of this review have highlighted the substantial quantity of research which has focused on the validity, reliability and responsiveness to change of measures of PA. A substantial number of review articles have been conducted on the measurement of PA in adult populations. The majority of such reviews were not systematic in nature. Of the systematic reviews articles identified, the methodological quality (as assessed by the AMSTAR quality assessment tool) was relatively poor, with 3 reviews considered low quality, 16 articles considered medium quality and 3 articles considered high quality. An obvious increase in the quantity of research using objective measures of PA over the past number of decades is apparent. Unfortunately, with the enormous quantity of research on the methodological effectiveness of PA measures comes extreme variability in study design, data processing and statistical analysis conducted. Such variability makes comparison between measurement type and specific measurement devices/tools extremely difficult. The sometimes questionable study designs and research questions in some of the existing published literature is a reanalysis of “suitable” data, rather than from a study designed to collect data to answer a specific research questions. The authors propose that to aid researchers in making informed decisions on the best available measure of PA, the development of “best practise” protocols for study design and data collection, analysis and synthesis are required, which can be employed across all measures, providing comparable information that is easy for researchers from outside of the field to digest. The authors also propose that any future undertaking of reviews on the measurement of PA follow best practise, and ensure that the reviews conducted are of the highest possible quality. Such improvements will provide researchers with the best available evidence for making a decision on which measure of PA to employ.
Strengths and limitations
This review of reviews had limitations that should be taken into account when considering the findings presented here. As this article reviewed existing literature reviews, and due to potential methodological errors within these reviews, it is likely that some relevant literature on the methodological effectiveness for measures of PA has been overlooked. Additionally, articles that have been published since the publication of each review will also have been overlooked. Due to the quantity of identified articles, and difficulties in contacting primary authors regarding articles published over the last 60 years, the primary data from these articles was not sourced. Although prior research has systematically reviewed the literature for accuracy of measures of PA, and some narrative reviews have compared the methodological effectiveness of different measures of PA, this is the first study to comprehensively examine and collate details on the validity, reliability and responsiveness to change of a range of measures of PA in adult populations. For researchers that are selecting a measure of PA, this will enable the comparison between different measures of PA within one article, rather than having to refer to a wide range of available literature that examines each single measure. Additionally, rather than focusing solely on information presented within each existing review of the literature, the original articles referred to within each review were sought and data was extracted independently.
In general, objective measures of PA demonstrate less variability in properties of methodological effectiveness than self-report measures. Although no “perfect” tool for the examination of PA exists, it is suggested that researchers aim to incorporate appropriate objective measures, specific to the behaviours of interests, when examining PA in adults in free-living environments. Other criteria beyond methodological effectiveness often influence tool selection, including cost and feasibility. However, researchers must be cognisant of the value of increased methodological effectiveness of any measurement method for PA in adults. Additionally, although a wealth of research exists in relation to the methodological effectiveness of PA measures, it is clear that the development of an appropriate and consistent approach to conducting research and reporting findings in this domain is necessary to enable researchers to easily compare findings across instruments.
Kohl HW, Craig CL, Lambert EV, Inoue S, Alkandari JR, Leetongin G, Kahlmeier S. Group LPASW: The pandemic of physical inactivity: global action for public health. Lancet. 2012;380:294–305.
Article PubMed Google Scholar
Haskell WL, Lee I-M, Pate RR, Powell KE, Blair SN, Franklin BA, Macera CA, Heath GW, Thompson PD, Bauman A. Physical activity and public health: updated recommendation for adults from the American College of Sports Medicine and the American Heart Association. Circulation. 2007;116:1081–93.
Owen N, Healy GN, Matthews CE, Dunstan DW. Too much sitting: the population-health science of sedentary behavior. Exerc Sport Sci Rev. 2010;38:105–13.
Article PubMed PubMed Central Google Scholar
Shephard RJ, Aoyagi Y. Measurement of human energy expenditure, with particular reference to field studies: an historical perspective. Eur J Appl Physiol. 2012;112:2785–815.
Warren JM, Ekelund U, Besson H, Mezzani A, Geladas N, Vanhees L. Assessment of physical activity - a review of methodologies with reference to epidemiological research: a report of the exercise physiology section of the European Association of Cardiovascular Prevention and Rehabilitation. Eur J Prev Cardiol. 2010;17:127–39.
Article Google Scholar
Dishman RK, Washburn RA, Schoeller DA. Measurement of physical activity. Quest. 2001;53:295–309.
Trost SG, McIver KL, Pate RR. Conducting accelerometer-based activity assessments in field-based research. Med Sci Sports Exerc. 2005;37:S531–43.
Adams SA, Matthews CE, Ebbeling CB, Moore CG, Cunningham JE, Fulton J, Hebert JR. The effect of social desirability and social approval on self-reports of physical activity. Am J Epidemiol. 2005;161:389–98.
Sallis JF, Saelens BE. Assessment of physical activity by self-report: status, limitations, and future directions. Res Q Exerc Sport. 2000;71:1–14.
Neilson HK, Robson PJ, Friedenreich CM, Csizmadi I. Estimating activity energy expenditure: how valid are physical activity questionnaires? Am J Clin Nutr. 2008;87:279–91.
CAS PubMed Google Scholar
van Poppel MNM, Chinapaw MJM, Mokkink LB, van Mechelen W, Terwee CB. Physical Activity Questionnaires for Adults A Systematic Review of Measurement Properties. Sports Med. 2010;40:565–600.
Ainslie PN, Reilly T, Westerterp KR. Estimating Human Energy Expenditure: A Review of Techniques with Particular Reference to Doubly Labelled Water. Sports Med. 2003;33:683–98.
Bort-Roig J, Gilson N, Puig-Ribera A, Contreras R, Trost S. Measuring and Influencing Physical Activity with Smartphone Technology: A Systematic Review. Sports Med. 2014;44:671–86.
Freedson P, Miller K. Objective monitoring of physical activity using motion sensors and heart rate. Res Q Exerc Sport. 2000;71:21–9.
Tudor-Locke CE, Myers AM. Methodological considerations for researchers and practitioners using pedometers to measure physical (ambulatory) activity. Res Q Exerc Sport. 2001;72:1–12.
Article CAS PubMed Google Scholar
Prince SA, Adamo KB, Hamel ME, Hardt J, Gorber SC, Tremblay M. A comparison of direct versus self-report measures for assessing physical activity in adults: a systematic review. Int J Behav Nutr Phys Act. 2008;5:56.
Bassett DR Jr, Mahar MT, Rowe DA, Morrow JR Jr. Walking and measurement. Med Sci Sports Exerc. 2008;40:S529–36.
Kim Y, Park I, Kang M. Convergent validity of the international physical activity questionnaire (IPAQ): meta-analysis. Public Health Nutr. 2013;16:440–52.
Kwak L, Proper KI, Hagströmer M, Sjöström M. The repeatability and validity of questionnaires assessing occupational physical activity - a systematic review. Scand J Work Environ Health. 2011;37:6–29.
Bassett DR Jr. Validity and reliability issues in objective monitoring of physical activity. Res Q Exerc Sport. 2000;71:S30–6.
Bonomi AG, Westerterp KR. Advances in physical activity monitoring and lifestyle interventions in obesity: a review. Int J Obes. 2012;36:167–77.
Article CAS Google Scholar
Washburn RA. Assessment of physical activity in older adults. Res Q Exerc Sport. 2000;71:79–88.
Pennathur A, Magham R, Contreras LR, Dowling W. Daily living activities in older adults: Part I - a review of physical activity and dietary intake assessment methods. Int J Ind Ergon. 2003;32:389–404.
Meyer K, Stolz C, Rott C, Laederach-Hofmann K. Physical activity assessment and health outcomes in old age: how valid are dose-response relationships in epidemiologic studies? Eur Rev Aging Phys Act. 2009;6:7–17.
Murphy SL. Review of physical activity measurement using accelerometers in older adults: considerations for research design and conduct. Prev Med. 2009;48:108–14.
Kowalski K, Rhodes R, Naylor PJ, Tuokko H, MacDonald S. Direct and indirect measurement of physical activity in older adults: a systematic review of the literature. Int J Behav Nutr Phys. 2012;9:148.
Garatachea N, Torres Luque G, Gonzalez Gallego J. Physical activity and energy expenditure measurements using accelerometers in older adults. Nutr Hosp. 2010;25:224–30.
Gorman E, Hanson HM, Yang PH, Khan KM, Liu-Ambrose T, Ashe MC. Accelerometry analysis of physical activity and sedentary behavior in older adults: a systematic review and data analysis. Eur Rev Aging Phys Act. 2014;11:35–49.
Forsen L, Loland NW, Vuillemin A, Chinapaw MJM, van Poppel MNM, Mokkink LB, van Mechelen W, Terwee CB. Self-Administered Physical Activity Questionnaires for the Elderly A Systematic Review of Measurement Properties. Sports Med. 2010;40:601–23.
Cheung VH, Gray L, Karunanithi M. Review of Accelerometry for Determining Daily Activity Among Elderly Patients. Arch Phys Med Rehabil. 2011;92:998–1014.
Helmerhorst HJ, Brage S, Warren J, Besson H, Ekelund U. A systematic review of reliability and objective criterion-related validity of physical activity questionnaires. Int J Behav Nutr Phys Act. 2012;9:103.
Kowalski K, Rhodes R, Naylor PJ, Tuokko H, MacDonald S. Direct and indirect measurement of physical activity in older adults: a systematic review of the literature. Int J Behav Nutr Phys Act. 2012;9:148.
Plasqui G, Westerterp KR. Physical activity assessment with accelerometers: an evaluation against doubly labeled water. Obesity. 2007;15:2371–9.
Reiser LM, Schlenk EA. Clinical use of physical activity measures. J Am Acad Nurse Pract. 2009;21:87–94.
Centre for reviews dissemination. Systematic reviews: CRD’s guidance for undertaking reviews in health care: Centre for Reviews and Dissemination; 2009.
Melanson EL Jr, Freedson PS, Blair S. Physical activity assessment: a review of methods. Crit Rev Food Sci Nutr. 1996;36:385–96.
Duncan MJ, Badland HM, Mummery WK. Applying GPS to enhance understanding of transport-related physical activity. J Sci Med Sport. 2009;12:549–56.
Shea BJ, Grimshaw JM, Wells GA, Boers M, Andersson N, Hamel C, Porter AC, Tugwell P, Moher D, Bouter LM. Development of AMSTAR: a measurement tool to assess the methodological quality of systematic reviews. BMC Med Res Methodol. 2007;7:10.
Berlin JE, Storti KL, Brach JS. Using activity monitors to measure physical activity in free-living conditions. Phys Ther . 2006;86:1137–45.
PubMed Google Scholar
Butte NF, Ekelund U, Westerterp KR. Assessing physical activity using wearable monitors: measures of physical activity. Med Sci Sports Exerc. 2012;44:5–12.
Chen KY, Bassett DR. The technology of accelerometry-based activity monitors: Current and future. Med Sci Sports Exerc. 2005;37:490–S500.
Corder K, Brage S, Ekelund U. Accelerometers and pedometers: methodology and clinical application. Curr Opin Clin Nutr Metab Care. 2007;10:597–603.
Davidson M, de Morton N. A systematic review of the Human Activity Profile. Clin Rehabil. 2007;21:151–62.
DeLany JP. Measurement of energy expenditure. Pediatric Blood and Cancer. 2012;58:129–34.
Haskell WL, Kiernan M. Methodologic issues in measuring physical activity and physical fitness when evaluating the role of dietary supplements for physically active people. Am J Clin Nutr. 2000;72:541s–50s.
Lamonte MJ, Ainsworth BE. Quantifying energy expenditure and physical activity in the context of dose response. Med Sci Sports Exerc. 2001;33:S370–8.
Lee PH, Macfarlane DJ, Lam TH, Stewart SM. Validity of the International Physical Activity Questionnaire Short Form (IPAQ-SF): a systematic review. Int J Behav Nutr Phys Act. 2011;8:115.
Levine JA. Measurement of energy expenditure. Public Health Nutr. 2005;8:1123–32.
Liu SP, Gao RX, Freedson PS. Computational Methods for Estimating Energy Expenditure in Human Physical Activities. Med Sci Sports Exerc. 2012;44:2138–46.
Lowe SA, Ólaighin G. Monitoring human health behaviour in one's living environment: A technological review. Med Eng Phys. 2014;36:147–68.
Mathie MJ, Coster AC, Lovell NH, Celler BG. Accelerometry: providing an integrated, practical method for long-term, ambulatory monitoring of human movement. Physiol Meas. 2004;25:R1–20.
Matthews CE. Calibration of accelerometer output for adults. Med Sci Sports Exerc. 2005;37:512–S522.
Pedišić Ž, Bauman A. Accelerometer-based measures in physical activity surveillance: current practices and issues. Br J Sports Med. 2014;49:219–23.
Pierannunzi C, Hu SS, Balluz L. A systematic review of publications assessing reliability and validity of the Behavioral Risk Factor Surveillance System (BRFSS), 2004-2011. BMC Med Res Methodol. 2013;13:49.
Plasqui G, Bonomi AG, Westerterp KR. Daily physical activity assessment with accelerometers: new insights and validation studies. Obes Rev. 2013;14:451–62.
Reilly JJ, Penpraze V, Hislop J, Davies G, Grant S, Paton JY. Objective measurement of physical activity and sedentary behaviour: review with new data. Arch Dis Child. 2008;93:614–9.
Ridgers ND, Fairclough S. Assessing free-living physical activity using accelerometry: Practical issues for researchers and practitioners. Eur J Sport Sci. 2011;11:205–13.
Schutz Y, Weinsier RL, Hunter GR. Assessment of free-living physical activity in humans: an overview of currently available and proposed new measures. Obes Res. 2001;9:368–79.
Shephard RJ. Limits to the measurement of habitual physical activity by questionnaires. Br J Sports Med. 2003;37:197–206.
Article CAS PubMed PubMed Central Google Scholar
Strath SJ, Kaminsky LA, Ainsworth BE, Ekelund U, Freedson PS, Gary RA, Richardson CR, Smith DT, Swartz AM, Council Clinical C, Council Cardiovasc Stroke N. Guide to the Assessment of Physical Activity: Clinical and Research Applications A Scientific Statement From the American Heart Association. Circulation. 2013;128:2259–79.
Tudor-Locke C, Rowe DA. Using cadence to study free-living ambulatory behaviour. Sports Med. 2012;42:381–98.
Tudor-Locke C, Williams JE, Reis JP, Pluto D. Utility of pedometers for assessing physical activity: convergent validity. Sports Med. 2002;32:795–808.
Tudor-Locke C, Williams JE, Reis JP, Pluto D. Utility of pedometers for assessing physical activity: construct validity. Sports Med. 2004;34:281–91.
Valanou EM, Bamia C, Trichopoulou A. Methodology of physical-activity and energy-expenditure assessment: A review. J Public Health. 2006;14:58–65.
Van Remoortel H, Giavedoni S, Raste Y, Burtin C, Louvaris Z, Gimeno-Santos E, Langer D, Glendenning A, Hopkinson NS, Vogiatzis I, et al. Validity of activity monitors in health and chronic disease: a systematic review. Int J Behav Nutr Phys Act. 2012;9:84.
Vanhees L, Lefevre J, Philippaerts R, Martens M, Huygens W, Troosters T, Beunen G. How to assess physical activity? How to assess physical fitness? Eur J Prev Cardiol. 2005;12:102–14.
Washburn RA, Heath GW, Jackson AW. Reliability and validity issues concerning large-scale surveillance of physical activity. Res Q Exerc Sport. 2000;71:104–13.
Welk GJ. Principles of design and analyses for the calibration of accelerometry-based activity monitors. Med Sci Sports Exerc. 2005;37:501–11.
Westerterp KR. Assessment of physical activity: a critical appraisal. Eur J Appl Physiol. 2009;105:823–8.
Westerterp KR, Plasqui G. Physical activity and human energy expenditure. Curr Opin Clin Nutr Metab Care. 2004;7:607–13.
Yang CC, Hsu YL. A review of accelerometry-based wearable motion detectors for physical activity monitoring. Sensors. 2010;10:7772–88.
Andrew NE, Gabbe BJ, Wolfe R, Cameron PA. Evaluation of Instruments for Measuring the Burden of Sport and Active Recreation Injury. Sports Med. 2010;40:141–61.
Adams SA, Matthews CE, Ebbeling CB, Moore CG, Cunningham JE, Fulton J, et al. The effect of social desirability and social approval on self-reports of physical activity. Am J Epidemiol. 2005;161:389–98.
Barnard J, Tapsell LC, Davies P, Brenninger V, Storlien L. Relationship of high energy expenditure and variation in dietary intake with reporting accuracy on 7 day food records and diet histories in a group of healthy adult volunteers. Eur J Clin Nutr. 2002;56:358–67.
Besson H, Brage S, Jakes RW, Ekelund U, Wareham NJ. Estimating physical activity energy expenditure, sedentary time, and physical activity intensity by self-report in adults. Am J Clin Nutr. 2010;91:106–14.
Bonnefoy M, Normand S, Pachiaudi C, Lacour JR, Laville M, Kostka T. Simultaneous validation of ten physical activity questionnaires in older men: a doubly labeled water study. J Am Geriatr Soc. 2001;49:28–35.
Colbert LH, Matthews CE, Havighurst TC, Kim K, Schoeller DA. Comparative validity of physical activity measures in older adults. Med Sci Sports Exerc. 2011;43:867–76.
Conway JM, Irwin ML, Ainsworth BE. Estimating energy expenditure from the Minnesota Leisure Time Physical Activity and Tecumseh Occupational Activity questionnaires–a doubly labeled water validation. J Clin Epidemiol. 2002;55:392–9.
Conway JM, Seale JL, Jacobs DR, Irwin ML, Ainsworth BE. Comparison of energy expenditure estimates from doubly labeled water, a physical activity questionnaire, and physical activity records. Am J Clin Nutr. 2002;75:519–25.
Fuller Z, Horgan G, O'Reilly L, Ritz P, Milne E, Stubbs R. Comparing different measures of energy expenditure in human subjects resident in a metabolic facility. Eur J Clin Nutr. 2008;62:560–9.
Goran MI, Poehlman ET. Total energy expenditure and energy requirements in healthy elderly persons. Metabolism. 1992;41:744–53.
Irwin ML, Ainsworth BE, Conway JM. Estimation of energy expenditure from physical activity measures: determinants of accuracy. Obes Res. 2001;9:517–25.
Maddison R, Mhurchu CN, Jiang Y, Vander Hoorn S, Rodgers A, Lawes CM, Rush E. International physical activity questionnaire (IPAQ) and New Zealand physical activity questionnaire (NZPAQ): A doubly labelled water validation. Int J Behav Nutr Phys Act. 2007;4:62.
Masse LC, Fulton JE, Watson KL, Mahar MT, Meyers MC, Wong WW. Influence of body composition on physical activity validation studies using doubly labeled water. J Appl Physiol. 2004;96:1357–64.
Morio B, Ritz P, Verdier E, Montaurier C, Beaufrere B, Vermorel M. Critical evaluation of the factorial and heart-rate recording methods for the determination of energy expenditure of free-living elderly people. Br J Nutr. 1997;78:709–22.
Paul DR, Rhodes DG, Kramer M, Baer DJ, Rumpler WV. Validation of a food frequency questionnaire by direct measurement of habitual ad libitum food intake. Am J Epidemiol. 2005;162:806–14.
Rothenberg E, Bosaeus I, Lernfelt B, Landahl S, Steen B. Energy intake and expenditure: validation of a diet history by heart rate monitoring, activity diary and doubly labeled water. Eur J Clin Nutr. 1998;52:832–8.
Rush E, Valencia ME, Plank LD. Validation of a 7-day physical activity diary against doubly-labelled water. Ann Hum Biol. 2008;35:416–21.
Schulz LO, Harper IT, Smith CJ, Kriska AM, Ravussin E. Energy intake and physical activity in Pima Indians: comparison with energy expenditure measured by doubly‐labeled water. Obes Res. 1994;2:541–8.
Starling RD, Toth MJ, Matthews DE, Poehlman ET. Energy Requirements and Physical Activity of Older Free-Living African-Americans: A Doubly Labeled Water Study 1. J Clin Endocrinol Metab. 1998;83:1529–34.
Staten LK, Taren DL, Howell WH, Tobar M, Poehlman ET, Hill A, Reid PM, Ritenbaugh C. Validation of the Arizona Activity Frequency Questionnaire using doubly labeled water. Med Sci Sports Exerc. 2001;33:1959–67.
Koebnick C, Wagner K, Thielecke F, Moeseneder J, Hoehne A, Franke A, Meyer H, Garcia A, Trippo U, Zunft H. Validation of a simplified physical activity record by doubly labeled water technique. Int J Obes. 2005;29:302–9.
Leenders N, Sherman WM, Nagaraja H, Kien CL. Evaluation of methods to assess physical activity in free-living conditions. Med Sci Sports Exerc. 2001;33:1233–40.
Livingstone M, Strain J, Prentice A, Coward W, Nevin G, Barker M, Hickey R, McKenna P, Whitehead R. Potential contribution of leisure activity to the energy expenditure patterns of sedentary populations. Br J Nutr. 1991;65:145–55.
Lof M, Forsum E. Validation of energy intake by dietary recall against different methods to assess energy expenditure. J Hum Nutr Diet. 2004;17:471–80.
Lof M, Hannestad U, Forsum E. Assessing physical activity of women of childbearing age. Ongoing work to develop and evaluate simple methods. Food Nutr Bull. 2002;23:30–3.
Mahabir S, Baer DJ, Giffen C, Clevidence BA, Campbell WS, Taylor PR, Hartman TJ. Comparison of energy expenditure estimates from 4 physical activity questionnaires with doubly labeled water estimates in postmenopausal women. Am J Clin Nutr. 2006;84:230–6.
Philippaerts R, Westerterp K, Lefevre J. Doubly labelled water validation of three physical activity questionnaires. Int J Sports Med. 1999;20:284–9.
Racette SB, Schoeller DA, Kushner RF. Comparison of heart rate and physical activity recall with doubly labeled water in obese women. Med Sci Sports Exerc. 1995;27:126–33.
Rafamantanantsoa HH, Ebine N, Yoshioka M, Higuchi H, Yoshitake Y, Tanaka H, Saitoh S, Jones PJH. Validation of three alternative methods to measure total energy expenditure against the doubly labeled water method for older Japanese men. J Nutr Sci Vitaminol (Tokyo). 2002;48:517–23.
Reilly J, Lord A, Bunker V, Prentice A, Coward W, Thomas A, Briggs R. Energy balance in healthy elderly women. Br J Nutr. 1993;69:21–7.
Schulz S, Westerterp KR, Brück K. Comparison of energy expenditure by the doubly labeled water technique with energy intake, heart rate, and activity recording in man. Am J Clin Nutr. 1989;49:1146–54.
Schuit AJ, Schouten EG, Westerterp KR, Saris WH. Validity of the Physical Activity Scale for the Elderly (PASE): according to energy expenditure assessed by the doubly labeled water method. J Clin Epidemiol. 1997;50:541–6.
Seale JL, Klein G, Friedmann J, Jensen GL, Mitchell DC, Smiciklas-Wright H. Energy expenditure measured by doubly labeled water, activity recall, and diet records in the rural elderly. Nutrition. 2002;18:568–73.
Starling RD, Matthews DE, Ades PA, Poehlman ET. Assessment of physical activity in older individuals: a doubly labeled water study. J Appl Physiol. 1999;86:2090–6.
Walsh MC, Hunter GR, Sirikul B, Gower BA. Comparison of self-reported with objectively assessed energy expenditure in black and white women before and after weight loss. Am J Clin Nutr. 2004;79:1013–9.
Washburn RA, Jacobsen DJ, Sonko BJ, Hill JO, Donnelly JE. The validity of the Stanford Seven-Day Physical Activity Recall in young adults. Med Sci Sports Exerc. 2003;35:1374–80.
Aadahl M, Jørgensen T. Validation of a new self-report instrument for measuring physical activity. Med Sci Sports Exerc. 2003;35:1196–202.
Ainsworth B, Bassett DR Jr, Strath SJ, Swartz AM, O'Brien WL, Thompson RW, Jones DA, Macera CA, Kimsey CD. Comparison of three methods for measuring the time spent in physical activity. Med Sci Sports Exerc. 2000;32:S457–64.
Ainsworth BE, Leon AS, Richardson MT, Jacobs DR, Paffenbarger R. Accuracy of the college alumnus physical activity questionnaire. J Clin Epidemiol. 1993;46:1403–11.
Ainsworth BE, Richardson MT, Jacobs DR, Leon AS, Sternfeld B. Accuracy of recall of occupational physical activity by questionnaire. J Clin Epidemiol. 1999;52:219–27.
Arroll B, Jackson R, Beaglehole R. Validation of a three-month physical activity recall questionnaire with a seven-day food intake and physical activity diary. Epidemiology. 1991;2:296–9.
Baranowski T, Dworkin RJ, Cieslik CJ, Hooks P, Clearman DR, Ray L, Dunn JK, Nader PR. Reliability and validity of self report of aerobic activity: Family Health Project. Res Q Exerc Sport. 1984;55:309–17.
Bassett DR Jr, Cureton AL, Ainsworth BE. Measurement of daily walking distance-questionnaire versus pedometer. Med Sci Sports Exerc. 2000;32:1018–23.
Boon RM, Hamlin MJ, Steel GD, Ross JJ. Validation of the New Zealand physical activity questionnaire (NZPAQ-LF) and the international physical activity questionnaire (IPAQ-LF) with accelerometry. Br J Sports Med. 2008;44:741–6.
Brown WJ, Burton NW, Marshall AL, Miller YD. Reliability and validity of a modified self‐administered version of the Active Australia physical activity survey in a sample of mid‐age women. Aust N Z J Public Health. 2008;32:535–41.
Bull FC, Maslin TS, Armstrong T. Global physical activity questionnaire (GPAQ): nine country reliability and validity study. J Phys Act Health. 2009;6:790–804.
Bulley C, Donaghy M, Payne A, Mutrie N. Validation and modification of the Scottish Physical Activity Questionnaire for use in a female student population. Int J Health Promot Educ. 2005;43:117–24.
Busse M, Van Deursen R, Wiles CM. Real-life step and activity measurement: reliability and validity. J Med Eng Technol. 2009;33:33–41.
Carter-Nolan PL, Adams-Campbell LL, Makambi K, Lewis S, Palmer JR, Rosenberg L. Validation of physical activity instruments: Black Women’s Health Study. Ethn Dis. 2006;16:943–7.
Cartmel B, Moon T. Comparison of two physical activity questionnaires, with a diary, for assessing physical activity in an elderly population. J Clin Epidemiol. 1992;45:877–83.
Craig CL, Marshall AL, Sjostrom M, Bauman AE, Booth ML, Ainsworth BE, Pratt M, Ekelund U, Yngve A, Sallis JF, Oja P. International physical activity questionnaire: 12-country reliability and validity. Med Sci Sports Exerc. 2003;35:1381–95.
Davidson L, McNeill G, Haggarty P, Smith JS, Franklin MF. Free-living energy expenditure of adult men assessed by continuous heart-rate monitoring and doubly-labelled water. Br J Nutr. 1997;78:695–708.
De Cocker KA, De Bourdeaudhuij IM, Cardon GM. What do pedometer counts represent? A comparison between pedometer data and data from four different questionnaires. Public Health Nutr. 2009;12:74–81.
Deng H, Macfarlane D, Thomas G, Lao X, Jiang C, Cheng K, Lam T. Reliability and validity of the IPAQ-Chinese: the Guangzhou Biobank Cohort study. Med Sci Sports Exerc. 2008;40:303–7.
Dinger MK. Reliability and convergent validity of the National College Health Risk Behavior Survey physical activity items. J Health Educ. 2003;34:162–6.
Dinger MK, Behrens TK, Han JL. Validity and reliability of the International Physical Activity Questionnaire in college students. J Health Educ. 2006;37:337–43.
Dishman R, Steinhardt M. Reliability and concurrent validity for a 7-d re-call of physical activity in college students. Med Sci Sports Exerc. 1988;20:14–25.
Duncan GE, Sydeman SJ, Perri MG, Limacher MC, Martin AD. Can sedentary adults accurately recall the intensity of their physical activity? Prev Med. 2001;33:18–26.
Ekelund U, Sepp H, Brage S, Becker W, Jakes R, Hennings M, Wareham NJ. Criterion-related validity of the last 7-day, short form of the International Physical Activity Questionnaire in Swedish adults. Public Health Nutr. 2006;9:258–65.
Fjeldsoe B, Marshall A, Miller Y. Measurement properties of the Australian women's activity survey. Med Sci Sports Exerc. 2009;41:1020–33.
Friedenreich CM, Courneya KS, Neilson HK, Matthews CE, Willis G, Irwin M, Troiano R, Ballard-Barbash R. Reliability and validity of the past year total physical activity questionnaire. Am J Epidemiol. 2006;163:959–70.
Grimm EK, Swartz AM, Hart T, Miller NE, Strath SJ. Comparison of the IPAQ-Short Form and accelerometry predictions of physical activity in older adults. J Aging Phys Act. 2012;20:64–79.
Hagstromer M, Ainsworth BE, Oja P, Sjostrom M. Comparison of a subjective and an objective measure of physical activity in a population sample. J Phys Act Health. 2010;7:541–50.
Hallal PC, Simoes E, Reichert FF, Azevedo MR, Ramos LR, Pratt M, Brownson RC. Validity and reliability of the telephone-administered International Physical Activity Questionnaire in Brazil. J Phys Act Health. 2010;7:402–9.
Hallal PC, Victora CG, Wells JCK, RdC L, Valle NJ. Comparison of short and full-length International Physical Activity Questionnaires. J Phys Act Health. 2004;1:227–34.
Hekler EB, Buman MP, Haskell WL, Conway TL, Cain KL, Sallis JF, Saelens BE, Frank LD, Kerr J, King AC. Reliability and validity of CHAMPS self-reported sedentary-to-vigorous intensity physical activity in older adults. J Phys Act Health. 2012;9:225–36.
Hopkins WG, Wilson NC, Russell DG. Validation of the physical activity instrument for the Life in New Zealand national survey. Am J Epidemiol. 1991;133:73–82.
Hurtig-Wennlöf A, Hagströmer M, Olsson LA. The International Physical Activity Questionnaire modified for the elderly: aspects of validity and feasibility. Public Health Nutr. 2010;13:1847–54.
Kurtze N, Rangul V, Hustvedt B-E. Reliability and validity of the international physical activity questionnaire in the Nord-Trøndelag health study (HUNT) population of men. BMC Med Res Methodol. 2008;8:63.
Lee PH, Yu Y, McDowell I, Leung GM, Lam T, Stewart SM. Performance of the international physical activity questionnaire (short form) in subgroups of the Hong Kong chinese population. Int J Behav Nutr Phys Act. 2011;8:1479–5868.
Google Scholar
Leenders NY, Sherman WM, Nagaraja H. Comparisons of four methods of estimating physical activity in adult women. Med Sci Sports Exerc. 2000;32:1320–6.
Lemmer JT, Ivey FM, Ryan AS, Martel GF, Hurlbut DE, Metter JE, Fozard JL, Fleg JL, Hurley BF. Effect of strength training on resting metabolic rate and physical activity: age and gender comparisons. Med Sci Sports Exerc. 2001;33:532–41.
Macfarlane D, Chan A, Cerin E. Examining the validity and reliability of the Chinese version of the International Physical Activity Questionnaire, long form (IPAQ-LC). Public Health Nutr. 2011;14:443–50.
Macfarlane DJ, Lee CC, Ho EY, Chan K, Chan DT. Reliability and validity of the Chinese version of IPAQ (short, last 7 days). J Sci Med Sport. 2007;10:45–51.
Macfarlane DJ, Lee CC, Ho EY, Chan K-L, Chan D. Convergent validity of six methods to assess physical activity in daily life. J Appl Physiol. 2006;101:1328–34.
Mäder U, Martin BW, Schutz Y, Marti B. Validity of four short physical activity questionnaires in middle-aged persons. Med Sci Sports Exerc. 2006;38:1255–66.
Masse LC, Eason KE, Tortolero SR, Kelder SH. Comparing participants’ rating and compendium coding to estimate physical activity intensities. Meas Phys Educ Exerc Sci. 2005;9:1–20.
Matthews CE, Ainsworth BE, Hanby C, Pate RR, Addy C, Freedson PS, Jones DA, Macera CA. Development and testing of a short physical activity recall questionnaire. Med Sci Sports Exerc. 2005;37:986–94.
Matthews CE, Freedson PS. Field trial of a three-dimensional activity monitor: comparison with self report. Med Sci Sports Exerc. 1995;27:1071–8.
Matthews CE, Keadle SK, Sampson J, Lyden K, Bowles HR, Moore SC, Libertine A, Freedson PS, Fowke JH. Validation of a previous-day recall measure of active and sedentary behaviors. Med Sci Sports Exerc. 2013;45:1629–38.
Matthews CE, Shu X-O, Yang G, Jin F, Ainsworth BE, Liu D, Gao Y-T, Zheng W. Reproducibility and validity of the Shanghai Women’s Health Study physical activity questionnaire. Am J Epidemiol. 2003;158:1114–22.
Meriwether RA, McMahon PM, Islam N, Steinmann WC. Physical activity assessment: validation of a clinical assessment tool. Am J Prev Med. 2006;31:484–91.
Moy KL, Scragg RK, McLean G, Carr H. The New Zealand Physical Activity Questionnaires: validation by heart-rate monitoring in a multiethnic population. J Phys Act Health. 2008;5:S45–61.
EEK N, SAG N, Wu Y, Salim A, Tai ES, Lee J, Van Dam RM. Validity of the International Physical Activity Questionnaire and the Singapore Prospective Study Program physical activity questionnaire in a multiethnic urban Asian population. BMC Med Res Methodol. 2011;11:141.
Nicaise V, Marshall S, Ainsworth BE. Domain-specific physical activity and self-report bias among low-income Latinas living in San Diego County. J Phys Act Health. 2011;8:881–90.
Norman A, Bellocco R, Bergström A, Wolk A. Validity and reproducibility of self-reported total physical activity--differences by relative weight. Int J Obes Relat Metab Disord. 2001;25:682–8.
Resnick B, King A, Riebe D, Ory M. Measuring Physical Activity in Older Adults Use of the Community Health Activities Model Program for Seniors Physical Activity Questionnaire and the Yale Physical Activity Survey in Three Behavior Change Consortium Studies. West J Nurs Res. 2008;30:673–89.
Richardson MT, Ainsworth BE, Jacobs DR, Leon AS. Validation of the Stanford 7-day recall to assess habitual physical activity. Ann Epidemiol. 2001;11:145–53.
Richardson MT, Leon AS, Jacobs DR Jr, Ainsworth BE, Serfass R. Ability of the Caltrac accelerometer to assess daily physical activity levels. J Cardiopulm Rehabil Prev. 1995;15:107–13.
Roman-Viñas B, Serra-Majem L, Hagströmer M, Ribas-Barba L, Sjöström M, Segura-Cardona R. International physical activity questionnaire: reliability and validity in a Spanish population. Eur J Sport Sci. 2010;10:297–304.
Saglam M, Arikan H, Savci S, Inal-Ince D, Bosnak-Guclu M, Karabulut E, Tokgozoglu L. International physical activity questionnaire: reliability and validity of the turkish version 1. Percept Mot Skills. 2010;111:278–84.
Schmidt MD, Freedson PS, Chasan-Taber L. Estimating physical activity using the CSA accelerometer and a physical activity log. Med Sci Sports Exerc. 2003;35:1605–11.
Sidney S, Jacobs DR, Haskell WL, Armstrong MA, Dimicco A, Oberman A, Savage PJ, Slattery ML, Sternfeld B, Van Hom L. Comparison of two methods of assessing physical activity in the Coronary Artery Risk Development in Young Adults (CARDIA) Study. Am J Epidemiol. 1991;133:1231–45.
Singh PN, Fraser GE, Knutsen SF, Lindsted KD, Bennett HW. Validity of a physical activity questionnaire among African-American Seventh-day Adventists. Med Sci Sports Exerc. 2001;33:468–75.
Sirard J, Melanson EL, Li L, Freedson PS. Field evaluation of the Computer Science and Applications, Inc. physical activity monitor. Med Sci Sports Exerc. 1998;32:695–700.
Smith BJ, Marshall AL, Huang N. Screening for physical activity in family practice: evaluation of two brief assessment tools. Am J Prev Med. 2005;29:256–64.
Sobngwi E, Mbanya JCN, Unwin NC, Aspray TJ, Alberti K. Development and validation of a questionnaire for the assessment of physical activity in epidemiological studies in Sub-Saharan Africa. Int J Epidemiol. 2001;30:1361–8.
Stel VS, Smit JH, Pluijm SM, Visser M, Deeg DJ, Lips P. Comparison of the LASA Physical Activity Questionnaire with a 7-day diary and pedometer. J Clin Epidemiol. 2004;57:252–8.
Strath SJ, Bassett DR, Swartz AM. Comparison of the college alumnus questionnaire physical activity index with objective monitoring. Ann Epidemiol. 2004;14:409–15.
Strath SJ, Bassett DR Jr, Ham SA, Swartz AM. Assessment of physical activity by telephone interview versus objective monitoring. Med Sci Sports Exerc. 2003;35:2112–8.
Suzuki I, Kawakami N, Shimizu H. Reliability and validity of a questionnaire for assessment of energy expenditure and physical activity in epidemiological studies. J Epidemiol. 1998;8:152–9.
Tehard B, Saris WH, Astrup A, Martinez JA, Taylor MA, Barbe P, Richterova B, Guy-Grand B, Sorensen T, Oppert J. Comparison of two physical activity questionnaires in obese subjects: the NUGENOB study. Med Sci Sports Exerc. 2005;37:1535–41.
Theou O, Jakobi JM, Vandervoort AA, Jones GR. A comparison of physical activity (PA) assessment tools across levels of frailty. Arch Gerontol Geriatr. 2012;54:e307–14.
Thuy AB, Blizzard L, Schmidt M, Hung Luc P, Magnussen C, Dwyer T. Reliability and validity of the global physical activity questionnaire in Vietnam. J Phys Act Health. 2010;7:410–8.
Timperio A, Salmon J, Crawford D. Validity and reliability of a physical activity recall instrument among overweight and non-overweight men and women. J Sci Med Sport. 2003;6:477–91.
Timperio A, Salmon J, Rosenberg M, Bull FC. Do logbooks influence recall of physical activity in validation studies? Med Sci Sports Exerc. 2004;36:1181–6.
Tomioka K, Iwamoto J, Saeki K, Okamoto N. Reliability and validity of the International Physical Activity Questionnaire (IPAQ) in elderly adults: the Fujiwara-kyo Study. J Epidemiol (Japan). 2010;21:459–65.
van der Ploeg HP, Merom D, Chau JY, Bittman M, Trost SG, Bauman AE. Advances in population surveillance for physical activity and sedentary behavior: reliability and validity of time use surveys. Am J Epidemiol. 2010;172:1199–206.
van der Ploeg HP, Tudor-Locke C, Marshall AL, Craig C, Hagströmer M, Sjöström M, Bauman A. Reliability and validity of the international physical activity questionnaire for assessing walking. Res Q Exerc Sport. 2010;81:97–101.
Vandelanotte C, De Bourdeaudhuij I, Philippaerts R, Sjöström M, Sallis J. Reliability and validity of a computerized and Dutch version of the International Physical Activity Questionnaire (IPAQ). J Phys Act Health. 2005;2:63–75.
Washburn RA, Goldfield SR, Smith KW, McKinlay JB. The validity of self-reported exercise-induced sweating as a measure of physical activity. Am J Epidemiol. 1990;132:107–13.
Welk GJ, Thompson RW, Galper DI. A temporal validation of scoring algorithms for the 7-day physical activity recall. Meas Phys Educ Exerc Sci. 2001;5:123–38.
Wendel-Vos GW, Schuit AJ, Saris WH, Kromhout D. Reproducibility and relative validity of the short questionnaire to assess health-enhancing physical activity. J Clin Epidemiol. 2003;56:1163–9.
Wickel EE, Welk GJ, Eisenmann JC. Concurrent validation of the Bouchard Diary with an accelerometry-based monitor. Med Sci Sports Exerc. 2006;38:373–9.
Wilbur J, Chandler P, Miller AM, Davis GC, Aaronson LS, Mayo K. Measuring adherence to a women’s walking program. West J Nurs Res. 2001;23:8–32.
Yore MM, Ham SA, Ainsworth BE, Kruger J, Reis JP, Kohl H 3rd, Macera CA. Reliability and validity of the instrument used in BRFSS to assess physical activity. Med Sci Sports Exerc. 2007;39:1267–74.
Chasan-Taber L, Erickson JB, Nasca PC, Chasan-Taber S, Freedson PS. Validity and reproducibility of a physical activity questionnaire in women. Med Sci Sports Exerc. 2002;34:987–92.
Kwak L, Kremers SP, Brug J, Van Baak MA. Measuring physical activity in field studies: Comparison of a questionnaire, 24-hour recall and an accelerometer. Eur J Sport Sci. 2007;7:193–201.
Lagerros YT, Mucci LA, Bellocco R, Nyrén O, Bälter O, Bälter KA. Validity and reliability of self-reported total energy expenditure using a novel instrument. Eur J Epidemiol. 2006;21:227–36.
Richardson MT, Ainsworth BE, Wu H-C, Jacobs DR, Leon AS. Ability of the Atherosclerosis Risk in Communities (ARIC)/Baecke Questionnaire to assess leisure-time physical activity. Int J Epidemiol. 1995;24:685–93.
Hart TL, Ainsworth BE, Tudor-Locke C. Objective and subjective measures of sedentary behavior and physical activity. Med Sci Sports Exerc. 2011;43:449–56.
Lee KS, Trost SG. Validity and reliability of the 3-day physical activity recall in Singaporean adolescents. Res Q Exerc Sport. 2005;76:101–6.
Bassett DR Jr, Ainsworth BE, Swartz AM, Strath SJ, O'Brien WL, King GA. Validity of four motion sensors in measuring moderate intensity physical activity. Med Sci Sports Exerc. 2000;32:S471–80.
Strath S, Bassett D Jr, Swartz A. Comparison of MTI accelerometer cut-points for predicting time spent in physical activity. Int J Sports Med. 2003;24:298–303.
Booth ML, Owen N, Bauman AE, Gore CJ. Retest reliability of recall measures of leisure-time physical activity in Australian adults. Int J Epidemiol. 1996;25:153–9.
Ainsworth BE, Jacobs DR Jr, Leon AS. Validity and reliability of self-reported physical activity status: the Lipid Research Clinics questionnaire. Med Sci Sports Exerc. 1993;25:92–8.
Ainsworth BE, Sternfeld B, Richardson MT, Jackson K. Evaluation of the kaiser physical activity survey in women. Med Sci Sports Exerc. 2000;32:1327–38.
Batty D. Reliability of a physical activity questionnaire in middle-aged men. Public Health. 2000;114:474–6.
Berthouze SE, Minaire PM, Chatard J-C, Boutet C, Castells J, Lacour J-R. A new tool for evaluating energy expenditure: the " QAPSE " development and validation. Med Sci Sports Exerc. 1993;25:1405–14.
Bharathi A, Sandhya N, Vaz M. The development and characteristics of a physical activity questionnaire for epidemiological studies in urban middle class Indians. Indian J Med Res. 2000;111:95–102.
Blair SN, Dowda M, Pate RR, Kronenfeld J, Howe HG, Parker G, Blair A, Fridinger F. Reliability of long-term recall of participation in physical activity by middle-aged men and women. Am J Epidemiol. 1991;133:266–75.
Bouchard C, Tremblay A, Leblanc C, Lortie G, Savard R, Theriault G. A method to assess energy expenditure in children and adults. Am J Clin Nutr. 1983;37:461–7.
Brown W, Trost S, Bauman A, Mummery K, Owen N. Test-retest reliability of four physical activity measures used in population surveys. J Sci Med Sport. 2004;7:205–15.
Brownson RC, Eyler AA, King AC, Shyu Y-L, Brown DR, Homan SM. Reliability of information on physical activity and other chronic disease risk factors among US women aged 40 years or older. Am J Epidemiol. 1999;149:379–91.
Chasan-Taber L, Schmidt MD, Roberts DE, Hosmer D, Markenson G, Freedson PS. Development and validation of a pregnancy physical activity questionnaire. Med Sci Sports Exerc. 2004;36:1750–60.
Chasan-Taber S, Rimm EB, Stampfer MJ, Spiegelman D, Colditz GA, Giovannucci E, Ascherio A, Willett WC. Reproducibility and validity of a self-administered physical activity questionnaire for male health professionals. Epidemiology. 1996;7:81–6.
Chinapaw MJ, Slootmaker SM, Schuit AJ, van Zuidam M, van Mechelen W. Reliability and validity of the Activity Questionnaire for Adults and Adolescents (AQuAA). BMC Med Res Methodol. 2009;9:58.
Cust AE, Armstrong BK, Smith BJ, Chau J, van der Ploeg HP, Bauman A. Self-reported confidence in recall as a predictor of validity and repeatability of physical activity questionnaire data. Epidemiology. 2009;20:433–41.
Cust AE, Smith BJ, Chau J, van der Ploeg HP, Friedenreich CM, Armstrong BK, Bauman A. Validity and repeatability of the EPIC physical activity questionnaire: a validation study using accelerometers as an objective measure. Int J Behav Nutr Phys Act. 2008;5:33.
Cyarto E, Marshall A, Dickinson R, Brown W. Measurement properties of the CHAMPS physical activity questionnaire in a sample of older Australians. J Sci Med Sport. 2006;9:319–26.
De Abajo S, Larriba R, Marquez S. Validity and reliability of the Yale Physical Activity Survey in Spanish elderly. J Sports Med Phys Fitness. 2001;41:479–85.
Dinger M, Oman R, Taylor E, Vesely S, Able J. Stability and convergent validity of the Physical Activity Scale for the Elderly (PASE). J Sports Med Phys Fitness. 2004;44:186–92.
Dipietro L, Caspersen CJ, Ostfeld AM, Nadel ER. A survey for assessing physical activity among older adults. Med Sci Sports Exerc. 1993;
Dolan SH, Williams DP, Ainsworth BE, Shaw JM. Development and reproducibility of the bone loading history questionnaire. Med Sci Sports Exerc. 2006;38:1121–31.
Dubbert PM, Vander Weg MW, Kirchner KA, Shaw B. Evaluation of the 7-day physical activity recall in urban and rural men. Med Sci Sports Exerc. 2004;36:1646–54.
Eagan MS, Lyle RM, George P. A new selfreported comprehensive historical activity questionnaire for young women. J Phys Act Health. 2005;2:35.
Evenson KR, McGinn AP. Test-retest reliability of adult surveillance measures for physical activity and inactivity. Am J Prev Med. 2005;28:470–8.
Folsom AR, Jacobs DR, Caspersen CJ, Gomez-Marin O, Knudsen J. Test-retest reliability of the Minnesota leisure time physical activity questionnaire. J Chronic Dis. 1986;39:505–11.
Friedenreich CM, Courneya KS, Bryant HE. The lifetime total physical activity questionnaire: development and reliability. Med Sci Sports Exerc. 1998;30:266–74.
Gauthier AP, Lariviere M, Young N. Psychometric properties of the IPAQ: a validation study in a sample of northern Franco-Ontarians. J Phys Act Health. 2009;6:S54–60.
Giles-Corti B, Timperio A, Cutt H, Pikora TJ, Bull FC, Knuiman M, Bulsara M, Van Niel K, Shilton T. Development of a reliable measure of walking within and outside the local neighborhood: RESIDE's Neighborhood Physical Activity Questionnaire. Prev Med. 2006;42:455–9.
Gill DP, Jones GR, Zou G, Speechley M. The Phone-FITT: a brief physical activity interview for older adults. J Aging Phys Act. 2008;16:292–315.
Hagiwara A, Ito N, Sawai K, Kazuma K. Validity and reliability of the Physical Activity Scale for the Elderly (PASE) in Japanese elderly people. Geriatr Gerontol Int. 2008;8:143–51.
Harada ND, Chiu V, King AC, Stewart AL. An evaluation of three self-report physical activity instruments for older adults. Med Sci Sports Exerc. 2001;33:962–70.
Hopkins W, Wilson N, Worsley F, Russell D. Reliability of the core questionnaire in The Life in New Zealand survey. N Z J Health Phys. 1991;24:21–2.
Iwai N, Hisamichi S, Hayakawa N, Inaba Y, Nagaoka T, Sugimori H, Seki N, Sakata K, Suzuki K, Tamakoshi A. Validity and reliability of single-item questions about physical activity. J Epidemiol. 2001;11:211–8.
Jacobs DR Jr, Ainsworth BE, Hartman TJ, Leon AS. A simultaneous evaluation of 10 commonly used physical activity questionnaires. Med Sci Sports Exerc. 1993;25:81–91.
Kolbe-Alexander TL, Lambert EV, Harkins JB, Ekelund U. Comparison of two methods of measuring physical activity in South African older adults. J Aging Phys Act. 2006;14:98–114.
Kurtze N, Rangul V, Hustvedt B-E, Flanders WD. Reliability and validity of self-reported physical activity in the Nord-Trøndelag Health Study (HUNT 2). Eur J Epidemiol. 2007;22:379–87.
Kurtze N, Rangul V, Hustvedt B-E, Flanders WD. Reliability and validity of self-reported physical activity in the Nord-Trøndelag Health Study—HUNT 1. Scand J Public Health. 2008;36:52–61.
Lakka TA, Salonen JT. Intra-person variability of various physical activity assessments in the Kuopio Ischaemic Heart Disease Risk Factor Study. Int J Epidemiol. 1992;21:467–72.
Lee MM, Whittemore AS, Jung DL. Reliability of recalled physical activity, cigarette smoking, and alcohol consumption. Ann Epidemiol. 1992;2:705–14.
Levin S, Jacobs DR, Ainsworth BE, Richardson MT, Leon AS. Intra-individual variation and estimates of usual physical activity. Ann Epidemiol . 1999;9:481–8.
Lowther M, Mutrie N, Loughlan C, McFarlane C. Development of a Scottish physical activity questionnaire: a tool for use in physical activity interventions. Br J Sports Med. 1999;33:244–9.
Matton L, Wijndaele K, Duvigneaud N, Duquet W, Philippaerts R, Thomis M, Lefevre J. Reliability and validity of the Flemish Physical Activity Computerized Questionnaire in adults. Res Q Exerc Sport. 2007;78:293–306.
Meyer A-M, Evenson KR, Morimoto L, Siscovick D, White E. Test-Retest Reliability of the WHI Physical Activity Questionnaire. Med Sci Sports Exerc. 2009;41:530–8.
O'Brien-Cousins S. An older adult exercise status inventory: reliability and validity. Journal of Sport Behavior. 1996;19:288–306.
Orsini N, Bellocco R, Bottai M, Pagano M, Wolk A. Reproducibility of the past year and historical self-administered total physical activity questionnaire among older women. Eur J Epidemiol. 2007;22:363–8.
Pettee GK, McClain JJ, Lee CD, Swan PD, Alvar BA, Mitros MR, Ainsworth BE. Evaluation of physical activity measures used in middle-aged women. Med Sci Sports Exerc. 2009;41:1403–12.
Philippaerts RM, Lefevre J. Reliability and validity of three physical activity questionnaires in Flemish males. Am J Epidemiol. 1998;147:982–90.
Pols MA, Peeters P, Kemper HC, Collette H. Repeatability and relative validity of two physical activity questionnaires in elderly women. Med Sci Sports Exerc. 1996;28:1020–5.
Pols MA, Peeters PH, Bueno-de-Mesquita HB, Ocke MC, Wentink CA, Kemper HC, Collette HJ. Validity and repeatability of a modified Baecke questionnaire on physical activity. Int J Epidemiol. 1995;24:381–8.
Pols MA, Peeters PH, Ocké MC, Bueno-de-Mesquita HB, Slimani N, Kemper HC, Collette HJ. Relative validity and repeatability of a new questionnaire on physical activity. Prev Med. 1997;26:37–43.
Rauh MJ, Hovell MF, Hofstetter CR, Sallis JF, Gleghorn A. Reliability and validity of self-reported physical activity in Latinos. Int J Epidemiol. 1992;21:966–71.
Reed JA, Phillips DA. Relationships between physical activity and the proximity of exercise facilities and home exercise equipment used by undergraduate university students. J Am Coll Health. 2005;53:285–90.
Reis JP, DuBose KD, Ainsworth BE, Macera CA, Yore MM. Reliability and validity of the occupational physical activity questionnaire. Med Sci Sports Exerc. 2005;37:2075–83.
Richardson MT, Leon AS, Jacobs DR, Ainsworth BE, Serfass R. Comprehensive evaluation of the Minnesota leisure time physical activity questionnaire. J Clin Epidemiol. 1994;47:271–81.
Roeykens J, Rogers R, Meeusen R, Magnus L, Borms J, de Meirleir K. Validity and reliability in a Flemish population of the WHO-MONICA Optional Study of Physical Activity Questionnaire. Med Sci Sports Exerc. 1998;30:1071–5.
Rütten A, Vuillemin A, Ooijendijk W, Schena F, Sjöström M, Stahl T, Vanden Auweele Y, Welshman J, Ziemainz H. Physical activity monitoring in europe. The European Physical Activity Surveillance System (EUPASS) approach and indicator testing. Public Health Nutr. 2003;6:377–84.
Sallis JF, Haskell WL, Wood PD, Fortmann SP, Rogers T, Blair SN, Paffenbarger RS. Physical activity assessment methodology in the Five-City Project. Am J Epidemiol. 1985;121:91–106.
Schuler PB, Richardson MT, Ochoa P, Wang MQ. Accuracy and repeatability of the Yale physical activity survey in assessing physical activity of older adults. Percept Mot Skills. 2001;93:163–77.
Singh PN, Tonstad S, Abbey DE, Fraser GE. Validity of selected physical activity questions in white Seventh-day Adventists and non-Adventists. Med Sci Sports Exerc. 1996;28:1026–37.
Smitherman TA, Dubbert PM, Grothe KB, Sung JH, Kendzor DE, Reis JP, Ainsworth BE, Newton RL Jr, Lesniak KT, Taylor HA Jr. Validation of the Jackson Heart Study physical activity survey in African Americans. J Phys Act Health. 2009;6:S124–32.
Stein AD, Lederman RI, Shea S. The Behavioral Risk Factor Surveillance System questionnaire: its reliability in a statewide sample. Am J Public Health. 1993;83:1768–72.
Stewart AL, Mills KM, King AC, Haskell WL, Gillis D, Ritter PL. CHAMPS physical activity questionnaire for older adults: outcomes for interventions. Med Sci Sports Exerc. 2001;33:1126–41.
Trinh OT, Do Nguyen N, van der Ploeg HP, Dibley MJ, Bauman A. Test-retest repeatability and relative validity of the Global Physical Activity Questionnaire in a developing country context. J Phys Act Health. 2009;6:S46–53.
Voorrips LE, Ravelli AC, Dongelmans A, Deurenberg P, van Staveren WA. A physical activity questionnaire for the elderly. Med Sci Sports Exerc. 1991;23:974–9.
Vuillemin A, Oppert J-M, Guillemin F, Essermeant L, Fontvieille A-M, Galan P, Kriska AM, Hercberg S. Self-administered questionnaire compared with interview to assess past-year physical activity. Med Sci Sports Exerc. 2000;32:1119–24.
Wareham NJ, Jakes RW, Rennie KL, Mitchell J, Hennings S, Day NE. Validity and repeatability of the EPIC-Norfolk physical activity questionnaire. Int J Epidemiol. 2002;31:168–74.
Wareham NJ, Jakes RW, Rennie KL, Schuit J, Mitchell J, Hennings S, Day NE. Validity and repeatability of a simple index derived from the short physical activity questionnaire used in the European Prospective Investigation into Cancer and Nutrition (EPIC) study. Public Health Nutr. 2003;6:407–13.
Washburn R, Cook T, LaPorte R. The objective assessment of physical activity in an occupationally active group. J Sports Med Phys Fitness. 1989;29:279–84.
Washburn RA, Smith KW, Jette AM, Janney CA. The Physical Activity Scale for the Elderly (PASE): development and evaluation. J Clin Epidemiol. 1993;46:153–62.
Weller I, Corey PN. A study of the reliability of the Canada Fitness Survey questionnaire. Med Sci Sports Exerc. 1998;30:1530–6.
Wilbur J, Holm K, Dan A. A quantitative survey to measure energy expenditure in midlife women. J Nurs Meas. 1993;1:29–40.
Yasunaga A, Park H, Watanabe E, Togo F, Park S, Shephard RJ, Aoyagi Y. Development and evaluation of the physical activity questionnaire for elderly Japanese: the Nakanojo study. J Aging Phys Act. 2007;15:398–411.
Yore MM, Ham SA, Ainsworth BE, Macera CA, Jones DA, Kohl HW III. Occupational physical activity: reliability and comparison of activity levels. J Phys Act Health. 2005;3
Giles K, Marshall AL. The repeatability and accuracy of CHAMPS as a measure of physical activity in a community sample of older Australian adults. J Phys Act Health. 2009;6:221–9.
Hayden-Wade HA, Coleman KJ, Sallis JF, Armstrong C. Validation of the telephone and in-person interview versions of the 7-day PAR. Med Sci Sports Exerc. 2003;35:801–9.
Hamdorf PA, Withers RT, Penhall RK, Haslam MV. Physical training effects on the fitness and habitual activity patterns of elderly women. Arch Phys Med Rehabil. 1992;73:603–8.
Abel MG, Hannon JC, Sell K, Lillie T, Conlin G, Anderson D. Validation of the Kenz Lifecorder EX and ActiGraph GT1M accelerometers for walking and running in adults. Appl Physiol Nutr Metab. 2008;33:1155–64.
Allen FR, Ambikairajah E, Lovell NH, Celler BG. Classification of a known sequence of motions and postures from accelerometry data using adapted Gaussian mixture models. Physiol Meas. 2006;27:935.
Aminian K, Robert P, Buchser E, Rutschmann B, Hayoz D, Depairon M. Physical activity monitoring based on accelerometry: validation and comparison with video observation. Med Biol Eng Comput. 1999;37:304–8.
Assah FK, Ekelund U, Brage S, Corder K, Wright A, Mbanya JC, Wareham NJ. Predicting Physical Activity Energy Expenditure Using Accelerometry in Adults From Sub‐Sahara Africa. Obesity. 2009;17:1588–95.
Assah FK, Ekelund U, Brage S, Wright A, Mbanya JC, Wareham NJ. Accuracy and validity of a combined heart rate and motion sensor for the measurement of free-living physical activity energy expenditure in adults in Cameroon. Int J Epidemiol. 2010;40:112–20.
Bonomi AG, Goris A, Yin B, Westerterp KR. Detection of type, duration, and intensity of physical activity using an accelerometer. Med Sci Sports Exerc. 2009;41:1770–7.
Bonomi AG, Plasqui G, Goris AH, Westerterp KR. Improving assessment of daily energy expenditure by identifying types of physical activity with a single accelerometer. J Appl Physiol. 2009;107:655–61.
Bonomi AG, Plasqui G, Goris AH, Westerterp KR. Estimation of Free‐Living Energy Expenditure Using a Novel Activity Monitor Designed to Minimize Obtrusiveness. Obesity. 2010;18:1845–51.
Bussmann JB, Tulen JH, Herel EC, Stam HJ. Quantification of physical activities by means of ambulatory accelerometry: a validation study. Psychophysiology. 1998;35:488–96.
Carter J, Wilkinson D, Blacker S, Rayson M, Bilzon J, Izard R, Coward A, Wright A, Nevill A, Rennie K. An investigation of a novel three-dimensional activity monitor to predict free-living energy expenditure. J Sports Sci. 2008;26:553–61.
Choquette S, Chuin A, Lalancette D-A, Brochu M, Dionne IJ. Predicting energy expenditure in elders with the metabolic cost of activities. Med Sci Sports Exerc. 2009;41:1915–20.
Conger SA, Strath S, Bassett D. Validity and reliability of the Fitsense FS-1 speedometer during walking and running. Int J Sports Med. 2005;26:208–13.
Connolly CP, Coe DP, Kendrick JM, Basset D, Thompson DL. Accuracy of physical activity monitors in pregnant women. Med Sci Sports Exerc. 2011;43:1100–5.
Dijkstra B, Kamsma Y, Zijlstra W. Detection of gait and postures using a miniaturised triaxial accelerometer-based system: accuracy in community-dwelling older adults. Arch Phys Med Rehabil. 2010;39:259–62.
Dugas L, Carstens M, Ebersole K, Schoeller D, Durazo-Arvizu R, Lambert E, Luke A. Energy expenditure in young adult urban informal settlement dwellers in South Africa. Eur J Clin Nutr. 2009;63:805–7.
Ermes M, Parkka J, Mantyjarvi J, Korhonen I. Detection of daily activities and sports with wearable sensors in controlled and uncontrolled conditions. IEEE Trans Inf Technol Biomed. 2008;12:20–6.
Esliger DW, Probert A, Connor GS, Bryan S, Laviolette M, Tremblay MS. Validity of the Actical accelerometer step-count function. Med Sci Sports Exerc. 2007;39:1200–4.
Godfrey A, Culhane K, Lyons G. Comparison of the performance of the activPAL™ Professional physical activity logger to a discrete accelerometer-based activity monitor. Med Eng Phys. 2007;29:930–4.
Grant PM, Dall PM, Mitchell SL, Granat MH. Activity-monitor accuracy in measuring step number and cadence in community-dwelling older adults. J Aging Phys Act. 2008;16:201–14.
Grant PM, Ryan CG, Tigbe WW, Granat MH. The validation of a novel activity monitor in the measurement of posture and motion during everyday activities. Br J Sports Med. 2006;40:992–7.
He Y, Li Y: Physical activity recognition utilizing the built-in kinematic sensors of a smartphone. Int J Distrib Sens N 2013, 2013.
Jacobi D, Perrin AE, Grosman N, Doré MF, Normand S, Oppert JM, Simon C. Physical Activity‐Related Energy Expenditure With the RT3 and TriTrac Accelerometers in Overweight Adults. Obesity. 2007;15:950–6.
Johannsen DL, Calabro MA, Stewart J, Franke W, Rood JC, Welk GJ. Accuracy of armband monitors for measuring daily energy expenditure in healthy adults. Med Sci Sports Exerc. 2010;42:2134–40.
Karantonis DM, Narayanan MR, Mathie M, Lovell NH, Celler BG. Implementation of a real-time human movement classifier using a triaxial accelerometer for ambulatory monitoring. IEEE Trans Inf Technol Biomed. 2006;10:156–67.
Koehler K, Braun H, De Marees M, Fusch G, Fusch C, Schaenzer W. Assessing energy expenditure in male endurance athletes: validity of the SenseWear Armband. Med Sci Sports Exerc. 2011;43:1328–33.
Kumahara H, Schutz Y, Ayabe M, Yoshioka M, Yoshitake Y, Shindo M, Ishii K, Tanaka H. The use of uniaxial accelerometry for the assessment of physical-activity-related energy expenditure: a validation study against whole-body indirect calorimetry. Br J Nutr. 2004;91:235–43.
Kwon S, Jamal M, Zamba GK, Stumbo P, Samuel I. Validation of a novel physical activity assessment device in morbidly obese females. J Obes. 2010;2010
Le Masurier GC, Lee SM, Tudor-Locke C. Motion sensor accuracy under controlled and free-living conditions. Med Sci Sports Exerc. 2004;36:905–10.
Le Masurier GC, Tudor-Locke C. Comparison of pedometer and accelerometer accuracy under controlled conditions. Med Sci Sports Exerc. 2003;35:867–71.
Leenders NY, Sherman WM, Nagaraja HN. Energy expenditure estimated by accelerometry and doubly labeled water: do they agree? Med Sci Sports Exerc. 2006;38:2165–72.
Levine JA, McCrady SK, Lanningham-Foster LM, Kane PH, Foster RC, Manohar CU. The role of free-living daily walking in human weight gain and obesity. Diabetes. 2008;57:548–54.
Liu S-H, Chang Y-J. Using accelerometers for physical actions recognition by a neural fuzzy network. Telemedicine and e-Health. 2009;15:867–76.
Löf M. Physical activity pattern and activity energy expenditure in healthy pregnant and non-pregnant Swedish women. Eur J Clin Nutr. 2011;65:1295–301.
Lof M, Hannestad U, Forsum E. Comparison of commonly used procedures, including the doubly-labelled water technique, in the estimation of total energy expenditure of women with special reference to the significance of body fatness. Br J Nutr. 2003;90:961–8.
Maddison R, Jiang Y, Vander Hoorn S, Ni Mhurchu C, Lawes CM, Rodgers A, Rush E. Estimating energy expenditure with the RT3 triaxial accelerometer. Res Q Exerc Sport. 2009;80:249–56.
Maddocks M, Petrou A, Skipper L, Wilcock A. Validity of three accelerometers during treadmill walking and motor vehicle travel. Br J Sports Med. 2010;44:606–8.
Marsh AP, Vance RM, Frederick TL, Hesselmann SA, Rejeski WJ. Objective assessment of activity in older adults at risk for mobility disability. Med Sci Sports Exerc. 2007;39:1020–6.
Mathie M, Celler BG, Lovell NH, Coster A. Classification of basic daily movements using a triaxial accelerometer. Med Biol Eng Comput. 2004;42:679–87.
Mathie M, Coster A, Lovell N, Celler B. Detection of daily physical activities using a triaxial accelerometer. Med Biol Eng Comput. 2003;41:296–301.
Najafi B, Aminian K, Paraschiv-Ionescu A, Loew F, Büla CJ, Robert P. Ambulatory system for human motion analysis using a kinematic sensor: monitoring of daily physical activity in the elderly. IEEE Trans Biomed Eng. 2003;50:711–23.
Plasqui G, Joosen AM, Kester AD, Goris AH, Westerterp KR. Measuring Free‐Living Energy Expenditure and Physical Activity with Triaxial Accelerometry. Obes Res. 2005;13:1363–9.
Preece SJ, Goulermas JY, Kenney LP, Howard D. A comparison of feature extraction methods for the classification of dynamic activities from accelerometer data. IEEE Trans Biomed Eng. 2009;56:871–9.
Rafamantanantsoa HH, Ebine N, Yoshioka M, Yoshitake Y, Tanaka H, Saitoh S, Jones PJH. The role of exercise physical activity in varying the total energy expenditure in healthy Japanese men 30 to 69 years of age. J Nutr Sci Vitaminol (Tokyo). 2003;49:120–4.
Rowlands AV, Stone MR, Eston RG. Influence of speed and step frequency during walking and running on motion sensor output. Med Sci Sports Exerc. 2007;39:716–27.
Ryan CG, Grant PM, Tigbe WW, Granat MH. The validity and reliability of a novel activity monitor as a measure of walking. Br J Sports Med. 2006;40:779–84.
Staudenmayer J, Pober D, Crouter S, Bassett D, Freedson P. An artificial neural network to estimate physical activity energy expenditure and identify physical activity type from an accelerometer. J Appl Physiol. 2009;107:1300–7.
St-Onge M, Mignault D, Allison DB, Rabasa-Lhoret R. Evaluation of a portable device to measure daily energy expenditure in free-living adults. Am J Clin Nutr. 2007;85:742–9.
Storti KL, Pettee KK, Brach JS, Talkowski JB, Richardson CR, Kriska AM. Gait speed and step-count monitor accuracy in community-dwelling older adults. Med Sci Sports Exerc. 2008;40:59–64.
Wu W, Dasgupta S, Ramirez EE, Peterson C, Norman GJ. Classification accuracies of physical activities using smartphone motion sensors. J Med Internet Res. 2012;14:e130.
Yang J-Y, Wang J-S, Chen Y-P. Using acceleration measurements for activity recognition: An effective learning algorithm for constructing neural classifiers. Pattern Recogn Lett. 2008;29:2213–20.
Zhang K, Werner P, Sun M, Pi‐Sunyer FX, Boozer CN. Measurement of human daily physical activity. Obes Res. 2003;11:33–40.
Lee MH, Kim J, Jee SH, Yoo SK. Integrated solution for physical activity monitoring based on mobile phone and PC. Healthc Inform Res. 2011;17:76–86.
Mackey DC, Manini TM, Schoeller DA, Koster A, Glynn NW, Goodpaster BH, Satterfield S, Newman AB, Harris TB, Cummings SR. Validation of an armband to measure daily energy expenditure in older adults. J Gerontol A Biol Sci Med Sci. 2011;66A:1108–13.
Article PubMed Central Google Scholar
Anderson I, Maitland J, Sherwood S, Barkhuus L, Chalmers M, Hall M, Brown B, Muller H. Shakra: tracking and sharing daily activity levels with unaugmented mobile phones. Mobile Networks and Applications. 2007;12:185–99.
Atallah L, Leong J, Lo B, Yang G-Z. Energy expenditure prediction using a miniaturized ear-worn sensor. Med Sci Sports Exerc. 2011;43:1369–77.
Ayen TG, Montoye HJ. Estimation of energy expenditure with a simulated three-dimensional accelerometer. Journal of Ambulatory Monitoring. 1988;1:293–301.
Balogun JA, Amusa LO, Onyewadume IU. Factors affecting Caltrac® and Calcount® accelerometer output. Phys Ther. 1988;68:1500–4.
Balogun JA, Martin DA, Clendenin MA. Calorimetric validation of the Caltrac® accelerometer during level walking. Phys Ther. 1989;69:501–9.
Bao L, Intille SS. Activity recognition from user-annotated acceleration data. Pervasive Computing. 2004;3001:1–17.
Barreira TV, Brouillette RM, Foil HC, Keller JN, Tudor-Locke C. Comparison of Older Adults’ Steps per Day Using an NL-1000 Pedometer and Two GT3X+ Accelerometer Filters. J Aging Phys Act. 2013;21:402–16.
Berntsen S, Hageberg R, Aandstad A, Mowinckel P, Anderssen SA, Carlsen K, Andersen LB. Validity of physical activity monitors in adults participating in free-living activities. Br J Sports Med. 2010;44:657–64.
Berntsen S, Stafne SN, Mørkved S. Physical activity monitor for recording energy expenditure in pregnancy. Acta Obstet Gynecol Scand. 2011;90:903–7.
Bouten CV, Koekkoek KT, Verduin M, Kodde R, Janssen JD. A triaxial accelerometer and portable data processing unit for the assessment of daily physical activity. IEEE Trans Biomed Eng. 1997;44:136–47.
Bouten CV, Sauren AA, Verduin M, Janssen J. Effects of placement and orientation of body-fixed accelerometers on the assessment of energy expenditure during walking. Med Biol Eng Comput. 1997;35:50–6.
Bouten CV, Westerterp KR, Verduin M, Janssen J. Assessment of energy expenditure for physical activity using a triaxiaI accelerometer. Med Sci Sports Exerc. 1994;26:1516–23.
Brage S, Brage N, Franks P, Ekelund U, Wareham N. Reliability and validity of the combined heart rate and movement sensor Actiheart. Eur J Clin Nutr. 2005;59:561–70.
Brage S, Wedderkopp N, Franks PW, Andersen LB, Froberg K. Reexamination of validity and reliability of the CSA monitor in walking and running. Med Sci Sports Exerc. 2003;35:1447–54.
Brooks AG, Gunn SM, Withers RT, Gore CJ, Plummer JL. Predicting walking METs and energy expenditure from speed or accelerometry. Med Sci Sports Exerc. 2005;37:1216–23.
Brugniaux JV, Niva A, Pulkkinen I, Laukkanen RM, Richalet J-P, Pichon AP. Polar Activity Watch 200: a new device to accurately assess energy expenditure. Br J Sports Med. 2010;44:245–9.
Cain KL, Conway TL, Adams MA, Husak LE, Sallis JF. Comparison of older and newer generations of ActiGraph accelerometers with the normal filter and the low frequency extension. Int J Behav Nutr Phys Act. 2013;10:51.
Campbell KL, Crocker P, McKenzie DC. Field evaluation of energy expenditure in women using Tritrac accelerometers. Med Sci Sports Exerc. 2002;34:1667–74.
Chen KY, Acra SA, Majchrzak K, Donahue CL, Baker L, Clemens L, Sun M, Buchowski MS. Predicting energy expenditure of physical activity using hip-and wrist-worn accelerometers. Diabetes Technology and Therapeutics. 2003;5:1023–33.
Chen KY, Sun M. Improving energy expenditure estimation by using a triaxial accelerometer. J Appl Physiol. 1997;83:2112–22.
Copeland JL, Esliger DW. Accelerometer assessment of physical activity in active, healthy older adults. J Aging Phys Act. 2009;17:17–30.
Crouter SE, Bassett DR. A new 2-regression model for the Actical accelerometer. Br J Sports Med. 2008;42:217–24.
Crouter SE, Churilla JR, Bassett DR. Accuracy of the Actiheart for the assessment of energy expenditure in adults. Eur J Clin Nutr. 2008;62:704–11.
Crouter SE, Churilla JR, Bassett DR Jr. Estimating energy expenditure using accelerometers. Eur J Appl Physiol. 2006;98:601–12.
Crouter SE, Clowers KG, Bassett DR. A novel method for using accelerometer data to predict energy expenditure. J Appl Physiol. 2006;100:1324–31.
Crouter SE, DellaValle DM, Haas JD, Frongillo EA, Bassett DR. Validity of actiGraph 2-regression model and Matthews and NHANES and cut-points for assessing free-Living physical activity. J Phys Act Health. 2013;10:504–14.
Crouter SE, DellaValle DM, Horton M, Haas JD, Frongillo EA, Bassett DR Jr. Validity of the Actical for estimating free-living physical activity. Eur J Appl Physiol. 2011;111:1381–9.
DeVoe D, Gotshall R, McArthur T. Comparison of the RT3 Research Tracker and Tritrac R3D accelerometers. Percept Mot Skills. 2003;97:510–8.
Donaire-Gonzalez D, de Nazelle A, Seto E, Mendez M, Nieuwenhuijsen MJ, Jerrett M. Comparison of physical activity measures using mobile phone-based CalFit and Actigraph. J Med Internet Res. 2013;15:111.
Drenowatz C, Eisenmann JC. Validation of the SenseWear Armband at high intensity exercise. Eur J Appl Physiol. 2011;111:883–7.
Duncan GE, Lester J, Migotsky S, Goh J, Higgins L, Borriello G. Accuracy of a novel multi-sensor board for measuring physical activity and energy expenditure. Eur J Appl Physiol. 2011;111:2025–32.
Esliger DW, Rowlands AV, Hurst TL, Catt M, Murray P, Eston RG. Validation of the GENEA Accelerometer. Med Sci Sports Exerc. 2011;43:1085–93.
Fehling PC, Smith DL, Warner SE, Dalsky GP. Comparison of accelerometers with oxygen consumption in older adults during exercise. Med Sci Sports Exerc. 1999;31:171–5.
Freedson PS, Melanson E, Sirard J. Calibration of the Computer Science and Applications, Inc. accelerometer. Med Sci Sports Exerc. 1998;30:777–81.
Fruin ML, Rankin JW. Validity of a multi-sensor armband in estimating rest and exercise energy expenditure. Med Sci Sports Exerc. 2004;36:1063–9.
Fudge BW, Wilson J, Easton C, Irwin L, Clark J, Haddow O, Kayser B, Pitsiladis YP. Estimation of oxygen uptake during fast running using accelerometry and heart rate. Med Sci Sports Exerc. 2007;39:192–8.
Hageman PA, Norman JF, Pfefferkorn KL, Reiss NJ, Riesberg KA. Comparison of two physical activity monitors during a 1-mile walking field test. J Exerc Physiol Online. 2004;7:102–10.
Ham SA, Reis JP, Strath SJ, Dubose KD, Ainsworth BE. Discrepancies between methods of identifying objectively determined physical activity. Med Sci Sports Exerc. 2007;39:52–8.
Harrington DM, Welk GJ, Donnelly AE. Validation of MET estimates and step measurement using the ActivPAL physical activity logger. J Sports Sci. 2011;29:627–33.
Haymes EM, Byrnes WC. Walking and running energy expenditure estimated by Caltrac and indirect calorimetry. Med Sci Sports Exerc. 1993;25:1365–9.
vanHees VT, Lummel RC, Westerterp KR. Estimating Activity‐related Energy Expenditure Under Sedentary Conditions Using a Tri‐axial Seismic Accelerometer. Obesity. 2009;17:1287–92.
Hendelman D, Miller K, Baggett C, Debold E, Freedson P. Validity of accelerometry for the assessment of moderate intensity physical activity in the field. Med Sci Sports Exerc. 2000;32:S442–9.
Herman Hansen B, Børtnes I, Hildebrand M, Holme I, Kolle E, Anderssen SA. Validity of the ActiGraph GT1M during walking and cycling. J Sports Sci. 2014;32:510–6.
Howe CA, Staudenmayer JW, Freedson PS. Accelerometer prediction of energy expenditure: vector magnitude versus vertical axis. Med Sci Sports Exerc. 2009;41:2199–206.
Hustvedt B-E, Christophersen A, Johnsen LR, Tomten H, McNeill G, Haggarty P, Løvø A. Description and validation of the ActiReg®: a novel instrument to measure physical activity and energy expenditure. Br J Nutr. 2004;92:1001–8.
Jakicic JM, Marcus M, Gallagher KI, Randall C, Thomas E, Goss FL, Robertson RJ. Evaluation of the SenseWear Pro Armband to assess energy expenditure during exercise. Med Sci Sports Exerc. 2004;36:897–904.
Jakicic JM, Winters C, Lagally K, Ho J, Robertson RJ, Wing RR. The accuracy of the TriTrac-R3D accelerometer to estimate energy expenditure. Med Sci Sports Exerc. 1999;31:747–54.
Johansson HP, Rossander-Hulthén L, Slinde F, Ekblom B. Accelerometry combined with heart rate telemetry in the assessment of total energy expenditure. Br J Nutr. 2006;95:631–9.
John D, Tyo B, Bassett DR. Comparison of four ActiGraph accelerometers during walking and running. Med Sci Sports Exerc. 2010;42:368–74.
Kane N, Simmons M, John D, Thompson D, Bassett D, Basset D. Validity of the Nike+ device during walking and running. Int J Sports Med. 2010;31:101–5.
King GA, Torres N, Potter C, Brooks TJ, Coleman KJ. Comparison of activity monitors to estimate energy cost of treadmill exercise. Med Sci Sports Exerc. 2004;36:1244–51.
Kuffel EE, Crouter SE, Haas JD, Frongillo EA, Bassett DR Jr. Validity of estimating minute-by-minute energy expenditure of continuous walking bouts by accelerometry. Int J Behav Nutr Phys Act. 2011;8:92.
Leenders NY, Nelson TE, Sherman WM. Ability of different physical activity monitors to detect movement during treadmill walking. Int J Sports Med. 2003;24:43–50.
Levine J, Melanson EL, Westerterp KR, Hill JO. Measurement of the components of nonexercise activity thermogenesis. Am J Physiol Endocrinol Metab. 2001;281:E670–5.
Levine JA. An accelerometer-based earpiece to monitor and quantify physical activity. J Phys Act Health. 2009;6:781–9.
Lyden K, Kozey SL, Staudenmeyer JW, Freedson PS. A comprehensive evaluation of commonly used accelerometer energy expenditure and MET prediction equations. Eur J Appl Physiol. 2011;111:187–201.
Maliszewski AF, Freedson PS, Ebbeling CJ, Crussemeyer J, Kastango KB. Validity of the Caltrac accelerometer in estimating energy expenditure and activity in children and adults. Pediatr Exerc Sci. 1991;3:141–51.
Matthews CE, Freedson PS, Hebert JR, Stanek E 3rd, Merriam PA, Ockene IS. Comparing physical activity assessment methods in the Seasonal Variation of Blood Cholesterol Study. Med Sci Sports Exerc. 2000;32:976–84.
Melanson EL Jr, Freedson PS. Validity of the Computer Science and Applications, Inc.(CSA) activity monitor. Med Sci Sports Exerc. 1995;27:934–40.
Midorikawa T, Tanaka S, Kaneko K, Koizumi K, Ishikawa‐Takata K, Futami J, Tabata I. Evaluation of Low‐Intensity Physical Activity by Triaxial Accelerometry. Obesity. 2007;15:3031–8.
Miller NE, Strath SJ, Swartz AM, Cashin SE. Estimating absolute and relative physical activity intensity across age via accelerometry in adults. J Aging Phys Act. 2010;18:158.
Nichols JF, Morgan CG, Sarkin JA, Sallis JF, Calfas KJ. Validity, reliability, and calibration of the Tritrac accelerometer as a measure of physical activity. Med Sci Sports Exerc. 1999;31:908–12.
Ohkawara K, Oshima Y, Hikihara Y, Ishikawa-Takata K, Tabata I, Tanaka S. Real-time estimation of daily physical activity intensity by a triaxial accelerometer and a gravity-removal classification algorithm. Br J Nutr. 2011;105:1681–91.
Pambianco G, Wing RR, Robertson R. Accuracy and reliability of the Caltrac accelerometer for estimating energy expenditure. Med Sci Sports Exerc. 1990;22:858–62.
Patterson SM, Krantz DS, Montgomery LC, Deuster PA, Hedges SM, Nebel LE. Automated physical activity monitoring: Validation and comparison with physiological and self‐report measures. Psychophysiology. 1993;30:296–305.
Paul DR, Kramer M, Moshfegh AJ, Baer DJ, Rumpler WV. Comparison of two different physical activity monitors. BMC Med Res Methodol. 2007;7:26.
Pober DM, Staudenmayer J, Raphael C, Freedson PS. Development of novel techniques to classify physical activity mode using accelerometers. Med Sci Sports Exerc. 2006;38:1626–34.
Pomeroy J, Brage S, Curtis JM, Swan PD, Knowler WC, Franks PW. Between-monitor differences in step counts are related to body size: implications for objective physical activity measurement. PLoS One. 2011;6:e18942.
Ried-Larsen M, Brønd JC, Brage S, Hansen BH, Grydeland M, Andersen LB, Møller NC. Mechanical and free living comparisons of four generations of the Actigraph activity monitor. Int J Behav Nutr Phys Act. 2012;9:113.
Rothney MP, Neumann M, Beziat A, Chen KY. An artificial neural network model of energy expenditure using nonintegrated acceleration signals. J Appl Physiol. 2007;103:1419–27.
Rothney MP, Schaefer EV, Neumann MM, Choi L, Chen KY. Validity of physical activity intensity predictions by ActiGraph, Actical, and RT3 accelerometers. Obesity. 2008;16:1946–52.
Rowlands AV, Thomas P, Eston RG, Topping R. Validation of the RT3 triaxial accelerometer for the assessment of physical activity. Med Sci Sports Exerc. 2004;36:518–24.
Sherman W, Morris D, Kirby T, Petosa R, Smith B, Frid D, Leenders N. Evaluation of a commercial accelerometer (Tritrac-R3 D) to measure energy expenditure during ambulation. Int J Sports Med. 1998;19:43–7.
Slootmaker S, Paw CA, Schuit A, Van Mechelen W, Koppes L. Concurrent validity of the PAM accelerometer relative to the MTI Actigraph using oxygen consumption as a reference. Scand J Med Sci Sports. 2009;19:36–43.
Spierer DK, Hagins M, Rundle A, Pappas E. A comparison of energy expenditure estimates from the Actiheart and Actical physical activity monitors during low intensity activities, walking, and jogging. Eur J Appl Physiol. 2011;111:659–67.
Stone MR, Esliger DW, Tremblay MS. Comparative validity assessment of five activity monitors: Does being a child matter? Pediatr Exerc Sci. 2007;19:291–309.
Strath SJ, Bassett DR Jr, Swartz A, Thompson DL. Simultaneous heart rate-motion sensor technique to estimate energy expenditure. Med Sci Sports Exerc. 2001;33:2118–23.
Swan PD, Byrnes WC, Haymes EM. Energy expenditure estimates of the Caltrac accelerometer for running, race walking, and stepping. Br J Sports Med. 1997;31:235–9.
Swartz AM, Strath SJ, Bassett DR Jr, O'Brien WL, King GA, Ainsworth BE. Estimation of energy expenditure using CSA accelerometers at hip and wrist sites. Med Sci Sports Exerc. 2000;32:S450–6.
Terrier P, Aminian K, Schutz Y. Can accelerometry accurately predict the energy cost of uphill/downhill walking? Ergonomics. 2001;44:48–62.
Thompson D, Batterham AM, Bock S, Robson C, Stokes K. Assessment of low-to-moderate intensity physical activity thermogenesis in young adults using synchronized heart rate and accelerometry with branched-equation modeling. The Journal of Nutrition. 2006;136:1037–42.
Wanner M, Martin BW, Meier F, Probst-Hensch N, Kriemler S. Effects of filter choice in GT3X accelerometer assessments of free-living activity. Med Sci Sports Exerc. 2013;45:170–7.
Welk GJ, Almeida J, Morss G. Laboratory calibration and validation of the Biotrainer and Actitrac activity monitors. Med Sci Sports Exerc. 2003;35:1057–64.
Welk GJ, Blair SN, Wood K, Jones S, Thompson RW. A comparative evaluation of three accelerometry-based physical activity monitors. Med Sci Sports Exerc. 2000;32:S489–97.
Welk GJ, McClain JJ, Eisenmann JC, Wickel EE. Field validation of the MTI Actigraph and BodyMedia armband monitor using the IDEEA monitor. Obesity. 2007;15:918–28.
Yngve A, Nilsson A, Sjostrom M, Ekelund U. Effect of monitor placement and of activity setting on the MTI accelerometer output. Med Sci Sports Exerc. 2003;35:320–6.
Brage S, Brage N, Ekelund U, Luan J, Franks PW, Froberg K, Wareham NJ. Effect of combined movement and heart rate monitor placement on physical activity estimates during treadmill locomotion and free-living. Eur J Appl Physiol. 2006;96:517–24.
Horner FE, Rayson M, Bilzon JL. Reliability and validity of the 3DNX accelerometer during mechanical and human treadmill exercise testing. Int J Obes. 2011;35:S88–97.
Kumahara H, Tanaka H, Schutz Y. Daily physical activity assessment: what is the importance of upper limb movements vs whole body movements? Int J Obes. 2004;28:1105–10.
McClain JJ, Sisson SB, Tudor-Locke C. Actigraph accelerometer interinstrument reliability during free-living in adults. Med Sci Sports Exerc. 2007;39:1509–14.
Meijer GA, Westerterp KR, Verhoeven FM, Koper HB, ten Hoor F. Methods to assess physical activity with special reference to motion sensors and accelerometers. IEEE Transactions on Biomedical Engineering. 1991;38:221–9.
Nichols JF, Morgan CG, Chabot LE, Sallis JF, Calfas KJ. Assessment of physical activity with the Computer Science and Applications, Inc., accelerometer: laboratory versus field validation. Res Q Exerc Sport. 2000;71:36–43.
Powell SM, Rowlands AV. Intermonitor variability of the RT3 accelerometer during typical physical activities. Med Sci Sports Exerc. 2004;36:324–30.
Reneman M, Helmus M. Interinstrument reliability of the RT3 accelerometer. Int J Rehabil Res. 2010;33:178–9.
Vanhelst J, Baquet G, Gottrand F, Béghin L. Comparative interinstrument reliability of uniaxial and triaxial accelerometers in free-living conditions 1, 2. Percept Mot Skills. 2012;114:584–94.
Brandes M, Rosenbaum D. Correlations between the step activity monitor and the DynaPort ADL-monitor. Clinical Biomechanics. 2004;19:91–4.
Dahlgren G, Carlsson D, Moorhead A, Häger-Ross C, McDonough SM. Test–retest reliability of step counts with the ActivPAL™ device in common daily activities. Gait Posture. 2010;32:386–90.
Gao KL, Tsang WW. Use of accelerometry to quantify the physical activity level of the elderly. H K Physio J. 2008;26:18–23.
Harris TJ, Owen CG, Victor CR, Adams R, Ekelund U, Cook DG. A comparison of questionnaire, accelerometer, and pedometer: measures in older people. Med Sci Sports Exerc. 2009;41:1392–402.
Hart TL, Swartz AM, Cashin SE, Strath SJ. How many days of monitoring predict physical activity and sedentary behaviour in older adults. Int J Behav Nutr Phys Act. 2011;16:62.
Matthews CE, Ainsworth BE, Thompson RW, Bassett DR Jr. Sources of variance in daily physical activity levels as measured by an accelerometer. Med Sci Sports Exerc. 2002;34:1376–81.
Montoye HJ, Washburn R, Servais S, Ertl A, Webster JG, Nagle FJ. Estimation of energy expenditure by a portable accelerometer. Med Sci Sports Exerc. 1983;15:403–7.
Nichols J, Patterson P, Early T. A validation of a physical activity monitor for young and older adults. Can J Sport Sci. 1992;17:299–303.
Rowe DA, Kemble CD, Robinson TS, Mahar MT. Daily walking in older adults: day-to-day variability and criterion-referenced validity of total daily step counts. J Phys Act Health. 2007;4:435–47.
Sirard JR, Forsyth A, Michael Oakes J, Schmitz KH. Accelerometer test-retest reliability by data processing algorithms: results from the Twin Cities Walking Study. J Phys Act Health. 2011;8:668–74.
Sugimoto A, Hara Y, Findley T, Yoncmoto K. A useful method for measuring daily physical activity by a three-direction monitor. Scand J Rehabil Med. 1997;29:37–42.
Welk GJ, Schaben JA, Morrow JR Jr. Reliability of accelerometry-based activity monitors: a generalizability study. Med Sci Sports Exerc. 2004;36:1637–45.
Baranowski T, Masse LC, Ragan B, Welk G. How many days was that? We’re still not sure, but we’re asking the question better! Med Sci Sports Exerc. 2008;40:S544.
Ayabe M, Ishii K, Takayama K, Aoki J, Tanaka H. Comparison of interdevice measurement difference of pedometers in younger and older adults. Br J Sports Med. 2010;44:95–9.
Bergman RJ, Bassett D, Klein DA. Validity of 2 devices for measuring steps taken by older adults in assisted-living facilities. J Phys Act Health. 2008;5:S166–75.
Clemes SA, O'Connell S, Rogan LM, Griffiths PL. Evaluation of a commercially available pedometer used to promote physical activity as part of a national programme. Br J Sports Med. 2010;44:1178–83.
Crouter SE, Schneider PL, Bassett DR Jr. Spring-levered versus piezo-electric pedometer accuracy in overweight and obese adults. Med Sci Sports Exerc. 2005;37:1673–9.
Cyarto EV, Myers A, Tudor-Locke C. Pedometer accuracy in nursing home and community-dwelling older adults. Med Sci Sports Exerc. 2004;36:205–9.
Foster RC, Lanningham-Foster LM, Manohar C, McCrady SK, Nysse LJ, Kaufman KR, Padgett DJ, Levine JA. Precision and accuracy of an ankle-worn accelerometer-based pedometer in step counting and energy expenditure. Prev Med. 2005;41:778–83.
Hasson R, Haller J, Pober D, Staudenmayer J, Freedson P. Validity of the Omron HJ-112 pedometer during treadmill walking. Med Sci Sports Exerc. 2009;41:805–9.
Karabulut M, Crouter SE, Bassett DR Jr. Comparison of two waist-mounted and two ankle-mounted electronic pedometers. Eur J Appl Physiol. 2005;95:335–43.
Melanson EL, Knoll JR, Bell ML, Donahoo WT, Hill J, Nysse LJ, Lanningham-Foster L, Peters JC, Levine JA. Commercially available pedometers: considerations for accurate step counting. Prev Med. 2004;39:361–8.
Park J, Ishikawa-Takata K, Tanaka S, Mekata Y, Tabata I. Effects of walking speed and step frequency on estimation of physical activity using accelerometers. J Physiol Anthropol. 2011;30:119–27.
Shepherd EF, Toloza E, McClung CD, Schmalzried TP. Step activity monitor: increased accuracy in quantifying ambulatory activity. J Orthop Res. 1999;17:703–8.
Swartz A, Bassett D Jr, Moore J, Thompson D, Strath S. Effects of body mass index on the accuracy of an electronic pedometer. Int J Sports Med. 2003;24:588–92.
Tudor-Locke C, Sisson SB, Lee SM, Craig CL, Plotnikoff RC, Bauman A. Evaluation of quality of commercial pedometers. Can J Public Health. 2006;97:S10–5.
Welk GJ, Differding JA, Thompson RW, Blair SN, Dziura J, Hart P. The utility of the Digi-walker step counter to assess daily physical activity patterns. Med Sci Sports Exerc. 2000;32:S481–8.
Schneider PL, Crouter SE, Lukajic O, Bassett DR. Accuracy and reliability of 10 pedometers for measuring steps over a 400-m walk. Med Sci Sports Exerc. 2003;35:1779–84.
Holbrook E, Barreira T, Kang M. Validity and reliability of Omron pedometers for prescribed and self-paced walking. Med Sci Sports Exerc. 2009;41:669–3.
Abel MG, Hannon JC, Eisenman PA, Ransdell LB, Pett M, Williams DP. Waist circumference, pedometer placement, and step-counting accuracy in youth. Res Q Exerc Sport. 2009;80:434–44.
Crouter SE, Schneider PL, Karabulut M, Bassett DR Jr. Validity of 10 electronic pedometers for measuring steps, distance, and energy cost. Med Sci Sports Exerc. 2003;35:1455–60.
Resnick B, Nahm E-S, Orwig D, Zimmerman SS, Magaziner J. Measurement of activity in older adults: reliability and validity of the Step Activity Monitor. J Nurs Meas. 2001;9:275–90.
Bassett DR Jr, Ainsworth BE, Leggett SR, Mathien CA, Main JA, Hunter DC, Duncan GE. Accuracy of five electronic pedometers for measuring distance walked. Med Sci Sports Exerc. 1996;28:1071–7.
McClain JJ, Hart TL, Getz RS, Tudor-Locke C. Convergent validity of 3 low cost motion sensors with the ActiGraph accelerometer. J Phys Act Health. 2010;7:662–70.
Tharion WJ, Yokota M, Buller MJ, DeLany JP, Hoyt RW. Total energy expenditure estimated using a foot-contact pedometer. Am J Case Rep. 2004;10:504–9.
Hoyt RW, Buller MJ, Santee WR, Yokota M, Weyand PG, Delany JP. Total energy expenditure estimated using foot-ground contact pedometry. Diabetes Technology and Therapeutics. 2004;6:71–81.
Barreira TV, Tudor-Locke C, Champagne CM, Broyles ST, Johnson WD, Katzmarzyk PT. Comparison of GT3X accelerometer and YAMAX pedometer steps/day in a free-living sample of overweight and obese adults. J Phys Act Health. 2013;10:263–70.
Behrens TK, Dinger MK. Comparisons of accelerometer and pedometer determined steps in free living samples. J Phys Act Health. 2011;8:390.
McClain JJ, Craig CL, Sisson SB, Tudor-Locke C. Comparison of Lifecorder EX and ActiGraph accelerometers under free-living conditions. Appl Physiol Nutr Metab. 2007;32:753–61.
Schneider PL, Crouter SE, Bassett DR. Pedometer measures of free-living physical activity: comparison of 13 models. Med Sci Sports Exerc. 2004;36:331–5.
Tudor-Locke C, Ainsworth BE, Thompson RW, Matthews CE. Comparison of pedometer and accelerometer measures of free-living physical activity. Med Sci Sports Exerc. 2002;34:2045–51.
Felton GM, Tudor-Locke C, Burkett L. Reliability of pedometer-determined free-living physical activity data in college women. Res Q Exerc Sport. 2006;77:304–8.
Strycker LA, Duncan SC, Chaumeton NR, Duncan TE, Toobert DJ. Reliability of pedometer data in samples of youth and older women. Int J Behav Nutr Phys Act. 2007;4:4.
Kang M, Bassett DR, Barreira TV, Tudor-Locke C, Ainsworth B, Reis JP, Strath S, Swartz A. How many days are enough? A study of 365 days of pedometer monitoring. Res Q Exerc Sport. 2009;80:445–53.
Tudor-Locke C, Burkett L, Reis J, Ainsworth B, Macera C, Wilson D. How many days of pedometer monitoring predict weekly physical activity in adults? Prev Med. 2005;40:293–8.
Tudor-Locke C. A preliminary study to determine instrument responsiveness to change with a walking program: physical activity logs versus pedometers. Res Q Exerc Sport. 2001;72:288–92.
Kashiwazaki H. Heart rate monitoring as a field method for estimating energy expenditure as evaluated by the doubly labeled water method. J Nutr Sci Vitaminol (Tokyo). 1999;45:79–94.
Livingstone M, Prentice AM, Coward W, Ceesay SM, Strain JJ, McKenna PG, Nevin GB, Barker ME, Hickey R. Simultaneous measurement of free-living energy expenditure by the doubly labeled water method and heart-rate monitoring. Am J Clin Nutr. 1990;52:59–65.
Ceesay SM, Prentice AM, Day KC, Murgatroyd PR, Goldberg GR, Scott W, Spurr G. The use of heart rate monitoring in the estimation of energy expenditure: a validation study using indirect whole-body calorimetry. Br J Nutr. 1989;61:175–86.
Leonard WR, Katzmarzyk PT, Stephen MA, Ross A. Comparison of the heart rate-monitoring and factorial methods: assessment of energy expenditure in highland and coastal Ecuadoreans. Am J Clin Nutr. 1995;61:1146–52.
Spurr G, Prentice A, Murgatroyd P, Goldberg G, Reina J, Christman N. Energy expenditure from minute-by-minute heart-rate recording: comparison with indirect calorimetry. Am J Clin Nutr. 1988;48:552–9.
Strath SJ, Swartz AM, Bassett DR Jr, O'Brien WL, King GA, Ainsworth BE. Evaluation of heart rate as a method for assessing moderate intensity physical activity. Med Sci Sports Exerc. 2000;32:S465–70.
Foerster F, Fahrenberg J. Motion pattern and posture: correctly assessed by calibrated accelerometers. Behav Res Methods Instrum Comput. 2000;32:450–7.
Foerster F, Smeja M, Fahrenberg J. Detection of posture and motion by accelerometry: a validation study in ambulatory monitoring. Comput Human Behav. 1999;15:571–83.
Nyan M, Tay F, Seah K, Sitoh Y. Classification of gait patterns in the time–frequency domain. J Biomech. 2006;39:2647–56.
Parkka J, Ermes M, Korpipaa P, Mantyjarvi J, Peltola J, Korhonen I. Activity classification using realistic data from wearable sensors. IEEE Trans Inf Technol Biomed. 2006;10:119–28.
Lau H-Y, Tong K-Y, Zhu H. Support vector machine for classification of walking conditions using miniature kinematic sensors. Med Biol Eng Comput. 2008;46:563–73.
Ng J, Sahakian AV, Swiryn S. Accelerometer-based body-position sensing for ambulatory electrocardiographic monitoring. Biomed Instrum Technol. 2003;37:338–46.
Rumo M, Amft O, Tröster G, Mäder U. A stepwise validation of a wearable system for estimating energy expenditure in field-based research. Physiol Meas. 2011;32:1983–2001.
Brage S, Brage N, Franks PW, Ekelund U, Wong M-Y, Andersen LB, Froberg K, Wareham NJ. Branched equation modeling of simultaneous accelerometry and heart rate monitoring improves estimate of directly measured physical activity energy expenditure. J Appl Physiol. 2004;96:343–51.
Moon JK, Butte NF. Combined heart rate and activity improve estimates of oxygen consumption and carbon dioxide production rates. J Appl Physiol. 1996;81:1754–61.
Rennie K, Rowsell T, Jebb S, Holburn D, Wareham N. A combined heart rate and movement sensor: proof of concept and preliminary testing study. Eur J Clin Nutr. 2000;54:409–14.
Edwards AG, Hill JO, Byrnes WC, Browning RC. Accuracy of optimized branched algorithms to assess activity-specific PAEE. Med Sci Sports Exerc. 2010;42:672.
Haskell WL, Yee MC, Evans A, Irby PJ. Simultaneous measurement of heart rate and body motion to quantitate physical activity. Med Sci Sports Exerc. 1993;25:109–15.
Luke A, Maki KC, Barkey N, Cooper R, McGEE D. Simultaneous monitoring of heart rate and motion to assess energy expenditure. Med Sci Sports Exerc. 1997;29:144–8.
Meijer GA, Westerterp KR, Koper H, ten Hoor F. Assessment of energy expenditure by recording heart rate and body acceleration. Med Sci Sports Exerc. 1989;21:343–7.
Strath SJ, Bassett DR Jr, Thompson DL, Swartz AM. Validity of the simultaneous heart rate-motion sensor technique for measuring energy expenditure. Med Sci Sports Exerc. 2002;34:888–94.
Strath SJ, Brage S, Ekelund U. Integration of physiological and accelerometer data to improve physical activity assessment. Med Sci Sports Exerc. 2005;37:S563–71.
Download references
Acknowledgements
The authors would like to acknowledge the contribution of all members of the DEDIPAC Knowledge Hub consortium for their help and assistance in the completion of this work.
The preparation of this paper was supported by the DEterminants of DIet and Physical ACtivity (DEDIPAC) knowledge hub. This work is supported by the Joint Programming Initiative ‘Healthy Diet for a Healthy Life’. This work was funded by the Health Research Board (HRB) of Ireland. Additional funding agencies supporting this work were (in alphabetical order of collaborative Member State): Germany: Project Management Agency in the German Aerospace Centre (PT-DLR); Italy: Ministry of Education, University and Research/ Ministry of Agriculture Food and Forestry Policies; The Netherlands: The Netherlands Organisation for Health Research and Development (ZonMw); Norway: The Research Council of Norway, Division for Society and Health; Poland: The National Centre for Research and Development; The United Kingdom: The Medical Research Council (MRC).
Availability of data and materials
All data examined during this study are included in this published article [and its supplementary materials].
Author contributions
HvdP, UE and AD were involved in the conception of the review title. KD, RSz, SC, AP, HvdP, UE and AD contributed to the design of the review protocol. KD conducted the database search. Several reviewers independently performed the selection of articles (KD, RSz, JM, RSt, MAM and MT) and examined the titles and abstracts of the identified references to exclude articles out of scope. Any disagreements on study inclusions were resolved through discussions with another reviewer (AD) and a consensus reached. KD, RSz JM, RSt, MAM and MT assessed the eligible papers, extracted the data, and discussed the findings. KD, RSz, MAM, MM, AP, HvdP, UE and AD drafted the paper and all authors listed reviewed the manuscript and contributed to subsequent drafts. All authors read and approved the final document.
Author information
Authors and affiliations.
Department of Sport and Health Science, Athlone Institute of Technology, Athlone, Ireland
Kieran P. Dowd
University School of Physical Education in Poznan, Poznan, Poland
Robert Szeklicki, Janusz Maciaszek, Rafal Stemplewski & Maciej Tomczak
Division of Endocrinology, Diabetology and Metabolism, Department of Internal Medicine, University of Turin, Corso Dogliotti 14, 10126, Torino, Italy
Marco Alessandro Minetto & Ezio Ghigo
School of Health Science, University of Ulster, Newtownabbey, UK
Marie H. Murphy
National Institute for Food and Nutrition Research, Rome, Italy
Angela Polito
Department of Public and Occupational Health, VU University Medical Center, EMGO Institute for Health and Care Research, Amsterdam, The Netherlands
Hidde van der Ploeg
Sydney School of Public Health, University of Sydney, Sydney, Australia
Medical Research Council (MRC) Epidemiology Unit, University of Cambridge, Cambridge, UK
Ulf Ekelund
The Department of Sport Medicine, Norwegian School of Sport Sciences, Oslo, Norway
Department of Physical Education and Sport Sciences, Health Research Institute, University of Limerick, Limerick, Ireland
Alan E. Donnelly
You can also search for this author in PubMed Google Scholar
Corresponding author
Correspondence to Alan E. Donnelly .
Ethics declarations
Ethics approval and consent to participate.
The paper is a literature review on methods, and only employs published research data. There were no human participants, and our University ethics committee does not approve non-human based research.
Consent for publication
Not applicable.
Competing interests
The authors declare that they have no competing interests.
Publisher’s Note
Springer Nature remains neutral with regard to jurisdictional claims in published maps and institutional affiliations.
Additional file
Additional file 1: table s1..
Criterion validity of self-reported measures of physical activity/energy expenditure. Table S2 . Concurrent validity of self-reported measures of physical activity/energy expenditure. Table S3 . Intra-instrument Reliability of self-reported measures of physical activity. Table S4a . Test-retest reliability of self-reported measures of physical activity/energy expenditure within a duration of less than or equal to one week. Table S4b . Test-retest reliability of self-reported measures of physical activity/energy expenditure within a duration of between 1 week and 4 weeks. Table S4c . Test-retest reliability of self-reported measures of physical activity/energy expenditure within a duration of between 4 weeks and 8 weeks. Table S4d . Test-retest reliability of self-reported measures of physical activity/energy expenditure within a duration of between 8 weeks and 1 year. Table S4e . Test-retest reliability of self-reported measures of physical activity/energy expenditure within a duration of greater than 1 year. Table S5 . Sensitivity to change over time of self-reported measures of physical activity/energy expenditure. Table S6 . Criterion validity of accelerometer activity monitor determined physical activity/energy expenditure. Table S7 . Concurrent validity of accelerometer/activity monitor determined physical activity/energy expenditure. Table S8 . Inter-instrument reliability of accelerometer/activity monitor determined physical activity/energy expenditure. Table S9 . Test-retest reliability of accelerometer/activity monitor determined physical activity/energy expenditure. Table S10 . Sensitivity to change over time of accelerometer devices. Table S11 . Details of studies that examined the Criterion Validity of Pedometers. Table S12 . Details of studies examining the concurrent validity of pedometers. Table S13 . Details of studies examining inter-instrument reliability in pedometer devices. Table S14 . Details of studies examining the test-retest reliability of pedometers. Table S15 . Details of studies examining the sensitivity to change of pedometers. Table S16 . Details of studies examining the criterion validity of heart rate monitoring devices. Table S17 . Details of studies examining the concurrent validity of heart rate monitoring devices. Table S18 . Details of studies examining the criterion validity of combined sensors. Table S19 . Details of studies examining the concurrent validity of combined sensors. Figure S1 . PRISMA Checklist. Figure S2a . Forest plot of percentage mean difference between self-report measures of energy expenditure compared to energy expenditure from activity logs/diaries. Figure S2b . Forest plot of percentage mean difference between self-report measures of energy expenditure and time spent in physical activity compared to other self-report measures of energy expenditure or time spent in physical activity. Figure S2c . Forest plot of percentage mean difference between self-report measures of energy expenditure compared to energy expenditure determined from activity monitors. Figure S2d . Forest plot of percentage mean difference between self-report measures of time spent in physical activity intensities (Light, Moderate and Moderate-to-Vigorous intensity physical activity) compared to time spent in physical activity intensities determined from activity monitors. Figure S2e . Forest plot of percentage mean difference between self-report measures of time spent in physical activity intensities (Vigorous physical activity, Total physical activity, times active, time standing, time stepping) compared to time spent in physical activity intensities determined from activity monitors. Figure S2f . Forest plot of percentage mean difference between self-report measures of energy expenditure and time spent in physical activity intensities (Vigorous physical activity, Total physical activity, times active, time standing, time stepping) compared to energy expenditure time spent in physical activity intensities determined from pedometers and heart rate monitors. Figure S3c . Forest plot of percentage mean difference between accelerometer/activity monitor determined variables (activity counts, time spent in light intensity physical activity, time spent in moderate intensity physical activity, time spent in moderate-to-vigorous intensity physical activity, time spent in vigorous intensity physical activity, total physical activity, stepping and energy expenditure) compared to an alternative accelerometer/activity monitor. Figure S3d . Forest plot of percentage mean difference between accelerometer/activity monitor determined energy expenditure (METs) in light intensity physical activity, moderate intensity physical activity, vigorous intensity physical activity and total physical activity (METs, MJ.d, KJ.h, KJ.kg.min -1 ) compared to estimates from indirect (IC) and whole room calorimetry (WRC). Figure S3e . Forest plot of percentage mean difference between accelerometer/activity monitor determined energy expenditure, energy expenditure from light intensity physical activity, moderate intensity physical activity, vigorous intensity physical activity, total physical activity compared to estimates from Heart Rate Monitoring (HRM) and Self-Report (SR) measures. Figure S3f . Forest plot of percentage mean difference between accelerometer/activity monitor determined energy expenditure (kcal.min -1 , kcal.kg.hr -1 ) compared to indirect calorimetry determined energy expenditure (kcal.min -1 , kcal.kg.hr -1 ). Figure S3g . Forest plot of percentage mean difference between accelerometer/activity monitor determined energy expenditure (METs.min -1 , METs.hr -1 ) compared to indirect calorimetry determined energy expenditure (METs.min -1 , METs.hr -1 ). Figure S3h . Forest plot of percentage mean difference between accelerometer/activity monitor determined total energy expenditure compared to indirect calorimetry determined total energy expenditure. Figure S3h (cont). Forest plot of percentage mean difference between accelerometer/activity monitor determined energy expenditure (kcal.min -1 , kcal.kg.hr -1 ) compared to indirect calorimetry determined energy expenditure (kcal.min -1 , kcal.kg.hr -1 ). Figure S4a . Forest plot of percentage mean difference between pedometer determined step count/energy expenditure/MVPA compared to concurrent measures (i.e. accelerometry, indirect calorimetry, pedometers). Figure S5a . Forest plot of percentage mean difference between heart rate monitor determined energy expenditure/physical activity level/physical activity intensity compared to concurrent measures (accelerometers, self-report, indirect calorimetry) Figure S6 . Forest plot of percentage mean difference between energy expenditure determined by combined sensors compared to concurrent measure (indirect calorimetry). (DOCX 1304 kb)
Rights and permissions
Open Access This article is distributed under the terms of the Creative Commons Attribution 4.0 International License ( http://creativecommons.org/licenses/by/4.0/ ), which permits unrestricted use, distribution, and reproduction in any medium, provided you give appropriate credit to the original author(s) and the source, provide a link to the Creative Commons license, and indicate if changes were made. The Creative Commons Public Domain Dedication waiver ( http://creativecommons.org/publicdomain/zero/1.0/ ) applies to the data made available in this article, unless otherwise stated.
Reprints and permissions
About this article
Cite this article.
Dowd, K.P., Szeklicki, R., Minetto, M.A. et al. A systematic literature review of reviews on techniques for physical activity measurement in adults: a DEDIPAC study. Int J Behav Nutr Phys Act 15 , 15 (2018). https://doi.org/10.1186/s12966-017-0636-2
Download citation
Received : 28 June 2017
Accepted : 18 December 2017
Published : 08 February 2018
DOI : https://doi.org/10.1186/s12966-017-0636-2
Share this article
Anyone you share the following link with will be able to read this content:
Sorry, a shareable link is not currently available for this article.
Provided by the Springer Nature SharedIt content-sharing initiative
- Physical activity
- Measurement
- Accelerometry
- Questionnaires
- Self-report
- Motion sensors
International Journal of Behavioral Nutrition and Physical Activity
ISSN: 1479-5868
- Submission enquiries: Access here and click Contact Us
- General enquiries: [email protected]
- Open access
- Published: 01 February 2022
The association between physical activity and mental health during the first year of the COVID-19 pandemic: a systematic review
- Priscila Marconcin 1 , 2 ,
- André O. Werneck 3 ,
- Miguel Peralta 2 , 4 ,
- Andreas Ihle 5 , 6 , 7 ,
- Élvio R. Gouveia 8 , 9 ,
- Gerson Ferrari 10 ,
- Hugo Sarmento 11 &
- Adilson Marques 2 , 3
BMC Public Health volume 22 , Article number: 209 ( 2022 ) Cite this article
30k Accesses
105 Citations
153 Altmetric
Metrics details
Introduction
The Coronavirus disease-19 (COVID-19) pandemic affected countries worldwide and has changed peoples’ lives. A reduction in physical activity and increased mental health problems were observed, mainly in the first year of the COVID-19 pandemic. Thus, this systematic review aims to examine the association between physical activity and mental health during the first year of the COVID-19 pandemic.
In July 2021, a search was applied to PubMed, Scopus, and Web of Science. Eligibility criteria included cross-sectional, prospective, and longitudinal study designs and studies published in English; outcomes included physical activity and mental health (e.g., depressive symptoms, anxiety, positive and negative effects, well-being).
Thirty-one studies were included in this review. Overall, the studies suggested that higher physical activity is associated with higher well-being, quality of life as well as lower depressive symptoms, anxiety, and stress, independently of age. There was no consensus for the optimal physical activity level for mitigating negative mental symptoms, neither for the frequency nor for the type of physical activity. Women were more vulnerable to mental health changes and men were more susceptive to physical activity changes.
Physical activity has been a good and effective choice to mitigate the negative effects of the COVID-19 pandemic on mental health during the first year of the COVID-19 pandemic. Public health policies should alert for possibilities to increase physical activity during the stay-at-home order in many countries worldwide.
Peer Review reports
The severe acute respiratory syndrome coronavirus-2 (SARS-CoV-2) is a highly contagious virus that infects humans and causes coronavirus disease-19 (COVID-19), which is currently having a damaging impact on almost all countries worldwide [ 1 ]. To bring this pandemic to an end, a large share of the world needs to be immune to the virus, and the safest way to achieve this is with a vaccine. Fortunately, in December 2020 the vaccination started in the United Kingdom [ 2 ] and is currently pursued in different countries [ 3 ]. Until November 2021, 53.3% of the world population has received at least one dose of a COVID-19 vaccine [ 4 ]. However, the number of infected people and deaths continues to grow [ 5 ]. The World Health Organization (WHO) published a weekly report and on 16th of November 2021 it was observed a increasing trend in new global weekly cases [ 6 ]. From the beginning of the pandemic, as a community mitigation strategy used to reduce the spread of COVID-19, most countries adopted the stay-at-home order as well as the stimulation of facemask wearing and hygiene habits [ 7 , 8 ].
As a consequence of the stay-at-home strategies, mainly during the first year of the COVID-19 pandemic, studies had reported multiple behaviour changes. Some common impacts include disturbed eating behaviours [ 9 ], changes in alcohol consumption [ 10 ], and substance use [ 11 ]. A wide range of psychological outcomes has been observed during the virus outbreak, including a reduction in well-being as well as increases in depressive and anxiety symptoms [ 12 , 13 ]. Considering the need for social distancing measures, the investigation of possible factors that can mitigate the negative effects of social distancing on mental health should help the promotion of intervention strategies.
Physical activity (PA) is well recognised as a key factor for the prevention and management of mental illness, including mental disorders such as depression and anxiety as well as the promotion of mental health such as well-being [ 14 , 15 ]. Nevertheless, globally, approximately 23% of adults and 81% of adolescents do not meet the WHO recommendations regarding PA for maintaining health [ 16 , 17 , 18 ]. This situation even worsened with the COVID-19 pandemic. Studies have demonstrated that PA declined and sedentary behaviour increased during the COVID-19 pandemic stay at home order in many countries, regardless of the subpopulation [ 19 ]. Different studies sought to investigate whether these changes in PA were associated with mental health indicators during the COVID-19 pandemic and a previous systematic review synthetised that PA is an effective strategy to face the psychological effects of the COVID-19 pandemic [ 20 ]. However, the previous review included articles published between 1 January 2019, and 15 July 2020, before the second wave of the COVID-19 pandemic. Therefore, our systematic review aimed to update those findings and clarify if PA is associated with mental health during the first full year of the COVID-19 pandemic and to analyse if PA mitigates the effects of the stay-at-home order on mental health. We aimed to explore the first year of the COVID-19 pandemic because it was the period when restrictive orders were strictest when people were strongly encouraged to comply with the stay-at-home order.
This systematic review focuses on peer-reviewed journal articles on the relationship of PA to mental health during the COVID-19 pandemic published until 30 July 2021.
Data sources and searches
A systematic review protocol was registered with the PROSPERO database on the 29th of January 2021 (IDCRD42021233921). A broad search strategy was employed. Searches were conducted on the 30th of January 2021, in the following electronic databases: PubMed, Scopus, and Web of Science. The search was performed in the three databases using the terms: (physical activity OR physical inactivity OR exercise OR training OR sport* OR fitness OR physical function* OR movement behavio* OR sedentary behavio*) AND (mental health OR psychological health OR depress* OR anxiety OR psychological function* OR mental function* OR wellbeing OR well-being OR burnout OR burn-out OR fear OR fears OR worries OR worry) AND (coronavirus disease OR COVID-19 OR SARS-CoV-2 OR lockdown OR shutdown OR quarantine OR confinement OR social isolation). These terms were searched in title and abstract of scientific articles. Additionally, cross-referencing search was performed in the full-text read of potentially included articles.
Study selection
Observational studies (cross-sectional, prospective, or longitudinal) were eligible for this review. Furthermore, studies were also required to meet the following criteria: (1) assessing PA by a validated instrument, (2) assessing mental health by a validated instrument, (3) presenting an analysis on the association between PA and mental health. Studies with samples including pregnant women, chronic disease patients, athletes, COVID-19 survivors, and frail older adults were excluded. Besides, studies reporting PA as a moderate or mediated variable were also excluded. Two co-authors screened titles and abstracts to identify articles that met the inclusion criteria. Two co-authors read the articles and decided whether they should be included in the analysis or excluded. The inclusion decision was consensual and in cases of disagreement, the decision was made by mutual agreement.
Data extraction and synthesis of results
Data extraction was completed independently by one co-author. Data extracted from all studies included study details (author, year of publication, study design, recruitment processes, and date and location of the study); participant characteristics (sex, mean age); outcome and instruments, and main findings. A table was made for articles that analysed the association between PA and mental health among adults, and another table for the analyses of the association between PA and mental health among children and adolescents.
Quality assessment
The risk of bias was assessed by two independent reviewers, using the Newcastle-Ottawa Scale (NOS) [ 21 ] which was also adapted for cross-sectional studies [ 22 ]. Therefore, we used the original scale for cohort studies and the adapted scale for the cross-sectional studies. The original scale varies between 0 and 9, while the adapted scale for cross-sectional studies varies between 0 and 10, with higher scores indicating research of better quality.
Narrative synthesis
Considering the heterogeneity of methods used for the estimation of the exposures and outcomes, it was not possible to conduct a meta-analysis. Therefore, we compared the findings across the included articles according to each outcome.
Results of the search
From the database search, 734 records were identified. After removing duplicates, the titles and abstracts of 328 articles were screened concerning the eligibility criteria, and 205 were excluded. The full texts of the remaining 1237 articles were evaluated and 92 were excluded for the following reasons: sample characteristics ( n = 24), data were not analysed regarding the association between PA and mental health variables ( n = 23), review studies ( n = 4), no valid instruments to assess PA ( n = 31) and mental health ( n = 6), the study was not in the period of the COVID-19 pandemic ( n = 4). Thirty-one studies were included in this review, 27 about adults and old adults and 4 about children and adolescents. The flow diagram of study search and selection was created according to the Preferred Reporting Items for Systematic Reviews and Meta-Analyses (PRISMA) [ 23 ] and is presented in Fig. 1 . The mean score of quality was 5.7 ± 1.5. More details are presented in Tables 1 and 2 .
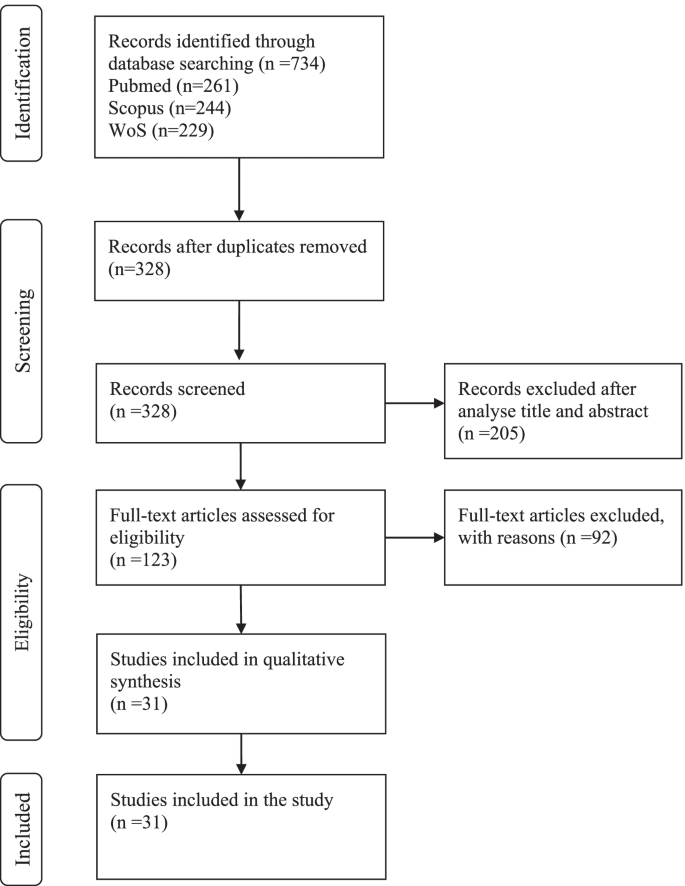
Flow diagram of study selection
The association between physical activity and mental health among adults and old adults
The details of the association between PA and mental health among adults and old adults are summarised in Table 1 , 27 studies were included [ 24 , 25 , 26 , 27 , 28 , 29 , 30 , 31 , 32 , 33 , 34 , 35 , 36 , 37 , 38 , 39 , 40 , 41 , 42 , 43 , 44 , 45 , 46 , 47 , 48 , 49 , 51 ].
Participants characteristics and date of filling the questionnaires
The number of participants in the 27 included studies varied between 66 [ 50 ] and 14,715 [ 40 ] participants. Regarding sex, with exception of four studies [ 24 , 31 , 40 , 48 ] the majority included more women than men. Concerning age, most articles presented the mean age range between 20 and 30 years [ 24 , 25 , 36 , 37 , 44 , 48 , 49 , 50 , 56 , 57 ], two article presented mean age between 30 and 40 years [ 33 , 43 ]; five articles presented mean age between 40 and 50 years [ 28 , 32 , 35 , 41 , 42 ]; and seven articles presented mean age above 50 years [ 26 , 27 , 34 , 45 , 46 , 47 , 51 ]. Four articles did not present mean age [ 31 , 38 , 39 , 40 ], for one article the age ranged between 21 and 35 years [ 31 ], for another, the age ranged between 21 and 40 years [ 38 ], for another, the age ranged between 17 and 69 years [ 40 ], and in one the age ranged between 27 and 53 years [ 39 ]. The majority of studies reported, the sample filled out online questionnaires. One study used interviewed by telephone call [ 34 ]. Twenty four studies conducted a cross-sectional analysis and four a longitudinal analysis.
Study location
The studies were carried out on five different continents. Ten studies from Europe [ 24 , 25 , 26 , 27 , 28 , 38 , 39 , 43 , 44 , 49 ], foure studies from Asia [ 36 , 40 , 46 , 48 , 50 ], seven studies from America [ 33 , 34 , 35 , 37 , 41 , 51 , 57 ] two studies from Africa [ 31 , 56 ], two study from Oceania [ 42 , 45 ], and two multi-centre study [ 32 , 47 ].
Outcomes and instruments
Concerning outcomes and instruments, 12 articles used the International Physical Activity Questionnaire (IPAQ) to assess PA and one article calculated an estimate of cardiorespiratory fitness (algorithm includes age, body composition, resting heart rate and PA) [ 57 ]. The others articles assessed PA with different instruments. Mental health included analyses of subjective well-being, sleep quality, depressive symptoms, anxiety, quality of life, psychological distress, motivation, resilience, affects (positive and negative), and health-related quality of life.
Main findings
Overall, all articles found a positive association between PA and better outcomes of mental health (e. g., depression, anxiety, well-being). Physical activity was explanatory variable for mental health [ 25 ]. Physical activity was positive associated with mental health [ 42 , 49 ]. Articles that observed a decrease in PA during the stay-at-home order also observed a decrease in well-being [ 47 , 56 ], negative changes in depressive symptoms [ 57 ], and negative changes in anxiety and stress symptoms [ 45 ]. This relationship seems to be bidirectional, since participants who decrease in mental health had greater reduction in physical activity [ 37 ]. Inactive people had worse well-being, highest depression and enxiety compared with moderately active and very active participants [ 24 , 51 ]. Also, inactive old adults had more depressive symptoms [ 26 ]. On the other hand, participants sufficiently active reported significantly lower depression and anxiety and higher life satisfaction. Moreover, it was found that exercise intensity seems to be important. Two studies founded that depression was significantly negatively correlated with moderate-intensity PA but not vigorous and walking/light exeeercuse [ 36 , 40 ]. Another one found that vigorous PA better predicted depressive symptoms than moderate PA [ 27 ]. Three studies suggested that the threshold of PA should be done to fells the benefits on mental health [ 28 , 39 , 50 ]. At least 4 h of MVPA reduced by 49% odds of depressive symptoms [ 28 ], and at leas 477 METs-min/week was associated with a 33% decrease in the probability of depressive symptoms [ 39 ]. On the other hand, a non-significant association was found between PA and anxiety [ 35 ], and between PA and health-related quality of life [ 46 ]. One study found that the decrease in mental wellbeing and increase in perceived stress was not related to changes in PA [ 44 ].
The association between physical activity and mental health among children and adolescents
The details of the association between PA and mental health among children and adolescents are shown in Table 2 , four studies were included [ 52 , 53 , 54 , 55 ].
The number of participants ranged between 64 and 4898 children and adolescents. More girls than boys participated in the studies. The mean ages were 11, 14, 15, and 16 years old. One study was longitudinal and presents two moments of assessment, also opting for phone or video calls to collect the data [ 52 ]. The other three studies were cross-sectional, and collected data by online surveys.
One study was from the USA [ 52 ], one from Greece [ 54 ] and the other two were from China [ 53 , 55 ].
Three studies assessed PA by the International physical activity questionnaire (IPAQ) questionnaire [ 53 , 54 , 55 ], and another one used a 24-h physical activity recall [ 52 ]. Regarding mental health, different outcomes were assessed, such as anxiety, positive and negative affect, insomnia, depressive symptoms, psychological well-being and mood states.
Moderate physical activity was associated with less state anxiety [ 52 , 53 ]. Positive affect was not related to physical activity [ 52 ]. Higher levels of physical activity were also significantly associated with lower levels of total mood disturbance [ 55 ]. Regarding the dose of physical activity, days of physical activity per week was stronger predictor of well-being than minutes of physical activity per week [ 54 ].
This systematic review focuses on the association between PA and mental health during the first year of the COVID-19 pandemic. In particular, we sought to answer if PA mitigates the effects of the stay-at-home order on mental health. The COVID-19 pandemic generated numerous challenges for public health, particularly the significant burden of mental health in the population [ 58 , 59 ]. In addition, PA has been recognized as an effective mitigation strategy for improving mental health [ 60 ]. The COVID-19 pandemic has been affecting all continents in the world, at different scales. This study analysed 31 research articles, 27 about adults and old adults and 4 about children and adolescents. The articles are mainly based on cross-sectional studies and five are longitudinal studies. In nearly all of the studies comprised in the present systematic review, investigators used online surveys as the main procedure to collect data. Overall, the studies suggested that higher PA is associated with less negative mental health symptoms, such as depression, anxiety, well-being, and fear, independently of age.
The studies observed that women showed more depressive symptoms than men [ 28 , 39 , 50 , 53 , 55 ], and this trend increases with age [ 24 ] Furthermore, individuals with a lower level of masculinity traits (not specifically females) increased risk of developing depression [ 36 ], and women experienced more generalised anxiety [ 41 ]. The reduction of PA levels may mostly influence the mental well-being of females [ 38 , 49 ]. Those findings are expected since the literature is consistent in signalising sex differences in most mental disorders [ 61 ]. On the whole, the prevalence rates of anxiety and depression were both higher than the rates found in previous studies before the COVID-19 pandemic [ 36 , 37 , 43 , 45 , 48 , 57 ], which highlights a worsening in mental health during the first year of the COVID-19 pandemic. Regarding age, younger individuals experienced significantly higher anxiety and depression, also income influenced mental health, lower-income participants present worse mental health [ 37 ].
Five articles conducted a longitudinal study. Among those studies, four enrolled adults and old adults [ 28 , 44 , 46 , 50 , 51 ] and in one the sample comprised children [ 52 ]. Among the studies that have collected measures before and after the stay-at-home order, both observed a significant reduction in PA [ 44 , 46 ]. Two study collected measures after the stay-at-home order and during this period physical activity mean score decreased minimally [ 51 ], and depressive symptoms increase as the weeks of isolation go [ 28 ]. Other studies also reported a reduction in self-reported PA [ 26 , 33 , 34 , 37 , 38 , 42 , 43 , 45 , 56 , 57 ] and increase in sedentary behaviour [ 25 , 26 , 34 ]. Individuals who reported larger decrease in MVPA pre to during COVID-19 reported relatively poorer mental health [ 33 ]. Participants whose mental health got “worse” or “much worse” had greater reductions in physical activity [ 37 ]. Also, increased levels of physical activity were associated with stronger effects on wellbeing [ 42 ]. The reduction was more pronounced in men than women [ 32 , 38 , 44 ], in vigorous PA [ 38 ], and between those with lower health-related quality of life scores before the COVID-19 pandemic [ 46 ]. The possible explanation for this sex-difference is that men are more engaged in group/community PA and sports in clubs or gyms, and those were more impacted by the COVID-19 restrictions. Also, women are more engaged in low and moderate physical activities, which can be done at home. Besides, women spent more time in housework activities. Women without changes in childcare provision reported more opportunities to be physically active [ 41 ]. The same sex-differences were observed in an Italian study [ 62 ]. Increases in PA were observed for a minority, but the majority of the respective study samples demonstrating a positive change were individuals who did not meet recommended PA guidelines before the COVID-19 pandemic [ 32 , 38 ]. Additional reasons could be an increase in awareness for health issues and more time to pursue PA during the stay-at-home order [ 32 ]. These behaviour changes can help to maintain a more active lifestyle during the pandemic. Another study found an increase of 40% in PA in a sample that was already active before the COVID-19 pandemic [ 35 ]. PA could be used as a coping strategy to deal with the consequences of the pandemic. The place where individuals prefer to practice PA seems to be important, since active participants reported greater connectedness to nature and nature relatedness than the inactive population [ 35 ].
There was no consensus across studies for the optimal PA levels for mitigating negative mental symptoms. The more the physical activity is frequent and vigorous, the best people feel themselves [ 24 ]. Among Chinese students, 2500 METs minute/week of PA every week was the optimal dose to alleviate negative emotion [ 50 ]. On the other hand, a Spanish community sample study showed that 477 METs-minute/week was associated with a 33% decrease in the probabilities of notable depressive symptoms [ 39 ]. The difference between the values must be relativised considering the samples’ characteristics. The first one concerns students with a mean age of 20 years [ 50 ], and the second one concerns a community sample with a mean age of 43.2 years for women and 40.5 years for men [ 39 ]. In addition, it is claimed that at least 3000 METs-minute/week reduce the odds of depressive symptoms by 47% [ 39 ]. These studies used the IPAQ to assess PA, and according to IPAQ, to reach a minimally active category at least 600 METs minute/week is needed [ 63 ]. The American College of Sports Medicine also recommends for healthy adults aged 18–65 years at least 600 METs minute/week but did not specify the minimum dose to prevent depressive symptoms [ 14 , 64 ].
Studies also examined the association between PA and mental health according to PA intensity. Moderate-intensity PA (e.g., walking or jogging on a treadmill, using an elliptical trainer, cleaning house) is associated with better mental health outcomes than vigorous-intensity PA [ 36 , 40 ], and light-intensity PA [ 40 ]. On the other hand, vigorous-intensity PA better predicted depressive symptoms than moderate-intensity PA; also the effect size was higher for the association between vigorous-intensity PA and level of resilience compared with moderate-intensity PA [ 27 ]. One study found that performing high PA levels has no positive effect on depressive symptoms [ 39 ]. Another study explored the type of PA and showed that stretching and resistance training were associated with lower anxiety, and three types of PA (household chores, stretching, and resistance training) were associated with lower depression symptoms [ 48 ].
One study explored the association of specific types of physical exercise and mental health, and founded that home-based group entertainment exercise, rope skipping and badminton, Chinese traditional sports, video dancing and sensory-motor games present a greater reduction in mental health than others types [ 40 ].
Sedentary behaviour was observed in few studies and contradictory findings were observed. No association between sedentary time and depressive symptoms was observed [ 26 , 36 ]. However, other studies have shown that sedentary behaviour was associated with poorer mental health [ 25 ], well-being [ 32 ] and perceived stress [ 44 ].
Concerning the association between PA and mental health outcomes among children and adolescents, only four articles were selected. Some issues must be highlighted. This population had to face, beyond the reality that changed from the COVID-19 pandemic, the changes in the education system such as online learning became the main learning method for students and uncertainty of academic development, which probably caused more anxiety level [ 52 , 53 ]. Both moderate and highly active groups were significantly associated with less depressive symptoms [ 53 ] and anxiety [ 52 , 53 ], and only the most active adolescents reported significantly lower insomnia symptoms [ 53 ] and better mood states [ 55 ]. Days of physical activity per week was stronger predictor of well-being than minutes of physical activity per week [ 54 ].
Regarding old-age samples, the studies mentioned the particular vulnerability to changes in social circumstances, and highlight the importance of health-related quality of life [ 46 ] and levels of resilience [ 27 ] to deal with the consequences of the COVID-19 pandemic on the PA level. The stay-at-home order can cause greater distress and feelings of sadness, considered specific risk factor for depressive symptoms [ 34 ]. Mental health and physical activity decrease pre to during stay-at-home order [ 47 ]. In a group that were previously regular participants of a formal exercise program, MVPA was significantly higher within the non-depressed group compared with depressed group [ 26 ]. Being active previously of COVID-19 confinement did not prevent 30.4% of Brazilian older adults from having depressive symptoms, but these results is much lower than prevalence of depression in Brazilian general population, which is 68% [ 34 ].
The present systematic review had some limitations that must be mentioned. First, the studies present correlative analyses, not causal ones, thus randomised controlled trials must be conducted in the context of the COVID-19 pandemic and the stay-at-home order to clarify the direction of the association. However, beyond the COVID-19 context, randomised controlled trials showed that PA interventions show beneficial effects on mental health outcomes such as depression and anxiety [ 65 ]. Thus, a nuanced perspective particularly during the COVID-19 context in future research is needed. Moreover, the included studies with community samples were limited, and the analyses were mostly based on convenience samples with college students, which had specific characteristics and low mean age. Thus, future research needs to focus on representative study samples.
This review helps to clarify the positive association between PA and mental health during the first year of the COVID-19 pandemic, especially considering the effects of the stay-at-home order worldwide. Although there is an association between increased PA and improved mental health, further studies are needed, specifically randomised clinical trials, to identify the direction of this relationship, and what kind of PA, intensity, and frequency are most indicated to maximise the effects. Also, an investigation to examine the association during the second year of the COVID-19 pandemic is needed. The impact of the COVID-19 pandemic on mental health may be continuous and long-term [ 66 , 67 ]. Thus, public health agencies must provide timely and effective interventions, in which PA and exercise should be a priority.
Availability of data and materials
Data sharing is not applicable to this article as no datasets were generated or analysed during the current study.
Abbreviations
Coronavirus disease-19
Severe acute respiratory syndrome coronavirus-2
Physical activity
World Health Organization
International physical activity questionnaire
Metabolic equivalent
Conti P, Caraffa A, Gallenga CE, Kritas SK, Frydas I, Younes A, et al. The British variant of the new coronavirus-19 (Sars-Cov-2) should not create a vaccine problem. J Biol Regul Homeost Agents. 2020;35(1).
Ritchie H, Ortiz-Ospina E.; Beltekian D., Mathieu E., Hasell J., Macdonald B., Giattino C. and Roser M. Coronavirus (COVID-19) Vaccinations 2021 Available from: https://ourworldindata.org/covid-vaccinations .
Google Scholar
Organization WH. Coronavirus disease (COVID-19): Vaccines 2021 [Available from: https://www.who.int/news-room/q-a-detail/coronavirus-disease-(covid-19)-vaccines?adgroupsurvey={adgroupsurvey}&gclid=CjwKCAiA1eKBBhBZEiwAX3gqlwcwqCmOIN-6welx1Msuxpy-YUyvsWAV-BYZOJ1g1qS0oUYbLEPiZxoC9uQQAvD_BwE .
Our World in Data. Coronavirus (COVID-19) Vaccinations. 2021.
Organization WH. WHO Coronavirus Disease (COVID-19) Dashboard 2021 [Available from: https://covid19.who.int/?gclid=CjwKCAiAmrOBBhA0EiwArn3mfDVdryFCBj-_lYP99HuMas-LYQzoVDYdfWMBkTmfFA8QVtmS0ncCTRoCiFIQAvD_BwE .
WHO. Weekly epidemiological update on COVID-19 16 November 2021. 2021.
European Centre for Disease Prevention and Control. Data on country response measures to COVID-19 2021 [Available from: https://www.ecdc.europa.eu/en/publications-data/download-data-response-measures-covid-19 .
Moreland A, Herlihy C, Tynan MA, Sunshine G, McCord RF, Hilton C, et al. Timing of state and territorial COVID-19 stay-at-home orders and changes in population movement—United States, march 1–may 31, 2020. Morb Mortal Wkly Rep. 2020;69(35):1198.
Article CAS Google Scholar
Valentin F, Sylvain I, Oulmann Z, Rachel FR, Joël B, Pierre-Michel L, et al. COVID-19 pandemic lockdown and problematic eating behaviors in a student population. J Behav Addict. 2020;9(3):826–35.
Article Google Scholar
Chodkiewicz J, Talarowska M, Miniszewska J, Nawrocka N, Bilinski P. Alcohol consumption reported during the COVID-19 pandemic: the initial stage. Int J Environ Res Public Health. 2020;17(13):4677.
Article CAS PubMed Central Google Scholar
Wei Y, Shah R. Substance use disorder in the COVID-19 pandemic: a systematic review of vulnerabilities and complications. Pharmaceuticals. 2020;13(7):155.
Salari N, Hosseinian-Far A, Jalali R, Vaisi-Raygani A, Rasoulpoor S, Mohammadi M, et al. Prevalence of stress, anxiety, depression among the general population during the COVID-19 pandemic: a systematic review and meta-analysis. Glob Health. 2020;16(1):57.
Chen Q, Li M, Wang Y, Zhang L, Tan X. Changes in anxiety, depression, and stress in one week and one month later after the Wuhan shutdown against the COVID-19 epidemic. Disast Med Public Health Prepared. 2021;1-8.
Teychenne M, White RL, Richards J, Schuch FB, Rosenbaum S, Bennie JA. Do we need physical activity guidelines for mental health: what does the evidence tell us? Ment Health Phys Act. 2020;18:100315.
Marques A, Peralta M, Martins J, Catunda R, Matos MG, Saboga NL. Associations between physical activity and self-rated wellbeing in European adults: a population-based, cross-sectional study. Prev Med. 2016;91:18–23.
Article PubMed Google Scholar
WHO. Physical Inactivity: a global public health problem 2021 [Available from: https://www.who.int/ncds/prevention/physical-activity/inactivity-global-health-problem/en/ .
Marques A, Henriques-Neto D, Peralta M, Martins J, Demetriou Y, Schönbach DMI, et al. Prevalence of physical activity among adolescents from 105 low, middle, and high-income countries. Int J Environ Res Public Health. 2020;17(9).
Guthold R, Stevens GA, Riley LM, Bull FC. Worldwide trends in insufficient physical activity from 2001 to 2016: a pooled analysis of 358 population-based surveys with 1.9 million participants. Lancet Glob Health. 2018;6(10):e1077–e86.
Stockwell S, Trott M, Tully M, Shin J, Barnett Y, Butler L, et al. Changes in physical activity and sedentary behaviours from before to during the COVID-19 pandemic lockdown: a systematic review. BMJ Open Sport Exerc Med. 2021;7(1):e000960.
Violant-Holz V, Gallego-Jiménez MG, González-González CS, Muñoz-Violant S, Rodríguez MJ, Sansano-Nadal O, et al. Psychological health and physical activity levels during the COVID-19 pandemic: a systematic review. Int J Environ Res Public Health. 2020;17(24):9419.
Wells GA, Shea B, O’Connell D, Peterson J, Welch V, Losos M, et al. The Newcastle-Ottawa Scale (NOS) for assessing the quality if nonrandomized studies in meta-analyses. Available from: http://www.ohri.ca/programs/clinical_epidemiology/oxford.htm [cited 2021 Dec 28].
Modesti PA, Reboldi G, Cappuccio FP, Agyemang C, Remuzzi G, Rapi S, et al. Panethnic differences in blood pressure in Europe: a systematic review and Meta-analysis. PLoS One. 2016;11(1):e0147601.
Article PubMed PubMed Central Google Scholar
Moher D, Liberati A, Tetzlaff J, Altman DG. Preferred reporting items for systematic reviews and meta-analyses: the PRISMA statement. BMJ. 2009;339:b2535.
Abate Daga F, Agostino S, Peretti S, Beratto L. The impact of physical activity rate on subjective well-being among North-Western Italian population during COVID-19 nationwide lockdown. J Sports Med Phys Fitness. 2021.
Bird JM, Karageorghis CI, Hamer M. Relationships among behavioural regulations, physical activity, and mental health pre- and during COVID-19 UK lockdown. Psychol Sport Exerc. 2021;55:101945.
Carvalho J, Borges-Machado F, Pizarro AN, Bohn L, Barros D. Home confinement in previously active older adults: a cross-sectional analysis of physical fitness and physical activity behavior and their relationship with depressive symptoms. Front Psychol. 2021;12:643832.
Carriedo A, Cecchini JA, Fernandez-Rio J, Méndez-Giménez A. COVID-19, psychological well-being and physical activity levels in older adults during the Nationwide lockdown in Spain. Am J Geriatr Psychiatry. 2020;28(11):1146–55.
Cecchini JA, Carriedo A, Fernández-Río J, Méndez-Giménez A, González C, Sánchez-Martínez B, et al. A longitudinal study on depressive symptoms and physical activity during the Spanish lockdown. Int J Clin Health Psychol. 2021;21(1):100200.
Chouchou F, Augustini M, Caderby T, Caron N, Turpin NA, Dalleau G. The importance of sleep and physical activity on well-being during COVID-19 lockdown: Reunion island as a case study. Sleep Med. 2021;77:297–301.
Coughenour C, Gakh M, Pharr JR, Bungum T, Jalene S. Changes in depression and physical activity among college students on a diverse campus after a COVID-19 stay-at-home order. J Community Health. 2021;46(4):758–66.
Eric NO, Oghenebrorien AE, Onogimesike AT, Suoke OP. Mental wellbeing and exercise participation during covid-19 pandemic among adult residents of Nigeria's Niger delta. Int J Hum Move Sports Sci. 2020;8(6):534–42.
Faulkner J, O'Brien WJ, McGrane B, Wadsworth D, Batten J, Askew CD, et al. Physical activity, mental health and well-being of adults during initial COVID-19 containment strategies: a multi-country cross-sectional analysis. J Sci Med Sport. 2020;24(4):320–6.
Gierc M, Riazi NA, Fagan MJ, Di Sebastiano KM, Kandola M, Priebe CS, et al. Strange days: adult physical activity and mental health in the first two months of the COVID-19 pandemic. Front Public Health. 2021;9:567552.
Lage A, Carrapatoso S, de Queiroz S, Neto E, Gomes S, Soares-Miranda L, et al. Associations between depressive symptoms and physical activity intensity in an older adult population during COVID-19 lockdown. Front Psychol. 2021;12:644106.
Lesser IA, Nienhuis CP. The Impact of COVID-19 on Physical Activity Behavior and Well-Being of Canadians. Int J Environ Res Public Health. 2020;17(11):3899.
Lin J, Guo T, Becker B, Yu Q, Chen ST, Brendon S, et al. Depression is associated with moderate-intensity physical activity among college students during the COVID-19 pandemic: differs by activity level, gender and gender role. Psychol Res Behav Manag. 2020;13:1123–34.
Marashi MY, Nicholson E, Ogrodnik M, Fenesi B, Heisz JJ. A mental health paradox: mental health was both a motivator and barrier to physical activity during the COVID-19 pandemic. PLoS One. 2021;16(4):e0239244.
Article CAS PubMed PubMed Central Google Scholar
Maugeri G, Castrogiovanni P, Battaglia G, Pippi R, D'Agata V, Palma A, et al. The impact of physical activity on psychological health during Covid-19 pandemic in Italy. Heliyon. 2020;6(6):e04315.
Méndez-Giménez A, Cecchini JA, Fernández-Río J, Carriedo A. Physical activity and prevention of depressive symptoms in the Spanish population during confinement due to COVID-19. Psicothema. 2021;33(1):111–7.
PubMed Google Scholar
Nie YJ, Ma YY, Wu YK, Li JH, Liu T, Zhang C, et al. Association between physical exercise and mental health during the COVID-19 outbreak in China: a Nationwide cross-sectional study. Front Psychiatry. 2021;12:722448.
Nienhuis CP, Lesser IA. The Impact of COVID-19 on Women's Physical Activity Behavior and Mental Well-Being. Int J Environ Res Public Health. 2020;17(23):9036.
O'Brien L, Forster J. Physical activity supporting connection to nature, and helping to maintain wellbeing during the Covid-19 restrictions in England. Int J Environ Res Public Health. 2021;18(9):4585.
Ozdemir F, Cansel N, Kizilay F, Guldogan E, Ucuz I, Sinanoglu B, et al. The role of physical activity on mental health and quality of life during COVID-19 outbreak: a cross-sectional study. Eur J Integrat Med. 2020;40:101248.
Savage MJ, James R, Magistro D, Donaldson J, Healy LC, Nevill M, et al. Mental health and movement behaviour during the COVID-19 pandemic in UK university students: prospective cohort study. Ment Health Phys Act. 2020;19.
Stanton R, To QG, Khalesi S, Williams SL, Alley SJ, Thwaite TL, et al. Depression, Anxiety and Stress during COVID-19: Associations with Changes in Physical Activity, Sleep, Tobacco and Alcohol Use in Australian Adults. Int J Environ Res Public Health. 2020;17(11):4065.
Suzuki Y, Maeda N, Hirado D, Shirakawa T, Urabe Y. Physical Activity Changes and Its Risk Factors among Community-Dwelling Japanese Older Adults during the COVID-19 Epidemic: Associations with Subjective Well-Being and Health-Related Quality of Life. Int J Environ Res Public Health. 2020;17(18):6591.
Trabelsi K, Ammar A, Masmoudi L, Boukhris O, Chtourou H, Bouaziz B, et al. Sleep quality and physical activity as predictors of mental wellbeing variance in older adults during COVID-19 lockdown: ECLB COVID-19 international online survey. Int J Environ Res Public Health. 2021;18(8):4329.
Xiang MQ, Tan XM, Sun J, Yang HY, Zhao XP, Liu L, et al. Relationship of physical activity with anxiety and depression symptoms in Chinese college students during the COVID-19 outbreak. Front Psychol. 2020;11:582436.
Zalewska A, Gałczyk M, Sobolewski M, Białokoz-Kalinowska I. Depression as compared to level of physical activity and internet addiction among polish physiotherapy students during the COVID-19 pandemic. Int J Environ Res Public Health. 2021;18(19):10072.
Zhang Y, Zhang H, Ma X, Di Q. Mental Health Problems during the COVID-19 Pandemics and the Mitigation Effects of Exercise: A Longitudinal Study of College Students in China. Int J Environ Res Public Health. 2020;17(10):3722.
Young DR, Hong BD, Lo T, Inzhakova G, Cohen DA, Sidell MA. The longitudinal associations of physical activity, time spent outdoors in nature and symptoms of depression and anxiety during COVID-19 quarantine and social distancing in the United States. Prev Med. 2021;154:106863.
Alves JM, Yunker AG, DeFendis A, Xiang AH, Page KA. Associations between affect, physical activity, and anxiety among US children during COVID-19. medRxiv. 2020.
Chi X, Liang K, Chen ST, Huang Q, Huang L, Yu Q, et al. Mental health problems among Chinese adolescents during the COVID-19: the importance of nutrition and physical activity. Int J Clin Health Psychol. 2020;21:100218.
Morres ID, Galanis E, Hatzigeorgiadis A, Androutsos O, Theodorakis Y. Physical activity, sedentariness, eating behaviour and well-being during a COVID-19 lockdown period in Greek adolescents. Nutrients. 2021;13(5):1449.
Kang S, Sun Y, Zhang X, Sun F, Wang B, Zhu W. Is physical activity associated with mental health among Chinese adolescents during isolation in COVID-19 pandemic? J Epidemiol Global Health. 2020;11(1):26–33.
Chouchou F, Augustini M, Caderby T, Caron N, Turpin NA, Dalleau G. The importance of sleep and physical activity on well-being during COVID-19 lockdown: Reunion island as a case study. Sleep Med. 2020:297–301.
Coughenour C, Gakh M, Pharr JR, Bungum T, Jalene S. Changes in depression and physical activity among college students on a diverse campus after a COVID-19 stay-at-home order. J Community Health. 2020:758–66.
Lima CKT, Carvalho PMM, Lima I, Nunes J, Saraiva JS, de Souza RI, et al. The emotional impact of coronavirus 2019-nCoV (new coronavirus disease). Psychiatry Res. 2020;287:112915.
Hahad O, Gilan DA, Daiber A, Münzel T. Public mental health as one of the key factors in dealing with COVID-19. Gesundheitswesen. 2020;82(5):389–91.
PubMed PubMed Central Google Scholar
Jakobsson J, Malm C, Furberg M, Ekelund U, Svensson M. Physical activity during the coronavirus (COVID-19) pandemic: prevention of a decline in metabolic and immunological functions. Front Sports Active Living. 2020;2:57.
Riecher-Rössler A. Sex and gender differences in mental disorders. Lancet Psychiatry. 2017;4(1):8–9.
Giustino V, Parroco AM, Gennaro A, Musumeci G, Palma A, Battaglia G. Physical activity levels and related energy expenditure during COVID-19 quarantine among the Sicilian active population: a cross-sectional online survey study. Sustainability. 2020;12(11):4356.
Committee IR. Guidelines for data processing and analysis of the International Physical Activity Questionnaire (IPAQ)-short and long forms. http://www.ipaq.ki.se/scoring.pdf . 2005.
Thompson PD, Arena R, Riebe D, Pescatello LS. ACSM’s new preparticipation health screening recommendations from ACSM’s guidelines for exercise testing and prescription. Curr Sports Med Rep. 2013;12(4):215–7.
Neill RD, Lloyd K, Best P, Tully MA. The effects of interventions with physical activity components on adolescent mental health: systematic review and meta-analysis. Ment Health Phys Act. 2020;19:100359.
Gan Y, Ma J, Wu J, Chen Y, Zhu H, Hall BJ. Immediate and delayed psychological effects of province-wide lockdown and personal quarantine during the COVID-19 outbreak in China. Psychol Med. 2020:1–12.
Sher L. The impact of the COVID-19 pandemic on suicide rates. QJM. 2020;113(10):707–12.
Article CAS PubMed Google Scholar
Download references
Acknowledgements
AI acknowledges support from the Swiss National Centre of Competence in Research LIVES – Overcoming vulnerability: life course perspectives, granted by the Swiss National Science Foundation (grant number: 51NF40-185901). AI acknowledges support from the Swiss National Science Foundation (grant number: 10001C_189407). André Werneck is supported by the São Paulo Research Foundation (FAPESP) with a PhD scholarship (FAPESP process: 2019/24124-7). This paper presents independent research. The views expressed in this publication are those of the authors and not necessarily those of the acknowledged institutions.
No funding.
Author information
Authors and affiliations.
KinesioLab, Research Unit in Human Movement Analysis, Insituto Piaget, Almada, Portugal
Priscila Marconcin
CIPER, Faculdade de Motricidade Humana, Universidade de Lisboa, Lisbon, Portugal
Priscila Marconcin, Miguel Peralta & Adilson Marques
Center for Epidemiological Research in Nutrition and Health, Department of Nutrition, School of Public Health, São Paulo, Brazil
André O. Werneck & Adilson Marques
ISAMB, Universidade de Lisboa, Lisbon, Portugal
Miguel Peralta
Center for the Interdisciplinary Study of Gerontology and Vulnerability, University of Geneva, Geneva, Switzerland
Andreas Ihle
Swiss National Centre of Competence in Research LIVES – Overcoming vulnerability: Life course perspectives, Lausanne and Geneva, Switzerland
Department of Psychology, University of Geneva, Geneva, Switzerland
Universidade da Madeira, Funchal, Portugal
Élvio R. Gouveia
Interactive Technologies Institute, LARSyS, Funchal, Portugal
Laboratorio de Rendimiento Humano, Grupo de Estudio en Educación, Actividad Física y Salud (GEEAFyS), Universidad Católica del Maule, Talca, Chile
Gerson Ferrari
University of Coimbra, Research Unit for Sport and Physical Activity (CIDAF). Faculty of Sport Sciences and Physical Education, Coimbra, Portugal
Hugo Sarmento
You can also search for this author in PubMed Google Scholar
Contributions
All authors were involved in drafting the article or revising it critically for important intellectual content, and all authors approved the final version to be submitted for publication. PM, MP and AM had full access to all of the data in the study and take responsibility for the integrity of the data and the accuracy of the data analysis. PM, MP and AM, study conception and design. PM and MP acquisition of data. PM, MP and AM, analysis and interpretation of data.
Corresponding author
Correspondence to Priscila Marconcin .
Ethics declarations
Ethics approval and consent to participate.
Not applicable.
Consent for publication
Competing interests.
The authors declare that they have no competing interests.
Additional information
Publisher’s note.
Springer Nature remains neutral with regard to jurisdictional claims in published maps and institutional affiliations.
Rights and permissions
Open Access This article is licensed under a Creative Commons Attribution 4.0 International License, which permits use, sharing, adaptation, distribution and reproduction in any medium or format, as long as you give appropriate credit to the original author(s) and the source, provide a link to the Creative Commons licence, and indicate if changes were made. The images or other third party material in this article are included in the article's Creative Commons licence, unless indicated otherwise in a credit line to the material. If material is not included in the article's Creative Commons licence and your intended use is not permitted by statutory regulation or exceeds the permitted use, you will need to obtain permission directly from the copyright holder. To view a copy of this licence, visit http://creativecommons.org/licenses/by/4.0/ . The Creative Commons Public Domain Dedication waiver ( http://creativecommons.org/publicdomain/zero/1.0/ ) applies to the data made available in this article, unless otherwise stated in a credit line to the data.
Reprints and permissions
About this article
Cite this article.
Marconcin, P., Werneck, A.O., Peralta, M. et al. The association between physical activity and mental health during the first year of the COVID-19 pandemic: a systematic review. BMC Public Health 22 , 209 (2022). https://doi.org/10.1186/s12889-022-12590-6
Download citation
Received : 28 October 2021
Accepted : 17 January 2022
Published : 01 February 2022
DOI : https://doi.org/10.1186/s12889-022-12590-6
Share this article
Anyone you share the following link with will be able to read this content:
Sorry, a shareable link is not currently available for this article.
Provided by the Springer Nature SharedIt content-sharing initiative
- Mental health
BMC Public Health
ISSN: 1471-2458
- General enquiries: [email protected]
- Frontiers in Human Neuroscience
- Brain Health and Clinical Neuroscience
- Research Topics
Exercising body & brain: the effects of physical exercise on brain health
Total Downloads
Total Views and Downloads
About this Research Topic
The relationship between physical exercise and brain health is a burgeoning field of research in neuroscience, with a pivotal impact on our understanding of cognitive well-being, mental health, and aging. Existing studies evidence the positive influences of regular physical activity on brain health, suggesting its implications on learning, memory, and mood. Despite significant advancements, comprehensive analysis incorporating broader perspectives and deeper explorations remain scarce. The objective of this Research Topic is to create an enriching platform for focused discourse on the interconnection between physical exercise and brain health. The goal is to bring together theoretical and experimental research papers that depict a comprehensive overview of recent developments, examine the mechanistic underpinnings of the exercise-brain interaction, and delve into the future potential of this promising area. We welcome contributions that explore, but are not limited to, the following themes: • Impact of various types of exercises on mental health and cognitive functions. • Role of physical activity in stress, anxiety, and mood disorders management. • The molecular and neurochemical effects of exercise on the brain. • Exercise mitigating neurodegenerative disorders and age-related cognitive decline. • Effects of physical exercise on brain development and neuroplasticity. Manuscript types desired for this topic are Original Research, Review, Systematic Review, Mini Review, Perspective, and Opinion articles. Emphasis is on rigorous and high-quality methodology, analysis, and data presentation. The Research Topic places a high priority on interdisciplinary approaches and the potential practical implications of research findings.
Keywords : yoga, physical exercise, mental health
Important Note : All contributions to this Research Topic must be within the scope of the section and journal to which they are submitted, as defined in their mission statements. Frontiers reserves the right to guide an out-of-scope manuscript to a more suitable section or journal at any stage of peer review.
Topic Editors
Topic coordinators, submission deadlines.
Manuscript Summary | |
Manuscript |
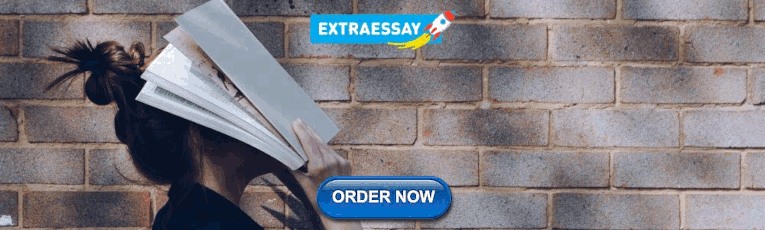
Participating Journals
Manuscripts can be submitted to this Research Topic via the following journals:
total views
- Demographics
No records found
total views article views downloads topic views
Top countries
Top referring sites, about frontiers research topics.
With their unique mixes of varied contributions from Original Research to Review Articles, Research Topics unify the most influential researchers, the latest key findings and historical advances in a hot research area! Find out more on how to host your own Frontiers Research Topic or contribute to one as an author.
Nutritional Considerations in Exercise-Based Heat Acclimation: A Narrative Review
- Review Article
- Published: 31 August 2024
Cite this article
- Paul Rosbrook ORCID: orcid.org/0000-0002-1767-1615 1 ,
- Lee M. Margolis 2 &
- J. Luke Pryor 1
In addition to its established thermoregulatory and cardiovascular effects, heat stress provokes alterations in macronutrient metabolism, gastrointestinal integrity, and appetite. Inadequate energy, carbohydrate, and protein intake have been implicated in reduced exercise and heat tolerance. Classic exercise heat acclimation (HA) protocols employ low-to-moderate–intensity exercise for 5–14 days, while recent studies have evolved the practice by implementing high-intensity and task-specific exercise during HA, which potentially results in impaired post-HA physical performance despite adequate heat adaptations. While there is robust literature demonstrating the performance benefit of various nutritional interventions during intensive training and competition, most HA studies implement few nutritional controls. This review summarizes the relationships between heat stress, HA, and intense exercise in connection with substrate metabolism, gastrointestinal function, and the potential consequences of reduced energy availability. We discuss the potential influence of macronutrient manipulations on HA study outcomes and suggest best practices to implement nutritional controls.
This is a preview of subscription content, log in via an institution to check access.
Access this article
Subscribe and save.
- Get 10 units per month
- Download Article/Chapter or eBook
- 1 Unit = 1 Article or 1 Chapter
- Cancel anytime
Price includes VAT (Russian Federation)
Instant access to the full article PDF.
Rent this article via DeepDyve
Institutional subscriptions
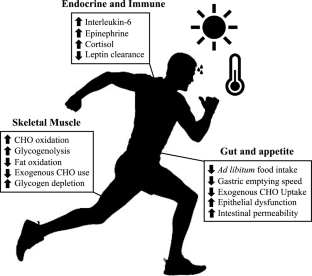
Similar content being viewed by others
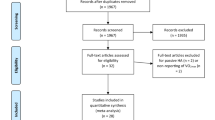
Effects of Heat Acclimation and Acclimatisation on Maximal Aerobic Capacity Compared to Exercise Alone in Both Thermoneutral and Hot Environments: A Meta-Analysis and Meta-Regression
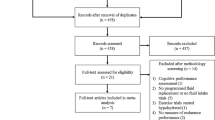
Impact of Ad Libitum Versus Programmed Drinking on Endurance Performance: A Systematic Review with Meta-Analysis
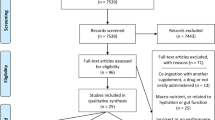
The Effect of Dietary Supplements on Endurance Exercise Performance and Core Temperature in Hot Environments: A Meta-analysis and Meta-regression
Febbraio M, Snow R, Hargreaves M, Stathis C, Martin I, Carey M. Muscle metabolism during exercise and heat stress in trained men: Effect of acclimation. J Appl Physiol. 1985;1994(76):589–97.
Google Scholar
Febbraio MA. Alterations in energy metabolism during exercise and heat stress. Sports Med. 2001;31:47–59.
Article PubMed Google Scholar
Hargreaves M, Angus D, Howlett K, Conus NM, Febbraio M. Effect of heat stress on glucose kinetics during exercise. J Appl Physiol. 1985;1996(81):1594–7.
Rosbrook P, Sweet D, Qiao J, Looney DP, Margolis LM, Hostler D, et al. Heat stress increases carbohydrate oxidation rates and oxygen uptake during prolonged load carriage exercise. Temperature. 2024;11:170–81.
Article Google Scholar
Periard JD, Eijsvogels TM, Daanen HA. Exercise under heat stress: thermoregulation, hydration, performance implications and mitigation strategies. Physiol Rev. 2021;101:1873–979.
Benjamin CL, Sekiguchi Y, Fry LA, Casa DJ. Performance changes following heat acclimation and the factors that influence these changes: meta-analysis and meta-regression. Front Physiol. 2019;10:1448.
Article PubMed PubMed Central Google Scholar
Lorenzo S, Halliwill JR, Sawka MN, Minson CT. Heat acclimation improves exercise performance. J Appl Physiol. 2010;109:1140–7.
Pryor JL, Johnson EC, Roberts WO, Pryor RR. Application of evidence-based recommendations for heat acclimation: Individual and team sport perspectives. Temperature. 2019;6:37–49.
Schmit C, Duffield R, Hausswirth C, Brisswalter J, Le Meur Y. Optimizing heat acclimation for endurance athletes: high- versus low-intensity training. Int J Sports Physiol Perform. 2018;13:816–23.
Reeve T, Gordon R, Laursen PB, Lee JKW, Tyler CJ. Impairment of cycling capacity in the heat in well-trained endurance athletes after high-intensity short-term heat acclimation. Int J Sports Physiol Perform. 2019;14:1058–65.
Waldron M, Fowler R, Heffernan S, Tallent J, Kilduff L, Jeffries O. Effects of heat acclimation and acclimatisation on maximal aerobic capacity compared to exercise alone in both thermoneutral and hot environments: a meta-analysis and meta-regression. Sports Med Auckl NZ. 2021;51:1509–25.
King DS, Costill DL, Fink WJ, Hargreaves M, Fielding RA. Muscle metabolism during exercise in the heat in unacclimatized and acclimatized humans. J Appl Physiol. 1985;59:1350–4.
McCubbin AJ, Allanson BA, Odgers JNC, Cort MM, Costa RJS, Cox GR, et al. Sports dietitians Australia position statement: nutrition for exercise in hot environments. Int J Sport Nutr Exerc Metab. 2020;30:83–98.
Houmard JA, Costill DL, Davis JA, Mitchell JB, Pascoe DD, Robergs RA. The influence of exercise intensity on heat acclimation in trained subjects. Med Sci Sports Exerc. 1990;22:615–20.
McIntyre RD, Zurawlew MJ, Mee JA, Walsh NP, Oliver SJ. A comparison of medium-term heat acclimation by post-exercise hot water immersion or exercise in the heat: adaptations, overreaching, and thyroid hormones. Am J Physiol Regul Integr Comp Physiol. 2022;323:R601–15.
Racinais S, Périard JD, Piscione J, Bourdon PC, Cocking S, Ihsan M, et al. Intensified training supersedes the impact of heat and/or altitude for increasing performance in Elite Rugby Union Players. Int J Sports Physiol Perform. 2021;16:1416–23.
Costill DL, Bowers R, Branam G, Sparks K. Muscle glycogen utilization during prolonged exercise on successive days. J Appl Physiol. 1971;31:834–8.
Areta JL, Taylor HL, Koehler K. Low energy availability: history, definition and evidence of its endocrine, metabolic and physiological effects in prospective studies in females and males. Eur J Appl Physiol. 2021;121:1–21.
Loucks AB, Kiens B, Wright HH. Energy availability in athletes. J Sports Sci. 2011;29(Suppl 1):S7-15.
Mountjoy M, Sundgot-Borgen J, Burke L, Ackerman KE, Blauwet C, Constantini N, et al. International Olympic Committee (IOC) Consensus Statement on Relative Energy Deficiency in Sport (RED-S): 2018 Update. Int J Sport Nutr Exerc Metab. 2018;28:316–31.
Heikura IA, Uusitalo ALT, Stellingwerff T, Bergland D, Mero AA, Burke LM. Low energy availability is difficult to assess but outcomes have large impact on bone injury rates in elite distance athletes. Int J Sport Nutr Exerc Metab. 2018;28:403–11.
Tornberg ÅB, Melin A, Koivula FM, Johansson A, Skouby S, Faber J, et al. Reduced neuromuscular performance in amenorrheic elite endurance athletes. Med Sci Sports Exerc. 2017;49:2478–85.
Kerksick CM, Arent S, Schoenfeld BJ, Stout JR, Campbell B, Wilborn CD, et al. International society of sports nutrition position stand: nutrient timing. J Int Soc Sports Nutr. 2017;14:33.
Gonzalez DE, McAllister MJ, Waldman HS, Ferrando AA, Joyce J, Barringer ND, et al. International society of sports nutrition position stand: tactical athlete nutrition. J Int Soc Sports Nutr. 2022;19:267–315.
Arent SM, Cintineo HP, McFadden BA, Chandler AJ, Arent MA. Nutrient timing: a garage door of opportunity? Nutrients. 2020;12:E1948.
Abbiss CR, Peiffer JJ, Peake JM, Nosaka K, Suzuki K, Martin DT, et al. Effect of carbohydrate ingestion and ambient temperature on muscle fatigue development in endurance-trained male cyclists. J Appl Physiol. 2008;104:1021–8.
Achten J, Halson SL, Moseley L, Rayson MP, Casey A, Jeukendrup AE. Higher dietary carbohydrate content during intensified running training results in better maintenance of performance and mood state. J Appl Physiol Bethesda Md. 1985;2004(96):1331–40.
Flouris AD, Dinas PC, Ioannou LG, Nybo L, Havenith G, Kenny GP, et al. Workers’ health and productivity under occupational heat strain: a systematic review and meta-analysis. Lancet Planet Health. 2018;2:e521–31.
Armstrong CG, Kenney WL. Effects of age and acclimation on responses to passive heat exposure. J Appl Physiol. 1993;75:2162–7.
Cheung SS, McLellan TM. Heat acclimation, aerobic fitness, and hydration effects on tolerance during uncompensable heat stress. J Appl Physiol. 1998;84:1731–9.
Cramer MN, Jay O. Biophysical aspects of human thermoregulation during heat stress. Auton Neurosci Basic Clin. 2016;196:3–13.
Foster J, Hodder SG, Lloyd AB, Havenith G. Individual responses to heat stress: implications for hyperthermia and physical work capacity. Front Physiol. 2020;11: 541483.
Kenney WL. A review of comparative responses of men and women to heat stress. Environ Res. 1985;37:1–11.
Selkirk GA, McLellan TM. Influence of aerobic fitness and body fatness on tolerance to uncompensable heat stress. J Appl Physiol. 2001;91:2055–63.
Starkie RL, Hargreaves M, Lambert DL, Proietto J, Febbraio MA. Effect of temperature on muscle metabolism during submaximal exercise in humans. Exp Physiol. 1999;84:775–84.
Cady EB, Jones DA, Lynn J, Newham DJ. Changes in force and intracellular metabolites during fatigue of human skeletal muscle. J Physiol. 1989;418:311–25.
Jentjens RLPG, Wagenmakers AJM, Jeukendrup AE. Heat stress increases muscle glycogen use but reduces the oxidation of ingested carbohydrates during exercise. J Appl Physiol. 1985;2002(92):1562–72.
Coyle EF. Substrate utilization during exercise in active people. Am J Clin Nutr. 1995;61:968S-979S.
Maunder E, Plews DJ, Merien F, Kilding AE. Exercise intensity regulates the effect of heat stress on substrate oxidation rates during exercise. Eur J Sport Sci. 2020;20:935–43.
Cheuvront SN, Kenefick RW, Montain SJ, Sawka MN. Mechanisms of aerobic performance impairment with heat stress and dehydration. J Appl Physiol. 1985;2010(109):1989–95.
Sawka MN, Burke LM, Eichner ER, Maughan RJ, Montain SJ, Stachenfeld NS. American College of Sports Medicine position stand. Exercise and fluid replacement. Med Sci Sports Exerc. 2007;39:377–90.
PubMed Google Scholar
González-Alonso J, Crandall CG, Johnson JM. The cardiovascular challenge of exercising in the heat. J Physiol. 2008;586:45–53.
Trangmar SJ, González-Alonso J. New insights into the impact of dehydration on blood flow and metabolism during exercise. Exerc Sport Sci Rev. 2017;45:146–53.
Logan-Sprenger HM, Heigenhauser GJF, Jones GL, Spriet LL. The effect of dehydration on muscle metabolism and time trial performance during prolonged cycling in males. Physiol Rep. 2015;3: e12483.
Khong TK, Selvanayagam VS, Sidhu SK, Yusof A. Role of carbohydrate in central fatigue: a systematic review. Scand J Med Sci Sports. 2017;27:376–84.
Davis JM, Bailey SP, Woods JA, Galiano FJ, Hamilton MT, Bartoli WP. Effects of carbohydrate feedings on plasma free tryptophan and branched-chain amino acids during prolonged cycling. Eur J Appl Physiol. 1992;65:513–9.
Robson P. Elucidating the unexplained underperformance syndrome in endurance athletes : the interleukin-6 hypothesis. Sports Med Auckl NZ. 2003;33:771–81.
Pedersen BK. Muscular interleukin-6 and its role as an energy sensor. Med Sci Sports Exerc. 2012;44:392–6.
Pedersen BK, Steensberg A, Fischer C, Keller C, Keller P, Plomgaard P, et al. Searching for the exercise factor: is IL-6 a candidate? J Muscle Res Cell Motil. 2003;24:113–9.
Kistner TM, Pedersen BK, Lieberman DE. Interleukin 6 as an energy allocator in muscle tissue. Nat Metab. 2022;4:170–9.
Robson-Ansley PJ, de Milander L, Collins M, Noakes TD. Acute interleukin-6 administration impairs athletic performance in healthy, trained male runners. Can J Appl Physiol Rev Can Physiol Appl. 2004;29:411–8.
Willmott AGB, Hayes M, James CA, Dekerle J, Gibson OR, Maxwell NS. Once- and twice-daily heat acclimation confer similar heat adaptations, inflammatory responses and exercise tolerance improvements. Physiol Rep. 2018;6: e13936.
Costello JT, Rendell RA, Furber M, Massey HC, Tipton MJ, Young JS, et al. Effects of acute or chronic heat exposure, exercise and dehydration on plasma cortisol, IL-6 and CRP levels in trained males. Cytokine. 2018;110:277–83.
Guy JH, Pyne DB, Deakin GB, Miller CM, Edwards AM. Acclimation training improves endurance cycling performance in the heat without inducing endotoxemia. Front Physiol. 2016;7:318.
Nybo L, Møller K, Volianitis S, Nielsen B, Secher NH. Effects of hyperthermia on cerebral blood flow and metabolism during prolonged exercise in humans. J Appl Physiol Bethesda Md. 1985;2002(93):58–64.
Nybo L, Nielsen B. Hyperthermia and central fatigue during prolonged exercise in humans. J Appl Physiol Bethesda Md. 1985;2001(91):1055–60.
Alkemade P, Gerrett N, Eijsvogels TMH, Daanen HAM. Individual characteristics associated with the magnitude of heat acclimation adaptations. Eur J Appl Physiol. 2021;121:1593–606.
Daanen H, Jonkman A, Layden J, Linnane D, Weller A. Optimising the acquisition and retention of heat acclimation. Int J Sports Med. 2011;32:822–8.
Pryor JL, Pryor RR, Vandermark LW, Adams EL, VanScoy RM, Casa DJ, et al. Intermittent exercise-heat exposures and intense physical activity sustain heat acclimation adaptations. J Sci Med Sport. 2019;22:117–22.
Armstrong LE, Francesconi RP, Kraemer WJ, Leva N, De Luca JP, Hubbard RW. Plasma cortisol, renin, and aldosterone during an intense heat acclimation program. Int J Sports Med. 1989;10:38–42.
Buono MJ, Martha SL, Heaney JH. Peripheral sweat gland function is improved with humid heat acclimation. J Therm Biol. 2009;34:127–30.
Horowitz M. Heat acclimation, epigenetics, and cytoprotection memory. Compr Physiol. 2014;4:199–230.
Young A, Sawka M, Levine L, Cadarette B, Pandolf K. Skeletal muscle metabolism during exercise is influenced by heat acclimation. J Appl Physiol. 1985;1986(59):1929–35.
Kirwan JP, Costill DL, Kuipers H, Burrell MJ, Fink WJ, Kovaleski JE, et al. Substrate utilization in leg muscle of men after heat acclimation. J Appl Physiol Bethesda Md. 1985;1987(63):31–5.
Hargreaves M, McConell G, Proietto J. Influence of muscle glycogen on glycogenolysis and glucose uptake during exercise in humans. J Appl Physiol. 1995;78:288–92.
Gupta A, Chauhan NR, Chowdhury D, Singh A, Meena RC, Chakrabarti A, et al. Heat stress modulated gastrointestinal barrier dysfunction: role of tight junctions and heat shock proteins. Scand J Gastroenterol. 2017;52:1315–9.
King MA, Rollo I, Baker LB. Nutritional considerations to counteract gastrointestinal permeability during exertional heat stress. J Appl Physiol. 2021;130:1754–65.
Pearce SC, Mani V, Weber TE, Rhoads RP, Patience JF, Baumgard LH, et al. Heat stress and reduced plane of nutrition decreases intestinal integrity and function in pigs. J Anim Sci. 2013;91:5183–93.
van Nieuwenhoven MA, Brouns F, Brummer RJ. The effect of physical exercise on parameters of gastrointestinal function. Neurogastroenterol Motil. 1999;11:431–9.
Ogden HB, Child RB, Fallowfield JL, Delves SK, Westwood CS, Layden JD. The gastrointestinal exertional heat stroke paradigm: pathophysiology, assessment, severity, aetiology and nutritional countermeasures. Nutrients. 2020;12:537.
Davison G, Marchbank T, March DS, Thatcher R, Playford RJ. Zinc carnosine works with bovine colostrum in truncating heavy exercise-induced increase in gut permeability in healthy volunteers. Am J Clin Nutr. 2016;104:526–36.
Pugh JN, Impey SG, Doran DA, Fleming SC, Morton JP, Close GL. Acute high-intensity interval running increases markers of gastrointestinal damage and permeability but not gastrointestinal symptoms. Appl Physiol Nutr Metab Physiol Appl Nutr Metab. 2017;42:941–7.
Snipe RMJ, Khoo A, Kitic CM, Gibson PR, Costa RJS. The impact of mild heat stress during prolonged running on gastrointestinal integrity, gastrointestinal symptoms, systemic endotoxin and cytokine profiles. Int J Sports Med. 2018;39:255–63.
Costa RJS, Camões-Costa V, Snipe RMJ, Dixon D, Russo I, Huschtscha Z. Impact of exercise-induced hypohydration on gastrointestinal integrity, function, symptoms, and systemic endotoxin and inflammatory profile. J Appl Physiol. 2019;126:1281–91.
Jonvik KL, Lenaerts K, Smeets JSJ, Kolkman JJ, Loon VAN LJC, Verdijk LB. Sucrose but not nitrate ingestion reduces strenuous cycling-induced intestinal injury. Med Sci Sports Exerc. 2019;51:436–44.
Barberio MD, Elmer DJ, Laird RH, Lee KA, Gladden B, Pascoe DD. Systemic LPS and inflammatory response during consecutive days of exercise in heat. Int J Sports Med. 2015;36:262–70.
Moss JN, Bayne FM, Castelli F, Naughton MR, Reeve TC, Trangmar SJ, et al. Short-term isothermic heat acclimation elicits beneficial adaptations but medium-term elicits a more complete adaptation. Eur J Appl Physiol. 2020;120:243–54.
Pugh JN, Sage S, Hutson M, Doran DA, Fleming SC, Highton J, et al. Glutamine supplementation reduces markers of intestinal permeability during running in the heat in a dose-dependent manner. Eur J Appl Physiol. 2017;117:2569–77.
Zuhl MN, Lanphere KR, Kravitz L, Mermier CM, Schneider S, Dokladny K, et al. Effects of oral glutamine supplementation on exercise-induced gastrointestinal permeability and tight junction protein expression. J Appl Physiol. 2014;116:183–91.
Ogden HB, Fallowfield JL, Child RB, Davison G, Fleming SC, Delves SK, et al. Acute L-glutamine supplementation does not improve gastrointestinal permeability, injury or microbial translocation in response to exhaustive high intensity exertional-heat stress. Eur J Sport Sci. 2022;22:1865–76.
Ogden HB, Fallowfield JL, Child RB, Davison G, Fleming SC, Delves SK, et al. No protective benefits of low dose acute L-glutamine supplementation on small intestinal permeability, epithelial injury and bacterial translocation biomarkers in response to subclinical exertional-heat stress: a randomized cross-over trial. Temperature. 2022;9:196–210.
Herman CP. Effects of heat on appetite. In: Marriott BM, editor. Nutritional needs in hot environments: applications for military personnel in field operations. Washington, DC: National Academies Press; 1993. p. 187–214.
He X, Lu Z, Ma B, Zhang L, Li J, Jiang Y, et al. Chronic heat stress alters hypothalamus integrity, the serum indexes and attenuates expressions of hypothalamic appetite genes in broilers. J Therm Biol. 2019;81:110–7.
Hunschede S, Schwartz A, Kubant R, Thomas SG, Anderson GH. The role of IL-6 in exercise-induced anorexia in normal-weight boys. Appl Physiol Nutr Metab. 2018;43:979–87.
McGlynn ML, Shute RJ, Slivka DR. The influence of heat on appetite-regulating adipokines. Curr Res Diabetes Obes J. 2020;12:128–32.
Bilski J, Mazur-Bialy AI, Surmiak M, Hubalewska-Mazgaj M, Pokorski J, Nitecki J, et al. Effect of acute sprint exercise on myokines and food intake hormones in young healthy men. Int J Mol Sci. 2020;21:8848.
Koehler K, Achtzehn S, Braun H, Mester J, Schaenzer W. Comparison of self-reported energy availability and metabolic hormones to assess adequacy of dietary energy intake in young elite athletes. Appl Physiol Nutr Metab. 2013;38:725–33.
Burrows TL, Ho YY, Rollo ME, Collins CE. Validity of dietary assessment methods when compared to the method of doubly labeled water: a systematic review in adults. Front Endocrinol [Internet]. 2019. https://doi.org/10.3389/fendo.2019.00850 . Accessed 17 July 2024.
McGuire A, Warrington G, Doyle L. Low energy availability in male athletes: a systematic review of incidence, associations, and effects. Transl SPORTS Med. 2020;3:173–87.
Murphy C, Koehler K. Caloric restriction induces anabolic resistance to resistance exercise. Eur J Appl Physiol. 2020;120:1155–64.
Kojima C, Ishibashi A, Tanabe Y, Iwayama K, Kamei A, Takahashi H, et al. Muscle glycogen content during endurance training under low energy availability. Med Sci Sports Exerc. 2020;52:187–95.
Areta JL, Burke LM, Camera DM, West DWD, Crawshay S, Moore DR, et al. Reduced resting skeletal muscle protein synthesis is rescued by resistance exercise and protein ingestion following short-term energy deficit. Am J Physiol Endocrinol Metab. 2014;306:E989-997.
Kuikman MA, Coates AM, Burr JF. Markers of low energy availability in overreached athletes: a systematic review and meta-analysis. Sports Med. 2022;52:2925–41.
Loucks DAB. Low energy availability in the marathon and other endurance sports. Sports Med. 2007;37:348–52.
Morris JG, Nevill ME, Boobis LH, Macdonald IA, Williams C. Muscle metabolism, temperature, and function during prolonged, intermittent, high-intensity running in air temperatures of 33 degrees and 17 degrees C. Int J Sports Med. 2005;26:805–14.
Neufer PD, Young AJ, Sawka MN. Gastric emptying during exercise: effects of heat stress and hypohydration. Eur J Appl Physiol. 1989;58:433–9.
Jeukendrup AE. Training the gut for athletes. Sports Med. 2017;47:101–10.
Baumgard LH, Rhoads RP Jr. Effects of heat stress on postabsorptive metabolism and energetics. Annu Rev Anim Biosci. 2013;1:311–37.
Jentjens RLPG, Underwood K, Achten J, Currell K, Mann CH, Jeukendrup AE. Exogenous carbohydrate oxidation rates are elevated after combined ingestion of glucose and fructose during exercise in the heat. J Appl Physiol. 1985;2006(100):807–16.
Carter J, Jeukendrup AE, Mundel T, Jones DA. Carbohydrate supplementation improves moderate and high-intensity exercise in the heat. Pflüg Arch. 2003;446:211–9.
Dolny DG, Lemon PW. Effect of ambient temperature on protein breakdown during prolonged exercise. J Appl Physiol Bethesda Md. 1985;1988(64):550–5.
Febbraio M, Snow RJ, Stathis CG, Hargreaves M, Carey MF. Effect of heat stress on muscle energy metabolism during exercise. J Appl Physiol. 1994;77:2827–31.
Goto K, Oda H, Morioka S, Naito T, Akema T, Kato H, et al. Skeletal muscle hypertrophy induced by low-intensity exercise with heat-stress in healthy human subjects. Jpn J Aerosp Env Med. 2007;44:13–8.
Snow RJ, Febbraio MA, Carey MF, Hargreaves M. Heat stress increases ammonia accumulation during exercise in humans. Exp Physiol. 1993;78:847–50.
Wheelock JB, Rhoads RP, Vanbaale MJ, Sanders SR, Baumgard LH. Effects of heat stress on energetic metabolism in lactating Holstein cows. J Dairy Sci. 2010;93:644–55.
Yunianto VD, Hayashi K, Kaneda S, Ohtsuka A, Tomita Y. Effect of environmental temperature on muscle protein turnover and heat production in tube-fed broiler chickens. Br J Nutr. 1997;77:897–909.
Li Z, McKenna ZJ, Kuennen MR, Magalhães de F C, Mermier CM, Amorim FT. The Potential Role of Exercise-Induced Muscle Damage in Exertional Heat Stroke. Sports Med. 2021;51:863–72.
Ogawa T, Asayama M, Miyagawa T. Effects of sweat gland training by repeated local heating. Jpn J Physiol. 1982;32:971–81.
Patterson MJ, Stocks JM, Taylor NAS. Sustained and generalized extracellular fluid expansion following heat acclimation. J Physiol. 2004;559:327–34.
Rodriguez NR, Di Marco NM, Langley S. American College of Sports Medicine position stand. Nutrition and athletic performance. Med Sci Sports Exerc. 2009;41:709–31.
Thomas DT, Erdman KA, Burke LM. Position of the Academy of Nutrition and Dietetics, Dietitians of Canada, and the American College of Sports Medicine: Nutrition and Athletic Performance. J Acad Nutr Diet. 2016;116:501–28.
McDermott BP, Anderson SA, Armstrong LE, Casa DJ, Cheuvront SN, Cooper L, et al. National Athletic Trainers’ Association position statement: fluid replacement for the physically active. J Athl Train. 2017;52:877–95.
Kerksick CM, Wilborn CD, Roberts MD, Smith-Ryan A, Kleiner SM, Jäger R, et al. ISSN exercise & sports nutrition review update: research & recommendations. J Int Soc Sports Nutr. 2018;15:1–57.
Headquarters, Department of the Army. Technical Bulletin, Medical (TB MED) 507: Heat Stress Control and Heat Casualty Management [Internet]. United States Army; 2022 [cited 2022 Jun 3]. Available from: https://armypubs.army.mil/epubs/DR_pubs/DR_a/ARN35159-TB_MED_507-000-WEB-1.pdf .
Pasiakos SM. Nutritional requirements for sustaining health and performance during exposure to extreme environments. Annu Rev Nutr. 2020;40:221–45.
Armstrong LE, Hubbard RW, Askew EW, De Luca JP, O’Brien C, Pasqualicchio A, et al. Responses to moderate and low sodium diets during exercise-heat acclimation. Int J Sport Nutr. 1993;3:207–21.
Lutz LJ, Gaffney-Stomberg E, Karl JP, Hughes JM, Guerriere KI, McClung JP. Dietary intake in relation to military dietary reference values during army basic combat training; a multi-center, cross-sectional study. Mil Med. 2019;184:e223–30.
U.S. Department of Defense. Natick PAM 30–25: Operational Rations of the Department of Defense, 9th Ed. [Internet]. Combat Feeding Directorate; 2012. Available from: https://api.army.mil/e2/c/downloads/306070.pdf . Accessed 17 July 2024.
Margolis LM, Crombie AP, McClung HL, McGraw SM, Rood JC, Montain SJ, et al. Energy requirements of US Army Special Operation Forces during military training. Nutrients. 2014;6:1945–55.
Steele SR, Mullenix PS, Martin MJ, Place RJ. The effect of combat rations on bowel habits in a combat environment. Am J Surg. 2005;189:518–21 ( discussion 521 ).
Karl JP, Armstrong NJ, McClung HL, Player RA, Rood JC, Racicot K, et al. A diet of U.S. military food rations alters gut microbiota composition and does not increase intestinal permeability. J Nutr Biochem. 2019;72:108217.
Institute of Medicine (US) Committee on Military Nutrition Research. Nutritional Needs in Hot Environments: Applications for Military Personnel in Field Operations [Internet]. Marriott BM, editor. Washington (DC): National Academies Press (US); 1993 [cited 2024 Jul 19]. Available from: http://www.ncbi.nlm.nih.gov/books/NBK236233/ .
Pitsiladis YP, Maughan RJ. The effects of exercise and diet manipulation on the capacity to perform prolonged exercise in the heat and in the cold in trained humans. J Physiol. 1999;517:919–30.
Ihsan M, Périard JD, Racinais S. How to integrate recovery during heat acclimation. Br J Sports Med. 2021;55:185–6.
Coggan AR, Coyle EF. Effect of carbohydrate feedings during high-intensity exercise. J Appl Physiol Bethesda Md. 1985;1988(65):1703–9.
Brenner I, Shek PN, Zamecnik J, Shephard RJ. Stress hormones and the immunological responses to heat and exercise. Int J Sports Med. 1998;19:130–43.
Hanstock HG, Walsh NP, Edwards JP, Fortes MB, Cosby SL, Nugent A, et al. Tear fluid SIgA as a noninvasive biomarker of mucosal immunity and common cold risk. Med Sci Sports Exerc. 2016;48:569–77.
Walsh NP, Oliver SJ. Exercise, immune function and respiratory infection: an update on the influence of training and environmental stress. Immunol Cell Biol. 2016;94:132–9.
Travers G, Nichols D, Riding N, González-Alonso J, Périard JD. Heat acclimation with controlled heart rate: influence of hydration status. Med Sci Sports Exerc. 2020;52:1815–24.
Marquet L-A, Brisswalter J, Louis J, Tiollier E, Burke LM, Hawley JA, et al. Enhanced endurance performance by periodization of carbohydrate intake: “Sleep Low” strategy. Med Sci Sports Exerc. 2016;48:663–72.
Mitchell DC, Cheng FW, Still CD, Jensen GL. A validation of Automated Self-Administered 24-Hour Dietary Recalls (ASA24) relative to interviewer-administered recalls using the Nutrition Data System for Research (NDSR). FASEB J. 2016;30:43.3.
Download references
Acknowledgements
No funds, grants, or support was received.
Author information
Authors and affiliations.
Center for Research & Education in Special Environments, Department of Exercise & Nutrition Sciences, State University of New York University at Buffalo, Buffalo, NY, USA
Paul Rosbrook & J. Luke Pryor
Military Nutrition Division, U.S. Army Research Institute for Environmental Medicine, Natick, MA, USA
Lee M. Margolis
You can also search for this author in PubMed Google Scholar
Corresponding author
Correspondence to Paul Rosbrook .
Ethics declarations
Author contributions.
P.R. conceptualized the topic, performed the literature search, and drafted the article. L.M.M. and J.L.P. provided critical revisions. All authors read and approved the final version.
Competing Interests
The authors declare no competing interests in the current article. The views expressed in this manuscript are those of the authors and do not reflect the official policy of the US Government, Department of Energy, Department of the Army, or Department of Defense.
Data and Code Availability
There are no data associated with this article.
There was no funding received to support this article.
Ethics Approval
Not applicable.
Consent to Participate
Consent for publication, rights and permissions.
Reprints and permissions
About this article
Rosbrook, P., Margolis, L.M. & Pryor, J.L. Nutritional Considerations in Exercise-Based Heat Acclimation: A Narrative Review. Sports Med (2024). https://doi.org/10.1007/s40279-024-02109-x
Download citation
Accepted : 15 August 2024
Published : 31 August 2024
DOI : https://doi.org/10.1007/s40279-024-02109-x
Share this article
Anyone you share the following link with will be able to read this content:
Sorry, a shareable link is not currently available for this article.
Provided by the Springer Nature SharedIt content-sharing initiative
- Find a journal
- Publish with us
- Track your research
- Open access
- Published: 31 August 2024
Optimizing labor duration with pilates: evidence from a systematic review and meta-analysis of randomized controlled trials
- Arezoo Haseli ORCID: orcid.org/0000-0003-1487-633X 1 ,
- Farideh Eghdampour 2 ,
- Hosna Zarei ORCID: orcid.org/0000-0002-3192-624X 3 ,
- Zahra Karimian 4 &
- Dara Rasoal ORCID: orcid.org/0000-0002-0068-943X 5
BMC Pregnancy and Childbirth volume 24 , Article number: 573 ( 2024 ) Cite this article
Metrics details
Pilates has captured interest due to its possible advantages during pregnancy and childbirth. Although research indicates that Pilates may reduce labor duration, alleviate pain, and improve satisfaction with the childbirth experience, consensus on these outcomes remains elusive, underscoring the necessity for additional studies.
This systematic review and meta-analysis aimed to assess the impact of Pilates exercises on labor duration among pregnant women.
The online database was searched to yield the literature using the terms of ‘Pilates’, ‘childbirth’, and ‘labor duration’, and similar terms including PubMed, Clinical Key, Scopus, Web of Science, Embase, and Cochrane Database of Systematic Reviews up to June 25, 2023. Studies were considered eligible if they were randomized or clinical controlled trials (RCTs/CCTs) published in English, focusing on healthy pregnant women without exercise contraindications. The studies needed to include interventions involving Pilates or exercise movement techniques, a comparison group with no exercise, and outcomes related to labor duration, the period of the active phase, and the second stage of delivery.
Eleven studies, totalling 1239 participants, were included in the analysis. These studies provided high-quality evidence from exercise only RCTs/CCTs. The findings indicated a significant reduction in the active phase of labor (8 RCTs, n = 1195; Mean Difference [MD] = -56.35, 95% Confidence Interval [CI] [-89.46 to -23.25]) and overall labor duration (8 RCTs, n = 898; MD = -93.93, 95% CI [-138.34 to -49.51]) in pregnant women who engaged in Pilates exercises compared to those who did not but doesn’t affect on the duration of the second stage of labor (7 RCTs, n = 1135; MD = -0.11, 95% CI [-7.21 to 6.99]).
Conclusions
While this review primarily addresses the effects of Pilates on healthy and low-risk pregnant women, the findings suggest a potential role for Pilates in shortening labor duration. Therefore, engaging in Pilates or similar physical activities is recommended for pregnant women to potentially facilitate a more efficient labor process.
Peer Review reports
Introduction
Pilates is characterized as a distinct form of resistance and strength training [ 1 ], and has been described as a “healing exercise” [ 2 ]. The primary objective of Pilates is to establish muscular strength and flexibility by fortifying weaker muscles and augmenting the elasticity, and preparing the body of a pregnant woman for childbirth and postnatal recovery [ 3 , 4 , 5 ]. Pilates, a form of exercise increasingly popular in health promotion programs worldwide, especially during pregnancy, emerges as a promising activity in this context [ 6 ]. Several studies have investigated the impact of Pilates on various aspects of pregnancy and childbirth [ 3 , 7 ]. Some studies suggest that Pilates may reduce perceived stress during pregnancy, potentially creating mental space for bonding with the unborn child and enhance maternal satisfaction [ 7 , 8 , 9 ]. This exercise method is recommended as a particularly relevant physical activity for healthy pregnant women, owing to its capacity to enhance postural coordination, flexibility, balance, and overall quality of life [ 8 , 10 ]. Moreover, the Pilates method has been used by pregnant women to improve the physical and psychological outcomes of pregnancy, potentially reducing pain such as back pain and pain during labor [ 11 , 12 ]. Additionally, Pilates has been reported to have no negative effects on gestational age, birth weight of infants, and APGAR scores [ 13 ]. However, the comparison of the effects of clinical Pilates exercises with and without childbirth training on pregnancy and birth results did not show any negative impact on the APGAR score [ 14 ].
Contemporary research posits that sedentary women should commence physical activity during pregnancy to mitigate these risks [ 15 ]. At the same time, there has been an increasing focus in recent years on enhancing the childbirth experience and employing techniques to reduce labor duration [ 16 ]. Another aspect that is described from previous studies is the established correlation between protracted labor and heightened risks of mortality, as well as maternal and perinatal complications [ 17 , 18 , 19 , 20 ]. These complications encompass a range of issues, including increased maternal fatigue, the necessity for induction and cesarean section, instrumental delivery, uterine atony, maternal mortality, elevated fetal distress, hypoxia, low apgar scores, and ultimately, fetal demise [ 21 ].
However, the effects of Pilates on childbirth outcomes are still a topic of debate. Some studies have shown that Pilates exercise alone had no significant effect on increasing the rate of natural childbirth in primiparous women [ 9 ]. Similarly, there is no consensus on the effects of Pilates methods with voluntary pelvic floor muscle contractions in pregnant women [ 22 ].
Research has also demonstrated that Pilates exercises applied during pregnancy could improve women’s core stability and balance levels and reduce their fear of childbirth [ 23 ]. While some studies suggest the potential benefits of Pilates on labor duration, there is still a lack of consensus on its overall impact. This systematic review and meta-analysis have been conducted with the objective of elucidating the impact of Pilates exercise on the duration of labor.
This systematic review has been meticulously conducted in alignment with the established protocols and recommendations outlined in the Preferred Reporting Items for Systematic Reviews and Meta-Analyses (PRISMA) guidelines [ 24 ].
Literature search and data collection
This research was conducted through June 2023 using the following search terms: (childbirth OR pregnancy OR pregnant OR labor OR obstetric) AND (Pilates OR physical activity OR exercise OR movement techniques) AND (duration of labor OR length of labor OR length of delivery). The research was carried out using the following databases: PubMed, Clinical Key, Scopus, Web of Science, Embase, and the Cochrane Database of Systematic Reviews. See the supplementary file for detailed search strategies specific to each database.
Studies selection and eligibility criteria
The main inclusion criteria for this systematic review, structured according to the PICOS framework, are as follows:
Population (P): Pregnant women. Intervention (I): Pilates exercises and physical activity. Comparison (C): No activity. Outcomes (O): Duration of labor, active phase duration, second stage duration. Study Design (S): Randomized Controlled Trials (RCT)/Clinical Controlled Trials (CCT).
Additionally, the studies must be; published in a peer-reviewed journal up to June 2023, and written in the English language.
Two authors (A.H. and H.Z.) initially screened the titles and abstracts of all retrieved records, and subsequently, reviewed the full texts independently. In instances of uncertainty regarding whether studies met the inclusion criteria, a third researcher (F.E.) was consulted, and decisions were made by consensus. We excluded articles not published in peer-reviewed journals, encompassing conference papers, theses, dissertations, books, book chapters, and reports from non-peer-reviewed sources, as well as those in languages other than English. Furthermore, we excluded studies that did not utilize a randomized controlled trial (RCT) or clinical controlled trial design. We included all studies published from the earliest date of consideration up to June 25, 2023, to ensure the relevance and timeliness of the evidence gathered. In cases where multiple reports originated from the same study, the most comprehensive report was selected. If the full text of an article was unavailable, the information provided in the abstract was utilized. However, if the abstract did not furnish sufficient information, the article was excluded from the study. Upon completion of the search, the EndNote program was employed to eliminate duplicates. Relevance checks were performed based on both the titles and abstracts, as well as the full texts. Additionally, the references of the included studies were reviewed to identify any potentially missing relevant papers.
Quality assessment
RCTs were included in the evaluation using the Cochrane Risk of Bias tool (version 1). This tool comprises several domains: selection, performance, detection, attrition, reporting, and other biases. The potential for bias in these assessments can be categorized as high, low, or unclear risk of bias. The quality of all included studies was independently assessed by two investigators, AH and HZ. In cases of disagreement, resolutions were sought through consultation with colleagues FA and DR.
Data extraction
The data were extracted into a sheet (Tables 1 and 2 ). The extracted data included the following items: summary characteristics such as author (s), year, sample size, study design, age, body weight, BMI, height, education, gestational age at study entry, session characteristics, and duration of labor. Overall labor duration is defined length of the first and second stages of labor. The first stage of labor is the time elapsed from the beginning of contractions to 10 cm of dilation; which includes the latent phase (the beginning of contractions up to 4 cm of dilation) and the active phase (the time elapsed from 4 to 10 cm. of dilation); the second stage is the time elapsed from full dilation (10 cm of dilation) until fetal expulsion [ 25 ].
Data analysis
Statistical analysis was conducted using Review Manager (RevMan) version 5.4 and STATA version 17. A significance level was set at a P value of less than 0.05. Overall effects were synthesized through the mean and standard deviation of labor length. Heterogeneity among studies was assessed using the I-squared test (I²). If the I² value exceeded 50%, outlier studies were first removed to homogenize the study sample. When heterogeneity persisted, random-effects models were utilized for the analysis instead of fixed-effect models. Publication bias was assessed using Egger’s test.
Equity, diversity, and inclusion statement
Our research team is dedicated to promoting diversity, equity, and inclusion in clinical practice, research, and training programs. Accordingly, the processes of data extraction, study selection, quality assessment, analysis, and interpretation were conducted independently to ensure results that accurately reflect the most diverse and realistic picture possible. We incorporated data from all primary research worldwide that met the inclusion criteria, without bias towards race, ethnicity, culture, socioeconomic status, or geographical regions.
Figure 1 presents a chart detailing the flow of studies through this meta-analysis. In total, 330 records were screened, comprising 316 studies identified through database searches and 14 studies identified through other sources. After the removal of duplicates (24 studies), 290 studies did not meet the inclusion criteria and were subsequently excluded. The remaining 16 articles were retrieved in full for further examination. Of these, eleven studies met the inclusion criteria. The remaining five studies were excluded for the following reasons: two were cohort studies, one was a secondary publication, and two studies did not report the effect size.

PRISMA flow diagram of the screening procedure
The quality assessment (risk of bias)
The authors have meticulously noted and classified the biases associated with the research methodologies into three distinct categories: low risk, high risk, and unclear risk. High Risk : Aktan [ 14 ], Ferreira [ 26 ], Gehan [ 27 ], Perales [ 28 ], and Mazzarino [ 29 ] were categorized as high risk due to methodological limitations, potential biases, and data reliability issues.
Barakat [ 30 ], Bolanthakodi [ 31 ] and Haakstad [ 32 ] exhibited robust methodology, adequate sample sizes, and clear results, justifying their low-risk categorization.
Unclear risk
Ghandali [ 9 ], Rodriguez-Blanque [ 33 ] and Price [ 34 ] was categorized as uncertain due to ambiguities in its methodology and potential unaddressed confounding factors. This classification adheres to the criteria set forth by the Cochrane Collaboration tool. Detailed insights into this categorization, along with an exhaustive breakdown of the types and instances of bias present in the study methodologies, are comprehensively illustrated in Fig. 2 .

Risk of bias graph for studies
Study characteristics
Relevant information from the 11 selected samples is concisely summarized in Table 2 . The meta-analytic sample comprised a total of 1239 participants, with 602 in the intervention group and 637 in the control group. The mean age of the studied participants ranged from 23.4 years [ 31 ] to 36.8 years [ 35 ]. Three studies were conducted in Spain [ 28 , 30 , 33 ], while the remaining were carried out in Turkey [ 14 ], Australia [ 29 ], Norway [ 32 ], Iran [ 9 ], Brazil [ 26 ], the USA [ 34 ], India [ 31 ], and Egypt [ 35 ]. All study designs were Randomized Control Trials, except for two studies which were Clinical Control Trials [ 26 , 35 ]. The number of exercise sessions in the studies varied from 7 [ 31 ] to 96 [ 34 ]. Some studies reported only weight [ 32 ], or body mass index (BMI) [ 9 , 26 , 28 , 30 , 34 , 35 ], while others included all of metrics (weight, height, and BMI) [ 14 , 29 , 31 , 33 ]. Three studies have presented only the duration of labor [ 14 , 29 , 33 ], while other studies have reported the duration of the active phase, the second stage, and/ or the duration of labor separately [ 9 , 26 , 28 , 30 , 31 , 32 , 34 , 35 ] (Tables 1 and 2 ). No studies reported an increased risk of adverse birth outcomes from Pilates exercises among previously inactive, healthy women.
Quantitative data synthesis
The pooled effect size of Pilates exercises indicates a significant reduction in duration of the active phase (Mean Difference [MD] = -56.35, 95% Confidence Interval [CI] [-89.46 to -23.25], p < 0.001) with a sample size of 1195.
The mean difference in active phase of labour (− 56.35) means that Pilates reduces the duration of active phase of labor by 56.35 min in the intervention group compared to the control group. The analysed data were heterogeneous (I² = 60.96%), hence random-effects models were utilized for the analysis, but the heterogeneity could not be fully resolved (see Fig. 3 A).
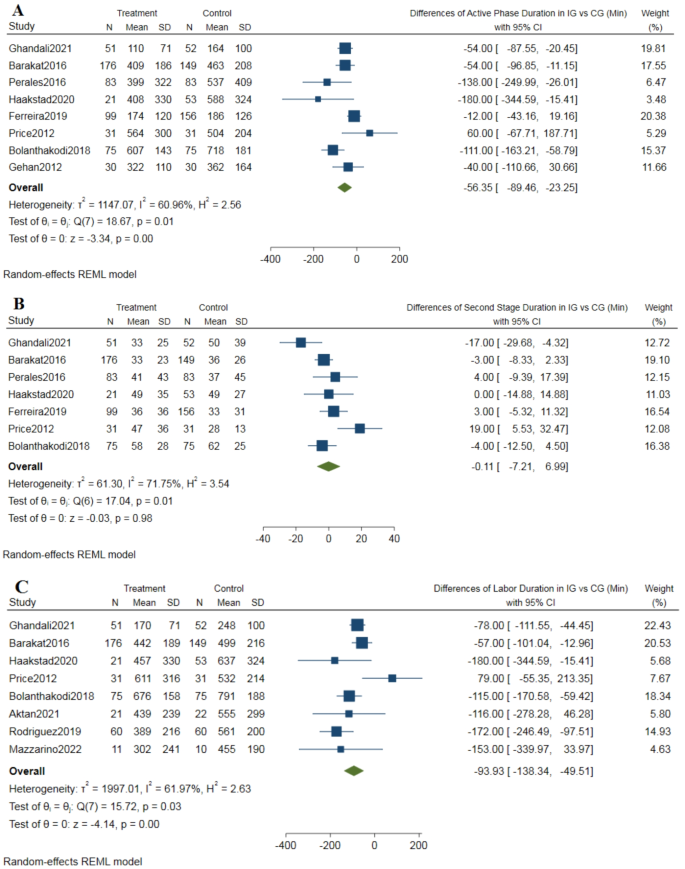
A ; Forest plot of duration of the active phase of labor, B ; Forest plot of duration of the second stage of labor, C ; Forest plot of duration of labor (sum of active phase and second stage)
The Pilates exercises didn’t decrease the duration of the second stage of labor (MD = -0.11, 95% CI [-7.21 to 6.99], P value = 0.98), in a sample of 1135 pregnant women. The pooled estimate was homogeneous (I² = 71.75%, using a random-effect model) (refer to Fig. 3 B).
Eight articles investigated the overall duration of labor (sample size = 898 pregnant women). The data indicated that the Pilates group experienced a shorter duration of labor compared to the control group (MD = -93.93, 95% CI [-138.34 to -49.51], P value = 0.001). The results showed heterogeneity (I² = 61.97%), and this heterogeneity could not be resolved (refer to Fig. 3 C).
Assessment of publication bias
We did not observe publication bias for the study in any of the variables studied. The estimated bias coefficient of labor duration was − 0.29 (Egger bias B = − 0.30 (95% CI: − 2.66–2.08) with a standard error of 0.97, giving a p -value of 0.77. Thus, the test provides no evidence for the presence of small-study effect. Thus, the test provides no evidence for the presence of small-study effect. Figure 4 presents the funnel plot result with the 95% confidence limit. The estimated bias coefficient of active phase duration was − 0.85 (Egger bias B = − 0.88 (95% CI: − 3.19–1.50) with a standard error of 0.96, giving a p -value of 0.41 and it was 1.17 (Egger bias B = 0.50 (95% CI: − 4.82–7.16) with a standard error of 2.33 ( p -value = 0.64) for second stage of labor.
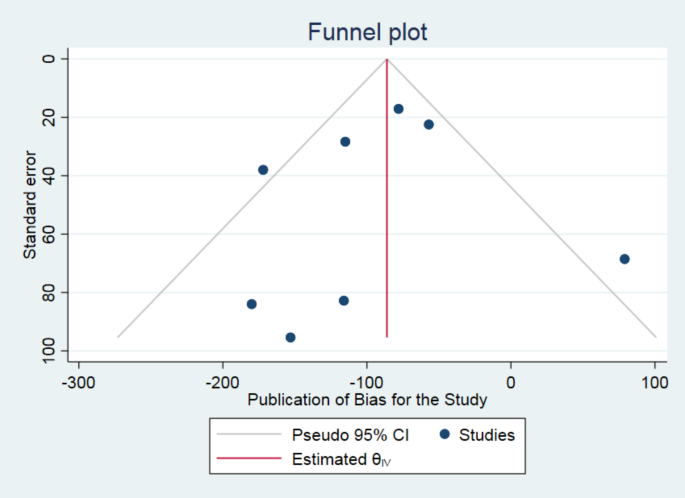
Presentation of funnel plot
The present study aimed to systematically review the literature regarding the efficacy of Pilates in reducing labor length. This meta-analysis was conducted using optimal methods for secondary analysis and included 11 primary studies, all of which were clinical trials. The analytical results indicate that Pilates exercises can significantly shorten the overall duration of labor, including both the active phase and the second stage.
The meta-analysis revealed a notable difference between the Pilates exercise group and control group in the duration of the first stage of labor, despite contradictory findings in the primary studies. The disparity in results from these primary studies could be attributed to variations in sample sizes (ranging from 60 to 325) and the timing of intervention initiation (between 12 and 39 weeks’ gestation). A detailed examination of the primary studies revealed that Pilates exercises were generally more effective in studies characterized by longer durations, higher frequency, and earlier commencement of the intervention [ 28 ]. Therefore, under these specific conditions, Pilates appears to strengthen pelvic muscles, increase pelvic diameter, relax muscles, and consequently enhance the condition of the birth canal parts. This, in turn, contributes to the shortening of the active phase of labor [ 29].
The findings of this study demonstrate that Pilates doesn’t affect the duration of the second stage of labor. This conclusion confirm with some earlier studies which indicated no significant difference in second stage’s length between women who practiced Pilates and those who did not [ 14 ]. It seems that the length of the second stage of labor, which is defined from the dilatation of the cervix to the expulsion of the fetus, is more influenced by the cephalo-pelvic proportion, birth weight, the strength of the mother’s perineal tissue, and station at complete dilatation [ 36 ] as well as differences in obstetric population characteristics and variation in clinical practice [ 37 ].
In this study, Pilates was observed to reduce the overall length of labor. Supporting this finding, the outcomes of several meta-analyses have underscored the positive impact of exercise programs on facilitating the childbirth process, particularly in shortening the duration of delivery [ 38 ]. However, there exists a divergence of opinion among researchers on this matter. For instance, the findings of two separate meta-analyses suggest no significant association between the regularity, intensity, and duration of exercise and the length of childbirth [ 39 , 40 ]. This discrepancy may be attributable to the type of exercise. Veisy et al., for example, focused on the influence of aerobic exercise on labor duration [ 39 ]. Contrastingly, Pilates, a non-aerobic form of exercise, is primarily recognized for its capacity to enhance physical, mental, and motor functions [ 15 ].
This form of exercise encompasses a series of low-impact routines designed to enhance strength and flexibility across the body. Regular participation in these exercises has been demonstrated to fortify the pelvic floor muscles and improve their structural functionality [ 41 ]. The enhancement of central and pelvic floor muscle strength, flexibility, and the adoption of proper breathing techniques through Pilates are known to facilitate the birthing process [ 9 , 42 ]. Additionally, Pilates, initially conceptualized as a body conditioning method termed ‘Contrology’, is founded on six key principles: breath, centering, concentration, control, precision, and flow [ 43 ]. Individual investigations into each of these elements have shown that they collectively contribute to a reduction in the duration of childbirth [ 44 , 45 ].
Notably, the impact of Pilates exercise on labor duration has not been previously analyzed in a systematic review or meta-analysis with a sample size of this scale. The breadth of studies and the substantial number of participants involved in this analysis afford some of the most valid and reliable conclusions that can be drawn on this subject.
Clinical implications
Considering that prolonged labor presents a significant clinical challenge in contemporary midwifery practice, leading to various complications for both mother and child, one effective strategy to enhance labor comfort is the reduction of labor duration, specifically through methods that alleviate the duration of pain. The findings of this review indicate that practicing Pilates during pregnancy serves as a viable approach to decreasing labor length. Pilates, characterized as a discipline that bolsters physical, mental, and motor capacities, comprises a series of low-impact exercises aimed at strengthening and increasing flexibility throughout the body [ 15 ]. Analysis of primary studies reveals that Pilates can be reduced labor duration.
To date, there have been no reports of risks associated with moderate-intensity exercise for either the mother or the infant [ 46 ]. In light of this, low-risk pregnant women are encouraged to engage in progressive aerobic and resistance exercises before, during, and after childbirth. Nevertheless, it is advisable for pregnant women to undergo clinical evaluation before initiating any exercise program to ensure there are no medical contraindications to continuing with the exercise [ 47 ]. Engaging in Pilates exercises of moderate intensity during pregnancy has been shown to facilitate the delivery process.
Our findings endorse the capability of Pilates exercise to reduce the duration of labor in pregnant women. Therefore, Pilates could potentially be incorporated into childbirth preparation classes as a beneficial practice.
Limitations
This study encountered several limitations, both at the level of the individual studies and the review itself. A notable limitation within the primary studies was the lack of reported data on the intensity of the Pilates exercises. Additionally, variations in the initiation timing and duration of exercise sessions may have influenced the results of the analysis. Another limitation at the study level was the heterogeneity of the samples. For instance, while some studies had exercises performed under professional supervision, others involved participants practicing at home without such oversight. Moreover, the participant demographics varied across studies, with many focusing on primiparous women, while others included both multiparous and primiparous participants. This variability, particularly in terms of parity, was not accounted for as a potential confounding factor, potentially contributing to the high heterogeneity observed in the meta-analyses. Consequently, the results of this study should be interpreted with caution.
At the review level, the mean effect size of the active phase period and labour duration was analysed using a random-effects model due to the heterogeneity of the studies. Furthermore, this review was limited to scientific papers published in English, representing another constraint on its scope.
The evidence presented herein underscores that engaging in Pilates exercises during pregnancy is not only safe but also beneficial in optimizing the delivery process and shortening labor duration. Consistent with the guidelines of the American College of Obstetricians and Gynecologists (ACOG), this study reinforces the recommendation that, despite physiological and anatomical changes during pregnancy, women should be encouraged to maintain physical activity. Pilates, as demonstrated, can shorten length of labor. It is recommended that midwives emphasize the use of this exercise in childbirth preparation classes to reduce the duration of childbirth.
Data availability
Data is provided within the manuscript or supplementary information files.
Doolan-Roy ED, Reagan K, Modisette M, Mattes LL. Return to Sport following anterior Cruciate Ligament Reconstruction in the Professional Female Ballet Dancer. J Women’s Sports Med. 2022;2:83–94.
Article Google Scholar
Levine B, Kaplanek B, Jaffe WL. Pilates training for Use in Rehabilitation after total hip and knee arthroplasty: a preliminary Report. Clin Orthop Relat Res. 2009;467:1468–75.
Article PubMed PubMed Central Google Scholar
Cruz-Ferreira A, Fernandes J, Laranjo L, Bernardo LM, Silva A. A systematic review of the effects of pilates method of exercise in healthy people. Arch Phys Med Rehabil. 2011;92:2071–81.
Article PubMed Google Scholar
Navas A, Artigues C, Leiva A, Portells E, Soler A, Cladera A, et al. Effectiveness and safety of moderate-intensity aerobic water exercise during pregnancy for reducing use of epidural analgesia during labor: protocol for a randomized clinical trial. BMC Pregnancy Childbirth. 2018;18:94.
King M, Green Y. Pilates workbook for pregnancy: Illustrated Step-by-step Matwork techniques. Berkerley, Canada: Ulysses; 2002.
Google Scholar
Mazzarino M, Kerr D, Wajswelner H, Morris ME. Pilates Method for Women’s Health: systematic review of Randomized controlled trials. Arch Phys Med Rehabil. 2015;96:2231–42.
Cuijlits I, van de Wetering A p., Endendijk Jj, van Baar A l., Potharst E. s., Pop V j. m. Risk and protective factors for pre- and postnatal bonding. Infant Mental Health Journal. 2019;40:768–85.
Hyun A-H, Cho J-Y, Koo J-H. Effect of home-based Tele-Pilates intervention on pregnant women: a pilot study. Healthcare. 2022;10:125.
Ghandali NY, Iravani M, Habibi A, Cheraghian B. The effectiveness of a pilates exercise program during pregnancy on childbirth outcomes: a randomised controlled clinical trial. BMC Pregnancy Childbirth. 2021;21:480.
Aladro-Gonzalvo AR, Machado-Díaz M, Moncada-Jiménez J, Hernández-Elizondo J, Araya-Vargas G. The effect of Pilates exercises on body composition: a systematic review. J Bodyw Mov Ther. 2012;16:109–14.
Feria-Ramírez C, Gonzalez-Sanz JD, Molina-Luque R, Molina-Recio G. The effects of the Pilates Method on pelvic floor injuries during pregnancy and childbirth: a quasi-experimental study. Int J Environ Res Public Health. 2021;18:6995.
Sukmawati S, Mamuroh L, Nurhakim F. Efektivitas Pilates Exercise untuk Mengurangi Nyeri pada Ibu Hamil. Malahayati Nurs J. 2023;5:2351–69.
Yaman S, Tulmaç ÖB, Canarslan B, Hançerli̇oğullari N. Effect of pilates during pregnancy on delivery outcomes. J Health Sci Med / JHSM. 2020;3:442–7.
Aktan B, Kayıkçıoğlu F, Akbayrak T. The comparison of the effects of clinical Pilates exercises with and without childbirth training on pregnancy and birth results. Int J Clin Pract. 2021;75:e14516.
Mazzarino M, Kerr D, Morris ME. Pilates program design and health benefits for pregnant women: A practitioners’ survey. J Bodyw Mov Ther. 2018;22:411–7.
Ghiasi A, Hasani M, Mollaahmadi L, Hashemzadeh M, Haseli A. The effect of aromatherapy on labor Pain relief: a systematic review of clinical trials. Iran J Obstet Gynecol Infertility. 2017;20:89–105.
Ijaiya MA, Adesina KT, Raji HO, Aboyeji AP, Olatinwo AO, Adeniran AS et al. Duration of labour with spontaneous onset at the University of Ilorin Teaching Hospital, Ilorin, Nigeria. Ann Afr Med. 2011;10.
Ijaiya MA, Aboyeji AP, Fakeye OO, Balogun OR, Nwachukwu DC, Abiodun MO. Pattern of cervical dilatation among parturients in Ilorin, Nigeria. Ann Afr Med. 2009;8.
MELAH G, EL-NAFATY A, MASSA A. Obstructed labour: a public health problem in Gombe, Gombe State, Nigeria. J Obstet Gynaecol. 2003;23:369–73.
Article PubMed CAS Google Scholar
Piper JM, Bolling DR, Newton ER. The second stage of labor: factors influencing duration. Am J Obstet Gynecol. 1991;165:976–9.
Cheng YW, Hopkins LM, Laros RK, Caughey AB. Duration of the second stage of labor in multiparous women: maternal and neonatal outcomes. American Journal of Obstetrics and Gynecology. 2007;196:585.e1-585.e6.
Dias NT, Ferreira LR, Fernandes MG, Resende APM, Pereira-Baldon VS. A pilates exercise program with pelvic floor muscle contraction: is it effective for pregnant women? A randomized controlled trial. Neurourol Urodyn. 2018;37:379–84.
Bulguroğlu Hİ, Bulguroğlu M, Özkul Ç, Gündüz AG. Investigation of the Effect of Pilates exercises on Postural Stability and fear of birth in pregnancy. Adnan Menderes Üniversitesi Sağlık Bilimleri Fakültesi Dergisi. 2023;7:530–40.
Page MJ, Moher D. Evaluations of the uptake and impact of the Preferred reporting items for systematic reviews and Meta-analyses (PRISMA) Statement and extensions: a scoping review. Syst Reviews. 2017;6:263.
Zhang J, Troendle J, Mikolajczyk R, Sundaram R, Beaver J, Fraser W. The natural history of the Normal First Stage of Labor. Obstet Gynecol. 2010;115:705.
Ferreira CLM, Guerra CML, Silva AITJ, Rosário HRV. do, Pereira MBFL de O. Exercise in Pregnancy: The Impact of an Intervention Program in the Duration of Labor and Mode of Delivery. Rev Bras Ginecol Obstet. 2019;41:68–75.
Gehan A, Khadiga S, Amir G, Eman A. Efficacy of antenatal exercises on maternal and neonatal outcomes in elderly primigravida. 2015;3:109–14.
Perales M, Calabria I, Lopez C, Franco E, Coteron J, Barakat R. Regular Exercise throughout pregnancy is Associated with a shorter first stage of labor. Am J Health Promot. 2016;30:149–57.
Mazzarino M, Kerr D, Morris ME. Feasibility of pilates for pregnant women: a randomised trial. J Bodyw Mov Ther. 2022;32:207–12.
Barakat R, Franco E, Perales M, López C, Mottola MF. Exercise during pregnancy is associated with a shorter duration of labor. A randomized clinical trial. Eur J Obstet Gynecol Reproductive Biology. 2018;224:33–40.
Bolanthakodi C, Raghunandan C, Saili A, Mondal S, Saxena P. Prenatal yoga: effects on Alleviation of Labor Pain and Birth outcomes. J Altern Complement Med. 2018;24:1181–8.
Haakstad LAH, Bø K. The marathon of labour—does regular exercise training influence course of labour and mode of delivery? Secondary analysis from a randomized controlled trial. Eur J Obstet Gynecol Reproductive Biology. 2020;251:8–13.
Rodríguez-Blanque R, Sánchez-García JC, Sánchez-López AM, Aguilar-Cordero MJ. Physical activity during pregnancy and its influence on delivery time: a randomized clinical trial. PeerJ. 2019;7:e6370.
Price BB, Amini SB, Kappeler K. Exercise in pregnancy: effect on fitness and obstetric outcomes-a randomized trial. Med Sci Sports Exerc. 2012;44:2263–9.
Gehan. Efficacy of antenatal exercises on maternal and neonatal outcomes in elderly primigravida. Kasr Al Ainy Med J. 2012;21:109.
Altman MR, Lydon-Rochelle MT. Prolonged second stage of labor and risk of adverse maternal and perinatal outcomes: a systematic review. Birth. 2006;33:315–22.
Cheng YW, Caughey AB. Second stage of labor. Clin Obstet Gynecol. 2015;58:227.
Cancela-Carral JM, Blanco B, López-Rodríguez A. Therapeutic aquatic Exercise in pregnancy: a systematic review and Meta-analysis. J Clin Med. 2022;11:501.
Veisy A, Mohammad Alizadeh Charandabi S, Hematzadeh S, Mirghafourvand M. Effect of prenatal aerobic exercises on maternal and neonatal outcomes: a systematic review and meta-analysis. Nurs Open. 2021;8:2301–17.
Davenport MH, Meah VL, Ruchat S-M, Davies GA, Skow RJ, Barrowman N, et al. Impact of prenatal exercise on neonatal and childhood outcomes: a systematic review and meta-analysis. Br J Sports Med. 2018;52:1386–96.
Torelli L, de Bella J, Rodrigues ZIK, Stüpp CA, Girão L, Sartori MJBC. Effectiveness of adding voluntary pelvic floor muscle contraction to a pilates exercise program: an assessor-masked randomized controlled trial. Int Urogynecol J. 2016;27:1743–52.
Artal R, O’Toole M. Guidelines of the American College of obstetricians and gynecologists for exercise during pregnancy and the postpartum period. Br J Sports Med. 2003;37:6–12.
Article PubMed PubMed Central CAS Google Scholar
Pilates JH, Miller WJ. Return to Life through Contrology. Ravenio Books; 1945.
Haseli A, Ghiasi A, Hashemzadeh M. Do breathing techniques enhance the Effect of Massage Therapy in reducing the length of Labor or not? A Randomized Clinical Trial. J Caring Sci. 2019;8:257–63.
Haseli A, Akbari M, Neisani Samani L, Haghani H, Jahdi F. The effect of abdominal massage along with breathing techniques during labor on duration of uterine contractions in primiparous women. Iran J Obstet Gynecol Infertility. 2017;20:1–8.
Davies G, La W, Mf M, C M, My A. Exercise in pregnancy and the postpartum period. J Obstet Gynaecol Can. 2003;25:516–29.
American College of Obstetricians and Gynecologists. Exercise during pregnancy and the postpartum period. Clin Obstet Gynecol. 2003;46:496–9.
Download references
Acknowledgements
We acknowledge all authors who contributed to the primary studies. This study is the result of research project No. 4020044, approved by the Student Research Committee at Kermanshah University of Medical Sciences, Kermanshah, Iran. Our gratitude extends to the officials of the Clinical Research Development Center at Motazedi Hospital, Kermanshah University of Medical Sciences, the Department of Midwifery at Kashan University of Medical Sciences, and the School of Health and Welfare at Dalarna University, Sweden, for their invaluable support and contributions.
The present study was supported by the Student Research Committee, Kermanshah University of Medical Sciences. Kermanshah, Iran (Grant number: 4020044).
Open access funding provided by Dalarna University.
Author information
Authors and affiliations.
Family Health and Population Growth Research Center, Health Institute, Kermanshah University of Medical Sciences, Kermanshah, Iran
Arezoo Haseli
Department of Midwifery, Islamic Azad University, Marand, Iran
Farideh Eghdampour
Student Research Committee, Kermanshah University of Medical Sciences, Kermanshah, Iran
Hosna Zarei
Department of Midwifery, Nursing and Midwifery Faculty, Kashan University of Medical Sciences, Kashan, Iran
Zahra Karimian
School of Health and Welfare, Dalarna University, Högskolegatan 2, Falun, 79188, Sweden
Dara Rasoal
You can also search for this author in PubMed Google Scholar
Contributions
AH and HZ designed the search strategy and selection criteria. They screened the eligibility of the articles, reviewed them independently, assessed the quality of the articles, and constructed the tables and figures. FE and DR, as the third and fifth co-authors, checked for contradictions and drafted the results and discussion sections. ZK conducted new and updated statistical analyses during the revisions to the journal. AH wrote the first draft of the manuscript, while DR edited and proofread the entire manuscript. Finally, FE also proofread the manuscript and provided comments. All authors read and approved the final version of the manuscript.
Corresponding author
Correspondence to Dara Rasoal .
Ethics declarations
Ethics approval and consent to participate.
This study was a research project approved by the Ethics Committee of the Research Deputy at Kermanshah University of Medical Sciences in Kermanshah (approval code: IR. KUMS. REC.1401.583).
Consent for publication
Competing interests.
The authors declare no competing interests.
Additional information
Publisher’s note.
Springer Nature remains neutral with regard to jurisdictional claims in published maps and institutional affiliations.
Electronic supplementary material
Below is the link to the electronic supplementary material.
Supplementary Material 1
Supplementary material 2, rights and permissions.
Open Access This article is licensed under a Creative Commons Attribution 4.0 International License, which permits use, sharing, adaptation, distribution and reproduction in any medium or format, as long as you give appropriate credit to the original author(s) and the source, provide a link to the Creative Commons licence, and indicate if changes were made. The images or other third party material in this article are included in the article’s Creative Commons licence, unless indicated otherwise in a credit line to the material. If material is not included in the article’s Creative Commons licence and your intended use is not permitted by statutory regulation or exceeds the permitted use, you will need to obtain permission directly from the copyright holder. To view a copy of this licence, visit http://creativecommons.org/licenses/by/4.0/ .
Reprints and permissions
About this article
Cite this article.
Haseli, A., Eghdampour, F., Zarei, H. et al. Optimizing labor duration with pilates: evidence from a systematic review and meta-analysis of randomized controlled trials. BMC Pregnancy Childbirth 24 , 573 (2024). https://doi.org/10.1186/s12884-024-06785-5
Download citation
Received : 10 December 2023
Accepted : 26 August 2024
Published : 31 August 2024
DOI : https://doi.org/10.1186/s12884-024-06785-5
Share this article
Anyone you share the following link with will be able to read this content:
Sorry, a shareable link is not currently available for this article.
Provided by the Springer Nature SharedIt content-sharing initiative
- Physical activity
- Meta-analysis
BMC Pregnancy and Childbirth
ISSN: 1471-2393
- Submission enquiries: [email protected]
- General enquiries: [email protected]

An official website of the United States government
The .gov means it’s official. Federal government websites often end in .gov or .mil. Before sharing sensitive information, make sure you’re on a federal government site.
The site is secure. The https:// ensures that you are connecting to the official website and that any information you provide is encrypted and transmitted securely.
- Publications
- Account settings
- My Bibliography
- Collections
- Citation manager
Save citation to file
Email citation, add to collections.
- Create a new collection
- Add to an existing collection
Add to My Bibliography
Your saved search, create a file for external citation management software, your rss feed.
- Search in PubMed
- Search in NLM Catalog
- Add to Search
Physical exercise and quality of life following cancer diagnosis: a literature review
Affiliation.
- 1 Faculty of Physical Education, University of Alberta, Edmonton, Canada.
- PMID: 10499138
- DOI: 10.1007/BF02908298
With almost 8 million Americans alive today who have been through the cancer experience, it is important to develop interventions to maintain quality of life (QOL) following cancer diagnosis. Physical exercise is an intervention that may address the broad range of QOL issues following cancer diagnosis including physical, functional, psychological, emotional, and social well-being. The purpose of the present article was to provide a comprehensive and critical review of the topic and to offer suggestions for future research. The review located 24 empirical studies published between 1980 and 1997. Eighteen of the studies were interventions (i.e. quasi-experimental or experimental) but most of these were preliminary efficacy studies that suffered from the common limitations of such designs. Overall, however, the studies have consistently demonstrated that physical exercise has a positive effect on QOL following cancer diagnosis, including physical and functional well-being (e.g. functional capacity, muscular strength, body composition, nausea, fatigue) and psychological and emotional well-being (e.g. personality functioning, mood states, self-esteem, and QOL). Besides overcoming the limitations of past research, recommendations for future research included: (a) extending the research beyond breast and early-stage cancers; (b) comparing and integrating physical exercise with other QOL interventions; (c) examining resistance exercises, the timing of the intervention, and contextual factors; (d) expanding the breadth of the QOL indicators examined; and (e) investigating the rates and determinants of recruitment and adherence to an exercise program following cancer diagnosis.
PubMed Disclaimer
Similar articles
- Resistance exercise interventions during and following cancer treatment: a systematic review. Focht BC, Clinton SK, Devor ST, Garver MJ, Lucas AR, Thomas-Ahner JM, Grainger E. Focht BC, et al. J Support Oncol. 2013 Jun;11(2):45-60. J Support Oncol. 2013. PMID: 23967493 Review.
- The development of an evidence-based physical self-management rehabilitation programme for cancer survivors. van Weert E, Hoekstra-Weebers JE, May AM, Korstjens I, Ros WJ, van der Schans CP. van Weert E, et al. Patient Educ Couns. 2008 May;71(2):169-90. doi: 10.1016/j.pec.2007.11.027. Epub 2008 Feb 5. Patient Educ Couns. 2008. PMID: 18255249 Review.
- Mediators of physical exercise for improvement in cancer survivors' quality of life. Buffart LM, Ros WJ, Chinapaw MJ, Brug J, Knol DL, Korstjens I, van Weert E, Mesters I, van den Borne B, Hoekstra-Weebers JE, May AM. Buffart LM, et al. Psychooncology. 2014 Mar;23(3):330-8. doi: 10.1002/pon.3428. Epub 2013 Oct 14. Psychooncology. 2014. PMID: 24123482 Clinical Trial.
- Comparison of the effects of a supervised exercise program and usual care in patients with colorectal cancer undergoing chemotherapy. Lin KY, Shun SC, Lai YH, Liang JT, Tsauo JY. Lin KY, et al. Cancer Nurs. 2014 Mar-Apr;37(2):E21-9. doi: 10.1097/NCC.0b013e3182791097. Cancer Nurs. 2014. PMID: 23357886
- Effects of aerobic and resistance exercise in breast cancer patients receiving adjuvant chemotherapy: a multicenter randomized controlled trial. Courneya KS, Segal RJ, Mackey JR, Gelmon K, Reid RD, Friedenreich CM, Ladha AB, Proulx C, Vallance JK, Lane K, Yasui Y, McKenzie DC. Courneya KS, et al. J Clin Oncol. 2007 Oct 1;25(28):4396-404. doi: 10.1200/JCO.2006.08.2024. Epub 2007 Sep 4. J Clin Oncol. 2007. PMID: 17785708 Clinical Trial.
- Sex differences of the association between handgrip strength and health-related quality of life among patients with cancer. Kim J, Kim Y, Oh JW, Lee S. Kim J, et al. Sci Rep. 2024 Apr 30;14(1):9876. doi: 10.1038/s41598-024-60710-6. Sci Rep. 2024. PMID: 38684776 Free PMC article.
- Results of an Inpatient Preventive Health Care Program to Improve Quality of Life, Psychosocial Health, and Work Ability in Austria. Thauerer B, Püspök J, Kullich W, Felder D, Steinecker-Frohnwieser B, Skoumal M. Thauerer B, et al. Int J Public Health. 2023 Sep 15;68:1606193. doi: 10.3389/ijph.2023.1606193. eCollection 2023. Int J Public Health. 2023. PMID: 37780133 Free PMC article.
- Comparison of the effects of high-intensity interval and moderate-intensity continuous training on inflammatory markers, cardiorespiratory fitness, and quality of life in breast cancer patients. Isanejad A, Nazari S, Gharib B, Motlagh AG. Isanejad A, et al. J Sport Health Sci. 2023 Nov;12(6):674-689. doi: 10.1016/j.jshs.2023.07.001. Epub 2023 Jul 8. J Sport Health Sci. 2023. PMID: 37423313 Free PMC article. Clinical Trial.
- Effects of Exercise Programs on Psychoemotional and Quality-of-Life Factors in Adult Patients with Cancer and Hematopoietic Stem Cell Transplantation or Bone Marrow Transplantation: A Systematic Review. Morales Rodríguez E, Lorenzo Calvo J, Granado-Peinado M, Pérez-Bilbao T, San Juan AF. Morales Rodríguez E, et al. Int J Environ Res Public Health. 2022 Nov 29;19(23):15896. doi: 10.3390/ijerph192315896. Int J Environ Res Public Health. 2022. PMID: 36497971 Free PMC article. Review.
- Recreational Running Motivations among Breast Cancer Survivors. Malchrowicz-Mośko E. Malchrowicz-Mośko E. Int J Environ Res Public Health. 2022 Nov 23;19(23):15500. doi: 10.3390/ijerph192315500. Int J Environ Res Public Health. 2022. PMID: 36497576 Free PMC article.
Publication types
- Search in MeSH
Related information
- Cited in Books
LinkOut - more resources
Full text sources.
- Ovid Technologies, Inc.
- Silverchair Information Systems
- MedlinePlus Consumer Health Information
- MedlinePlus Health Information
Miscellaneous
- NCI CPTAC Assay Portal
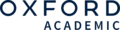
- Citation Manager
NCBI Literature Resources
MeSH PMC Bookshelf Disclaimer
The PubMed wordmark and PubMed logo are registered trademarks of the U.S. Department of Health and Human Services (HHS). Unauthorized use of these marks is strictly prohibited.
Information
- Author Services
Initiatives
You are accessing a machine-readable page. In order to be human-readable, please install an RSS reader.
All articles published by MDPI are made immediately available worldwide under an open access license. No special permission is required to reuse all or part of the article published by MDPI, including figures and tables. For articles published under an open access Creative Common CC BY license, any part of the article may be reused without permission provided that the original article is clearly cited. For more information, please refer to https://www.mdpi.com/openaccess .
Feature papers represent the most advanced research with significant potential for high impact in the field. A Feature Paper should be a substantial original Article that involves several techniques or approaches, provides an outlook for future research directions and describes possible research applications.
Feature papers are submitted upon individual invitation or recommendation by the scientific editors and must receive positive feedback from the reviewers.
Editor’s Choice articles are based on recommendations by the scientific editors of MDPI journals from around the world. Editors select a small number of articles recently published in the journal that they believe will be particularly interesting to readers, or important in the respective research area. The aim is to provide a snapshot of some of the most exciting work published in the various research areas of the journal.
Original Submission Date Received: .
- Active Journals
- Find a Journal
- Proceedings Series
- For Authors
- For Reviewers
- For Editors
- For Librarians
- For Publishers
- For Societies
- For Conference Organizers
- Open Access Policy
- Institutional Open Access Program
- Special Issues Guidelines
- Editorial Process
- Research and Publication Ethics
- Article Processing Charges
- Testimonials
- Preprints.org
- SciProfiles
- Encyclopedia
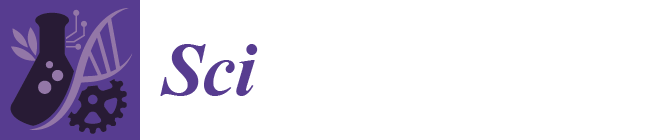
Article Menu

- Subscribe SciFeed
- Recommended Articles
- Google Scholar
- on Google Scholar
- Table of Contents
Find support for a specific problem in the support section of our website.
Please let us know what you think of our products and services.
Visit our dedicated information section to learn more about MDPI.
JSmol Viewer
Fortifying industry 4.0: internet of things security in cloud manufacturing through artificial intelligence and provenance blockchain—a thematic literature review.
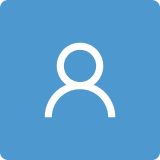
1. Introduction
1.1. rationale, 1.2. objectives.
- Deeper analysis and synthesis: To perform an in-depth analysis and synthesis of existing studies, identify gaps and areas requiring further research, and assess the significance of the existing research in addressing these gaps;
- Integration of findings: To present an informative framework by integrating the findings, providing valuable insights;
- Discussion on challenges and limitations: To justify the feasibility and challenges of the integrated framework and provide valuable insights for future research and implementation by discussing the limitations and potential challenges associated with the framework.
1.3. Research Questions
2. methodology, 2.1. search strategy.
- Category 1: Keywords related to cloud manufacturing, including “Industry 4.0”, “security risks”, “cloud manufacturing”, “design approaches”, and “design vulnerabilities”;
- Category 2: Keywords related to cybersecurity risks to the IoT, including “cybersecurity vulnerabilities”, “Internet of Things”, “cloud-based manufacturing”, “IoT security threats”, and “cloud manufacturing”;
- Category 3: Keywords related to provenance blockchain, including “blockchain technology”, “IoT security”, “data provenance”, “authentication”, and “threat mitigation”;
- Category 4: Keywords related to AI, including “Internet of Things”, “IoT security”, “predictive analytics”, “artificial intelligence”, “security measures”, and “future directions”.
2.2. Study Selection Process
- The cloud manufacturing design and specific aspects causing exposure to cybersecurity should be covered;
- Articles should comprehensively address the cybersecurity risks associated with IoT devices in cloud manufacturing;
- Blockchain security for IoT devices should be covered with some proposed models, at least theoretically;
- Articles should be related to IoT security in the context of provenance, blockchain, and AI, even if not integrated as a solution;
- AI for IoT security should be comprehensively covered.
- Articles that cover cloud manufacturing design in general terms were excluded;
- Articles that did not cover IoT security risks in the context of cloud manufacturing were excluded;
- Articles that did not discuss models of provenance and blockchain and provided only generic discussion were excluded;
- Articles that did not provide technical details of the role of AI in IoT security were excluded;
- Articles that did not provide technical details of the role of AI in IoT security, albeit providing generic discussion, were excluded.
2.3. Analysis of Data
- Cloud Manufacturing Design: The first theme focuses on the design of cloud manufacturing to establish the research context and identify the problem area;
- Cybersecurity Risks to IoT Devices: The second theme examines cybersecurity risks associated with IoT devices in a cloud manufacturing setting, aiming to establish a comprehensive problem description;
- Provenance and Provenance Blockchains: The third theme explores the concept of provenance and the use of provenance blockchains to enhance security and traceability;
- Artificial Intelligence in IoT Security: The fourth theme investigates the role of artificial intelligence in IoT security.
3.1. Theme 1: Cloud Manufacturing Design
3.2. theme 2: cybersecurity risks to iot used in cloud manufacturing processes, 3.3. theme 3: provenance blockchains for iot security, 3.4. theme 4: artificial intelligence for iot security, 3.5. review of blockchain frameworks.
- R0, R1, R2, and R3 = Organizations collaborating to build the blockchain network for provenance called BNP; R0 is the contracting authority, and others are suppliers;
- R1 has access only to the network configuration CC1; R2 and R3 have access to network configurations CC2 and CC3.
- P1, P2, and P3 = Blockchain peers for the suppliers R1, R2, and R3;
- O = Blockchain contracting authority (owner of the smart contracts);
- C1 and C2: Network channels for network configurations CC1 and CC2, respectively;
- CA0, CA1, CA2, and CA3: Certification authorities of the organisations R0, R1, R2, and R3, respectively;
- S1 and S2: State databases of network configurations CC1 and CC2;
- L1 and L2: Ledgers of network configurations CC1 and CC2;
- A1, A2, and A3: Off-chain cloud manufacturing applications maintained by R1, R2, and R3;
- ProvDB: Provenance database interfacing A, A2, and A3;
- AI: Artificial intelligence.
4. Discussion
4.1. design analysis, 4.2. challenges and limitations associated with iot security in cloud manufacturing, 5. conclusions and recommendations, 5.1. conclusions, 5.2. recommendations, author contributions, institutional review board statement, informed consent statement, data availability statement, acknowledgments, conflicts of interest.
- Ustundag, A.; Cevikcan, E.; Akdil, K.Y.; Ustundag, A.; Cevikcan, E. Maturity and readiness model for industry 4.0 strategy. In Industry 4.0: Managing the Digital Transformation ; Springer International Publishing: Cham, Switzerland, 2018; pp. 61–94. [ Google Scholar ]
- Ustundag, A.; Cevikcan, E.; Ervural, B.C.; Ervural, B. Overview of cyber security in the industry 4.0 era. In Industry 4.0: Managing the Digital Transformation ; Springer International Publishing: Cham, Switzerland, 2018; pp. 267–284. [ Google Scholar ]
- Salkin, C.; Oner, M.; Ustundag, A.; Cevikcan, E. A conceptual framework for Industry 4.0. In Industry 4.0: Managing the Digital Transformation ; Springer International Publishing: Cham, Switzerland, 2018; pp. 3–23. [ Google Scholar ]
- Sobers, R. Cybersecurity Statistics and Trends for 2022 Varonis. 2022. Available online: https://www.varonis.com/blog/cybersecurity-statistics/ (accessed on 4 March 2022).
- Brooks, C. Cybersecurity in 2022—A Fresh Look at Some Very Alarming Stats Forbes. 2022. Available online: https://www.forbes.com/sites/chuckbrooks/2022/01/21/cybersecurity-in-2022--a-fresh-look-at-some-very-alarming-stats/?sh=53d0641b6b61 (accessed on 4 March 2023).
- DiMase, D.; Collier, Z.A.; Heffner, K.; Linkov, I. Systems engineering framework for cyber physical security and resilience. Environ. Syst. Decis. 2015 , 35 , 291–300. [ Google Scholar ] [ CrossRef ]
- Lepri, B.; Oliver, N.; Letouzé, E.; Pentland, A.; Vinck, P. Fair, transparent, and accountable algorithmic decision-making processes. Philos. Technol. 2018 , 31 , 611–627. [ Google Scholar ] [ CrossRef ]
- Nwafor, E.; Campbell, A.; Hill, D.; Bloom, G. Towards a provenance collection framework for internet of things devices. In Proceedings of the 2017 IEEE SmartWorld, Ubiquitous Intelligence & Computing, Advanced & Trusted Computed, Scalable Computing & Communications, Cloud & Big Data Computing, Internet of People and Smart City Innovation (SmartWorld/SCALCOM/UIC/ATC/CBDCom/IOP/SCI), San Francisco, CA, USA, 4–8 August 2017; IEEE: New York, NY, USA, 2017; pp. 1–6. [ Google Scholar ]
- Reddy, E.; Cakici, B.; Ballestero, A. Beyond mystery: Putting algorithmic accountability in context. Big Data Soc. 2019 , 6 , 2053951719826856. [ Google Scholar ] [ CrossRef ]
- Banerjee, A. Blockchain Technology: Supply Chain Insights from ERP. In Advances in Computers ; Volume 111: Blockchain Technology: Platforms, Tools and Use Cases; Raj, P., Deka, G., Eds.; Elsevier: Amsterdam, The Netherlands, 2018. [ Google Scholar ]
- Terzi, S.; Nizamas, A.; Tzovaras, D.; Zacharaki, A.; Votis, K.; Stamelos, I.; Ioannidis, D. Transforming the Supply-chain management and Industry Logistics with Blockchain Smart Contracts. In Proceedings of the PCI ‘19, Nicosia, Cyprus, 28–30 November 2019; ACM: New York, NY, USA, 2019; pp. 1–6. [ Google Scholar ]
- Obaidat, M.A.; Obeidat, S.; Holst, J.; Al Hayajneh, A.; Brown, J. A Comprehensive and Systematic Survey on the Internet of Things: Security and Privacy Challenges, Security Frameworks, Enabling Technologies, Threats, Vulnerabilities and Countermeasures. Computers 2020 , 9 , 44. [ Google Scholar ] [ CrossRef ]
- Kaaniche, N.; Belguith, S.; Laurent, M.; Gehani, A.; Russello, G. Prov-Trust: Towards a trustworthy SGX-based data provenance system. In Proceedings of the 17th International Joint Conference on e-Business and Telecommunications—Volume 3: SECRYPT, Paris, France, 8–10 July 2020; ScitePress: Setúbal, Portugal, 2020; pp. 225–237. [ Google Scholar ]
- Obaidat, M.A.; Brown, J. Perspectives of Blockchain in Cybersecurity: Applications and Future Developments. In Industry Use Cases on Blockchain Technology Applications in IoT and the Financial Sector ; Mahmood, Z., Ed.; IGI Global: Hershey, PA, USA, 2021; pp. 109–131. [ Google Scholar ]
- Ramachandran, A.; Kantarcioglu, M. Smart provenance: A distributed, blockchain based data provenance system. In Proceedings of the Eighth ACM Conference on Data and Application Security and Privacy, Tempe, AZ, USA, 19–21 March 2018; pp. 35–42. [ Google Scholar ]
- Veisi, P. Visualizing Provenance in a Supply Chain Using Ethereum Blockchain. Master’s Thesis, Department of Computer Science, University of Saskatchewan, Saskatoon, SK, Canada, 2019; pp. 1–81. [ Google Scholar ]
- Zaman, S.; Alhazmi, K.; Aseeri, M.A.; Ahmed, M.R.; Khan, R.T.; Kaiser, M.S.; Mahmud, M. Security Threats and Artificial Intelligence Based Countermeasures for Internet of Things Networks: A Comprehensive Survey. IEEE Access 2021 , 9 , 94668–94690. [ Google Scholar ] [ CrossRef ]
- Alcaraz, C.; Lopez, J.; Zhou, J.; Roman, R. Secure SCADA Framework for the Protection of Energy Control Systems. Concurr. Comput. Pract. Exp. 2011 , 23 , 1414–1430. [ Google Scholar ] [ CrossRef ]
- Bailey, D.; Wright, E. Practical SCADA for Industry ; Elsevier: London, UK, 2003. [ Google Scholar ]
- Ipakchi, A.; Albuyeh, F. Grid of the Future. IEEE Power Energy Mag. 2009 , 7 , 52–62. [ Google Scholar ] [ CrossRef ]
- Karnouskos, S.; Colombo, A.W. Architecting the next generation of service-based SCADA/DCS system of systems. In Proceedings of the IECON 2011—37th Annual Conference of the IEEE Industrial Electronics Society, Melbourne, VIC, Australia, 7–10 November 2011; IEEE: New York, NY, USA, 2011; pp. 1–6. [ Google Scholar ]
- Carlsson, O. Engineering of IoT Automation Systems. Ph.D. Thesis, Lule’a University of Technology, Lule’a, Sweden, 2017. [ Google Scholar ]
- Ghomi, E.J.; Rahmani, A.M.; Qader, N.N. Cloud manufacturing: Challenges, recent advances, open research issues, and future trends. Int. J. Adv. Manuf. Technol. 2019 , 102 , 3613–3639. [ Google Scholar ] [ CrossRef ]
- Gupta, R.; Phanden, R.K.; Sharma, S.; Srivastava, P.; Chaturvedi, P. Security in manufacturing systems in the age of industry 4.0: Pitfalls and possibilities in Advances in Industrial and Production Engineering ; Lecture Notes in Mechanical Engineering; Phanden, R.K., Mathiyazhagan, K., Kumar, R., Paulo Davim, J., Eds.; Springer International Publishing: Singapore, 2021; pp. 105–113. [ Google Scholar ]
- Skulj, G.; Vrabic, R.; Butala, P.; Sluga, A. Decentralised network architecture for cloud manufacturing. Int. J. Comput. Integr. Manuf. 2017 , 30 , 395–408. [ Google Scholar ]
- Liu, C.; Su, Z.; Xu, Z.; Lu, Y. Service-oriented industrial internet of things gateway for cloud manufacturing. Robot. Comput. Integr. Manuf. 2022 , 73 , 102217. [ Google Scholar ] [ CrossRef ]
- Lim, M.; Xiong, W.; Wang, C. Cloud manufacturing architecture: A critical analysis of its development, characteristics and future agenda to support its adoption. Ind. Manag. Data Syst. 2021 , 121 , 2143–2180. [ Google Scholar ] [ CrossRef ]
- Liu, Y.; Wang, L.; Wang, X.V.; Xu, X.; Zhang, L. Scheduling in cloud manufacturing: State-of-the-art and research challenges. Int. J. Prod. Res. 2019 , 57 , 4854–4879. [ Google Scholar ] [ CrossRef ]
- Haghnegahdar, L.; Joshi, S.S.; Dahotre, N.B. From IoT-based cloud manufacturing approach to intelligent additive manufacturing: Industrial Internet of Things—An overview. Int. J. Adv. Manuf. Technol. 2022 , 119 , 1461–1478. [ Google Scholar ] [ CrossRef ]
- Obaidat, M.; Khodiaeva, M.; Obeidat, S.; Salane, D.; Holst, J. Security Architecture Framework for Internet of Things (IoT). In 2019 IEEE 10th Annual Ubiquitous Computing, Electronics & Mobile Communication Conference (UEMCON), New York, NY, USA, 10–12 October 2019 ; IEEE: New York, NY, USA, 2019. [ Google Scholar ]
- Sadeghi, A.; Wachsmann, C.; Waidner, M. Security and Privacy Challenges in Industrial Internet of Things. In Proceedings of the DAC ’15, San Francisco, CA, USA, 7–11 June 2015; ACM: New York, NY, USA, 2015; pp. 1–6. [ Google Scholar ]
- Urquhart, L.; McAuley, D. Avoiding the internet of insecure industrial things. Comput. Law Secur. Rev. 2018 , 34 , 450–466. [ Google Scholar ] [ CrossRef ]
- Khodjaeva, M.; Obaidat, M.; Salane, D. Mitigating Threats and Vulnerabilities of RFID in IoT Through Outsourcing Computations for Public Key Cryptography. In Security, Privacy and Trust in the IoT Environment ; Mahmood, Z., Ed.; Springer: Cham, Switzerland, 2019; pp. 39–60. [ Google Scholar ]
- Li, J.; Chen, X.; Huang, Q.; Wong, D.S. Digital provenance: Enabling secure data forensics in cloud computing. Future Gener. Comput. Syst. 2014 , 37 , 259–266. [ Google Scholar ] [ CrossRef ]
- Carata, L. Provenance-Based Computing. Ph.D. Thesis, University of Cambridge, Cambridge, UK, 2018; pp. 1–132. [ Google Scholar ]
- Ali, S.; Wang, G.; Bhuiyan, M.Z.A.; Jiang, H. Secure data provenance in cloud-centric internet of things via blockchain smart contracts. In Proceedings of the 2018 IEEE SmartWorld, Ubiquitous Intelligence & Computing, Advanced & Trusted Computing, Scalable Computing & Communications, Cloud & Big Data Computing, Internet of People and Smart City Innovation (SmartWorld/SCALCOM/UIC/ATC/CBDCom/IOP/SCI), Guangzhou, China, 8–12 October 2018; IEEE: New York, NY, USA, 2018; pp. 991–998. [ Google Scholar ]
- Walker-Roberts, S.; Hammoudeh, M.; Aldabbas, O.; Aydin, M.; Dehghantanha, A. Threats on the horizon: Understanding security threats in the era of cyber-physical systems. J. Supercomput. 2020 , 76 , 2643–2664. [ Google Scholar ] [ CrossRef ]
- Ali, M. Provenance-Based Data Traceability Model and Policy Enforcement Framework for Cloud Services. Ph.D. Thesis, University of Southampton, Southampton, UK, 2016; pp. 1–232. [ Google Scholar ]
- Liang, X.; Shetty, S.; Tosh, D.; Kamhoua, C.; Kwait, K.; Njilla, L. ProvChain: A Blockchain-based Data Provenance Architecture in Cloud Environment with Enhanced Privacy and Availability. In Proceedings of the 2017 17th IEEE/ACM International Symposium on Cluster, Cloud and Grid Computing, Madrid, Spain, 14–17 May 2017; IEEE: New York, NY, USA, 2017; pp. 468–477. [ Google Scholar ]
- Arumugam, S.S.; Umashankar, V.; Narendra, N.C.; Badrinath, R.; Mujumdar, A.P.; Holler, J.; Herranz, A.H. IoT Enabled Smart Logistics Using Smart Contracts. In Proceedings of the 2018 8th International Conference on Logistics, Informatics and Service Sciences (LISS), Toronto, ON, Canada, 3–6 August 2018; IEEE: New York, NY, USA, 2018; pp. 24–246. [ Google Scholar ]
- Suhail, S.; Hong, C.S.; Ahmad, Z.U.; Zafar, F.; Khan, A. Introducing Secure Provenance in IoT: Requirements and Challenges, In 2016 International Workshop on Secure Internet of Things, Heraklion, Greece, 26–30 September 2016 ; IEEE: New York, NY, USA, 2016; pp. 39–46. [ Google Scholar ]
- Sigwart, M.; Borkowski, M.; Peise, M.; Schulte, S.; Tai, S. A secure and extensible blockchain-based data provenance framework for the Internet of Things. Pers. Ubiquitous Comput. 2020 , 28 , 1–15. [ Google Scholar ] [ CrossRef ]
- Wu, H.; Han, H.; Wang, X.; Sun, S. Research on Artificial Intelligence Enhancing Internet of Things Security: A Survey. IEEE Access 2020 , 8 , 153826–153848. [ Google Scholar ] [ CrossRef ]
- Xu, Z.; Liu, W.; Huang, J.; Yang, C.; Lu, J.; Tan, H. Artificial Intelligence for Securing IoT Services in Edge Computing: A Survey. Secur. Commun. Netw. 2020 , 2020 , 8872586. [ Google Scholar ] [ CrossRef ]
- Bagaa, M.; Taleb, T.; Bernabe, J.B.; Skarmeta, A. A Machine Learning Security Framework for Iot Systems. IEEE Access 2020 , 8 , 114066–114077. [ Google Scholar ] [ CrossRef ]
- Khilar, R.; Mariyappan, K.; Christo, M.S.; Amutharaj, J.; Anitha, T.; Rajendran, T.; Batu, A. Artificial Intelligence-Based Security Protocols to Resist Attacks in Internet of Things. Wirel. Commun. Mob. Comput. 2022 , 2022 , 1440538. [ Google Scholar ] [ CrossRef ]
- Kuzlu, M.; Fair, C.; Guler, O. Role of Artificial Intelligence in the Internet of Things (IoT) cybersecurity. Discov. Internet Things 2021 , 1 , 7. [ Google Scholar ] [ CrossRef ]
- Iwendi, C.; Rehman, S.U.; Javed, A.R.; Khan, S.; Srivastava, G. Sustainable Security for the Internet of Things Using Artificial Intelligence Architectures. ACM Trans. Internet Technol. 2021 , 21 , 73.1–73.22. [ Google Scholar ] [ CrossRef ]
- Abdullah, M.; Baashar, Y.; Alhussain, H.; Alwadain, A.; Aziz, N.; Capretz, L.F.; Abdulkadir, S.J. Detecting Cybersecurity attacks in Internet of Things Using Artificial Intelligence Methods: A Systematic Literature Review. Electronics 2022 , 11 , 198. [ Google Scholar ] [ CrossRef ]
- Wasson, C.S. System Analysis, Design, and Development Concepts, Principles, and Practices ; Wiley: Hoboken, NJ, USA, 2006. [ Google Scholar ]
- Ethereum. Intro to Ethereum. Ethereum.Org. 2023. Available online: https://ethereum.org/en/developers/docs/intro-to-ethereum/ (accessed on 2 May 2023).
- Ethereum. Introduction to Smart Contracts. Ethereum.Org. 2023. Available online: https://ethereum.org/en/developers/docs/smart-contracts/ (accessed on 2 May 2023).
- Ethereum. Intro to DApps. Ethereum.Org. 2023. Available online: https://ethereum.org/en/developers/docs/dapps/ (accessed on 2 May 2023).
- Hyperledger. Fabric Docs—Introduction. Hyperledger Fabric. 2023. Available online: https://hyperledger-fabric.readthedocs.io/en/release-2.5/blockchain.html (accessed on 2 May 2023).
- Hyperledger. Hyperledger Fabric Model. Hyperledger Fabric Docs, 2023d. Available online: https://hyperledger-fabric.readthedocs.io/en/release-2.5/fabric_model.html (accessed on 4 May 2023).
- Anees, T.; Habib, Q.; Al-Shamayleh, A.S.; Khalil, W.; Obaidat, M.A.; Akhunzada, A. The Integration of WoT and Edge Computing: Issues and Challenges. Sustainability 2023 , 15 , 5983. [ Google Scholar ] [ CrossRef ]
- Barclay, I.; Preece, A.; Taylor, I. Defining the Collective Intelligence Supply Chain. In Proceedings of the AAAI FSS-18: Artificial Intelligence in Government and Public Sector, Arlington, WA, USA, 18–21 October 2018; Online Research at Cardiff University: Cardiff, UK, 2018; pp. 1–6. [ Google Scholar ]
Click here to enlarge figure
The statements, opinions and data contained in all publications are solely those of the individual author(s) and contributor(s) and not of MDPI and/or the editor(s). MDPI and/or the editor(s) disclaim responsibility for any injury to people or property resulting from any ideas, methods, instructions or products referred to in the content. |
Share and Cite
Umer, M.A.; Belay, E.G.; Gouveia, L.B. Fortifying Industry 4.0: Internet of Things Security in Cloud Manufacturing through Artificial Intelligence and Provenance Blockchain—A Thematic Literature Review. Sci 2024 , 6 , 51. https://doi.org/10.3390/sci6030051
Umer MA, Belay EG, Gouveia LB. Fortifying Industry 4.0: Internet of Things Security in Cloud Manufacturing through Artificial Intelligence and Provenance Blockchain—A Thematic Literature Review. Sci . 2024; 6(3):51. https://doi.org/10.3390/sci6030051
Umer, Mifta Ahmed, Elefelious Getachew Belay, and Luis Borges Gouveia. 2024. "Fortifying Industry 4.0: Internet of Things Security in Cloud Manufacturing through Artificial Intelligence and Provenance Blockchain—A Thematic Literature Review" Sci 6, no. 3: 51. https://doi.org/10.3390/sci6030051
Article Metrics
Further information, mdpi initiatives, follow mdpi.
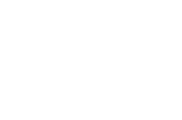
Subscribe to receive issue release notifications and newsletters from MDPI journals

An official website of the United States government
The .gov means it’s official. Federal government websites often end in .gov or .mil. Before sharing sensitive information, make sure you’re on a federal government site.
The site is secure. The https:// ensures that you are connecting to the official website and that any information you provide is encrypted and transmitted securely.
- Publications
- Account settings
Preview improvements coming to the PMC website in October 2024. Learn More or Try it out now .
- Advanced Search
- Journal List
- Springer Nature - PMC COVID-19 Collection

Physical exercise as a tool to help the immune system against COVID-19: an integrative review of the current literature
Matheus pelinski da silveira.
1 Federal University of Fronteira Sul, Campus Chapecó, SC 484 - KM 02, 89802-000 Chapecó, SC Brazil
Kimberly Kamila da Silva Fagundes
Matheus ribeiro bizuti, Édina starck, renata calciolari rossi.
2 University of Oeste Paulista (UNOESTE), Presidente Prudente, Brazil
Débora Tavares de Resende e Silva
Acute viral respiratory infections are the main infectious disease in the world. In 2020, a new disease caused by severe acute respiratory syndrome coronavirus 2 (SARS-CoV-2), coronavirus disease 2019 (COVID-19), became a global pandemic. The immune response to the virus depends on factors such as genetics, age and physical state, and its main input receptor is the angiotensin-converting enzyme 2. The practice of physical exercises acts as a modulator of the immune system. During and after physical exercise, pro- and anti-inflammatory cytokines are released, lymphocyte circulation increases, as well as cell recruitment. Such practice has an effect on the lower incidence, intensity of symptoms and mortality in viral infections observed in people who practice physical activity regularly, and its correct execution must be considered to avoid damage. The initial response is given mainly by type I interferons (IFN-I), which drive the action macrophages and lymphocytes, followed by lymphocyte action. A suppression of the IFN-I response has been noted in COVID-19. Severe conditions have been associated with storms of pro-inflammatory cytokines and lymphopenia, as well as circulatory changes and virus dispersion to other organs. The practice of physical activities strengthens the immune system, suggesting a benefit in the response to viral communicable diseases. Thus, regular practice of adequate intensity is suggested as an auxiliary tool in strengthening and preparing the immune system for COVID-19. Further studies are needed to associate physical exercise with SARS-CoV-2 infection.
Introduction
Acute respiratory infections (ARIs) are caused by respiratory viruses and bacteria, being the most infectious disease in humans [ 1 , 2 ]. These can be caused by more than 200 different viruses, with rhinovirus being the most common etiological agent [ 3 – 5 ]. In December 2019, a new coronavirus outbreak was reported in China, being called the Severe Acute Respiratory Syndrome Coronavirus 2 (SARS-CoV-2), spreading rapidly and infecting more than 14 million people, being declared a Health Emergency International Public Service on January 30, 2020 [ 6 , 7 ].
The main mode of transmission is contact with droplets containing viral particles eliminated through the cough or sneeze of an infected person, and the incubation period usually varies from 2 to 14 days. Approximately 80% of the cases are asymptomatic or with mild symptoms, and the others can be severe or critical and can lead to death [ 8 ]. The development of Coronavirus Disease 2019 (COVID-19) is dependent on the interaction between SARS-CoV-2 and the host’s immune system, the immune response being influenced by genetics (HLA genes), age, sex, nutritional status and status physical [ 9 ].
The immune response includes two stages, innate immunity and adaptive immunity. The first one comprises physical and chemical barriers and the action of cells such as macrophages, dendritic cells (DCs), natural killer cells (NKs), neutrophils and molecules such as cytokines, interleukins (ILs), nitric oxide (NO) and superoxide anion (O2–). The second one has as mechanism of action the T lymphocytes (TCD4 + and TCD8 +) and B lymphocytes and their products, such as antibodies and cytokines. Furthermore, the adaptive immune response can be subdivided into cellular immunity (mediate by cells as macrophages and lymphocytes) and humoral immunity (mediates by cells as macrophages and lymphocytes) and humoral immunity (mediated by antibodies) [ 10 , 11 ]. The regular practice of physical exercises promotes improvements in quality of life and can act in the immune response, reducing the risk of developing systemic inflammatory processes and stimulating cellular immunity [ 12 ].
Therefore, the present article aims to perform an integrative review of the literature relating the role of physical exercise on the immune system in the fight against COVID-19. For this purpose, the bibliographic study included knowledge about respiratory infections, influences of physical exercise on the immune system and proposed the comprehension of the most recent information about the immunopathogenesis of SARS-CoV-2 infection, also comprising its relationship with the host’s physical and health conditions.
Influence of physical exercise on the immunological response
Physical activity is considered one of the main components of healthy living. In addition to the functions related to the prevention of excess body weight, systemic inflammation and chronic non-communicable diseases, a potential benefit of physical exercise in reducing communicable diseases, including viral pathologies, is suggested [ 13 ].
The practice of physical exercise, both in its acute form and in its chronic form, significantly alters the immune system [ 14 , 15 ]. Studies indicate that the modulation of the immune response related to exercise depends on factors such as regularity, intensity, duration and type of effort applied [ 13 , 16 ].
Moderate-intensity physical exercises stimulate cellular immunity, while prolonged or high-intensity practices without appropriate rest can trigger decreased cellular immunity, increasing the propensity for infectious diseases [ 14 , 15 ]. According to the International Society for Exercise and Immunology (ISEI), the immunological decrease occurs after the practice of prolonged physical exercise, that is, after 90 min of moderate- to high-intensity physical activity [ 17 ]. Cellular changes due to physical activity are illustrated in Fig. 1 .

Effect of physical activity on the immune system.
Source : The Authors (2020)
Effects of physical exercise on immune system components
Cytokines are classified as anti-inflammatory and pro-inflammatory according to their functions. Among the anti-inflammatory cytokines, we highlight IL-10 and transforming growth factor-beta (TGF-β), responsible for inhibiting the production of pro-inflammatory cytokines [ 18 ]. Among the pro-inflammatory cytokines, we highlight IL-1, IL-2, IL-12, IL-18, interferon-gamma (IFN-γ) and tumor necrosis factor-alpha (TNF-α) [ 19 ]. Cytokine production can be modified due to hormonal or oxidative stress and physical exercise. The muscle contraction has the effect of increasing the release of antinflammatory and pro-inflammatory cytokines at levels that vary according to the volume of contractile mass involved, duration and intensity of exercise [ 20 ].
Neutrophils
During the practice of physical exercise, the activation of the muscle fiber is responsible for increasing the release of calcium (Ca2 +) and, therefore, promoting the synthesis of pro-inflammatory cytokines, namely TNF-α and IL-1β, which act in the regulation of selectins, which, in turn, attract neutrophils to the site [ 21 ]. The neutrophilia induced by physical activity is due to the release of neutrophils from the bone marrow due to the influence of cortisol [ 22 ].
After aerobic physical exercise (approximately 24 h), there is a significant reduction in neutrophil chemotaxis, however, without compromising bactericidal activity. The reduction in neutrophil chemotaxis is reversed within 48 h after physical activity, during which the opportunistic activity of infectious microorganisms can occur [ 23 ].
Physical activity is also responsible for increasing the concentration of circulating leukocytes [ 24 ]. This is due to shearing of immune cells in blood vessels, especially secondary lymphoid tissues such as liver, spleen and lung [ 25 ]. The leukocyte concentration remains high with a peak of 30–120 min after constant physical activity, which may persist for up to 24 h after [ 24 ].
Antigen-presenting cells (APCs)
The practice of aerobic physical exercise in an exacerbated manner is responsible for decreasing the expression of Toll-like receptors (TLRs) in macrophages, considerably reducing the presentation of antigens to T lymphocytes, thus causing the suppression of the inflammatory T helper type 1 (Th1) response. Thus, the failure to develop an inflammatory activity precludes possible tissue damage resulting from inflammatory mediators and, consequently, the risk of chronic inflammatory processes. However, the susceptibility to infections due to intracellular microorganisms increases [ 26 ].
Natural killer cells (NKs)
During physical activity, blood flow increases in order to supply the metabolic demands of the human body. The recruitment of NK cells occurs through cellular stress promoted by exercise and a consequent decrease in adhesion molecules induced by catecholamines [ 27 ]. However, physical activity lasting more than three hours causes the concentration of NK cells to return to the pre-exercise state or even lower than this. This is because the NK cells migrate to the muscle injury site [ 28 ].
Lymphocytes
During moderate physical exercise, the concentration of lymphocytes increases in the vascular bed and, after strenuous exercise, decreases to levels below the pre-exercise period [ 29 , 30 ]. The CD4 +:CD8 + ratio decreases as TCD8 + cells increase [ 14 ].
TCD4 + cells decrease due to the increase in NK cells [ 14 , 31 ]. After physical activity, the lymphocyte concentration decreases due to the apoptosis mechanism [ 32 ]. Thus, the increase in lymphocyte concentrations favors the Th1-mediated immune response, preventing infections by intracellular microorganisms [ 24 ] (Fig. 2 ).

Innate immune response to the presence of viruses.
SARS-COV-2 virology and the role of the immune system in COVID-19 infection
SARS-CoV-2 is constituted by single-strand positive-sense RNA and belongs to the genus Betacoronavirus, lineage B and subgenus Sarbecovirus. Viral genome studies have identified similarity of SARS-CoV-2 with bat coronaviruses, as well as coronaviruses responsible for two previous pandemics: the Severe Acute Respiratory Syndrome-Related Coronavirus (SARS-CoV) and the Middle Eastern Respiratory Syndrome Coronavirus (MERS-CoV) [ 33 , 34 ].
Similar to SARS-CoV, the novel coronavirus uses a structural glycoprotein to infect cells: the envelope Spike protein (S), using the Angiotensin-Converting Enzyme-2 (ACE2) as entry receptor [ 33 , 34 ]. ACE2 consists of a cell membrane protein abundantly expressed in the organism, which is present in cardiac, pulmonary, renal, intestinal and vascular cells, and the binding of SARS-CoV-2 with this enzyme has a strong affinity, which may explain the high transmissibility of the virus [ 8 , 9 , 34 ].
Due to mainly respiratory symptoms, it is believed that the target cells of SARS-CoV-2 are in lower airways [ 35 ], as is the case of type 2 pneumocytes or alveolar cells, the main site of expression of ACE2 receptors [ 8 ]. The result of the interaction between the S receptor binding domain (RBD) and ACE2 is the fusion of the viral and host membranes, which proceeds for viral replication and dissemination and may reach the other cells with ACE2 expression in the organism [ 8 ].
With the purpose of containing the infection, the innate and adaptive immune system is activated by mechanisms that are not yet completely elucidated. In spite of this, it is known that effective immunological actions are essential to control viral replication and dissemination, cellular inflammation and tissue injury, and many studies have reported that the immune response of the host influences the severity of COVID-19 [ 9 , 36 – 38 ].
Immune response to coronavirus infections
To initiate the antiviral response, cells of the innate immune system need to recognize the infection, a process performed through pattern-recognition receptors (PRRs) such as TLR, NOD-like receptor (NLR), C-type lectin-like receptor (CLR), RIG-I-like receptor (RLR) and free-molecule receptors in the cytoplasm, which detect pathogen-associated molecular patterns (PAMPs). Once viral nucleic acids are recognized as PAMPs, PRRs activate molecular pathways of inflammatory response, stimulating chemotaxis, maturation of immune cells, phagocytosis and expression of inflammatory factors [ 39 ].
Viral recognition by TLR3, TLR7 and RIG-I receptors leads to activation of the nuclear factor-κB (NF-κB) and IRF3 signaling cascade, with nuclear transcription and expression of type I interferons (IFNs-I) and pro-inflammatory cytokines, creating the first line of defense against viral infections. The IFNs-I (IFN-α and IFN-β) are the most important antiviral cytokines, and they act as immunomodulators influencing the activities of macrophages and lymphocytes, performing actions such as protection of non-infected cells, containment of viral replication and effective activation of the adaptive immune system [ 8 , 9 , 39 ].
A suppression or delay of IFNs-I response—due to viral evasion mechanisms—results in impairment of early infection control, hyperinflammatory infiltrate of neutrophils, macrophages and monocytes into the lungs, production of cytokines by these cells and lung tissue damage. This process, described in SARS-CoV and MERS-CoV, has been suggested as a possible strategy to trigger or collaborate to the pathology of COVID-19 [ 8 , 9 ].
Macrophages and DCs act as APCs for lymphocytes via MHC and produce a microenvironment of signaling cytokines, activating the adaptive immune system. T lymphocytes perform important functions against viral microorganisms, since TCD8 + can cause direct cytotoxicity against infected cells and TCD4 + stimulate B lymphocytes to produce neutralizing antibodies. In turn, T helper lymphocytes (Th)—predominantly Th1—contribute to the organization of the adaptive response and release cytokines that are able to recruit monocytes and neutrophils and to promote other cascades of pro-inflammatory molecules, amplifying the immune response [ 8 , 39 ] (Fig. 3 ).

Adaptive immune response to the presence of viruses.
The complement system can also be activated and has an important role in coronavirus infections, because it helps the innate immune system to identify antigens. However, its activation can contribute to the disease due to its potent capacity to stimulate neutrophils and to recruit inflammatory cells, which can trigger tissue damage [ 39 ].
Unbalanced immunological responses at COVID-19
Studies suggest that in mild cases, pulmonary tissue macrophages are capable of containing SARS-CoV-2 and innate and adaptive immune responses are efficiently activated against viral replication. However, severe cases of COVID-19 are associated with an imbalance in antiviral immunity, characterized by two main situations: a pro-inflammatory cytokine storm and a lymphopenia state [ 37 , 38 ].
The degree of lymphopenia and cytokine storm was related to the severity of COVID-19 [ 7 ], and similar situations had already been reported in other respiratory viral infections, including influenza, SARS-CoV and MERS-CoV. Activation of the complement system and abnormalities in coagulation were also observed in severe patients with markers such as C-reactive protein (CRP), dimero-D and fibrin degradation products, which are usually elevated in advanced stages of the disease [ 37 , 38 ].
The mechanism of cytokine storm and lymphopenia associated with circulatory alterations and viral dissemination to several organs was proposed as being responsible for viral sepsis. Among the complications of this condition are acute respiratory distress syndrome (ARDS), septic shock, multiple organ failure (MOF) and death [ 8 , 38 ].
Cytokine storm
In patients with severe COVID-19, increased levels of cytokines were observed, including IL-1β, IL-2, IL-6, IL-8, IL-10, IL-17, interferon-gamma (IFN-γ), tumor necrosis factor alpha (TNF-α), granulocyte-colony stimulating factor (G-CSF), gamma-induced protein 10 (IP10), monocyte chemoattractant protein 1 (MCP1), macrophage inflammatory protein 1 alpha (MIP1-α) and other molecules, characterizing the cytokine storm [ 37 , 38 ]. Additionally, elevations of IL-1β, IFN-γ, IP10 and MCP1 in infections by the novel coronavirus were associated with the Th1 response; however, an increase in interleukins of the T helper type 2 (Th2) profile, such as IL-4, IL-5, IL10, which suppress the inflammation, was also associated with a greater severity of COVID-19, which may demonstrate an imbalance in immune regulation and an attempt to minimize tissue inflammatory damage [ 35 , 40 ].
The cytokine storm generates an immunological system attack against the organism, which can cause substantial lesions in organs such as the lung, heart, brain, kidneys, spleen, liver and lymph nodes. The increase of neutrophils, macrophages and monocytes in association with a dysfunction of the IFN-I response has been reported as the main cause of lethality in SARS-CoV and MERS-CoV pneumonia, and a similar conclusion has been suggested for SARS-CoV-2 [ 9 , 37 , 38 ].
In the lungs, alveolar macrophages and epithelial cells are the most responsible for the production of cytokines and chemokines. During infection by the novel coronavirus, excessive secretion of these molecules by the immune cells mediates a massive pulmonary infiltrate of neutrophils, monocytes and macrophages, which results in alveolar damage due to wall thickening and formation of hyaline membranes. In addition, the accumulation of neutrophils in the lungs increases the production of reactive oxygen species (ROS) and pro-inflammatory molecules, predisposing to injury [ 41 ]. Therefore, high levels of pro-inflammatory cytokines are associated with respiratory insufficiency, ARDS and may lead to shock, MOF and death in the COVID-19 [ 37 , 38 ].
In comparison, pulmonary disease caused by SARS-CoV also presents with formation of hyaline membranes, desquamation of alveolar space pneumocytes and interstitial infiltration with lymphocytes and mononuclear cells. In the serum of patients who develop SARS, there are high levels of cytokines and pro-inflammatory chemokines [ 42 ].
Additionally, it has been observed that during viral infections, pro-inflammatory cytokines can stimulate an increase in the levels of ACE2 protein, the receptor for SARS-CoV-2. A larger quantity of this protein may accelerate the entry of the novel coronavirus into the host cells and contribute to its dissemination in the organism, negatively influencing the antiviral response [ 34 ].
Changes in cellular counts
In critically ill patients, a state of immune suppression is also described, with a significant and sustained decrease in the absolute number of TCD4 + and TCD8 + lymphocytes, possible reduction of B lymphocytes, NK cells, monocytes, eosinophils and basophils [ 37 , 38 ]. The study conducted by Liu et al. [ 7 ] did not observe significant changes in the total counts of B lymphocytes, NK cells and monocytes; however, there was a considerable reduction in the lymphocyte count in peripheral blood of patients with severe COVID-19 at the beginning of the disease, especially cytotoxic TCD8 + cells [ 7 ].
Activation markers for TCD4 + and TCD8 + lymphocytes showed excessive stimulation and exhaustion markers for TCD8 + lymphocytes were elevated in the disease, suggesting lymphocyte dysfunction [ 9 , 37 ]. Additionally, an increase in the number of neutrophils and a greater neutrophil-to-CD8 + T cell ratio (N8R) was associated with severe COVID-19 and proposed as the most significant predictor of poor prognosis [ 7 , 37 ].
Cell death induced by interaction between Fas and Fas ligand by activation of the TNF-related apoptosis inducing ligand axis and by direct infection of T lymphocytes by SARS-CoV-2 may be responsible for the origin of lymphopenia in COVID-19 [ 38 ]. The cytokine storm can also influence the lymphopenia, since in the study of Liu et al. [ 7 ], the peaks in cytokine levels IL-2, IL-4, IL-10, TNF-α and IFN-γ coincided with the lowest T lymphocyte counts, about 4–6 days after the onset of severe COVID-19; therefore, the restoration of T cell numbers was associated with reductions in circulating cytokines [ 7 ].
The fact that T cells are important regulators of the activation of the immune system during a viral infection may explain how lymphopenia is related to the worsening of inflammatory responses [ 7 ]. In consequence of lymphopenia and lymphocyte dysfunction, the adaptive immune response is ineffective and the infection is not adequately controlled, further increasing the stimulation of cytokines and cellular infiltrations [ 38 ].
Coagulation disorders
It was observed that patients with SARS-CoV-2 infection present increased risk of venous thromboembolism (VTE) and disseminated intravascular coagulation (DIC). Coagulopathy associated with COVID-19, as it may be named, is characterized by hypercoagulability and thrombosis and is associated with worse prognosis in infection. Among the altered coagulation parameters in patients with severe COVID-19 are exacerbated coagulation activation, coagulation factor consumption, prolongation of prothrombin time (PT) and activated partial thromboplastin time (aPPT), moderate to severe thrombocytopenia, increased D-dimer and reduction of fibrinogen [ 43 ].
Through a retrospective analysis of 183 patients with coronavirus pneumonia, high levels of the D-dimer and fibrin degradation product have been identified, in addition to prolonged PT, as well as aPPT in patients who had deceased. Tang et al. [ 44 ] considered the D-dimer as an important coagulopathy marker in cases of SARS-COV-2 infection. The same findings were found in the studies by Han et al. [ 45 ] who see the use of hemostasis tests as tools to be used in early diagnosis and in monitoring disease progression [ 46 ].
Blood coagulation is the fastest mechanism in the confinement and inactivation of infections, being the first and the last defense line of the innate immune system to take place in tissues and blood circulation. The sore promotes the activation of the endothelial cells and the dysfunction of the endothelium, thus generating a pro-thrombotic state [ 47 ]. In cases hyperactivation of the immune system, the coagulation may become intravascular and disseminated, therefore causing multiple organ failure. After all, the amount of vascular endothelial lesions of organs and tissues is, due to their activation intent, inversely proportional to the amount of existing coagulation factors [ 48 ].
Thereupon, the first clinical studies carried out in patients with pneumonia caused by the Coronavirus confirmed the occurrence of organ dysfunction and coagulopathy as possible causes of the negative outcomes of the disease [ 44 ]. The activation of the immune system, in response to the infection, leads the production of cytokines and tissue factor expression. The cytokines, in large amounts, harm the gas exchange and lead not only to inflammation but fibrinolysis, thus increasing D-dimer concentration [ 49 ].
The tissue factor is related to an increase in thrombin generation and fibrin deposition, leading to hypercoagulability and CIVD and, thus, a worse prognosis [ 46 , 49 ]. As a contributor to the coagulation process, there is the presence of polymorphonuclear leukocytes (PMN) that are activated during the inflammatory process, releasing extracellular neutrophil traps (NETs) which contain proteases that generate the inactivation of endogenous anticoagulants and the propagation of a procoagulant state. The interaction of activated platelets with PMN can form vaso-occlusive thrombotic complexes [ 47 ].
Some authors also elucidate the virus’s relationship with the ECA2 functional receptor, present in the arterial and venous endothelial cells of most human organs and part of both axes of the renin–angiotensin system, the vasoconstrictor ECA/Ang/AT1R and the vasodilator ECA2/Ang-(1-7)/MAS [ 50 , 51 ]. Dalan et al. [ 52 ] cite that both aging and metabolic disorders positively regulate the ECA/Ang/AT1R axis, leading to inflammatory, oxidative, vasoconstrictor and fibrotic effects. Therefore, the ECA2/Ang-(1-7)/MAS axis is negatively regulated, resulting in a decrease in the anti-inflammatory and anti-fibrotic effects [ 52 ]. The connection of SARS-COV-2 to the ECA2 receiver affects the balance between ECA/Ang/AT1R and ECA2/Ang-(1-7)/MAS, making the effects of the ECA/Ang/AT1R axis even more prominent [ 53 ].
Host condition and COVID-19
The different immune responses of the host to the SARS-CoV-2 infection may explain the reason why men and women, young and old, infected by the virus can suffer a different severity of the disease [ 54 ]. Therefore, a considerably higher mortality rate was observed in patients with advanced chronological age [ 55 ].
Immune aging is related to an increase in individuals’ susceptibility to infections, due to the decline in immune function, which can occur at any stage of the immune response. Such changes can be seen, especially when associated with emotional stress [ 56 ]. Immune senescence is associated with the suppression of the activation and presentation of antigens by macrophages, which consequently prevent the migration of dendritic cells and the activation made by Toll receptors with less effect [ 56 , 57 ]. Ewers et al. [ 58 ] mention the decline and proliferation of T cells, in addition to the increased production of pro-inflammatory cytokines IL-1, IL-6 and TNF-α in the elderly.
Another point is the imbalance between Th1 and Th2 cytokines, generating an increase in the susceptibility of these individuals as infections by viruses and extracellular bacteria [ 58 ]. In view of this, aging is associated with a constitutive pro-inflammatory environment due to persistent and low-grade immune activation, which can lead to increased tissue damage caused by infections [ 57 ].
Also, there should be taken into account the positive regulation of the ACE-Ang-II-AT axis that leads to pro-inflammatory and pro-fibrotic effects. Although not very detailed, it is still suggested the occurrence of a greater number of ECA2 receptors associated with aging, which would increase this imbalance. After all, it is through this receptor that SARS-COV-2 infects humans and thus contributes to the development of COVID-19 in older people [ 52 ].
Obesity, type 2 diabetes mellitus (T2DM) and metabolic syndrome (MS)
The precarious metabolic health is considered the main risk factor for the development of severe forms of COVID-19. This may occur in T2DM, obesity and MS, possibly due to immune dysfunction in synergism with pathophysiological complications of these comorbidities [ 59 ].
Increased ACE2 expression is a protective adaptive mechanism in T2DM; however, it may facilitate the viral entry and spread of SARS-CoV-2 in the body [ 59 ]. Adipose tissue also exhibits high expression of ACE2, so the population with obesity may present greater vulnerability to COVID-19 [ 43 ].
It was observed that the expression of ACE2 in the adipose tissue of obese patients allows viral entry in adipocytes and makes this tissue a reservoir for the viral dissemination of SARS-CoV-2, since it is viscerally distributed [ 43 , 51 ]. In addition, obesity is an important factor for the development of T2DM—especially when associated with low levels of physical activity and poor physical conditioning—and as mentioned, both diseases are related to higher expression of ACE2, increasing the risk of advanced infection by SARS-CoV-2 [ 43 ].
Metabolic disorders lead to immune activation of tissues such as the adipose, increasing the concentration of low-grade chronic inflammation plasma markers, called metabolic inflammation or meta-inflammation [ 61 ]. In this sense, the release of pro-inflammatory adipokines such as leptin, TNF-α, IL-6 and IL-1b is observed, with a reduction in anti-inflammatory action through the suppression of adiponectins [ 62 , 63 ]. The presented relationship is directly proportional to the presence of adipose tissue, the same that can be regulated through the practice of physical activity [ 64 ].
The pro-inflammatory state found in metabolic syndrome and T2DM may increase the probability of an unbalanced inflammatory response in COVID-19, like the cytokine storm described in patients with severe disease [ 59 ]. Similarly, as obesity is a state of low-grade chronic inflammation, it shows a potential for immune amplification of pathogens, as the regulatory elements of the immune response are absent or dysfunctional and this may contribute to the cytokine storm, which is already in greater concentration in obese individuals and which sustains and activates multiple cytokine pathways for a long time after the viral insult [ 65 , 66 ].
Damage to blood vessels caused by chronic diseases, such as T2DM, associated with hypercoagulability present in COVID-19 may intensify the risk of infection complications [ 59 ]. Obesity can also aggravate endothelial dysfunction present in COVID-19 due to inflammation triggered by perivascular and vascular adipose tissue, combined with changes in the synthesis of endogenous vasoactive agents, leading to platelet hyperactivation, leukocyte adhesion and other modifications related to endothelial inflammation, prothrombosis and proatherogenesis [ 43 ].
Hypercoagulability is directly proportional to the severity of overweight in obese patients. Among the pathophysiological mechanisms are the action of adipocytokinins, with leptin and adiponectin, overactivity of coagulation factors, reduction of fibrinolytic function and, once again, increased inflammation (TNF and IL-6). Other contributors include elevated oxidative stress, lipid and glucose tolerance disorders, MS and venous stasis. Thus, it is considered a synergic effect of obesity and MS on the state of hypercoagulability in COVID-19, aggravating the risk of VTE and DIC even more [ 43 ].
There is also a greater amount of macrophages in the adipose tissue of obese individuals due to areas of microhypoxia, which lead to the nuclear factor-κB (NF-κB) pathway activation, thus increasing the expression of genes involved in inflammation [ 61 ]. This condition is the result of the attraction of monocytes in the circulation made by chemokines; when they infiltrate the adipose tissue, they transform into macrophages, which, in turn, release TNF-α and IL-6 which induce the tissue’s resistance to insulin [ 63 ]. Insulin resistance is also related to the host’s immune response, as it can inhibit the resolution of T cell-mediated inflammation [ 61 ].
The adipose tissue is not the only one affected by the deposition of fats, because of that, bone marrow and thymus also present significant changes to the immune system of obese individuals and those with MS [ 67 ]. Thus, there is a marked deregulation of immune responses, which leads to a lower presence of circulating T cells, reducing the response to pathogens [ 60 , 61 , 65 ].
Physical exercise and COVID-19
Despite the lack of accurate data on how physical activity improves the immune response against the new coronavirus, there is evidence of lower rates of ARI incidence, duration and intensity of symptoms and risk of mortality from infectious respiratory diseases in individuals who exercise at high levels appropriate. Furthermore, different studies suggest that regular physical exercise is directly related to decreased mortality from pneumonia and influenza, improvements in cardiorespiratory function, vaccine response, metabolism of glucose, lipids and insulin [ 13 , 16 ].
Increased immune surveillance against infections has been proposed as a mechanism responsible for improving the immune response related to physical exercise. Moderate-intensity physical activity is responsible for providing an increase in the anti-pathogenic activity of macrophages, at the same time as elevations in the circulation of immune cells, immunoglobulins and anti-inflammatory cytokines occur, thereby reducing the burden of pathogen on organs such as the lung and the risk of lung damage due to the influx of inflammatory cells [ 12 ].
During regular physical exercise practices, inflammatory responses and stress hormones are decreased; in contrast, lymphocytes, NK cells, immature B cells and monocytes are at high levels. Thus, there is an improvement in immunovigilance, as well as a reduction in the systemic inflammatory process, factors that corroborate that regular physical activity helps to improve the immune system, while helping to prevent respiratory diseases and thus protect against infections such as COVID-19 [ 68 ].
Dynamic exercises that generate cardiorespiratory overload promote the mobilization and redistribution of effector lymphocytes, mediated by catecholamines. This action primarily stimulates subtypes of lymphocytes capable of migrating from reservoirs—such as blood vessels, spleen and bone marrow—to lymphoid tissues and organs—such as the upper respiratory tract, lungs and intestines, aiming at recognizing and fighting pathogens and, thus, increasing immune surveillance and improving the antiviral response [ 16 ].
Similarly, regular exercise practices at moderate levels favor the function of the human body’s immune surveillance against pathogens, as they stimulate an exchange of white blood cells between the circulatory system and tissues, a fact that reduces morbidity and mortality from acute respiratory disease and infections viral. They are also capable of promoting protection against infections triggered by intracellular microorganisms, as viral agents, given that the predominant immune response is mediated by Th1 cells [ 68 ].
Regular exercise of moderate intensity has already been associated with a reduction in respiratory infections compared to sedentariness. However, exhaustive physical practices before or during an infectious condition, such as influenza or COVID-19, can trigger severe illness due to changes in the immune system [ 40 , 68 ]. This occurs due to the production of Th2 anti-inflammatory cytokines in order to reduce muscle tissue damage, but in strenuous activities this effect can reach immunosuppression levels, thus providing the opportunity for infections [ 12 , 40 ]. Therefore, attention should be paid to the importance of developing physical training at appropriate levels of execution.
To the detriment of the world demographic change and the habits arising from the technological revolution, the population is aging more, becoming more obese and, consequently, less active when it comes to physical exercise. In this way, the immune system undergoes negative changes; that is, there is a functional impairment of innate immunity and adaptive immunity called immunosenescence, which results in greater susceptibility to infectious diseases and systemic inflammatory processes, decreased response to antibodies and, therefore, compromised immunological surveillance [ 68 ].
Therefore, for the elderly population, physical activity is even more essential, as these individuals generally have greater comorbidities and, in relation to the new coronavirus, are more vulnerable to contracting the disease [ 69 ]. Damiot et al. [ 70 ] suggested that individuals who have remained active throughout their lives have less pronounced immunosenescence characteristics, which may be a possible protective factor against the development of complications caused by COVID-19.
In this sense, beneficial effects of regular physical exercise have been reported in the elderly population, including reduction in oxidative stress, improvement in immune competence and reduction in cellular changes related to immunosenescence [ 13 , 16 ]. Elderly individuals who maintain continued physical activity have levels of TCD4 + and TCD8 + lymphocytes similar to younger individuals, in addition to not having harmful defects in the recruitment of lymphocytes during the infectious process [ 58 ].
According to the study by Ferrer et al. [ 71 ] with 116 elderly volunteers, through physical activity there is a decrease in the current levels of IL-6, as well as an increase in the expression of IL-10 in active individuals [ 71 ]. A low presence of circulating pro-inflammatory cytokines is observed in contrast to the increase in anti-inflammatory cytokines. Thus, there are positive changes in the immune system of these individuals, including enhancements in host response and vaccine immunoprotection [ 70 ].
Similarly, while prolonged maintenance or worsening of obesity and MS perpetuates deregulation of immune responses, promoting greater risks of the individual developing diseases and increasing their vulnerability to infection by the novel coronavirus [ 43 , 59 , 61 ]. An association between physical activity and reduction of inflammatory markers in obese and overweight patients is suggested [ 13 ].
Luzi and Radaelli [ 72 ] add the lack of physical activity as an important factor among obese patients, as it impairs the immune response against microbial agents, from the activation of macrophages to the inhibition of pro-inflammatory cytokines. On the other hand, both metabolic health and immune health benefit from the practice of physical activity, which reduces the risk of infectious complications. Thus, regular physical exercise appears as a preventive measure in the defense of the host against viral infection [ 72 ].
Muscle contraction is responsible for the transient increase in circulating levels of IL-6 cytokine, in proportion to the duration of physical activity and the amount of muscle mass recruited. The elevation of this interleukin seems to be followed by increases in antinflammatory cytokines, such as IL-10, released by cells of innate immunity and responsible for promoting an antinflammatory environment, inhibiting inflammatory mediators to limit tissue damage. This effect may be beneficial in cases of chronic inflammation, such as obesity, T2DM and MS, and may reduce the risk of a pathogenic inflammatory response such as the cytokine storm present in severe COVID-19 [ 40 , 59 ].
In addition, IL-10 is associated with enhanced insulin sensibility and glycemic metabolism [ 40 ]. Physical practice is able to reduce the excessive concentration of pro-inflammatory adipocin leptin and improve sensitivity to leptin and insulin [ 72 , 73 ]. In patients with T2DM and new coronavirus infection, good glycemic control has been associated with better prognosis in COVID-19 [ 59 ].
Thus, physical exercise is shown to be an immunomodulatory and non-pharmacological intervention, achieving positive immunomodulation through exercises of light to moderate intensity [ 72 ]. Through exercise, there is an improvement in the response to infection in obese individuals, due to immune and cellular restoration [ 74 ]. Although COVID-19 is not primarily a metabolic disease, there is a need to maintain metabolic control of glucose, lipid levels and blood pressure in order to prevent metabolic and cardiovascular complications, as well as to reduce the local inflammatory response and block the virus entering the cells [ 75 ].
As seen, innate immunity has an important role in the pathogenesis of COVID-19 and ARDS, due to inflammatory cascades, recruitment of neutrophils, macrophages and DC cells and increased production of ROSs. In turn, by the modulation mechanism of chemokine production, physical training and therapeutic exercises can attenuate alveolar neutrophilia in the face of lung injury [ 41 ].
Furthermore, the expression of the extracellular superoxide dismutase enzyme (EcSOD), an important antioxidant in the body and highly present in the lungs, is enhanced by resistance physical activity and was associated with inhibition of endothelial activation and inflammatory adhesion, with potential benefit to reduce oxidative stress and tissue damage in COVID-19 [ 41 ]. The amplification of the antioxidant defense generated by routine physical activity also contributes to immunological surveillance [ 13 ].
Moreover, Womack, Nagelkirk and Coughlin [ 76 ] point out that through the intensity of physical exercise, a change in the potential for coagulation, platelet aggregation and fibrinolysis can be seen. As an example, there is long-term training through aerobic exercises where a decrease in the clotting potential is observed in healthy individuals. Thus, it is suggested that the practice of physical exercises contributes to reducing the risk of ischemic events depending on their intensity and duration [ 76 ] and may contribute to attenuating coagulation disorders associated with SARS-CoV-2 infection.
The anti-inflammatory, antioxidant and endothelial activation inhibitor benefits may also be linked to the reduction in hypercoagulability related to COVID-19. This is because, as previously mentioned, the exacerbated activation of the immune system increases the expression of the tissue factor and, consequently, the predisposition to the formation of thrombi; in addition, metabolic disorders, oxidant stress and changes in senescence positively stimulate the vasoconstrictor axis ECA/Ang/AT1R, contributing to endothelial imbalances [ 46 , 47 , 49 – 53 , 69 ]. Therefore, the immunometabolic improvements promoted by physical exercise may help in the control of coagulation disorders in the COVID-19 (Fig. 4 ).

Benefits of regular moderate-intensity physical activity on factors that influence the response against to COVID-19.
Finally, in view of the quarantine status adopted in several countries as a measure to prevent and control the spread of SARS-CoV-2 during the COVID-19 pandemic, social isolation and restrictions on the movement of people reduced the practice of physical activity, predisposing the population to adopt sedentary behavior [ 77 ]. Social distancing is a sine qua non in reducing the speed of contagion of COVID-19 and associated deaths. However, due to these measures, sports clubs, gyms and fitness spaces have suspended their activities in order to reduce agglomerations; thus, difficulties regarding physical exercise were imposed [ 78 ].
Therefore, despite being one of the main strategies against COVID-19, social isolation has been related to behavioral and physiological changes, including the increased prevalence of sedentarism and eating disorders (food compulsion, hyperphagia), resulting in negative consequences for metabolic health, such as weight gain, growth of fat tissue, hyperglycemia and insulin resistance and loss of muscle tissue [ 59 ]. Since this condition can harm the body’s defenses and contribute significantly to the reduction in individuals’ physical condition, functional and health loss, the adoption of healthy habits and an exercise routine can help in maintaining health [ 59 , 69 ].
It is significant to consider that contexts like this increase the susceptibility to stressful events and elevations of glucocorticoids (cortisol), with consequent inhibition of the functions of NK cells and TCD8 + lymphocytes in the antiviral response. However, good physical conditioning was associated with lower risks of reactivation of latent viral infections in situations of isolation and confinement, indicating a favored immune system compared to individuals with less physical fitness [ 16 ].
Physical activity is considered a non-medication practice for the prevention and treatment of diseases of psychological, physical and/or metabolic origin [ 78 ]. Regular physical exercise should be encouraged during social isolation as a preventive measure for health, given that exercise is essential during the period of fight against the spread of coronavirus [ 69 ].
The American College of Sports Medicine (ACSM) recommends that the practice of moderate physical exercise should be maintained during the quarantine period, since it helps in the immune reinforcement against SARS-CoV-2. The WHO recommends that asymptomatic and healthy individuals should exercise at least 150 min per week for adults and 300 min per week for children and adolescents. These times can be distributed during the days of the week and according to the person’s routine [ 69 ]. It is important to emphasize that physical activity should be interrupted and a health professional should be consulted in case symptoms such as fever, dyspnea at rest and dry cough are manifested, because these symptoms can be related to COVID-19 [ 69 , 79 ].
In social isolation, the home environment has become the ideal and necessary place for physical activity. Activities that are satisfactory and that allow better exploring the home space should be sought. Activities of daily living such as organization of spaces, cleaning and maintenance also help in coping with COVID-19. In environments with children, playing and exercising with them is a great way to promote energy expenditure, thus leaving the beginning of sedentary rest. Meditation, stretching and relaxation are allies in combating a sedentary lifestyle. It is important to avoid long rest periods, which should be intercalated with active practices [ 69 ].
The ACSM has published guidelines for moderate-intensity activities that can be practiced during the pandemic period, including aerobic exercises and strength training, indoors, like at home, or outdoors, when permitted by government authorities. Options for aerobic activities to be performed at home include walk briskly around the house, up- and downstairs, dancing and jumping rope. When possible, walking or running outdoors, cycling, gardening work and family games are interesting alternatives, as long as infection prevention measures are maintained [ 79 ].
Among the strength activities, ACSM indicates downloading a strength workout app that does not require any equipment and suggests exercises such as squat, sit-ups, push-ups, lunges and yoga practice, which can also help in anxious states [ 79 ]. Oliveira Neto et al. [ 80 ] suggest resistance exercises based on ACSM recommendations to be performed at home, including exercises involving the muscles of the lower body, upper body and limbs, and lower limbs, which can be adapted for beginners in physical practice or experienced people.
Activities that make use of the individual’s own body weight, associated with resistance training as well as the use of elastic bands, provide excellent health results, results similar to those achieved by traditional gyms. Thus, objects such as backpacks, books, market bags and water bottles can be used as an auxiliary tool in resistance physical activity. Exercises such as squats, jumping jacks and going up and down steps can be effective in physical training [ 78 ].
As for the intensity and volume of physical exercise practices, these must be moderate, as they exceed both the volume and the intensity, effects such as momentary immunosuppression are achieved, thus providing greater vulnerability as to the contagion of the novel coronavirus. If individuals want to practice high-intensity exercises, a reduction in exercise volume should be adopted as a preventive measure, in order to avoid strenuous exercises [ 78 ].
In addition, technological tools can contribute to the better performance of these activities in the home environment, as video calls with a physical education professional facilitate the orientation of the exercises to be performed, providing support that has, as a consequence, better results and greater safety in the execution of the exercises. Regardless of whether or not you are in the risk group for COVID-19, regular exercise, according to the ACSM, should be regularly performed, given that it aims to improve the immune system, reduce stress perceived and decrease anxiety disorders [ 78 ].
There are still gaps in the knowledge regarding the pathogenic mechanisms involved in SARS-CoV-2 infection. However, there is consensus in the scientific literature about the important involvement of the immune system in the susceptibility, progression and outcome of COVID-19. The imbalance in innate and adaptive immune responses, characterized mainly by changes such as cytokine storm and lymphopenia, in addition to the disorders in coagulation- and host-related conditions, including obesity, metabolic syndrome and aging (immunosenescence), is among the factors notoriously associated with a worse prognosis of infection.
The benefits of exercise—regular and at appropriate intensity levels—for the immune system in respiratory infections such as COVID-19 include increased immunovigilance and improved immune competence, which help in the control of pathogens, a fact that becomes more important considering the immunosenescence and susceptibility of the elderly population to severe infection. Other favorable effects in relation to host factors, such as prevention or reduction of overweight, increased physical and cardiopulmonary conditioning, attenuation of the systemic pro-inflammatory and pro-thrombotic states, decrease in oxidative stress, improvements in glycemic, insulinic and lipidic metabolisms, besides the enhancement of the vaccination response, also indicate how adequately physical activity can help the organism’s immune response against COVID-19.
In the COVID-19 pandemic situation, adopting mitigation practices is an essential strategy to reduce the risks related to the novel coronavirus infection. These interventions include the use of personal protective equipment (PPE), adherence to hygiene procedures and social isolation measures, as well as actions that lead to a healthier lifestyle, minimize stress factors and strengthen the immune system, such as regular physical activity.
However, remaining active at appropriate levels seems to be a challenge in a context of confinement and social isolation, which emphasizes the importance of developing training with recommendations adapted to the new routine of the population. Fortunately, there are viable alternatives for performing physical exercises in restricted environments, enabling the population to enjoy the advantages of physical training for health in the context of OVID-19.
Finally, future studies that deepen the relationship between physical activity and infection by SARS-CoV-2, including the influences of exercise on metabolic and immunological disorders present in COVID-19, will certainly be relevant in view of the probable benefits already mentioned and considering the impacts of infection by the novel coronavirus in the global context. Faced with the possibility of new pandemics by previously unknown microorganisms, without totally effective prevention measures, vaccines or specific treatments of proven efficacy, the host organism’s capacity against infections becomes the most important line of defense, thus emphasizing the importance of investing in lifestyle habits that promote health and well-being, such as the practice of physical activity.
Authors’ contributions
DTRS had the idea for the article. ES, KKSF e MRB performed the literature search, data analysis and translation. MPS drafted and revised the work.
Not applicable.
Compliance with ethical standards
The authors declare that they have no conflict of interest.
This article does not contain any studies with human participants or animals performed by any of the authors, and informed consent is not a standard required.
Publisher's Note
Springer Nature remains neutral with regard to jurisdictional claims in published maps and institutional affiliations.
Contributor Information
Matheus Pelinski da Silveira, Email: moc.liamg@iksnilepsuehtam .
Kimberly Kamila da Silva Fagundes, Email: moc.liamtoh@k-ylrebmyk .
Matheus Ribeiro Bizuti, Email: [email protected]_suehtam .
Édina Starck, Email: [email protected] .
Renata Calciolari Rossi, Email: rb.moc.arret@iraloiclacataner .
Débora Tavares de Resende e Silva, Email: [email protected] .
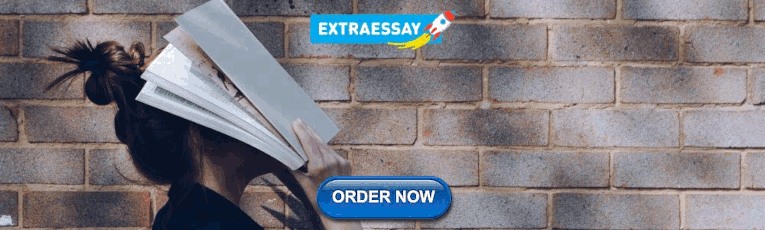
IMAGES
VIDEO
COMMENTS
Sedentary lifestyle is a major risk factor for noncommunicable diseases such as cardiovascular diseases, cancer and diabetes. It has been estimated that approximately 3.2 million deaths each year are attributable to insufficient levels of physical activity. We evaluated the available evidence from Cochrane systematic reviews (CSRs) on the effectiveness of exercise/physical activity for various ...
In one review, exercise was found as one of the nondeployment-related factors positively impacting well-being of military personnel ... A comprehensive review on physical exercise, physical fitness, activities of daily living, and quality-of-life. ... A literature review. Int J Geriatr Psychiatry. 2014; 29 (8):771-783. [Google Scholar]
2. Definitions of Physical Activity, Exercise, Training, Sport, and Health. Definitions and terms are based on "Physical activity in the prevention and treatment of disease" (FYSS, www.fyss.se [Swedish] []), World Health Organization (WHO) [] and the US Department of Human Services [].The definition of physical activity in FYSS is: "Physical activity is defined purely physiologically, as ...
Physical exercise is a potential intervention for enhancing cognitive function across the lifespan. However, while studies employing long-term exercise interventions consistently show positive ...
Background: The aim of the present work is the elaboration of a systematic review of existing research on physical fitness, self-efficacy for physical exercise, and quality of life in adulthood.
Abstract. Purpose of review: The health benefits of physical activity and exercise are clear; virtually everyone can benefit from becoming more physically active. Most international guidelines recommend a goal of 150 min/week of moderate-to-vigorous intensity physical activity. Many agencies have translated these recommendations to indicate ...
Available data in literature suggest that physical exercise also positively affects cognitive domains of ADHD (attention deficit hyperactivity disorder) children ... The second purpose of our review concerns exercise addiction and any individual and situational factors that have been considered as possible predictors for the onset of a sport ...
The links between increased participation in Physical Activity (PA) and improvements in health are well established. As this body of evidence has grown, so too has the search for measures of PA with high levels of methodological effectiveness (i.e. validity, reliability and responsiveness to change). The aim of this "review of reviews" was to provide a comprehensive overview of the ...
Physical activity reduces cardiovascular risk through lowering of blood pressure, improved glucose tolerance, reduced obesity, improvement in lipid profile, enhanced fibrinolysis, improved endothelial function and enhanced parasympathetic autonomic tone. Conclusion: Physical exercise has many health benefits and the evidence for this continues ...
The existing literature suggests that physical activity (PA), exercise, and aerobic fitness promote well-being and reduce risk of mental health problems. In this review, we focus on PA, exercise, and fitness as modifiable resilience factors that may help promote self-regulation via strengthening of top-down control of bottom-up processes in the ...
This study aimed to examine the relationship between physical activity and happiness through systematically reviewing the existing literature. A systematic search of major databases including PubMed/Medline, PsychInfo, SportDiscus, and Embase was performed in 2017 for original research articles published post-1980 with the related keywords of happiness and physical activity. From 1142 ...
Substantial evidence has established the value of high levels of physical activity, exercise training (ET), and overall cardiorespiratory fitness in the prevention and treatment of cardiovascular diseases. This article reviews some basics of exercise physiology and the acute and chronic responses of ET, as well as the effect of physical activity and cardiorespiratory fitness on cardiovascular ...
1. Introduction. Physical activity refers to any bodily movement that is produced by the skeletal muscles and results in energy expenditure; whilst exercise is a subset of physical activity since it is a planned, structured and repetitive process that aims to maintain and improve physical fitness (Caspersen et al., 1985).There is a growing body of interest that physical activity and exercise ...
The literature review summary has 4 main sections. This Introduction provides background information about the rationale for focusing on older adults and the process for reviewing the literature and developing the conclusions. The Methods utilized by the Literature Review Team are detailed in the following section.
A recent systematic review revealed that evening exercise may positively affect sleep, but vigorous exercise might impair sleep-onset latency, total sleep time [Citation 9]. The relationship between PA intensity (i.e. moderate exercise, vigorous exercise) and sleep quality needs to be specified even though the benefits of physical exercise and ...
Level of physical activity. Recommendations regarding the level of PA required for physical health benefits and the prevention of chronic disease, across the lifespan, are well established (U.S. Department of Health and Human Services, Citation 2018).It is recommended that children and adolescents aged 6-17 years engage in PA of moderate to vigorous intensity for a minimum of 60 min per day.
Exercise and physical activity (PA) reduce the risk of cardio- and cerebrovascular disease, cancer, obesity, and falls, and improve mental health, ... This systematic literature review is the first to investigate effect sizes of PA promotion counselling in primary care settings. Though only interventions based on counselling were included, the ...
The Coronavirus disease-19 (COVID-19) pandemic affected countries worldwide and has changed peoples' lives. A reduction in physical activity and increased mental health problems were observed, mainly in the first year of the COVID-19 pandemic. Thus, this systematic review aims to examine the association between physical activity and mental health during the first year of the COVID-19 pandemic.
The relationship between physical exercise and brain health is a burgeoning field of research in neuroscience, with a pivotal impact on our understanding of cognitive well-being, mental health, and aging. Existing studies evidence the positive influences of regular physical activity on brain health, suggesting its implications on learning, memory, and mood. Despite significant advancements ...
A large body of literature examines the impact of physical activity and exercise on mental health. There is a growing interest in the use of exercise in the treatment of depression and anxiety, as a primary option or as an adjunct to pharmacological treatment. A literature review was completed to identify the evidence
The concepts of physical activity, exercise, and sports in relation to mental health are the focus of this special issue of The International Review of Psychiatry. The issue covers a broad topic as there are many facets to the theme of physical activity and mental health, such as wellness, aging, and concussion management.
Background: Currently, little is known about early mobilization and exercise in individuals with COVID-19. Objective: To describe the indication and safety of early mobilization and exercises in mild to severe COVID-19 patients and to investigate the use of telerehabilitation to deliver exercise programs to these patients. Methods: This narrative literature review was conducted performing a ...
Exercise is the standard treatment for fatigue in heart failure (HF) patients. However, no study has investigated the effect of exercise on improving fatigue and HR-QoL in HF patients. Our study adhered to the Cochrane Handbook for Systematic Reviews of Interventions and followed the PRISMA statement. The date of the last search was October 31 ...
In her review of research on meaning making, Park (2010) encountered similar difficulties, leading her to conclude that due to its "nebulous contours" (p. 267) an exhaustive review of all pertinent literature on the topic was impossible. We therefore opted for a narrative review as a scholarly report of a body of literature aiming to ...
Physical exercise and yoga may help in the management of cravings for substances, especially in people who may not have access to other forms of therapy. ... This review also analysed published literature from India to understand the effects of exercise on mental health and the implications for disease management and treatment in the Indian ...
This review will focus on the nutrition-related perturbations that may be experienced during high-intensity exercise HA and gaps in current literature. Future directions for research in nutritional readiness during heat stress is also overviewed. ... multiple studies of high-intensity exercise HA displaying impaired physical performance ...
Background Pilates has captured interest due to its possible advantages during pregnancy and childbirth. Although research indicates that Pilates may reduce labor duration, alleviate pain, and improve satisfaction with the childbirth experience, consensus on these outcomes remains elusive, underscoring the necessity for additional studies. Aim This systematic review and meta-analysis aimed to ...
Physical exercise is an intervention that may address the broad range of QOL issues following cancer diagnosis including physical, functional, psychological, emotional, and social well-being. The purpose of the present article was to provide a comprehensive and critical review of the topic and to offer suggestions for future research.
The impacts can be very serious because cyber-attacks can penetrate operations carried out in the physical infrastructure, causing explosions, crashes, collisions, and other incidents. This research is a thematic literature review of the deterrence to such attacks by protecting IoT devices by employing provenance blockchain and artificial ...
Therefore, the present article aims to perform an integrative review of the literature relating the role of physical exercise on the immune system in the fight against COVID-19. For this purpose, the bibliographic study included knowledge about respiratory infections, influences of physical exercise on the immune system and proposed the ...