An official website of the United States government
Here’s how you know
Official websites use .gov A .gov website belongs to an official government organization in the United States.
Secure .gov websites use HTTPS A lock ( Lock Locked padlock icon ) or https:// means you’ve safely connected to the .gov website. Share sensitive information only on official, secure websites.
- Entire Site
- Research & Funding
- Health Information
- About NIDDK
- Diabetes Overview
- What Is Diabetes?
- Monogenic Diabetes
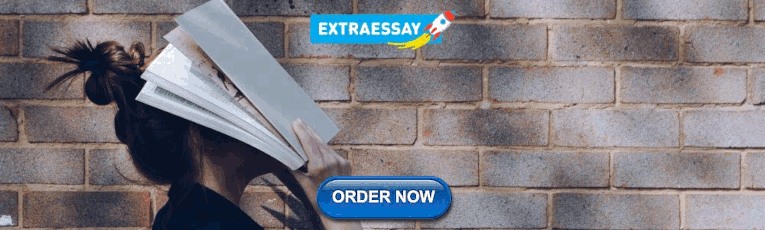
Monogenic Diabetes (Neonatal Diabetes Mellitus & MODY)
On this page:
What are monogenic forms of diabetes?
What is neonatal diabetes mellitus (ndm), what is maturity onset diabetes of the young (mody), how is monogenic diabetes diagnosed, what do i need to know about genetic testing and counseling, how is monogenic diabetes treated and managed, clinical trials.
The most common forms of diabetes, type 1 and type 2 , are polygenic, meaning they are related to a change, or defect, in multiple genes. Environmental factors, such as obesity in the case of type 2 diabetes, also play a part in the development of polygenic forms of diabetes. Polygenic forms of diabetes often run in families. Doctors diagnose polygenic forms of diabetes by testing blood glucose, also known as blood sugar, in individuals with risk factors or symptoms of diabetes.
Genes provide the instructions for making proteins within the cell. If a gene has a change or mutation, the protein may not function properly. Genetic mutations that cause diabetes affect proteins that play a role in the ability of the body to produce insulin or in the ability of insulin to lower blood glucose . People typically have two copies of most genes, with one gene inherited from each parent.
Some rare forms of diabetes result from mutations or changes in a single gene and are called monogenic. In the United States, monogenic forms of diabetes account for about 1 to 4 percent of all cases of diabetes. 1,2,3,4 In most cases of monogenic diabetes, the gene mutation is inherited from one or both parents. Sometimes the gene mutation develops spontaneously, meaning that the mutation is not carried by either of the parents. Most mutations that cause monogenic diabetes reduce the body’s ability to produce insulin, a protein produced in the pancreas that helps the body use glucose for energy.
Neonatal diabetes mellitus (NDM) and maturity-onset diabetes of the young (MODY) are the two main forms of monogenic diabetes. NDM occurs in newborns and young infants. MODY is much more common than NDM and usually first occurs in adolescence or early adulthood.
Most cases of monogenic diabetes are incorrectly diagnosed. For example, when high blood glucose is first detected in adulthood, type 2 diabetes is often diagnosed instead of monogenic diabetes. If your health care provider thinks you might have monogenic diabetes, genetic testing may be needed to diagnose it and to identify which type. Testing of other family members may also be indicated to determine whether they are at risk for or already have a monogenic form of diabetes that is passed down from generation to generation. Some monogenic forms of diabetes can be treated with oral diabetes medicines (pills), while other forms require insulin injections. A correct diagnosis allows for proper treatment and can lead to better glucose control and improved health in the long term.
NDM is a monogenic form of diabetes that occurs in the first 6 to 12 months of life. NDM is a rare condition accounting for up to 1 in 400,000 infants in the United States. 4 Infants with NDM do not produce enough insulin, leading to an increase in blood glucose. NDM is often mistaken for type 1 diabetes, but type 1 diabetes is very rarely seen before 6 months of age. Diabetes that occurs in the first 6 months of life almost always has a genetic cause. Researchers have identified a number of specific genes and mutations that can cause NDM. In about half of those with NDM, the condition is lifelong and is called permanent neonatal diabetes mellitus (PNDM). In the rest of those with NDM, the condition is transient, or temporary, and disappears during infancy but can reappear later in life. This type of NDM is called transient neonatal diabetes mellitus (TNDM).
Clinical features of NDM depend on the gene mutations a person has. Signs of NDM include frequent urination, rapid breathing, and dehydration. 5 NDM can be diagnosed by finding elevated levels of glucose in blood or urine. The lack of insulin may cause the body to produce chemicals called ketones , resulting in a potentially life-threatening condition called diabetic ketoacidosis . Most fetuses with NDM do not grow well in the womb, and newborns with NDM are much smaller than those of the same gestational age, a condition called intrauterine growth restriction. After birth, some infants fail to gain weight and grow as rapidly as other infants of the same age and sex. Appropriate therapy may improve and normalize growth and development.
MODY is a monogenic form of diabetes that usually first occurs during adolescence or early adulthood. MODY accounts for up to 2 percent of all cases of diabetes in the United States in people ages 20 and younger. 3
A number of different gene mutations have been shown to cause MODY, all of which limit the ability of the pancreas to produce insulin. This leads to high blood glucose levels and, in time, may damage body tissues, particularly the eyes, kidneys, nerves, and blood vessels.
Clinical features of MODY depend on the gene mutations a person has. People with certain types of mutations may have slightly high blood sugar levels that remain stable throughout life, have mild or no symptoms of diabetes, and do not develop any long-term complications. Their high blood glucose levels may only be discovered during routine blood tests. However, other mutations require specific treatment with either insulin or a type of oral diabetes medication called sulfonylureas.
MODY may be confused with type 1 or type 2 diabetes. In the past, people with MODY did not generally have overweight, obesity, or other risk factors for type 2 diabetes, such as high blood pressure or abnormal blood fat levels. However, as more people in the United States become overweight or have obesity, people with MODY may also be overweight or have obesity.
Although both type 2 diabetes and MODY can run in families, people with MODY typically have a family history of diabetes in multiple successive generations, meaning MODY is present in a grandparent, a parent, and a child.
Genetic testing can diagnose most forms of monogenic diabetes. A correct diagnosis with proper treatment should lead to better glucose control and improved health in the long term.
Genetic testing is recommended if 6
- diabetes is diagnosed within the first 6 months of age
- diabetes is diagnosed in children and young adults, particularly those with a strong family history of diabetes, who do not have typical features of type 1 or type 2 diabetes, such as the presence of diabetes-related autoantibodies, obesity, and other metabolic features
- a person has stable, mild fasting hyperglycemia, especially if obesity is not present

Genetic testing for monogenic diabetes involves providing a blood or saliva sample from which DNA is isolated. The DNA is analyzed for changes in the genes that cause monogenic diabetes. Genetic testing is done by specialized labs.
Abnormal results can determine the gene responsible for diabetes in a particular individual or show whether someone is likely to develop a monogenic form of diabetes in the future. Genetic testing can be helpful in selecting the most appropriate treatment for individuals with monogenic diabetes. Testing is also important in planning for pregnancy and to understand the risk of having a child with monogenic diabetes if you, your partner, or your family members have monogenic diabetes.
Most forms of NDM and MODY are caused by autosomal dominant mutations, meaning that the condition can be passed on to children when only one parent carries or has the disease gene. With dominant mutations, a parent who carries the gene has a 50 percent chance of having an affected child with monogenic diabetes.

In contrast, with autosomal recessive disease, a mutation must be inherited from both parents. In this instance, a child has a 25 percent chance of having monogenic diabetes.
For recessive forms of monogenic diabetes, testing can indicate whether parents or siblings without disease are carriers for recessive genetic conditions that could be inherited by their children.
While not as common, it is possible to inherit mutations from the mother only (X-linked mutations). Also not as common are mutations that occur spontaneously.

More information about the genes that cause NDM and MODY, the types of mutations responsible for the disease (autosomal dominant, autosomal recessive, X-linked, etc.), and clinical features is provided in the American Diabetes Association Standards of Medical Care in Diabetes .
If you suspect that you or a member of your family may have a monogenic form of diabetes, you should seek help from health care professionals—physicians and genetic counselors—who have specialized knowledge and experience in this area. They can determine whether genetic testing is appropriate; select the genetic tests that should be performed; and provide information about the basic principles of genetics, genetic testing options, and confidentiality issues. They also can review the test results with the patient or parent after testing, make recommendations about how to proceed, and discuss testing options for other family members.
Treatment varies depending on the specific genetic mutation that has caused a person’s monogenic diabetes. People with certain forms of MODY and NDM can be treated with a sulfonylurea, an oral diabetes medicine that helps the body release more insulin into the blood. Other people may need insulin injections. Some people with MODY may not need medications and are able to manage their diabetes with lifestyle changes alone, which include physical activity and healthy food choices. Your physician and diabetes care team will work with you to develop a plan to treat and manage your diabetes based on the results of genetic testing.
The National Institute of Diabetes and Digestive and Kidney Diseases (NIDDK) and other components of the National Institutes of Health (NIH) conduct and support research into many diseases and conditions.
What are clinical trials, and are they right for you?
Clinical trials are part of clinical research and at the heart of all medical advances. Clinical trials look at new ways to prevent, detect, or treat disease. Researchers also use clinical trials to look at other aspects of care, such as improving the quality of life for people with chronic illnesses. Find out if clinical trials are right for you .
What clinical trials are open?
Clinical trials that are currently open and are recruiting can be viewed at www.ClinicalTrials.gov .
This content is provided as a service of the National Institute of Diabetes and Digestive and Kidney Diseases (NIDDK), part of the National Institutes of Health. NIDDK translates and disseminates research findings to increase knowledge and understanding about health and disease among patients, health professionals, and the public. Content produced by NIDDK is carefully reviewed by NIDDK scientists and other experts.
The NIDDK would like to thank: Louis Philipson, M.D., Ph.D., University of Chicago, Department of Medicine, Endocrinology, Director, Kovler Diabetes Center
REVIEW article
Neonatal diabetes mellitus.

- 1 Paediatric Endocrinology, Gynaecology and Diabetology, Necker–Enfants Malades University Hospital, Assistance Publique-Hôpitaux de Paris, IMAGINE Institute, ENDO-European Reference Network Team, Paris, France
- 2 Faculty of Medicine, Université de Paris, Paris, France
- 3 INSERM U1016, Cochin Institute, Paris, France
- 4 Paediatric Endocrinology, Diabetology and Obesity Unit, Lausanne University Hospital, University of Lausanne, Lausanne, Switzerland
- 5 Inserm UMR-1018-CESP, Necker–Enfants Malades University Hospital Paedopsychiatry Department, Cochin University Hospital Paediatrics Department, Institut Universitaire de France, Assistance Publique-Hôpitaux de Paris, Université de Paris, Paris, France
- 6 Genetics Department, Robert-Debré University Hospital, Assistance Publique-Hôpitaux de Paris, Paris, France
- 7 INSERM U1138, Cordeliers Research Centre, Paris, France
Neonatal Diabetes (ND) mellitus is a rare genetic disease (1 in 90,000 live births). It is defined by the presence of severe hyperglycaemia associated with insufficient or no circulating insulin, occurring mainly before 6 months of age and rarely between 6 months and 1 year. Such hyperglycaemia requires either transient treatment with insulin in about half of cases, or permanent insulin treatment. The disease is explained by two major groups of mechanism: malformation of the pancreas with altered insulin-secreting cells development/survival or abnormal function of the existing pancreatic β cell. The most frequent genetic causes of neonatal diabetes mellitus with abnormal β cell function are abnormalities of the 6q24 locus and mutations of the ABCC8 or KCNJ11 genes coding for the potassium channel in the pancreatic β cell. Other genes are associated with pancreas malformation or insufficient β cells development or destruction of β cells. Clinically, compared to patients with an ABCC8 or KCNJ11 mutation, patients with a 6q24 abnormality have lower birth weight and height, are younger at diagnosis and remission, and have a higher malformation frequency. Patients with an ABCC8 or KCNJ11 mutation have neurological and neuropsychological disorders in all those tested carefully. Up to 86% of patients who go into remission have recurrent diabetes when they reach puberty, with no difference due to the genetic origin. All these results reinforce the importance of prolonged follow-up by a multidisciplinary pediatric team, and later doctors specializing in adult medicine. 90% of the patients with an ABCC8 or KCNJ11 mutation as well as those with 6q24 anomalies are amenable to a successful switch from insulin injection to oral sulfonylureas.
Diabetes mellitus in very young children or neonatal diabetes is a rare genetic disease (minimal incidence: 1 in 90,000 live births) with variations within different ethnic groups ( 1 – 3 ). It is defined by the presence of severe hyperglycaemia requiring treatment and occurs between the neonatal period and infancy. It occurs mainly before 6 months of age (155/173 probands in our published cohort) and rarely between 6 months and 1 year (18/173) ( 4 ). In the Finnish population for example, after 6 months of age, patients with diabetes had high HLA risk genotypes and islet autoantibodies, reflecting the autoimmune character of diabetes ( 5 ). This hyperglycaemia is associated with insufficient or no circulating insulin ( 3 ). Two clinical forms have been distinguished, based on the duration of the treatment: a so called “transient form” and a permanent form.
The disease is explained by two major groups of mechanism: malformation of the pancreas or abnormal function of the pancreatic β cell that secretes insulin (by poor insulin cell mass development or malfunction of a cell component or by destruction of the β cell) ( Table 1 ) (see Figure 1 for the normal functioning of the β cell).

Table 1 . Genetic causes of monogenic neonatal diabetes based on physiopathological mechanisms [excluding 6q24 locus abnormalities (MIM *601410, *603044, and *612192)].

Figure 1 . Mechanism of insulin secretion in response to glucose and glibenclamide.
Genetic Causes
Abnormal β cell function.
The most frequent genetic causes of neonatal diabetes with normal pancreas morphology are abnormalities of the 6q24 locus and mutations of the genes coding for the ATP-dependent potassium channel.
6q24 (MIM#601410 and 603044)
The first genetic causes identified were abnormalities of the 6q24 locus, which include paternal uniparental disomy of 6q24 (pUPD6), partial duplication of paternal 6q24 and relaxation of the maternal 6q24 imprinted locus. This locus contains a CpG island, presenting differential methylation depending on the parental origin (non-methylation on the paternal allele, methylation on the maternal allele) ( 6 ). To date, the methylation abnormality has not been found in the parents of affected children. Methylation is used to down-regulate gene transcription of the methylated allele. All these abnormalities lead to over-expression of imprinted genes located in 6q24, such as PLAGL1/ZAC (pleiomorphic adenoma gene-like 1) and HYMAI (Hydatidiform mole-associated and imprinted transcript), which are the most “likely” candidate genes ( 6 – 8 ). PLAGL-1 codes for a transcription factor involved in regulation of stopping the cell cycle and apoptosis and in induction of the receptor 1 gene for human pituitary adenylate cyclase-activating polypeptide (PACAP1, which is a potent stimulant of insulin secretion). The function of the HYMAI gene is unknown ( 9 ). The mechanism responsible for the diabetes could be linked to a developmental defect in the β cells but the fact that remission of the diabetes occurs means that an abnormality in β cell function cannot be ruled out ( 10 ). The 6q24 abnormalities are associated with “transient” neonatal diabetes ( 7 , 8 , 11 ).
The ZFP57 gene (MIM * 612192) is involved in maintaining methylation of the DNA during the very early stages of embryogenesis. It is localized at 6p22.1. Homozygous mutations leading to a lack of protein or non-functional protein are associated with widespread DNA hypomethylation, including hypomethylation of the 6q24 locus ( 12 ). However, there are patients who have a 6q24 methylation abnormality not due to mutations of this gene ( 12 ).
Mutations of the ABCC8 and KCNJ11 Genes Coding for the K ATP Channel: (MIM * 600509 and * 600937)
The ATP-dependent potassium channel (K ATP channel) plays a central role in stimulating insulin secretion by the pancreatic β cell in response to glucose. At low blood sugar levels (e.g., fasting), the K ATP channels are open (activated) and their activity maintains a hyperpolarized resting membrane potential (around −70 mV). A rise in blood sugar level (e.g., post-prandial) causes increased passage of glucose into the β cell. Glucose enters the glycolysis pathway, which increases the intracellular ATP concentration. This causes the K ATP channels to close (inhibition), which leads to the intracellular potassium accumulation that causes membrane depolarization. This depolarization activates the voltage-dependent calcium channels, leading to Ca 2+ ions entering the β cell, then enabling exocytosis of the secretion vesicles and release of insulin into the bloodstream ( Figure 1 ).
The K ATP channel is an octamer formed from two types of subunits: the Kir6.2 subunits form the channel selective for the incoming corrective potassium enclosed in SUR1 ion-channel regulator subunits ( 13 , 14 ). They are coded by the KCNJ11 and ABCC8 genes, respectively.
Activating mutations in one of these two genes are responsible for neonatal diabetes with normal pancreas morphology ( 15 – 17 ). They result in the K ATP channel remaining permanently open, so that it no longer controls membrane potential in response to glucose and therefore blocks the event cascade that leads to insulin release.
Mutations of the Insulin Gene (INS) (MIM * 176730)
The third cause of neonatal diabetes, by frequency, is mutations of the insulin gene ( INS ). The majority are heterozygous mutations affecting the structure of preproinsulin; these are transmitted in an autosomal dominant manner ( 18 , 19 ). The abnormal proinsulin undergoes degradation in the endoplasmic reticulum, leading to severe endoplasmic reticulum (ER) stress and β cell death. This process has been described in mouse models ( 20 ) and in man ( 21 , 22 ). Recent evidence suggests that INS mutations do not necessarily lead to beta-cell death but rather the chronic ER stress interferes with beta-cell growth and development ( 23 ).
Some mutations alter expression of the protein. They are transmitted in a recessive manner, in the majority of cases in consanguineous families. These mutations affect the insulin promoter directly of by mutation in factor that enhances its activity ( 24 , 25 ).
Mutations of the Glucokinase Gene (MIM * 138079)
Glucokinase is responsible for the first step of glucose metabolism in the β cell. It acts as a “sensor” of blood glucose, making it possible to control the quantity of insulin secreted. Nonsense mutations of the glucokinase gene cause MODY 2 (Maturity onset diabetes in the youth type 2), which usually presents as moderate hyperglycaemia ( 26 ). Transmission is heterozygous. In the homozygous state, these nonsense mutations cause neonatal diabetes by complete deficiency of glucokinase-mediated glycolysis ( 27 ). This is not a frequent cause of neonatal diabetes ( 28 , 29 ). However, an assay of the fasting blood glucose concentration is required from both parents, particularly if there is a history of gestational diabetes. The discovery of discreet glucose intolerance in both parents should therefore lead to a search for glucokinase gene mutations.
Abnormal Pancreas Morphology
Several genes are linked to neonatal diabetes with abnormal pancreas morphology and precise description is beyond the scope of this chapter (see Table 1 for a brief information). These genes are involved in development of the pancreas at various stages in early morphogenesis. These neonatal diabetes cases may be associated with a deficiency of the exocrine pancreas, based on the severity of pancreatic damage or to other congenital malformations. Mutation of the RFX-6 gene deserves a specific comment. The RFX-6 transcription factor is involved in the differentiation of beta-cells in the pancreas during embryonic development of the pancreas. It is also expressed in mature cells where it has a role in regulating insulin transcription and secretion. It actually controls the expression and activation of calcium channels and its inactivation alters insulin secretion in response to glucose. A few cases of neonatal diabetes have been reported. Patients display developmental abnormalities of the pancreas and of the digestive tract. The mechanism is linked to both a developmental and a functional disorder of the endocrine pancreas. Transmission is autosomal recessive ( Table 1 ).
Autoimmune Neonatal Diabetes Mellitus
Most patients diagnosed with diabetes between 6 and 12 months of age will have the “typical” type 1 diabetes mellitus seen in older children with positive autoantibodies against the beta cell. Autoimmune diabetes is very rare before 6 months of age and will most often be linked to specific causes.
IPEX Syndrome ( Table 1 )
Mutations of the FOXP3 gene may be responsible for enteropathy, immune dysregulation and polyendocrinopathy. It is a cause of neonatal diabetes associated with early autoimmunity directed against the beta cells of the pancreas. This diagnosis should be considered in male infants presenting diabetes associated with immune deficiency and/or severe infections. Immunosuppressant treatment can be considered (serolimus, corticosteroids) but bone marrow transplant must be considered as soon as the child's clinical condition allows. Insulin treatment will be combined with specialized nutritional management (parenteral ± enteral nutrition) before and after the transplant. It should be noted that, while correcting immune deficiencies, this will not eliminate the diabetes.
Down Syndrome and Neonatal Diabetes
Patients with Down syndrome (DS) resulting from trisomy 21 are more likely to have childhood diabetes mellitus. Professor Hattersley's group found 13 infants affected by DS who were diagnosed with diabetes before the age of 6 months. Trisomy 21 was seven times more likely in their PNDM cohort than in the general population (13 of 1,522 = 85 of 10,000 observed vs. 12.6 of 10,000 expected). Known PNDM genes explains 82.9% of non-DS PNDM in their work. None of the 13 DS-PNDM patients had a mutation in those genes. The conclusion from this work is that trisomy 21 is a cause of autoimmune PNDM that is not HLA associated ( 30 ).
Other mutations, such as the activating STAT3 mutations have been described which cause neonatal diabetes associated with beta-cell autoimmunity ( Table 1 ).
Clinical Description
There are two clinical forms of neonatal diabetes based on the duration of insulin-dependency. In the transient form, treatment may be stopped at any time from the first weeks of life to 5 years of age ( 4 ). In the permanent forms, life-long treatment is necessary.
The clinical difference between transient and permanent neonatal diabetes is not always underpinned by distinct molecular mechanisms. Abnormalities of the 6q24 locus are exclusively linked to transient neonatal diabetes. However, mutations of the ABCC8, KCNJ11 , and INS genes are linked to both permanent and transient forms ( 17 , 18 , 25 ). Other genetic causes are associated with permanent neonatal diabetes.
Neonatal diabetes is usually diagnosed before 6 months of age. However, the age of diagnosis varies depending on genetic causes: diabetes due to a 6q24 locus abnormality appears before the age of 1 month in 93% of cases and before the age of 3 months in 100% of cases. In ABCC8 and KCNJ11 gene mutations, it appears before the age of 1 month in 30% of cases and between 1 and 6 months in 66% of cases ( 4 ).
At birth, patients have a birth-weight below the 10th percentile in 62% of cases ( 4 ), highlighting the crucial role of insulin secretion in fetal growth. This intrauterine growth retardation is found in all genetic groups with a greater proportion in patients with a 6q24 abnormality than those carrying a ABCC8 or KCNJ11 mutation (92 vs. 48%, p < 0.001) ( 4 ).
Half of patients with a detectable pancreas by ultrasound experience remission from the diabetes in our cohort ( 4 ). This occurs at the age of about 4 months. There is a difference depending on the genetic cause. Patients with a 6q24 locus abnormality are in remission before the age of 1 year in 97% of cases (median age 14 weeks) while remission may go as far as the age of 5 years in patients with an ABCC8 or KCNJ11 mutation (median age 39 weeks) ( 4 , 31 ). Patients with a rare recessive mutation of the INS gene have remission at a median age of 12 weeks ( 24 ), whereas the majority of the INS gene mutations are dominant and they never go into remission. The diabetes frequently relapses (in up to 86% of cases) at the onset of puberty, probably due to the insulin resistance of puberty ( 4 , 32 ). There is no difference between the genetic groups.
Depending on the genetic cause, patients with neonatal diabetes may have other clinical signs associated with diabetes ( Table 1 ).
In neonatal diabetes with normal pancreas morphology, there are associated neurological disorders and developmental defects. Approximately 25% of patients with a mutation of the ABCC8 or KCNJ11 genes have neurological disorders ranging from psychomotor disorders to delayed cognitive development associated with severe epilepsy (DEND syndrome: Developmental delay, Epilepsy, and Neonatal Diabetes) ( 33 ). In addition, we have shown that when patients undergo detailed neuro-psychomotor and neuropsychological tests, an attention deficit or language disorder extending as far as dyslexia is found in 100% of cases ( 4 ).
Patients with a 6q24 locus abnormality may have developmental defects (macroglossia, umbilical hernia, cardiac malformations, renal and urinary malformations, non-autoimmune anemia, hypothyroidism with gland in situ ) and neurological disorders ( 4 , 11 ).
In neonatal diabetes with abnormal pancreas morphology or with β cell destruction, the associated malformations depend on the genetic causes and are often grouped into defined syndromes ( Table 1 ). Figure 2 illustrates a diagnostic strategy by molecular biology.

Figure 2 . Molecular biology approach to neonatal diabetes ( 44 ).
Recent long-term follow-up data in TNDM support a decrease in maximal insulin secretion capacity to both glucose and arginine stimuli that reflect low insulin mass ( 34 ). This study also showed that, regardless of the underlying genetic abnormalities or the duration of diabetes, TNDM was associated with learning difficulties at school. The high relapse rate and absence of identified predictors of relapse in TNDM suggest a need for an HbA1c assay at least every 2 years throughout childhood and for an HbA1c assay and oral glucose tolerance test every year throughout adolescence ( 34 ). During childhood, close attention should be directed to education and neurodevelopmental milestones, in TNDM patients with and without diabetes ( 34 ).
Therapeutic Aspects
Drug treatment.
Due to the early onset and associated delayed intrauterine retardation, patients with neonatal diabetes very often receive their initial treatment in a neonatal department. The initial treatment aims to rebalance carbohydrate metabolism. It should be started immediately following diagnosis. The treatment consists of the balance between a calorie and carbohydrate intake necessary to restore normal weight without being excessive to avoid the risk of future insulin resistance (15–18 g/kg/d carbohydrate) and sufficient insulin-based treatment to achieve the correct metabolic equilibrium. Restricting intake below the nutritional recommendations for children with low birth weight is ineffective given the physiopathology of circulating insulin deficiency.
Insulin-based treatment is difficult to manage due to the very low weight. The therapeutic margins between hypoglycemia and hyperglycemia are small, and both are harmful for neurological development of the newborn. Using an insulin pump with or without dilution of the insulin to 1:10 in 0.9% NaCl (or with a bona-fide diluent if available) can sometimes improve manageability of the insulin during the first weeks of life ( 35 , 36 ). Blood glucose meters must be able to give a reliable measurement of capillary blood sugar level with the smallest possible quantity of blood (e.g., 0.3 μl blood). Few “conventional” blood glucose meters meet this criterion. Conventional capillary measurements can be done on the side edge of all the fingers, using auto-lancets offering variable pricking depths. This offers the advantage of sparing newborns' heels. An alternative is to use continuous glucose sensors, either isolated or combined with an insulin pump. In addition to enabling rapid access to interstitial blood glucose (they provide a proxy but do not actually measure the blood glucose value), they can now be coupled to the insulin pump, making it possible to activate the system to stop the insulin pump during hypoglycemia or before it occurs. They also have the advantage of minimizing the number of pricks of the skin. Used under suitable hygiene conditions, there is no increase in skin infections. It is advisable to involve experienced clinicians when treating the child and using these techniques.
Patients with ABCC8 or KCNJ11 mutations are treated successfully using hypoglycemic sulfonylureas, which act by binding to the regulator SUR1 subunit of the potassium channel ( 37 ) ( Figure 1 ). The mutated channels remain sensitive to sulfonylureas in 90% of cases, having an inhibitory effect on the potassium channel of the pancreatic β cell and restoring insulin secretion in response to a meal ( 38 ). Sulfonylurea therapy appears to be safe and often successful in neonatal diabetes patients before genetic testing results are available ( 39 ). An empiric inpatient trial of sulfonylurea can be therefore considered ( 39 ). However, obtaining a genetic diagnosis remains imperative to inform long-term management and prognosis.
It has now been demonstrated that treatment with Sulfonylureas provide a better metabolic equilibrium than insulin by normalizing the HbA1c while strongly reducing the incidence of hypoglycemia in cases of neonatal diabetes with ABCC8 or KCNJ11 mutations. It was also shown recently that hypoglycemic sulphonylureas were able to improve neurological, neuropsychological and visuomotor impairment if they are introduced early in the child's life ( 33 , 40 , 41 ). Finally, a recent study has shown that it could sometimes be used successfully to replace insulin in neonatal diabetes associated with chromosome 6 methylation abnormalities ( 42 ). This emphasizes the importance of making a genetic diagnosis rapidly after diagnosing neonatal diabetes, and especially the early introduction of sulphonylureas. The clinician's aim will be to treat the child with the maximum dose that normalizes blood glucose levels (pre-prandial target: 70–120 mg/dL—post-prandial target: 100–145 mg/dL) without causing hypoglycemia, in order to optimize the drug's effect on the central nervous system. Sulphonylureas are currently only available as a 5 mg tablet and are not licensed for indications in neonatal diabetes. However, glibenclamide has recently obtained the orphan-drug indication from the European Medicine Agency (EMA) in neonatal diabetes. Unlicensed administration is currently achieved by parents through crushing and extemporaneous dilution of the tablets. However, the crushed tablets are poorly soluble in water, which may lead to variations in the dosage actually received by the child. To resolve this problem, a sulphonylurea suspension called Amglidia R has demonstratable efficacy in this indication ( 43 ) and has recently obtained a European Marketing Authorization; it has been available in France under a temporary authorization for use (ATU: Autorisation Temporaire d'Utilization) since 2019. It will enable dosages to be adapted more accurately.
An Appendix added to this text describes succinctly the practical aspects of the switch from insulin injection to the glibenclamide suspension licensed in European Union for children and refers to the official summary of product characteristics for detailed information.
Importance of the Genetic Diagnosis
Genetic analyses enables the diagnosis of monogenic diabetes in nearly 83% of diabetes diagnosed before the age of 6 months ( 30 ). This genetic diagnosis is essential as it will both influence the therapeutic treatment and make it possible to predict potential diabetes-related complications or illnesses. Genetic analyses must be carried out when diagnosing diabetes mellitus in all of the following children: age <6 months when diabetes mellitus is detected, or between 6 months and 1 year if extra-pancreatic features and/or no evidence of pancreas autoimmunity and/or multiple autoimmune disorders or unusual family history or associated congenital defects ( Figure 2 ) ( 44 ). Testing should not be delayed until other symptoms of the disease appear or potential remission of the disease. It is also of utmost importance to identify if the sulfonylureas can be introduced successfully as high-dose sulfonylurea therapy has been shown to be an appropriate treatment for patients with KCNJ11 permanent neonatal diabetes from diagnosis. This therapy has been shown to be safe and highly effective, maintaining excellent glycemic control for at least 10 years ( 45 ).
Neonatal diabetes is a model of rare human genetic disease, important in the understanding of the development and function of the pancreatic β cell, and in helping to resolve the pathophysiology of more frequent adult diabetes, such as type 2 diabetes. Neonatal diabetes is often associated with specific neuropsychological or developmental disorders of underlying genetic causes. A multidisciplinary approach is therefore essential. All clinicians called upon to treat a patient with neonatal diabetes should look for these clinical signs. Knowing the natural history and complete phenotype of this disease makes it possible, firstly, to offer patients better treatment and, secondly, to broaden the scope of genetic analyses to genes involved in the development and function of other organs. Long-term follow-up should be implemented, including for the so-called “transient” forms of neonatal diabetes.
Author Contributions
All authors listed have made a substantial, direct and intellectual contribution to the work, and approved it for publication.
Conflict of Interest
MP is a scientific advisor for AMMTEK.
The remaining authors declare that the research was conducted in the absence of any commercial or financial relationships that could be construed as a potential conflict of interest.
Acknowledgments
We were grateful to Prof. Paul Czernichow, Paris, France, for his active participation in our neonatal diabetes project. We thank Mme. Nathalie Pouvreau at the Robert Debré Hospital, Paris, for the biological diagnosis and the support of the bank. We also thank all clinicians in France and abroad, as well as the children and their families, who trust us in this field.
Supplementary Material
The Supplementary Material for this article can be found online at: https://www.frontiersin.org/articles/10.3389/fped.2020.540718/full#supplementary-material
1. Iafusco D, Massa O, Pasquino B, Colombo C, Iughetti L, Bizzarri C, et al. Minimal incidence of neonatal/infancy onset diabetes in Italy is 1:90,000 live births. Acta Diabetol. (2012) 49:405–8. doi: 10.1007/s00592-011-0331-8
PubMed Abstract | CrossRef Full Text | Google Scholar
2. Kanakatti Shankar R, Pihoker C, Dolan LM, Standiford D, Badaru A, Dabelea D, et al. Permanent neonatal diabetes mellitus: prevalence and genetic diagnosis in the SEARCH for diabetes in Youth Study. Pediatr Diabetes. (2013) 14:174–80. doi: 10.1111/pedi.12003
3. Polak M, Cave H. Neonatal diabetes mellitus: a disease linked to multiple mechanisms. Orphanet J Rare Dis. (2007) 2:12. doi: 10.1186/1750-1172-2-12
4. Busiah K, Drunat S, Vaivre-Douret L, Bonnefond A, Simon A, Flechtner I, et al. Neuropsychological dysfunction and neurodevelopmental defects associated with genetic changes in infants with neonatal diabetes mellitus: a prospective cohort study. Lancet Diabetes Endocrinol. (2013) 1:199–207. doi: 10.1016/S2213-8587(13)70059-7
CrossRef Full Text | Google Scholar
5. Huopio H, Miettinen PJ, Ilonen J, Nykänen P, Veijola R, Keskinen P, et al. Clinical, genetic, and biochemical characteristics of early-onset diabetes in the Finnish population. J Clin Endocrinol Metab. (2016) 101:3018–26. doi: 10.1210/jc.2015-4296
6. Temple IK, Gardner RJ, Robinson DO, Kibirige MS, Ferguson AW, Baum JD, et al. Further evidence for an imprinted gene for neonatal diabetes localised to chromosome 6q22-q23. Hum Mol Genet. (1996) 5:1117–21. doi: 10.1093/hmg/5.8.1117
7. Cave H, Polak M, Drunat S, Denamur E, Czernichow P. Refinement of the 6q chromosomal region implicated in transient neonatal diabetes. Diabetes. (2000) 49:108–13. doi: 10.2337/diabetes.49.1.108
8. Gardner RJ, Mackay DJ, Mungall AJ, Polychronakos C, Siebert R, Shield JP, et al. An imprinted locus associated with transient neonatal diabetes mellitus. Hum Mol Genet. (2000) 9:589–96. doi: 10.1093/hmg/9.4.589
9. Arima T, Drewell RA, Arney KL, Inoue J, Makita Y, Hata A, et al. A conserved imprinting control region at the HYMAI/ZAC domain is implicated in transient neonatal diabetes mellitus. Hum Mol Genet. (2001) 10:1475–83. doi: 10.1093/hmg/10.14.1475
10. Ma D, Shield JP, Dean W, Leclerc I, Knauf C, Burcelin RR, et al. Impaired glucose homeostasis in transgenic mice expressing the human transient neonatal diabetes mellitus locus, TNDM. J Clin Invest. (2004) 114:339–48. doi: 10.1172/JCI200419876
11. Docherty LE, Kabwama S, Lehmann A, Hawke E, Harrison L, Flanagan SE, et al. Clinical presentation of 6q24 transient neonatal diabetes mellitus (6q24 TNDM) and genotype-phenotype correlation in an international cohort of patients. Diabetologia. (2013) 56:758–62. doi: 10.1007/s00125-013-2832-1
12. Mackay DJ, Callaway JL, Marks SM, White HE, Acerini CL, Boonen SE, et al. Hypomethylation of multiple imprinted loci in individuals with transient neonatal diabetes is associated with mutations in ZFP57. Nat Genet. (2008) 40:949–51. doi: 10.1038/ng.187
13. Clement JP, Kunjilwar K, Gonzalez G, Schwanstecher M, Panten U, Aguilar-Bryan L, et al. Association and stoichiometry of KATP channel subunits. Neuron. (1997) 18:827–38. doi: 10.1016/S0896-6273(00)80321-9
14. Ashcroft FM, Gribble FM. Correlating structure and function in ATP-sensitive K + channels. Trends Neurosci. (1998) 21:288–94. doi: 10.1016/S0166-2236(98)01225-9
15. Gloyn AL, Pearson ER, Antcliff JF, Proks P, Bruining GJ, Slingerland AS, et al. Activating mutations in the gene encoding the ATP-sensitive potassium-channel subunit Kir6.2 and permanent neonatal diabetes. N Engl J Med. (2004) 350:1838–49. doi: 10.1056/NEJMoa032922
16. Vaxillaire M, Populaire C, Busiah K, Cave H, Gloyn AL, Hattersley AT, et al. Kir6.2 mutations are a common cause of permanent neonatal diabetes in a large cohort of French patients. Diabetes. (2004) 53:2719–22. doi: 10.2337/diabetes.53.10.2719
17. Babenko AP, Polak M, Cave H, Busiah K, Czernichow P, Scharfmann R, et al. Activating mutations in the ABCC8 gene in neonatal diabetes mellitus. N Engl J Med. (2006) 355:456–66. doi: 10.1056/NEJMoa055068
18. Stoy J, Edghill EL, Flanagan SE, Ye H, Paz VP, Pluzhnikov A, et al. Insulin gene mutations as a cause of permanent neonatal diabetes. Proc Natl Acad Sci USA. (2007) 104:15040–4. doi: 10.1073/pnas.0707291104
19. Polak M, Dechaume A, Cave H, Nimri R, Crosnier H, Sulmont V, et al. Heterozygous missense mutations in the insulin gene are linked to permanent diabetes appearing in the neonatal period or in early-infancy: a report from the French ND study group. Diabetes. (2008) 57:1115–9. doi: 10.2337/db07-1358
20. Izumi T, Yokota-Hashimoto H, Zhao S, Wang J, Halban PA, Takeuchi T. Dominant negative pathogenesis by mutant proinsulin in the Akita diabetic mouse. Diabetes. (2003) 52:409–16. doi: 10.2337/diabetes.52.2.409
21. Colombo C, Porzio O, Liu M, Massa O, Vasta M, Salardi S, et al. Seven mutations in the human insulin gene linked to permanent neonatal/infancy-onset diabetes mellitus. J Clin Invest. (2008) 118:2148–56. doi: 10.1172/JCI33777
22. Meur G, Simon A, Harun N, Virally M, Dechaume A, Bonnefond A, et al. Insulin gene mutations resulting in early-onset diabetes: marked differences in clinical presentation, metabolic status, and pathogenic effect through endoplasmic reticulum retention. Diabetes. (2010) 59:653–61. doi: 10.2337/db09-1091
23. Balboa D, Saarimäki-Vire J, Borshagovski D, Survila M, Lindholm P, Galli E, et al. Insulin mutations impair beta-cell development in a patient-derived iPSC model of neonatal diabetes. Elife. (2018) 7:e38519. doi: 10.7554/eLife.38519
24. Garin I, Edghill EL, Akerman I, Rubio-Cabezas O, Rica I, Locke JM, et al. Recessive mutations in the INS gene result in neonatal diabetes through reduced insulin biosynthesis. Proc Natl Acad Sci USA. (2010) 107:3105–10. doi: 10.1073/pnas.0910533107
25. Bonnefond A, Lomberk G, Buttar N, Busiah K, Vaillant E, Lobbens S, et al. Disruption of a novel Kruppel-like transcription factor p300-regulated pathway for insulin biosynthesis revealed by studies of the c.-331 INS mutation found in neonatal diabetes mellitus. J Biol Chem. (2011) 286:28414–24. doi: 10.1074/jbc.M110.215822
26. Stoffel M, Froguel P, Takeda J, Zouali H, Vionnet N, Nishi S, et al. Human glucokinase gene: isolation, characterization, and identification of two missense mutations linked to early-onset non-insulin-dependent (type 2) diabetes mellitus. Proc Natl Acad Sci USA. (1992) 89:7698. doi: 10.1073/pnas.89.16.7698
27. Njolstad PR, Sovik O, Cuesta-Munoz A, Bjorkhaug L, Massa O, Barbetti F, et al. Neonatal diabetes mellitus due to complete glucokinase deficiency. N Engl J Med. (2001) 344:1588–92. doi: 10.1056/NEJM200105243442104
28. Gloyn AL, Ellard S, Shield JP, Temple IK, Mackay DJ, Polak M, et al. Complete glucokinase deficiency is not a common cause of permanent neonatal diabetes. Diabetologia. (2002) 45:290. doi: 10.1007/s00125-001-0746-9
29. Vaxillaire M, Samson C, Cave H, Metz C, Froguel P, Polak M. Glucokinase gene mutations are not a common cause of permanent neonatal diabetes in France. Diabetologia. (2002) 45:454–5. doi: 10.1007/s00125-001-0741-1
30. Johnson MB, De Franco E, Greeley SAW, Letourneau LR, Gillespie KM; International DS-PNDM Consortium, Wakeling MN, et al. Trisomy 21 is a cause of permanent neonatal diabetes that is autoimmune but not HLA associated. Diabetes. (2019) 68:1528–35. doi: 10.2337/db19-0045
31. Gloyn AL, Reimann F, Girard C, Edghill EL, Proks P, Pearson ER, et al. Relapsing diabetes can result from moderately activating mutations in KCNJ11. Hum Mol Genet. (2005) 14:925–34. doi: 10.1093/hmg/ddi086
32. Amiel SA, Caprio S, Sherwin RS, Plewe G, Haymond MW, Tamborlane WV. Insulin resistance of puberty: a defect restricted to peripheral glucose metabolism. J Clin Endocrinol Metab. (1991) 72:277–82. doi: 10.1210/jcem-72-2-277
33. Slingerland AS, Hurkx W, Noordam K, Flanagan SE, Jukema JW, Meiners LC, et al. Sulphonylurea therapy improves cognition in a patient with the V59M KCNJ11 mutation. Diabet Med. (2008) 25:277–81. doi: 10.1111/j.1464-5491.2007.02373.x
34. Le Bourgeois F, Beltrand J, Baz B, Julla JB, Riveline JP, Simon A, et al. TNDM long-term follow-up study group long-term metabolic and socioeducational outcomes of transient neonatal diabetes: a longitudinal and cross-sectional study. Diabetes Care . (2020) 43:1191–9. doi: 10.2337/dc19-0324
35. Rabbone I, Barbetti F, Marigliano M, Bonfanti R, Piccinno E, Ortolani F, et al. Successful treatment of young infants presenting neonatal diabetes mellitus with continuous subcutaneous insulin infusion before genetic diagnosis. Acta Diabetol. (2016) 53:559–65. doi: 10.1007/s00592-015-0828-7
36. Tubiana-Rufi N. Insulin pump thapy in noenatal diabetes. Endocr Dev. (2007) 12:67–74. doi: 10.1159/000109606
37. Pearson ER, Flechtner I, Njolstad PR, Malecki MT, Flanagan SE, Larkin B, et al. Switching from insulin to oral sulfonylureas in patients with diabetes due to Kir6.2 mutations. N Engl J Med. (2006) 355:467–77. doi: 10.1056/NEJMoa061759
38. Garcin L, Mericq V, Fauret-Amsellem AL, Cave H, Polak M, Beltrand J. Neonatal diabetes due to potassium channel mutation: response to sulfonylurea according to the genotype. Pediatr Diabetes . (2020) 21:932–41. doi: 10.1111/pedi.13041
39. Carmody D, Bell CD, Hwang JL, Dickens JT, Sima DI, Felipe DL, et al. Sulfonylurea treatment before genetic testing in neonatal diabetes: pros and cons. J Clin Endocrinol Metab. (2014) 99:2709–14. doi: 10.1210/jc.2014-2494
40. Slingerland AS, Nuboer R, Hadders-Algra M, Hattersley AT, Bruining GJ. Improved motor development and good long-term glycaemic control with sulfonylurea treatment in a patient with the syndrome of intermediate developmental delay, early-onset generalised epilepsy and neonatal diabetes associated with the V59M mutation in the KCNJ11 gene. Diabetologia. (2006) 49:2559–63. doi: 10.1007/s00125-006-0407-0
41. Shah RP, Spruyt K, Kragie BC, Greeley SAW, Msall ME. Visuomotor performance in KCNJ11-related neonatal diabetes is impaired in children with DEND-associated mutations and may be improved by early treatment with sulfonylureas. Diabetes Care. (2012) 35:2086–8. doi: 10.2337/dc11-2225
42. Garcin L, Kariyawasam D, Busiah K, Fauret-Amsellem A-L, Le Bourgeois F, Vaivre-Douret L, et al. Successful off-label sulfonylurea treatment of neonatal diabetes mellitus due to chromosome 6 abnormalities. Pediatr Diabetes. (2018) 19:663–9. doi: 10.1111/pedi.12635
43. Beltrand J, Baptiste A, Busiah K, Bouazza N, Godot C, Boucheron A, et al. Glibenclamide oral suspension: suitable and effective in patients with neonatal diabetes. Pediatr Diabetes. (2019) 20:246–54. doi: 10.1111/pedi.12823
44. Hattersley AT, Greeley SAW, Polak M, Rubio-Cabezas O, Njølstad PR, Mlynarski W, et al. ISPAD clinical practice consensus guidelines 2018: the diagnosis and management of monogenic diabetes in children and adolescents. Pediatr Diabetes. (2018) 19:47–63. doi: 10.1111/pedi.12772
45. Bowman P, Sulen Å, Barbetti F, Beltrand J, Svalastoga P, Codner E, et al. Effectiveness and safety of long-term treatment with sulfonylureas in patients with neonatal diabetes due to KCNJ11 mutations: an international cohort study. Lancet Diabetes Endocrinol . (2018) 6:637–46. doi: 10.1016/S2213-8587(18)30106-2
Keywords: neonatal diabetes mellitus, chromosome 6q24 abnormality, associated malformations, neuropsychological disorder, KCNJ11 (Kir6.2), ABCC8, sulfonylurea receptor (SUR1)
Citation: Beltrand J, Busiah K, Vaivre-Douret L, Fauret AL, Berdugo M, Cavé H and Polak M (2020) Neonatal Diabetes Mellitus. Front. Pediatr. 8:540718. doi: 10.3389/fped.2020.540718
Received: 05 March 2020; Accepted: 13 August 2020; Published: 30 September 2020.
Reviewed by:
Copyright © 2020 Beltrand, Busiah, Vaivre-Douret, Fauret, Berdugo, Cavé and Polak. This is an open-access article distributed under the terms of the Creative Commons Attribution License (CC BY) . The use, distribution or reproduction in other forums is permitted, provided the original author(s) and the copyright owner(s) are credited and that the original publication in this journal is cited, in accordance with accepted academic practice. No use, distribution or reproduction is permitted which does not comply with these terms.
*Correspondence: Michel Polak, michel.polak@aphp.fr
† These authors share first authorship
Disclaimer: All claims expressed in this article are solely those of the authors and do not necessarily represent those of their affiliated organizations, or those of the publisher, the editors and the reviewers. Any product that may be evaluated in this article or claim that may be made by its manufacturer is not guaranteed or endorsed by the publisher.

An official website of the United States government
The .gov means it’s official. Federal government websites often end in .gov or .mil. Before sharing sensitive information, make sure you’re on a federal government site.
The site is secure. The https:// ensures that you are connecting to the official website and that any information you provide is encrypted and transmitted securely.
- Publications
- Account settings
- My Bibliography
- Collections
- Citation manager
Save citation to file
Email citation, add to collections.
- Create a new collection
- Add to an existing collection
Add to My Bibliography
Your saved search, create a file for external citation management software, your rss feed.
- Search in PubMed
- Search in NLM Catalog
- Add to Search
Neonatal Diabetes Mellitus: An Update on Diagnosis and Management
Affiliations.
- 1 Section of Adult and Pediatric Endocrinology, Diabetes, and Metabolism, MC 5053, 5841 South Maryland Avenue, Chicago, IL 60637, USA.
- 2 Monogenic Diabetes Registry, University of Chicago Medicine, Kovler Diabetes Center, 900 East 57th Street, Chicago, IL 60637, USA.
- 3 Section of Adult and Pediatric Endocrinology, Diabetes, and Metabolism, Kovler Diabetes Center, The University of Chicago, 900 East 57th Street, Chicago, IL 60637, USA. Electronic address: [email protected].
- PMID: 29406006
- PMCID: PMC5928785
- DOI: 10.1016/j.clp.2017.10.006
Neonatal diabetes mellitus is likely to be due to an underlying monogenic defect when it occurs at less than 6 months of age. Early recognition and urgent genetic testing are important for predicting the clinical course and raising awareness of possible additional features. Early treatment of sulfonylurea-responsive types of neonatal diabetes may improve neurologic outcomes. It is important to distinguish neonatal diabetes mellitus from other causes of hyperglycemia in newborns. Other causes include infection, stress, inadequate pancreatic insulin production in preterm infants, among others. This review explores the diagnostic approach, mutation types, management, and clinical course of neonatal diabetes.
Keywords: Genetic; Glyburide; Insulin; Monogenic diabetes; Neonatal diabetes.
Copyright © 2017 Elsevier Inc. All rights reserved.
PubMed Disclaimer
Mechanisms of overexpression of imprinted…
Mechanisms of overexpression of imprinted genes causing 6q24-related neonatal diabetes. Diabetes in all…
Algorithm for considering sulfonylurea trial.
Similar articles
- Personalized precision medicine in extreme preterm infants with transient neonatal diabetes mellitus. Kylat RI, Senguttuvan R, Bader MY. Kylat RI, et al. J Pediatr Endocrinol Metab. 2017 May 1;30(5):593-596. doi: 10.1515/jpem-2016-0261. J Pediatr Endocrinol Metab. 2017. PMID: 28350539
- [Neonatal diabetes mellitus]. Huopio H, Otonkoski T. Huopio H, et al. Duodecim. 2011;127(6):534-41. Duodecim. 2011. PMID: 21528518 Review. Finnish.
- Remission of severe neonatal diabetes with very early sulfonylurea treatment. Marshall BA, Green RP, Wambach J, White NH, Remedi MS, Nichols CG. Marshall BA, et al. Diabetes Care. 2015 Mar;38(3):e38-9. doi: 10.2337/dc14-2124. Diabetes Care. 2015. PMID: 25715421 Free PMC article. No abstract available.
- [Neonatal diabetes mellitus: treatment with sulfonylurea in a preterm born infant]. Wendelin G, Haim M, Reiterer F, Borkenstein M, Müller W. Wendelin G, et al. Klin Padiatr. 2009 Mar-Apr;221(2):100. doi: 10.1055/s-0029-1202867. Epub 2009 Mar 4. Klin Padiatr. 2009. PMID: 19263329 German. No abstract available.
- Neonatal diabetes mellitus: a disease linked to multiple mechanisms. Polak M, Cavé H. Polak M, et al. Orphanet J Rare Dis. 2007 Mar 9;2:12. doi: 10.1186/1750-1172-2-12. Orphanet J Rare Dis. 2007. PMID: 17349054 Free PMC article. Review.
- Transient diabetes mellitus with ABCC8 variant successfully treated with sulfonylurea: Two case reports and review of literature. Shen LH, Cui Y, Fu DX, Yang W, Wu SN, Wang HZ, Yang HH, Chen YX, Wei HY. Shen LH, et al. World J Diabetes. 2024 Aug 15;15(8):1811-1819. doi: 10.4239/wjd.v15.i8.1811. World J Diabetes. 2024. PMID: 39192869 Free PMC article.
- The quality of reporting in case reports of permanent neonatal diabetes mellitus: a cross-sectional study. Jia P, Wang L, Yang X, Pei W, Xu C, Feng J, Han Y. Jia P, et al. BMC Med Res Methodol. 2024 May 20;24(1):117. doi: 10.1186/s12874-024-02226-1. BMC Med Res Methodol. 2024. PMID: 38769533 Free PMC article.
- Atypical Diabetes: What Have We Learned and What Does the Future Hold? Stone SI, Balasubramanyam A, Posey JE. Stone SI, et al. Diabetes Care. 2024 May 1;47(5):770-781. doi: 10.2337/dci23-0038. Diabetes Care. 2024. PMID: 38329838 Free PMC article. Review.
- Not All Diabetic Ketoacidosis in Infant Is Type 1: A Case Report Permanent Neonatal Diabetes. Al Homyani DK, Al Homaiani L. Al Homyani DK, et al. AACE Clin Case Rep. 2023 Oct 17;10(1):7-9. doi: 10.1016/j.aace.2023.10.004. eCollection 2024 Jan-Feb. AACE Clin Case Rep. 2023. PMID: 38303767 Free PMC article.
- Genetic perspectives on childhood monogenic diabetes: Diagnosis, management, and future directions. Sun HY, Lin XY. Sun HY, et al. World J Diabetes. 2023 Dec 15;14(12):1738-1753. doi: 10.4239/wjd.v14.i12.1738. World J Diabetes. 2023. PMID: 38222792 Free PMC article. Review.
- Letourneau L, Carmody D, Wroblewski K, Denson A, Sanyoura M, Naylor RN, Philipson LH, Greeley SAW. Diabetes presentation and management in infancy: high risk of diabetic ketoacidosis (DKA) Press; - PMC - PubMed
- Franco De, Flanagan SE, Houghton JA, Allen HL, Mackay DJ, Temple IK, Ellard S, Hattersley AT. The effect of early, comprehensive genomic testing on clinical care in neonatal diabetes: an international cohort study. [cited 2017 Jul 16];The Lancet. 2015 386:957–63. Internet. Available from: http://linkinghub.elsevier.com/retrieve/pii/S0140673615600988 . - PMC - PubMed
- Shah RP, Spruyt K, Kragie BC, Greeley SAW, Msall ME. Visuomotor Performance in KCNJ11-Related Neonatal Diabetes Is Impaired in Children With DEND-Associated Mutations and May Be Improved by Early Treatment With Sulfonylureas. [cited 2017 Jul 16];Diabetes Care. 2012 35:2086–8. Internet. Available from: http://care.diabetesjournals.org/cgi/doi/10.2337/dc11-2225 . - DOI - PMC - PubMed
- Hattersley A, Bruining J, Shield J, Njolstad P, Donaghue K. ISPAD Clinical Practice Consensus Guidelines 2006-2007 The diagnosis and management of monogenic diabetes in children. [cited 2017 Jul 16];Pediatr Diabetes. 2006 7:352–60. Internet. Available from: http://doi.wiley.com/10.1111/j.1399-5448.2006.00217.x . - DOI - PubMed
- Besser REJ, Flanagan SE, Mackay DGJ, Temple IK, Shepherd MH, Shields BM, Ellard S, Hattersley AT. Prematurity and Genetic Testing for Neonatal Diabetes. [cited 2017 Jul 16];PEDIATRICS. 2016 138:e20153926–e20153926. Internet. Available from: http://pediatrics.aappublications.org/cgi/doi/10.1542/peds.2015-3926 . - DOI - PMC - PubMed
Publication types
- Search in MeSH
Related information
- Cited in Books
- PubChem Compound (MeSH Keyword)
Grants and funding
- K23 DK094866/DK/NIDDK NIH HHS/United States
- P30 DK020595/DK/NIDDK NIH HHS/United States
- R01 DK104942/DK/NIDDK NIH HHS/United States
- T32 DK064582/DK/NIDDK NIH HHS/United States
LinkOut - more resources
Full text sources.
- Elsevier Science
- Europe PubMed Central
- PubMed Central
- W.B. Saunders
Other Literature Sources
- scite Smart Citations
- MedlinePlus Health Information
- Citation Manager
NCBI Literature Resources
MeSH PMC Bookshelf Disclaimer
The PubMed wordmark and PubMed logo are registered trademarks of the U.S. Department of Health and Human Services (HHS). Unauthorized use of these marks is strictly prohibited.
Thank you for visiting nature.com. You are using a browser version with limited support for CSS. To obtain the best experience, we recommend you use a more up to date browser (or turn off compatibility mode in Internet Explorer). In the meantime, to ensure continued support, we are displaying the site without styles and JavaScript.
- View all journals
- Explore content
- About the journal
- Publish with us
- Sign up for alerts
- Review Article
- Published: 29 November 2011
Management of diabetes mellitus in infants
- Beate Karges 1 ,
- Thomas Meissner 2 ,
- Andrea Icks 3 ,
- Thomas Kapellen 4 &
- Reinhard W. Holl 5
Nature Reviews Endocrinology volume 8 , pages 201–211 ( 2012 ) Cite this article
1907 Accesses
43 Citations
Metrics details
- Paediatrics
- Therapeutics
- Type 1 diabetes
Diabetes mellitus diagnosed during the first 2 years of life differs from the disease in older children regarding its causes, clinical characteristics, treatment options and needs in terms of education and psychosocial support. Over the past decade, new genetic causes of neonatal diabetes mellitus have been elucidated, including monogenic β-cell defects and chromosome 6q24 abnormalities. In patients with KCNJ11 or ABCC8 mutations and diabetes mellitus, oral sulfonylurea offers an easy and effective treatment option. Type 1 diabetes mellitus in infants is characterized by a more rapid disease onset, poorer residual β-cell function and lower rate of partial remission than in older children. Insulin therapy in infants with type 1 diabetes mellitus or other monogenic causes of diabetes mellitus is a challenge, and novel data highlight the value of continuous subcutaneous insulin infusion in this very young patient population. Infants are entirely dependent on caregivers for insulin therapy, nutrition and glucose monitoring, which emphasizes the need for appropriate education and psychosocial support of parents. To achieve optimal long-term metabolic control with low rates of acute and chronic complications, continuous and structured diabetes care should be provided by a multidisciplinary health-care team.
Diabetes mellitus in infants and children differs in etiology, clinical presentation and therapeutic options; heterogeneous etiologies of diabetes mellitus in infancy include genetic abnormalities, developmental defects and autoimmune disease
Monogenic forms of neonatal diabetes mellitus almost always occur in the first 6 months of life and very rarely after 12 months; onset of diabetes mellitus in infants aged >6 months is mostly due to type 1 diabetes mellitus (T1DM)
Infants with T1DM exhibit rapid disease onset, poor residual β-cell function and a low rate of transient recovery
Insulin is preferentially provided by continuous subcutaneous infusion
Treatment with sulfonylurea is possible in most patients with mutations in the genes that encode the ATP-sensitive inward rectifier potassium (K ATP ) channel
Special needs of infants with diabetes mellitus include comprehensive education of caregivers and provision of ongoing diabetes care
This is a preview of subscription content, access via your institution
Access options
Subscribe to this journal
Receive 12 print issues and online access
195,33 € per year
only 16,28 € per issue
Buy this article
- Purchase on SpringerLink
- Instant access to full article PDF
Prices may be subject to local taxes which are calculated during checkout

Similar content being viewed by others
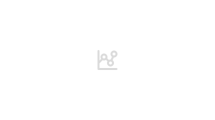
Type 1 diabetes mellitus management in young children: implementation of current technologies

Early exposures and inherent factors in premature newborns are associated with type 1 diabetes
Precision treatment of beta-cell monogenic diabetes: a systematic review.
Komulainen, J. et al . Clinical, autoimmune, and genetic characteristics of very young children with type 1 diabetes. Childhood Diabetes in Finland (DiMe) Study Group. Diabetes Care 22 , 1950–1955 (1999).
Article CAS PubMed Google Scholar
Nimri, R., Phillip, M. & Shalitin, S. Children diagnosed with diabetes during infancy have unique clinical characteristics. Horm. Res. 67 , 263–267 (2007).
CAS PubMed Google Scholar
Aguilar-Bryan, L. & Bryan, J. Neonatal diabetes mellitus. Endocr. Rev. 29 , 265–291 (2008).
Article CAS PubMed PubMed Central Google Scholar
Metz, C. et al . Neonatal diabetes mellitus: chromosomal analysis in transient and permanent cases. J. Pediatr. 141 , 483–489 (2002).
Article PubMed Google Scholar
Temple, I. K. et al . Transient neonatal diabetes: widening the understanding of the etiopathogenesis of diabetes. Diabetes 49 , 1359–1366 (2000).
Babenko, A. P. et al . Activating mutations in the ABCC8 gene in neonatal diabetes mellitus. N. Engl. J. Med. 355 , 456–466 (2006).
Flanagan, S. E. et al . Mutations in ATP-sensitive K + channel genes cause transient neonatal diabetes and permanent diabetes in childhood or adulthood. Diabetes 56 , 1930–1937 (2007).
Garin, I. et al . Recessive mutations in the INS gene result in neonatal diabetes through reduced insulin biosynthesis. Proc. Natl Acad. Sci. USA 107 , 3105–3110 (2010).
Article PubMed PubMed Central Google Scholar
Mackay, D. J. & Temple, I. K. Transient neonatal diabetes mellitus type 1. Am. J. Med. Genet. C Semin. Med. Genet. 154C , 335–342 (2010).
Mackay, D. J. et al . Hypomethylation of multiple imprinted loci in individuals with transient neonatal diabetes is associated with mutations in ZFP57. Nat. Genet. 40 , 949–951 (2008).
Søvik, O. et al . Familial occurrence of neonatal diabetes with duplications in chromosome 6q24: treatment with sulfonylurea and 40-yr follow-up. Pediatr. Diabetes 10.1111/j.1399-5448.2011.00776.x.
Edghill, E. L. et al . Insulin mutation screening in 1,044 patients with diabetes: mutations in the INS gene are a common cause of neonatal diabetes but a rare cause of diabetes diagnosed in childhood or adulthood. Diabetes 57 , 1034–1042 (2008).
Ellard, S. et al . Permanent neonatal diabetes caused by dominant, recessive, or compound heterozygous SUR1 mutations with opposite functional effects. Am. J. Hum. Genet. 81 , 375–382 (2007).
Gloyn, A. L. et al . Activating mutations in the gene encoding the ATP-sensitive potassium-channel subunit Kir6.2 and permanent neonatal diabetes. N. Engl. J. Med. 350 , 1838–1849 (2004).
Sagen, J. V. et al . Permanent neonatal diabetes due to mutations in KCNJ11 encoding Kir6.2: patient characteristics and initial response to sulfonylurea therapy. Diabetes 53 , 2713–2718 (2004).
de Wet, H. et al . Increased ATPase activity produced by mutations at arginine-1380 in nucleotide-binding domain 2 of ABCC8 causes neonatal diabetes. Proc. Natl Acad. Sci. USA 104 , 18988–18992 (2007).
Proks, P. et al . A heterozygous activating mutation in the sulphonylurea receptor SUR1 (ABCC8) causes neonatal diabetes. Hum. Mol. Genet. 15 , 1793–1800 (2006).
Thomas, P. M. et al . Mutations in the sulfonylurea receptor gene in familial persistent hyperinsulinemic hypoglycemia of infancy. Science 268 , 426–429 (1995).
Pearson, E. R. et al . Switching from insulin to oral sulfonylureas in patients with diabetes due to Kir6.2 mutations. N. Engl. J. Med. 355 , 467–477 (2006).
Rafiq, M. et al . Effective treatment with oral sulfonylureas in patients with diabetes due to sulfonylurea receptor 1 (SUR1) mutations. Diabetes Care 31 , 204–209 (2008).
Zwaveling-Soonawala, N. et al . Successful transfer to sulfonylurea therapy in an infant with developmental delay, epilepsy and neonatal diabetes (DEND) syndrome and a novel ABCC8 gene mutation. Diabetologia 54 , 469–471 (2011).
Slingerland, A. S. et al . Referral rates for diagnostic testing support an incidence of permanent neonatal diabetes in three European countries of at least 1 in 260,000 live births. Diabetologia 52 , 1683–1685 (2009).
Colombo, C. et al . Seven mutations in the human insulin gene linked to permanent neonatal/infancy-onset diabetes mellitus. J. Clin. Invest. 118 , 2148–2156 (2008).
CAS PubMed PubMed Central Google Scholar
Polak, M. et al . Heterozygous missense mutations in the insulin gene are linked to permanent diabetes appearing in the neonatal period or in early infancy: a report from the French ND (Neonatal Diabetes) Study Group. Diabetes 57 , 1115–1119 (2008).
Støy, J. et al . Insulin gene mutations as a cause of permanent neonatal diabetes. Proc. Natl Acad. Sci. USA 104 , 15040–15044 (2007).
Njølstad, P. R. et al . Neonatal diabetes mellitus due to complete glucokinase deficiency. N. Engl. J. Med. 344 , 1588–1592 (2001).
Russo, L. et al . Permanent diabetes during the first year of life: multiple gene screening in 54 patients. Diabetologia 54 , 1693–1701 (2011).
Rubio-Cabezas, O. et al . Wolcott–Rallison syndrome is the most common genetic cause of permanent neonatal diabetes in consanguineous families. J. Clin. Endocrinol. Metab. 94 , 4162–4170 (2009).
Senée, V. et al . Wolcott-Rallison syndrome: clinical, genetic, and functional study of EIF2AK3 mutations and suggestion of genetic heterogeneity. Diabetes 53 , 1876–1883 (2004).
Bennett, C. L. et al . The immune dysregulation, polyendocrinopathy, enteropathy, X-linked syndrome (IPEX) is caused by mutations of FOXP3. Nat. Genet. 27 , 20–21 (2001).
Rubio-Cabezas, O. et al . Clinical heterogeneity in patients with FOXP3 mutations presenting with permanent neonatal diabetes. Diabetes Care 32 , 111–116 (2009).
Rubio-Cabezas, O. et al . Permanent neonatal diabetes and enteric anendocrinosis associated with biallelic mutations in NEUROG3. Diabetes 60 , 1349–1353 (2011).
Rubio-Cabezas, O. et al . Homozygous mutations in NEUROD1 are responsible for a novel syndrome of permanent neonatal diabetes and neurological abnormalities. Diabetes 59 , 2326–2331 (2011).
Article Google Scholar
Wang, J. et al . Mutant neurogenin-3 in congenital malabsorptive diarrhea. N. Engl. J. Med. 355 , 270–280 (2006).
Dimitri, P. et al . Novel GLIS3 mutations demonstrate an extended multisystem phenotype. Eur. J. Endocrinol. 164 , 437–443 (2011).
Senée, V. et al . Mutations in GLIS3 are responsible for a rare syndrome with neonatal diabetes mellitus and congenital hypothyroidism. Nat. Genet. 38 , 682–687 (2006).
Solomon, B. D. et al . Compound heterozygosity for mutations in PAX6 in a patient with complex brain anomaly, neonatal diabetes mellitus, and microophthalmia. Am. J. Med. Genet. A 149A , 2543–2546 (2009).
Wen, J. H. et al . Paired box 6 (PAX6) regulates glucose metabolism via proinsulin processing mediated by prohormone convertase 1/3 (PC1/3). Diabetologia 52 , 504–513 (2009).
Bergmann, A. K. et al . Thiamine-responsive megaloblastic anemia: identification of novel compound heterozygotes and mutation update. J. Pediatr. 155 , 888–892 e1 (2009).
Labay, V. et al . Mutations in SLC19A2 cause thiamine-responsive megaloblastic anaemia associated with diabetes mellitus and deafness. Nat. Genet. 22 , 300–304 (1999).
Kentrup, H., Altmüller, J., Pfäffle, R. & Heimann, G. Neonatal diabetes mellitus with hypergalactosemia. Eur. J. Endocrinol. 141 , 379–381 (1999).
Santer, R. et al . Mutations in GLUT2, the gene for the liver-type glucose transporter, in patients with Fanconi–Bickel syndrome. Nat. Genet. 17 , 324–326 (1997).
Yoo, H. W., Shin, Y. L., Seo, E. J. & Kim, G. H. Identification of a novel mutation in the GLUT2 gene in a patient with Fanconi–Bickel syndrome presenting with neonatal diabetes mellitus and galactosaemia. Eur. J. Pediatr. 161 , 351–353 (2002).
Nicolino, M. et al . A novel hypomorphic PDX1 mutation responsible for permanent neonatal diabetes with subclinical exocrine deficiency. Diabetes 59 , 733–740 (2010).
Stoffers, D. A., Zinkin, N. T., Stanojevic, V., Clarke, W. L. & Habener, J. F. Pancreatic agenesis attributable to a single nucleotide deletion in the human IPF1 gene coding sequence. Nat. Genet. 15 , 106–110 (1997).
Sellick, G. S. et al . Mutations in PTF1A cause pancreatic and cerebellar agenesis. Nat. Genet. 36 , 1301–1305 (2004).
Smith, S. B. et al . Rfx6 directs islet formation and insulin production in mice and humans. Nature 463 , 775–780 (2010).
Beardsall, K., Pesterfield, C. L. & Acerini, C. L. Neonatal diabetes and insulin pump therapy. Arch. Dis. Child. Fetal Neonatal Ed. 96 , F223–F224 (2011).
Grulich-Henn, J. et al . Entities and frequency of neonatal diabetes: data from the diabetes documentation and quality management system (DPV). Diabet. Med. 27 , 709–712 (2010).
Vaziri-Sani, F. et al . ZnT8 autoantibody titers in type 1 diabetes patients decline rapidly after clinical onset. Autoimmunity 43 , 598–606 (2010).
Erlich, H. et al . HLA DR-DQ haplotypes and genotypes and type 1 diabetes risk: analysis of the type 1 diabetes genetics consortium families. Diabetes 57 , 1084–1092 (2008).
Barrett, J. C. et al . Genome-wide association study and meta-analysis find that over 40 loci affect risk of type 1 diabetes. Nat. Genet. 41 , 703–707 (2009).
Concannon, P. et al . Genome-wide scan for linkage to type 1 diabetes in 2,496 multiplex families from the Type 1 Diabetes Genetics Consortium. Diabetes 58 , 1018–1022 (2009).
Holmberg, H., Wahlberg, J., Vaarala, O. & Ludvigsson, J. Short duration of breast-feeding as a risk-factor for beta-cell autoantibodies in 5-year-old children from the general population. Br. J. Nutr. 97 , 111–116 (2007).
Norris, J. M. et al . Timing of initial cereal exposure in infancy and risk of islet autoimmunity. JAMA 290 , 1713–1720 (2003).
Virtanen, S. M. et al . Early introduction of root vegetables in infancy associated with advanced ss-cell autoimmunity in young children with human leukocyte antigen-conferred susceptibility to type 1 diabetes. Diabet. Med. 28 , 965–971 (2011).
Ziegler, A. G., Schmid, S., Huber, D., Hummel, M. & Bonifacio, E. Early infant feeding and risk of developing type 1 diabetes-associated autoantibodies. JAMA 290 , 1721–1728 (2003).
Yeung, W. C., Rawlinson, W. D. & Craig, M. E. Enterovirus infection and type 1 diabetes mellitus: systematic review and meta-analysis of observational molecular studies. BMJ 342 , d35 (2011).
Zipitis, C. S. & Akobeng, A. K. Vitamin D supplementation in early childhood and risk of type 1 diabetes: a systematic review and meta-analysis. Arch. Dis. Child. 93 , 512–517 (2008).
Harder, T. et al . Birth weight, early weight gain, and subsequent risk of type 1 diabetes: systematic review and meta-analysis. Am. J. Epidemiol. 169 , 1428–1436 (2009).
Vaarala, O., Atkinson, M. A. & Neu, J. The “perfect storm” for type 1 diabetes: the complex interplay between intestinal microbiota, gut permeability, and mucosal immunity. Diabetes 57 , 2555–2562 (2008).
Dahlquist, G. G., Nyström, L. & Patterson, C. C. Incidence of type 1 diabetes in Sweden among individuals aged 0–34 years, 1983–2007: an analysis of time trends. Diabetes Care 34 , 1754–1759 (2011).
Patterson, C. C., Dahlquist, G. G., Gyürüs, E., Green, A. & Soltész, G. Incidence trends for childhood type 1 diabetes in Europe during 1989–2003 and predicted new cases 2005–20: a multicentre prospective registration study. Lancet 373 , 2027–2033 (2009).
Rubio-Cabezas, O., Klupa, T. & Malecki, M. T. Permanent neonatal diabetes mellitus--the importance of diabetes differential diagnosis in neonates and infants. Eur. J. Clin. Invest. 41 , 323–333 (2011).
Berhan, Y., Waernbaum, I., Lind, T., Möllsten, A. & Dahlquist, G. Thirty years of prospective nationwide incidence of childhood type 1 diabetes: the accelerating increase by time tends to level off in Sweden. Diabetes 60 , 577–581 (2011).
Altamirano-Bustamante, N. et al . Economic family burden of metabolic control in children and adolescents with type 1 diabetes mellitus. J. Pediatr. Endocrinol. Metab. 21 , 1163–1168 (2008).
Ying, A. K. et al . Predictors of direct costs of diabetes care in pediatric patients with type 1 diabetes. Pediatr. Diabetes 12 , 177–182 (2011).
Icks, A. et al . Direct costs of pediatric diabetes care in Germany and their predictors. Exp. Clin. Endocrinol. Diabetes 112 , 302–309 (2004).
Wiréhn, A. B., Andersson, A., Ostgren, C. J. & Carstensen, J. Age-specific direct healthcare costs attributable to diabetes in a Swedish population: a register-based analysis. Diabet. Med. 25 , 732–737 (2008).
Bächle, C. et al . Direct diabetes-related costs in young patients with early onset and long-lasting type 1 diabetes [a864]. Diabetologia 54 (Suppl. 1), S353 (2011).
Google Scholar
Greeley, S. A. et al . The cost-effectiveness of personalized genetic medicine: the case of genetic testing in neonatal diabetes. Diabetes Care 34 , 622–627 (2011).
Hekkala, A., Reunanen, A., Koski, M., Knip, M. & Veijola, R. Age-related differences in the frequency of ketoacidosis at diagnosis of type 1 diabetes in children and adolescents. Diabetes Care 33 , 1500–1502 (2010).
Dahlquist, G. & Källén, B. Mortality in childhood-onset type 1 diabetes: a population-based study. Diabetes Care 28 , 2384–2387 (2005).
Temple, I. K. & Shield, J. P. 6q24 transient neonatal diabetes. Rev. Endocr. Metab. Disord. 11 , 199–204 (2010).
American Diabetes Association. Diagnosis and classification of diabetes mellitus. Diabetes Care 33 (Suppl. 1), S62–S69 (2010).
Edghill, E. L., Flanagan, S. E. & Ellard, S. Permanent neonatal diabetes due to activating mutations in ABCC8 and KCNJ11. Rev. Endocr. Metab. Disord. 11 , 193–198 (2010).
Bonnefond, A. et al . Molecular diagnosis of neonatal diabetes mellitus using next-generation sequencing of the whole exome. Plos ONE 5 , e13630 (2010).
Wambach, J. A., Marshall, B. A., Koster, J. C., White, N. H. & Nichols, C. G. Successful sulfonylurea treatment of an insulin-naive neonate with diabetes mellitus due to a KCNJ11 mutation. Pediatr. Diabetes 11 , 286–288 (2010).
Danne, T. et al . A comparison of postprandial and preprandial administration of insulin aspart in children and adolescents with type 1 diabetes. Diabetes Care 26 , 2359–2364 (2003).
Danne, T. et al . Parental preference of prandial insulin aspart compared with preprandial human insulin in a basal-bolus scheme with NPH insulin in a 12-wk crossover study of preschool children with type 1 diabetes. Pediatr. Diabetes 8 , 278–285 (2007).
Bachran, R. et al . Basal rates and circadian profiles in continuous subcutaneous insulin infusion (CSII) differ for preschool children, prepubertal children, adolescents and young adults. Pediatr. Diabetes 10.1111/j.1399-5448.2011.00777.x.
Szypowska, A., Lipka, M., Błazik, M., Groele, L. & Pańkowska, E. Insulin requirement in preschoolers treated with insulin pumps at the onset of type 1 diabetes mellitus. Acta Paediatr. 98 , 527–530 (2009).
Alemzadeh, R., Berhe, T. & Wyatt, D. T. Flexible insulin therapy with glargine insulin improved glycemic control and reduced severe hypoglycemia among preschool-aged children with type 1 diabetes mellitus. Pediatrics 115 , 1320–1324 (2005).
Tubiana-Rufi, N. Insulin pump therapy in neonatal diabetes. Endocr. Dev. 12 , 67–74 (2007).
Kapellen, T. M. et al . Changes in the use of analogue insulins in 37,206 children and adolescents with type 1 diabetes in 275 German and Austrian centers during the last twelve years. Exp. Clin. Endocrinol. Diabetes 117 , 329–335 (2009).
Fox, L. A., Buckloh, L. M., Smith, S. D., Wysocki, T. & Mauras, N. A randomized controlled trial of insulin pump therapy in young children with type 1 diabetes. Diabetes Care 28 , 1277–1281 (2005).
Mack-Fogg, J. E., Orlowski, C. C. & Jospe, N. Continuous subcutaneous insulin infusion in toddlers and children with type 1 diabetes mellitus is safe and effective. Pediatr. Diabetes 6 , 17–21 (2005).
Pańkowska, E., Błazik, M., Dziechciarz, P., Szypowska, A. & Szajewska, H. Continuous subcutaneous insulin infusion vs. multiple daily injections in children with type 1 diabetes: a systematic review and meta-analysis of randomized control trials. Pediatr. Diabetes 10 , 52–58 (2009).
Berghaeuser, M. A. et al . Continuous subcutaneous insulin infusion in toddlers starting at diagnosis of type 1 diabetes mellitus. A multicenter analysis of 104 patients from 63 centres in Germany and Austria. Pediatr. Diabetes 9 , 590–595 (2008).
Wilson, D. M. et al . A two-center randomized controlled feasibility trial of insulin pump therapy in young children with diabetes. Diabetes Care 28 , 15–19 (2005).
Sulmont, V. et al . Metabolic control in children with diabetes mellitus who are younger than 6 years at diagnosis: continuous subcutaneous insulin infusion as a first line treatment? J. Pediatr. 157 , 103–107 (2010).
Müller, G. The molecular mechanism of the insulin-mimetic/sensitizing activity of the antidiabetic sulfonylurea drug Amaryl. Mol. Med. 6 , 907–933 (2000).
Hattersley, A. & Pearson, E. Transferring patients with diabetes due to Kir6.2 mutation from insulin to suphonylureas. Diabetes Genes [online] , (2011).
Klupa, T. et al . Efficacy and safety of sulfonylurea use in permanent neonatal diabetes due to KCNJ11 gene mutations: 34-month median follow-up. Diabetes Technol. Ther. 12 , 387–391 (2010).
Ziegler, R. et al . Frequency of SMBG correlates with HbA1c and acute complications in children and adolescents with type 1 diabetes. Pediatr. Diabetes 12 , 11–17 (2011).
Kordonouri, O. et al . Sensor-augmented pump therapy from the diagnosis of childhood type 1 diabetes: results of the Paediatric Onset Study (ONSET) after 12 months of treatment. Diabetologia 53 , 2487–2495 (2010).
Deiss, D., Kordonouri, O., Meyer, K. & Danne, T. Long hypoglycaemic periods detected by subcutaneous continuous glucose monitoring in toddlers and pre-school children with diabetes mellitus. Diabet. Med. 18 , 337–338 (2001).
Slover, R. H. et al . Effectiveness of sensor-augmented pump therapy in children and adolescents with type 1 diabetes in the STAR 3 study. Pediatr. Diabetes 10.1111/j.1399-5448.2011.00793.x.
Tamborlane, W. V. et al . Continuous glucose monitoring and intensive treatment of type 1 diabetes. N. Engl. J. Med. 359 , 1464–1476 (2008).
Hovorka, R. et al . Overnight closed loop insulin delivery (artificial pancreas) in adults with type 1 diabetes: crossover randomised controlled studies. BMJ 342 , d1855 (2011).
Elleri, D. et al . Automated overnight closed-loop glucose control in young children with type 1 diabetes. Diabetes Technol. Ther. 13 , 419–424 (2011).
Keymeulen, B. et al . Four-year metabolic outcome of a randomised controlled CD3-antibody trial in recent-onset type 1 diabetic patients depends on their age and baseline residual beta cell mass. Diabetologia 53 , 614–623 (2010).
Ludvigsson, J. et al . GAD treatment and insulin secretion in recent-onset type 1 diabetes. N. Engl. J. Med. 359 , 1909–1920 (2008).
Mastrandrea, L. et al . Etanercept treatment in children with new-onset type 1 diabetes: pilot randomized, placebo-controlled, double-blind study. Diabetes Care 32 , 1244–1249 (2009).
Wherrett, D. K. et al . Antigen-based therapy with glutamic acid decarboxylase (GAD) vaccine in patients with recent-onset type 1 diabetes: a randomised double-blind trial. Lancet 378 , 319–327 (2011).
Näntö-Salonen, K. et al . Nasal insulin to prevent type 1 diabetes in children with HLA genotypes and autoantibodies conferring increased risk of disease: a double-blind, randomised controlled trial. Lancet 372 , 1746–1755 (2008).
Knip, M. et al . Dietary intervention in infancy and later signs of beta-cell autoimmunity. N. Engl. J. Med. 363 , 1900–1908 (2010).
Couri, C. E. et al . C-peptide levels and insulin independence following autologous nonmyeloablative hematopoietic stem cell transplantation in newly diagnosed type 1 diabetes mellitus. JAMA 301 , 1573–1579 (2009).
Smart, C., Aslander-van Vliet, E. & Waldron, S. Nutritional management in children and adolescents with diabetes. Pediatr. Diabetes 10 (Suppl. 12), 100–117 (2009).
Sauer, C. W. & Kim, J. H. Human milk macronutrient analysis using point-of-care near-infrared spectrophotometry. J. Perinatol. 31 , 339–343 (2011).
Detlofson, I., Kroon, M. & Aman, J. Oral bedtime cornstarch supplementation reduces the risk for nocturnal hypoglycaemia in young children with type 1 diabetes. Acta Paediatr. 88 , 595–597 (1999).
Lange, K., Sassmann, H., von Schütz, W., Kordonouri, O. & Danne, T. Prerequisites for age-appropriate education in type 1 diabetes: a model programme for paediatric diabetes education in Germany. Pediatr. Diabetes 8 (Suppl. 6), 63–71 (2007).
Swift, P. G. Diabetes education in children and adolescents. Pediatr. Diabetes 10 (Suppl. 12), 51–57 (2009).
McCrimmon, R. J., Gold, A. E., Deary, I. J., Kelnar, C. J. & Frier, B. M. Symptoms of hypoglycemia in children with IDDM. Diabetes Care 18 , 858–861 (1995).
Hershey, T. et al . Frequency and timing of severe hypoglycemia affects spatial memory in children with type 1 diabetes. Diabetes Care 28 , 2372–2377 (2005).
Wagner, V. M., Grabert, M. & Holl, R. W. Severe hypoglycaemia, metabolic control and diabetes management in children with type 1 diabetes in the decade after the Diabetes Control and Complications Trial—a large-scale multicentre study. Eur. J. Pediatr. 164 , 73–79 (2005).
Schoenle, E. J., Schoenle, D., Molinari, L. & Largo, R. H. Impaired intellectual development in children with Type I diabetes: association with HbA(1c), age at diagnosis and sex. Diabetologia 45 , 108–114 (2002).
Ferguson, S. C. et al . Influence of an early-onset age of type 1 diabetes on cerebral structure and cognitive function. Diabetes Care 28 , 1431–1437 (2005).
Northam, E. A. et al . Neuropsychological profiles of children with type 1 diabetes 6 years after disease onset. Diabetes Care 24 , 1541–1546 (2001).
Brink, S. et al . Sick day management in children and adolescents with diabetes. Pediatr. Diabetes 10 (Suppl. 12), 146–153 (2009).
Laffel, L. M. et al . Sick day management using blood 3-hydroxybutyrate (3-OHB) compared with urine ketone monitoring reduces hospital visits in young people with T1DM: a randomized clinical trial. Diabet. Med. 23 , 278–284 (2006).
Hatton, D. L., Canam, C., Thorne, S. & Hughes, A. M. Parents' perceptions of caring for an infant or toddler with diabetes. J. Adv. Nurs. 22 , 569–577 (1995).
Karges, B. et al . Low discomfort and pain associated with intensified insulin therapy in children and adolescents. Diabetes Res. Clin. Pract. 80 , 96–101 (2008).
Haugstvedt, A., Wentzel-Larsen, T., Rokne, B. & Graue, M. Perceived family burden and emotional distress: similarities and differences between mothers and fathers of children with type 1 diabetes in a population-based study. Pediatr. Diabetes 12 , 107–114 (2011).
Patton, S. R., Dolan, L. M., Smith, L. B., Thomas, I. H. & Powers, S. W. Pediatric parenting stress and its relation to depressive symptoms and fear of hypoglycemia in parents of young children with type 1 diabetes mellitus. J. Clin. Psychol. Med. Settings http://dx.doi.org/10.1007/s10880-011-9256-1 .
Forsander, G. A., Sundelin, J. & Persson, B. Influence of the initial management regimen and family social situation on glycemic control and medical care in children with type I diabetes mellitus. Acta Paediatr. 89 , 1462–1468 (2000).
Sullivan-Bolyai, S. et al . Helping other mothers effectively work at raising young children with type 1 diabetes. Diabetes Educ. 30 , 476–484 (2004).
Chisholm, V. et al . Predictors of treatment adherence in young children with type 1 diabetes. J. Adv. Nurs. 57 , 482–493 (2007).
Winkley, K., Ismail, K., Landau, S. & Eisler, I. Psychological interventions to improve glycaemic control in patients with type 1 diabetes: systematic review and meta-analysis of randomised controlled trials. BMJ 333 , 65 (2006).
Pihoker, C., Forsander, G., Wolfsdorf, J. & Klingensmith, G. J. The delivery of ambulatory diabetes care to children and adolescents with diabetes. Pediatr. Diabetes 10 (Suppl. 12), 58–70 (2009).
Fröhlich-Reiterer, E. E. et al . Anthropometry, metabolic control, and follow-up in children and adolescents with type 1 diabetes mellitus and biopsy-proven celiac disease. J. Pediatr. 158 , 589–593 e2 (2011).
Warncke, K. et al . Polyendocrinopathy in children, adolescents, and young adults with type 1 diabetes: a multicenter analysis of 28,671 patients from the German/Austrian DPV-Wiss database. Diabetes Care 33 , 2010–2012 (2010).
Jansà, M. et al . Telecare in a structured therapeutic education programme addressed to patients with type 1 diabetes and poor metabolic control. Diabetes Res. Clin. Pract. 74 , 26–32 (2006).
Gerstl, E. M. et al . Metabolic control as reflected by HbA1c in children, adolescents and young adults with type-1 diabetes mellitus: combined longitudinal analysis including 27,035 patients from 207 centers in Germany and Austria during the last decade. Eur. J. Pediatr. 167 , 447–453 (2008).
Margeirsdottir, H. D., Larsen, J. R., Kummernes, S. J., Brunborg, C. & Dahl-Jorgensen, K. The establishment of a new national network leads to quality improvement in childhood diabetes: implementation of the ISPAD Guidelines. Pediatr. Diabetes 11 , 88–95 (2010).
Svensson, J., Johannesen, J., Mortensen, H. B. & Nordly, S. Improved metabolic outcome in a Danish diabetic paediatric population aged 0–18 yr: results from a nationwide continuous Registration. Pediatr. Diabetes 10 , 461–467 (2009).
de Beaufort, C. E. et al . Continuing stability of center differences in pediatric diabetes care: do advances in diabetes treatment improve outcome? The Hvidoere Study Group on Childhood Diabetes. Diabetes Care 30 , 2245–2250 (2007).
Karges, B. et al . Long-acting insulin analogs and the risk of diabetic ketoacidosis in children and adolescents with type 1 diabetes: a prospective study of 10,682 patients from 271 institutions. Diabetes Care 33 , 1031–1033 (2010).
Download references
Acknowledgements
The authors' work was supported by the BMBF Kompetenznetz Diabetes Mellitus (Competence Network for Diabetes Mellitus) funded by the Federal Ministry of Education and Research (FKZ 01GI0859).
Author information
Authors and affiliations.
Division of Endocrinology and Diabetes, RWTH Aachen University, Pauwelsstraße 30, Aachen, D-52074, Germany
Beate Karges
Department of General Pediatrics and Neonatology, University Children's Hospital, Heinrich Heine University of Düsseldorf, Moorenstraße 5, Düsseldorf, D-40225, Germany
- Thomas Meissner
Department of Public Health and German Diabetes Center, Heinrich Heine University of Düsseldorf, Moorenstraße 5, Düsseldorf, D-40225, Germany
Andrea Icks
Department of Pediatrics, University of Leipzig, Liebigstraße 20a, Leipzig, D-04103, Germany
Thomas Kapellen
Institute of Epidemiology and Medical Biometry, University of Ulm, Albert-Einstein-Allee 41, Ulm, D-89081, Germany
- Reinhard W. Holl
You can also search for this author in PubMed Google Scholar
Contributions
B. Karges, A. Icks, T. Kapellen and R. W. Holl researched the data for the article. All authors contributed equally to all other aspects of the article.
Corresponding author
Correspondence to Beate Karges .
Ethics declarations
Competing interests.
T. Kapellen declares an association with the following companies: Medtronic (speakers bureau), Roche (speakers bureau). The other authors declare no competing interests.
Rights and permissions
Reprints and permissions
About this article
Cite this article.
Karges, B., Meissner, T., Icks, A. et al. Management of diabetes mellitus in infants. Nat Rev Endocrinol 8 , 201–211 (2012). https://doi.org/10.1038/nrendo.2011.204
Download citation
Published : 29 November 2011
Issue Date : April 2012
DOI : https://doi.org/10.1038/nrendo.2011.204
Share this article
Anyone you share the following link with will be able to read this content:
Sorry, a shareable link is not currently available for this article.
Provided by the Springer Nature SharedIt content-sharing initiative
This article is cited by
Diabetes management in wolcott-rallison syndrome: analysis from the german/austrian dpv database.
- Alena Welters
Orphanet Journal of Rare Diseases (2020)
Case report: maternal mosaicism resulting in inheritance of a novel GATA6 mutation causing pancreatic agenesis and neonatal diabetes mellitus
- Elisa De Franco
- John J. Mitchell
Diagnostic Pathology (2017)
Ketoacidosis at first presentation of type 1 diabetes mellitus among children: a study from Kuwait
- Azza Aly Shaltout
- Arshad Mohamed Channanath
- M. Alkhawari
Scientific Reports (2016)
Quick links
- Explore articles by subject
- Guide to authors
- Editorial policies
Sign up for the Nature Briefing newsletter — what matters in science, free to your inbox daily.

- ajp-v1-id1004
Introduction
Case report.

Austin J Pediatr. 2014;1(1): 1004.
A Case of Neonatal Diabetes Presentation, Diagnosis and Management
Michael Yafi*
Division of Pediatric Endocrinology, University of Texas Houston Health Science Center, USA
*Corresponding author: Michael Yafi, Division of Pediatric Endocrinology, University of Texas Houston Health Science Center, 6431 Fannin Street, Suite 3.122, Houston, TX 77030, USA
Received: April 18, 2014; Accepted: May 19, 2014; Published: May 20, 2014
We present a case of neonatal diabetes with special focus on the diagnostic therapeutic and education problems faced by the pediatric endocrinology team (physicians, nurses and diabetes educators) during the hospital course.
Keywords: NDM: Neonatal Diabetes Mellitus
Neonatal diabetes mellitus (NDM) is an extremely rare presentation of diabetes. Affected infants are often found to be hyperglycemic (but rarely ketotic). Once considered a single disease, neonatal DM is now known to be caused by mutation affecting insulin synthesis and release, and by several mutations causing severe insulin resistance. Knowing the cause is important to selection of appropriate therapy. The therapy is also different from the typical pediatric because of size and diet. Training the family to care for the diabetic neonate is challenging for all. This case report will review the presentation, work up needed to reach the diagnosis, and the management plan and goals.
A preterm male infant was born at 37 weeks’ gestation, weighing 1565 grams. His mother is a 25 year old G2P0010. The mother denied any history of diabetes. The baby’s parents were first cousins of Pakistani origin. Her pregnancy was complicated by intrauterine growth retardation (IUGR) and low amniotic fluid index score (AFI). Labor was induced because of severe IUGR.
The APGAR scores were 8 at 1 minute and 9 at 5 minutes. Patient was admitted to the neonatal intensive care unit because of the severely wasted appearance. On physical examination at birth, temperature was 96.5°–97.1° F, heart rate 118, respiratory rate 31, and blood pressure 83⁄48. The patient was very pale with light skin; the head was normocephalic without deformities. Cardiovascular and respiratory examinations were normal. The abdomen was soft, not distended, with a 3 vessel cord. The genitourinary examination showed bilateral descended testicles. The neurological examination showed excellent tone. Weight, length and FOC were below 3rd percentile.
Initial glucose levels were 85,81,91 mg⁄dl
Initial documented central glucose level was 175 mg⁄dl, but at 24 hours of life patient started having hyperglycemia with central glucose measurements of 697 and 843 mg⁄dl. At the age of 25 hours, an insulin drip was started at 0.1 units ⁄Kg⁄h and the pediatric endocrinology team was consulted.
I – Medical Workup
A. laboratory work.
- Central glucose level: The first three levels were 175–697– 843 mg⁄dl
- Insulin level (obtained after starting insulin drip) was 0.5 IU⁄ml at day 2 of life (normal fasting range 2–13 iu⁄ml)
- C–peptide levels (obtained after starting insulin drip) was 0.02 ng⁄ml at day 2 of life and less than 0.05 ng⁄ml at day 4 of life (normal levels 0.5–2.7 ng⁄ml)
- Lipid panel, liver enzymes, electrolytes were normal
- Hemoglobin A1C level obtained at day 29 of life was 6.3 %
B. Radiology Studies
Abdominal ultrasound showed no pancreatic abnormalities.
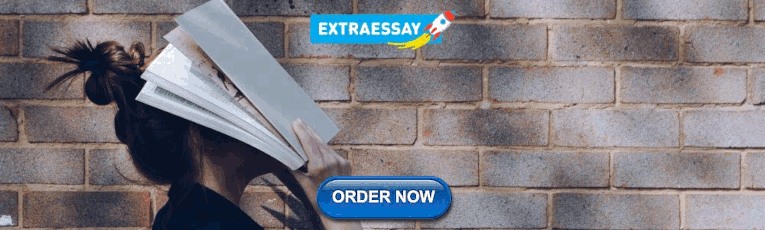
C. Genetic Testing
The neonatal diabetes mellitus by Athena Diagnostic was obtained. This test can detect mutations (point mutations, deletions, insertions and re–arrangements) in the coding sequences of the most common genes that are known to cause this condition including:
Glucokinase (GCK)
Potassium channel J11 (KCNJ11)
ATP–binding cassette transporter subfamily C member 8 (ABCC8)
Insulin (INS)
Insulin promoter factor 1 (IPF1)
The methodology uses Polymerase Chain Reaction (PCR), DNA sequencing of entire protein coding regions of genes.
The result of this DNA sequencing of the most common genes causing neonatal diabetes (IPF1, GCK, KCnJII and ABCC8) was unremarkable. However, a homozygous intronic mutation was found in the insulin gene:
No other abnormal variants were detected. Therefore, this was assumed to be the cause of neonatal diabetes. The parents were offered testing for the same mutation but declined.
II � Management plan and goals
At the seventh day of life, patient started feeding on breast milk with some added formula (Neosure 22). He was able to feed every 3 hours. At that time we changed the insulin therapy from the insulin drip to basal bolus subcutaneous insulin synchronized with feeds, as follows:
- Insulin glargine (r DNA origin) 0.25 units daily
- Insulin lispro 0.25 units every six hours (every other feeds) for glucose levels 300–450 mg⁄dl and 0.5 units for glucose levels above 450 mg⁄dl
The hospital pharmacist was able to dilute insulin to reach low concentration. Each 1 ml insulin was diluted with 3 ml of normal saline before each dose with estimated viability of 1 hour.
The blood sugar was tested prior to each feed. Short acting insulin dose was not given when blood sugar was below 100 mg⁄dl. The baby had few hypoglycemic episodes that were corrected by glucose infusions. There was no seizure episodes related to hypoglycemia. Continuous Glucose Monitoring Sensor (CGMS) was considered to monitor fluctuations in blood sugar, but lack of ample subcutaneous fat precluded the stability of the sensor’s needle. The aim of the therapy was to treat hyperglycemia while preventing hypoglycemia episodes. Blood glucose was kept in the range of 150–200 mg⁄dl.
At two months of age, the patient had gained weight (3.35 kg), had increased subcutaneous fat and was requiring a larger daily dose of long acting insulin (total dose of 2 units per day). The patient was discharged home at the age of two months with scheduled follow–up in 1 week.
The major obstacles in treating neonatal diabetes mellitus that we faced were:
- Difficulty in preparing very low dose insulin. Most of the manufacturers of commercially available insulin advise against any form of dilutions to prevent any possibility of dysfunction. We had no other choice.
- Difficulty with insulin administration in a very thin newborn with lack of subcutaneous fat tissue. ntramuscular insulin injection may also cause erratic insulin absorption
- Difficulty in predicting milk intake. Like any normal newborn, feeding may be variable. The patient experienced hypoglycemic episodes on days that he was not able to finish or tolerate his feeds.
- Consequences of multiple blood sticks to test blood sugar and frequent blood draws that could theoretically cause anemia. We have adhered to our hospital protocol to prevent this from occurring.
- Physical and psychological factors that affected the family members of the patient on daily diabetes management.
Neonatal diabetes mellitus (NDM) is an extremely rare presentation of diabetes. The National Institute of Health (NIH) estimates the incidence of NDM between 1 in 100,000 to 1 in 500,000 live births. [1] Varying by region.
An Italian study reported an incidence of 1 in 90,000 live births in Italy [2]. Since the condition is usually caused by a single gene mutation, it is expected to have a higher incidence in geographical regions with high rates of consanguinity, as was seen in our subject family.
Initially neonatal diabetes seemed divided in two types: Transient (TNDM) and Permanent (PNDM). This classification clearly depends on the duration and progression of the condition [3]. In almost 50% of the cases, NDM is transient disappearing in infancy with possibility of recurrence later on in life. In the other half, NDM is permanent.
NDM should be differentiated from other causes of hyperglycemia in the newborn including iatrogenic causes, stress and insulin resistance [4].
NDM should be differentiated also from type 1 diabetes mellitus (autoimmune) that may start at early infancy (as early as 6 months of age) [1]. Type 1 diabetes is almost always associated with positive immune markers for diabetes (Table).

Neonatal Hyperglycemia | |||||||
Iatrogenic Stress | Neonatal Diabetes Mellitus (NDM) | ||||||
| Transient TNDM | Recurrence
| Permanent PNDM | ||||
| Negative Immune markers Early presentation | Positive Immune Markers Age after 6 months | |||||
Syndromic form | Specific Genetic Mutation | ||||||
Congenital Lipodystrophy | Insulin Resistance | Common | Rare | Type 1 diabetes mellitus | |||
| KCNJII GCK ABCC8 IPF1 | INS FOXP3 ZPF57 EIF2AK3 SLC2A2 |
|
Persistent hyperglycemia, insulin deficiency (low insulin and C–peptide levels) are common features in making the diagnosis. Lack of immune markers for type 1 diabetes may be deceiving for late onset presentation. The most important aspect of the confirming the diagnosis is molecular genetic testing. There are more than a dozen genes⁄loci associated with neonatal diabetes [5–9].
Some examples of the known mutations causing neonatal diabetes are: [5].
6q24, solute carrier family 2A2 (SLC2A2)
SLC 19 A2, eukaryotic translation initiation factor 2 alpha kinase 3 (EIF2AK3)
Pancreas transcription factor 1 subunit alpha (IPF1A)
Hepatocyte nuclear factor 1 homeobox B (HNF1B)
Forkhead box P 3 (FOXP3)
Zinc finger protein 57 (ZFP57)
The prevalence of these mutations can vary from one region to another worldwide [5,10] but KCNJII, ABCC8, GCK and IPF1 are the most commonly reported.
Genetic testing may play a very important role in transferring therapy from insulin to sulfonylurea since patients with certain mutations in the pancreatic ATP sensitive K+ channel proteins like the sulfonylurea receptor 1 (SUR1) and inward rectifier K+ channel Kir 6.2 (Kir 6.2) may respond well to sulfonylurea therapy [10–13] instead of insulin.
There are some syndromic forms of neonatal diabetes described like Berardinelli–Seip Syndrome [14], associated with insulin resistance and congenital lipodystrophy and Wolcott–Rallison Syndrome [15] associated with multiple epiphyseal dysplasia, osteopenia, mental retardation and hepatic and renal dysfunction.
- National Diabetes Information Clearinghouse (NDIC)
- Iafusco D, Massa O, Pasquino B, Colombo C, Iughetti L, Bizzarri C, et al. Minimal incidence of neonatal/infancy onset diabetes in Italy is 1:90,000 live births. Acta Diabetol. 2012; 49: 405-408.
- Temple IK, Gardner RJ, Mackay DJ, Barber JC, Robinson DO, Shield JP. Transient neonatal diabetes: widening the understanding of the etiopathogenesis of diabetes. Diabetes. 2000; 49: 1359-1366.
- Hemachandra A, Cowett R. Neonatal hyperglycemia. Pediatrics in Review.1999; 20: e16-e24.
- Jahnavi S, Poovazhagi V, Mohan V, Bodhini D, Raghupathy P, Amutha A, et al. Clinical and molecular characterization of neonatal diabetes and monogenic syndromic diabetes in Asian Indian children. Clin Genet. 2013; 83: 439-445.
- Babenko AP, Polak M, Cavé H, Busiah K, Czernichow P, Scharfmann R, et al. Activating mutations in the ABCC8 gene in neonatal diabetes mellitus. N Engl J Med. 2006; 355: 456-466.
- Gloyn AL, Pearson ER, Antcliff JF, Proks P, Bruining GJ, Slingerland AS, et al. Activating mutations in the gene encoding the ATP-sensitive potassium-channel subunit Kir6.2 and permanent neonatal diabetes. N Engl J Med. 2004; 350: 1838-1849.
- Clement JP 4th, Kunjilwar K, Gonzalez G, Schwanstecher M, Panten U, Aguilar-Bryan L, et al. Association and stoichiometry of K(ATP) channel subunits. Neuron. 1997; 18: 827-838.
- Ellard S, Flanagan SE, Girard CA, Patch AM, Harries LW, Parrish A, et al. Permanent neonatal diabetes caused by dominant, recessive, or compound heterozygous SUR1 mutations with opposite functional effects. Am J Hum Genet. 2007; 81: 375-382.
- Stanik J, Gasperikova D, Paskova M, Barak L, Javorkova J, Jancova E, et al. Prevalence of permanent neonatal diabetes in Slovakia and successful replacement of insulin with sulfonylurea therapy in KCNJ11 and ABCC8 mutation carriers. J Clin Endocrinol Metab. 2007; 92: 1276-1282.
- Sagen JV, Raeder H, Hathout E, Shehadeh N, Gudmundsson K, Baevre H, et al. Permanent neonatal diabetes due to mutations in KCNJ11 encoding Kir6.2: patient characteristics and initial response to sulfonylurea therapy. Diabetes. 2004; 53: 2713-2718.
- Pearson ER, Flechtner I, Njølstad PR, Malecki MT, Flanagan SE, Larkin B, et al. Switching from insulin to oral sulfonylureas in patients with diabetes due to Kir6.2 mutations. N Engl J Med. 2006; 355: 467-477.
- Rafiq M, Flanagan SE, Patch AM, Shields BM, Ellard S, Hattersley AT. Neonatal Diabetes International Collaborative Group. Effective treatment with oral sulfonylureas in patients with diabetes due to sulfonylurea receptor 1 (SUR1) mutations. Diabetes Care. 2008; 31: 204-209.
- Agarwal AK, Garg A. Congenital generalized lipodystrophy: significance of triglyceride biosynthetic pathways. Trends Endocrinol Metab. 2003; 14: 214-221.
- Rubio-Cabezas O, Patch AM, Minton JA, Flanagan SE, Edghill EL, Hussain K, et al. Wolcott-Rallison syndrome is the most common genetic cause of permanent neonatal diabetes in consanguineous families. J Clin Endocrinol Metab. 2009; 94: 4162-4170.

Citation: Yafi M. A Case of Neonatal Diabetes Presentation, Diagnosis and Management. Austin J Pediatr. 2014;1(1): 1004. ISSN: 2381-8999
Europe PMC requires Javascript to function effectively.
Either your web browser doesn't support Javascript or it is currently turned off. In the latter case, please turn on Javascript support in your web browser and reload this page.
Classification of Neonatal Diabetes
- First Online: 08 April 2023
Cite this chapter
- Elisa De Franco 3 &
- Matthew B. Johnson 3
161 Accesses
1 Citations
Neonatal Diabetes Mellitus (NDM) is diagnosed in the first 6 months of life and is most likely to have a monogenic cause rather than being polygenic type 1 diabetes.
Before widespread genetic testing and the identification of the main genetic subtypes, NDM was classified based on disease progression and presence of additional syndromic features. Recent genetic discoveries have identified >30 genetic causes of NDM, mostly affecting genes involved in beta-cell development, function, and survival. A molecular diagnosis guides treatment and is now the gold standard for NDM disease classification.
This chapter discusses the clinical and genetic features of neonatal and early-onset diabetes, and how NDM classification helps management of the patients’ disease.
This is a preview of subscription content, log in via an institution to check access.
Access this chapter
Subscribe and save.
- Get 10 units per month
- Download Article/Chapter or eBook
- 1 Unit = 1 Article or 1 Chapter
- Cancel anytime
- Available as PDF
- Read on any device
- Instant download
- Own it forever
- Available as EPUB and PDF
- Compact, lightweight edition
- Dispatched in 3 to 5 business days
- Free shipping worldwide - see info
- Durable hardcover edition
Tax calculation will be finalised at checkout
Purchases are for personal use only
Institutional subscriptions
Edghill EL, Dix RJ, Flanagan SE, Bingley PJ, Hattersley AT, Ellard S, et al. HLA genotyping supports a nonautoimmune etiology in patients diagnosed with diabetes under the age of 6 months. Diabetes. 2006;55(6):1895–8.
Article CAS PubMed Google Scholar
Iafusco D, Stazi MA, Cotichini R, Cotellessa M, Martinucci ME, Mazzella M, et al. Permanent diabetes mellitus in the first year of life. Diabetologia. 2002;45(6):798–804.
Flanagan SE, Patch AM, Mackay DJ, Edghill EL, Gloyn AL, Robinson D, et al. Mutations in ATP-sensitive K+ channel genes cause transient neonatal diabetes and permanent diabetes in childhood or adulthood. Diabetes. 2007;56(7):1930–7.
Gardner RJ, Mackay DJ, Mungall AJ, Polychronakos C, Siebert R, Shield JP, et al. An imprinted locus associated with transient neonatal diabetes mellitus. Hum Mol Genet. 2000;9(4):589–96.
Babenko AP, Polak M, Cave H, Busiah K, Czernichow P, Scharfmann R, et al. Activating mutations in the ABCC8 gene in neonatal diabetes mellitus. N Engl J Med. 2006;355(5):456–66.
Gloyn AL, Reimann F, Girard C, Edghill EL, Proks P, Pearson ER, et al. Relapsing diabetes can result from moderately activating mutations in KCNJ11. Hum Mol Genet. 2005;14(7):925–34.
Pearson ER, Flechtner I, Njolstad PR, Malecki MT, Flanagan SE, Larkin B, et al. Switching from insulin to oral sulfonylureas in patients with diabetes due to Kir6.2 mutations. N Engl J Med. 2006;355(5):467–77.
Rafiq M, Flanagan SE, Patch AM, Shields BM, Ellard S, Hattersley AT. Effective treatment with oral sulfonylureas in patients with diabetes due to sulfonylurea receptor 1 (SUR1) mutations. Diabetes Care. 2008;31(2):204–9.
De Franco E, Flanagan SE, Houghton JA, Lango Allen H, Mackay DJ, Temple IK, et al. The effect of early, comprehensive genomic testing on clinical care in neonatal diabetes: an international cohort study. Lancet. 2015;386(9997):957–63.
Article PubMed PubMed Central Google Scholar
Allen HL, Flanagan SE, Shaw-Smith C, De Franco E, Akerman I, Caswell R, et al. GATA6 haploinsufficiency causes pancreatic agenesis in humans. Nat Genet. 2011;44(1):20–2.
Gloyn AL, Pearson ER, Antcliff JF, Proks P, Bruining GJ, Slingerland AS, et al. Activating mutations in the gene encoding the ATP-sensitive potassium-channel subunit Kir6.2 and permanent neonatal diabetes. N Engl J Med. 2004;350(18):1838–49.
Proks P, Arnold AL, Bruining J, Girard C, Flanagan SE, Larkin B, et al. A heterozygous activating mutation in the sulphonylurea receptor SUR1 (ABCC8) causes neonatal diabetes. Hum Mol Genet. 2006;15(11):1793–800.
Ellard S, Flanagan SE, Girard CA, Patch AM, Harries LW, Parrish A, et al. Permanent neonatal diabetes caused by dominant, recessive, or compound heterozygous SUR1 mutations with opposite functional effects. Am J Hum Genet. 2007;81(2):375–82.
Article CAS PubMed PubMed Central Google Scholar
Bowman P, Day J, Torrens L, Shepherd MH, Knight BA, Ford TJ, et al. Cognitive, neurological, and behavioral features in adults with KCNJ11 neonatal diabetes. Diabetes Care. 2019;42(2):215–24.
Busiah K, Drunat S, Vaivre-Douret L, Bonnefond A, Simon A, Flechtner I, et al. Neuropsychological dysfunction and developmental defects associated with genetic changes in infants with neonatal diabetes mellitus: a prospective cohort study [corrected]. Lancet Diabetes Endocrinol. 2013;1(3):199–207.
Bowman P, Sulen A, Barbetti F, Beltrand J, Svalastoga P, Codner E, et al. Effectiveness and safety of long-term treatment with sulfonylureas in patients with neonatal diabetes due to KCNJ11 mutations: an international cohort study. Lancet Diabetes Endocrinol. 2018;6:637.
Bowman P, Mathews F, Barbetti F, Shepherd MH, Sanchez J, Piccini B, et al. Long-term follow-up of glycemic and neurological outcomes in an international series of patients with sulfonylurea-treated ABCC8 permanent neonatal diabetes. Diabetes Care. 2021;44(1):35–42.
Garin I, Edghill EL, Akerman I, Rubio-Cabezas O, Rica I, Locke JM, et al. Recessive mutations in the INS gene result in neonatal diabetes through reduced insulin biosynthesis. Proc Natl Acad Sci U S A. 2010;107(7):3105–10.
Njolstad PR, Sovik O, Cuesta-Munoz A, Bjorkhaug L, Massa O, Barbetti F, et al. Neonatal diabetes mellitus due to complete glucokinase deficiency. N Engl J Med. 2001;344(21):1588–92.
Vionnet N, Stoffel M, Takeda J, Yasuda K, Bell GI, Zouali H, et al. Nonsense mutation in the glucokinase gene causes early-onset non-insulin-dependent diabetes mellitus. Nature. 1992;356(6371):721–2.
Raimondo A, Chakera AJ, Thomsen SK, Colclough K, Barrett A, De Franco E, et al. Phenotypic severity of homozygous GCK mutations causing neonatal or childhood-onset diabetes is primarily mediated through effects on protein stability. Hum Mol Genet. 2014;23(24):6432–40.
Santer R, Groth S, Kinner M, Dombrowski A, Berry GT, Brodehl J, et al. The mutation spectrum of the facilitative glucose transporter gene SLC2A2 (GLUT2) in patients with Fanconi-Bickel syndrome. Hum Genet. 2002;110(1):21–9.
Sansbury FH, Flanagan SE, Houghton JA, Shuixian Shen FL, Al-Senani AM, Habeb AM, et al. SLC2A2 mutations can cause neonatal diabetes, suggesting GLUT2 may have a role in human insulin secretion. Diabetologia. 2012;55(9):2381–5.
Labay V, Raz T, Baron D, Mandel H, Williams H, Barrett T, et al. Mutations in SLC19A2 cause thiamine-responsive megaloblastic anaemia associated with diabetes mellitus and deafness. Nat Genet. 1999;22(3):300–4.
Bay A, Keskin M, Hizli S, Uygun H, Dai A, Gumruk F. Thiamine-responsive megaloblastic anemia syndrome. Int J Hematol. 2010;92(3):524–6.
Article PubMed Google Scholar
Bergmann AK, Sahai I, Falcone JF, Fleming J, Bagg A, Borgna-Pignati C, et al. Thiamine-responsive megaloblastic anemia: identification of novel compound heterozygotes and mutation update. J Pediatr. 2009;155(6):888–92.e1.
Mandel H, Berant M, Hazani A, Naveh Y. Thiamine-dependent beriberi in the “thiamine-responsive anemia syndrome”. N Engl J Med. 1984;311(13):836–8.
Habeb AM, Flanagan SE, Zulali MA, Abdullah MA, Pomahacova R, Boyadzhiev V, et al. Pharmacogenomics in diabetes: outcomes of thiamine therapy in TRMA syndrome. Diabetologia. 2018;61(5):1027–36.
De Franco E, Watson RA, Weninger WJ, Wong CC, Flanagan SE, Caswell R, et al. A specific CNOT1 mutation results in a novel syndrome of pancreatic agenesis and holoprosencephaly through impaired pancreatic and neurological development. Am J Hum Genet. 2019;104(5):985–9.
Stoffers DA, Zinkin NT, Stanojevic V, Clarke WL, Habener JF. Pancreatic agenesis attributable to a single nucleotide deletion in the human IPF1 gene coding sequence. Nat Genet. 1997;15(1):106–10.
Jonsson J, Carlsson L, Edlund T, Edlund H. Insulin-promoter-factor 1 is required for pancreas development in mice. Nature. 1994;371(6498):606–9.
Nicolino M, Claiborn KC, Senee V, Boland A, Stoffers DA, Julier C. A novel hypomorphic PDX1 mutation responsible for permanent neonatal diabetes with subclinical exocrine deficiency. Diabetes. 2010;59(3):733–40.
De Franco E, Shaw-Smith C, Flanagan SE, Edghill EL, Wolf J, Otte V, et al. Biallelic PDX1 (insulin promoter factor 1) mutations causing neonatal diabetes without exocrine pancreatic insufficiency. Diabet Med. 2013;30(5):e197–200.
Sellick GS, Barker KT, Stolte-Dijkstra I, Fleischmann C, Coleman RJ, Garrett C, et al. Mutations in PTF1A cause pancreatic and cerebellar agenesis. Nat Genet. 2004;36(12):1301–5.
Weedon MN, Cebola I, Patch AM, Flanagan SE, De Franco E, Caswell R, et al. Recessive mutations in a distal PTF1A enhancer cause isolated pancreatic agenesis. Nat Genet. 2014;46(1):61–4.
Demirbilek H, Cayir A, Flanagan SE, Yıldırım R, Kor Y, Gurbuz F, et al. Clinical characteristics and long-term follow-up of patients with diabetes due to PTF1A enhancer mutations. J Clin Endocrinol Metab. 2020;105(12):e4351–9.
Houghton JA, Swift GH, Shaw-Smith C, Flanagan SE, de Franco E, Caswell R, et al. Isolated pancreatic aplasia due to a hypomorphic PTF1A mutation. Diabetes. 2016;65(9):2810–5.
Horikawa Y, Iwasaki N, Hara M, Furuta H, Hinokio Y, Cockburn BN, et al. Mutation in hepatocyte nuclear factor-1 beta gene (TCF2) associated with MODY. Nat Genet. 1997;17(4):384–5.
Bellanne-Chantelot C, Chauveau D, Gautier JF, Dubois-Laforgue D, Clauin S, Beaufils S, et al. Clinical spectrum associated with hepatocyte nuclear factor-1beta mutations. Ann Intern Med. 2004;140(7):510–7.
Yorifuji T, Kurokawa K, Mamada M, Imai T, Kawai M, Nishi Y, et al. Neonatal diabetes mellitus and neonatal polycystic, dysplastic kidneys: phenotypically discordant recurrence of a mutation in the hepatocyte nuclear factor-1beta gene due to germline mosaicism. J Clin Endocrinol Metab. 2004;89(6):2905–8.
Edghill EL, Bingham C, Slingerland AS, Minton JA, Noordam C, Ellard S, et al. Hepatocyte nuclear factor-1 beta mutations cause neonatal diabetes and intrauterine growth retardation: support for a critical role of HNF-1beta in human pancreatic development. Diabet Med. 2006;23(12):1301–6.
Smith SB, Qu HQ, Taleb N, Kishimoto NY, Scheel DW, Lu Y, et al. Rfx6 directs islet formation and insulin production in mice and humans. Nature. 2010;463(7282):775–80.
Sansbury FH, Kirel B, Caswell R, Lango Allen H, Flanagan SE, Hattersley AT, et al. Biallelic RFX6 mutations can cause childhood as well as neonatal onset diabetes mellitus. Eur J Hum Genet. 2015;23:1750.
Google Scholar
Spiegel R, Dobbie A, Hartman C, de Vries L, Ellard S, Shalev SA. Clinical characterization of a newly described neonatal diabetes syndrome caused by RFX6 mutations. Am J Med Genet A. 2011;155a(11):2821–5.
Patel KA, Kettunen J, Laakso M, Stancakova A, Laver TW, Colclough K, et al. Heterozygous RFX6 protein truncating variants are associated with MODY with reduced penetrance. Nat Commun. 2017;8(1):888.
Kodo K, Nishizawa T, Furutani M, Arai S, Yamamura E, Joo K, et al. GATA6 mutations cause human cardiac outflow tract defects by disrupting semaphorin-plexin signaling. Proc Natl Acad Sci U S A. 2009;106(33):13933–8.
De Franco E, Shaw-Smith C, Flanagan SE, Shepherd MH, Hattersley AT, Ellard S. GATA6 mutations cause a broad phenotypic spectrum of diabetes from pancreatic agenesis to adult-onset diabetes without exocrine insufficiency. Diabetes. 2013;62(3):993–7.
Rajagopal SK, Ma Q, Obler D, Shen J, Manichaikul A, Tomita-Mitchell A, et al. Spectrum of heart disease associated with murine and human GATA4 mutation. J Mol Cell Cardiol. 2007;43(6):677–85.
Tomita-Mitchell A, Maslen CL, Morris CD, Garg V, Goldmuntz E. GATA4 sequence variants in patients with congenital heart disease. J Med Genet. 2007;44(12):779–83.
D’Amato E, Giacopelli F, Giannattasio A, D’Annunzio G, Bocciardi R, Musso M, et al. Genetic investigation in an Italian child with an unusual association of atrial septal defect, attributable to a new familial GATA4 gene mutation, and neonatal diabetes due to pancreatic agenesis. Diabet Med. 2010;27(10):1195–200.
Shaw-Smith C, De Franco E, Lango Allen H, Batlle M, Flanagan SE, Borowiec M, et al. GATA4 mutations are a cause of neonatal and childhood-onset diabetes. Diabetes. 2014;63(8):2888–94.
Kruszka P, Berger SI, Weiss K, Everson JL, Martinez AF, Hong S, et al. A CCR4-NOT transcription complex, subunit 1, CNOT1, variant associated with Holoprosencephaly. Am J Hum Genet. 2019;104(5):990–3.
Senee V, Chelala C, Duchatelet S, Feng D, Blanc H, Cossec JC, et al. Mutations in GLIS3 are responsible for a rare syndrome with neonatal diabetes mellitus and congenital hypothyroidism. Nat Genet. 2006;38:682–7.
Alghamdi KA, Alsaedi AB, Aljasser A, Altawil A, Kamal NM. Extended clinical features associated with novel Glis3 mutation: a case report. BMC Endocr Disord. 2017;17(1):14.
Dimitri P, De Franco E, Habeb AM, Gurbuz F, Moussa K, Taha D, et al. An emerging, recognizable facial phenotype in association with mutations in GLI-similar 3 (GLIS3). Am J Med Genet A. 2016;170(7):1918–23.
Splittstoesser V, Vollbach H, Plamper M, Garbe W, De Franco E, Houghton JAL, et al. Case report: extended clinical spectrum of the neonatal diabetes with congenital hypothyroidism syndrome. Front Endocrinol (Lausanne). 2021;12:665336.
Dimitri P, Habeb AM, Gurbuz F, Millward A, Wallis S, Moussa K, et al. Expanding the clinical spectrum associated with GLIS3 mutations. J Clin Endocrinol Metab. 2015;100(10):E1362–9.
Demirbilek H, Hatipoglu N, Gul U, Tatli ZU, Ellard S, Flanagan SE, et al. Permanent neonatal diabetes mellitus and neurological abnormalities due to a novel homozygous missense mutation in NEUROD1. Pediatr Diabetes. 2018;19:898.
Rubio-Cabezas O, Minton JA, Kantor I, Williams D, Ellard S, Hattersley AT. Homozygous mutations in NEUROD1 are responsible for a novel syndrome of permanent neonatal diabetes and neurological abnormalities. Diabetes. 2010;59(9):2326–31.
Wang J, Cortina G, Wu SV, Tran R, Cho JH, Tsai MJ, et al. Mutant neurogenin-3 in congenital malabsorptive diarrhea. N Engl J Med. 2006;355(3):270–80.
Hancili S, Bonnefond A, Philippe J, Vaillant E, De Graeve F, Sand O, et al. A novel NEUROG3 mutation in neonatal diabetes associated with a neuro-intestinal syndrome. Pediatr Diabetes. 2018;19(3):381–7.
Pinney SE, Oliver-Krasinski J, Ernst L, Hughes N, Patel P, Stoffers DA, et al. Neonatal diabetes and congenital malabsorptive diarrhea attributable to a novel mutation in the human neurogenin-3 gene coding sequence. J Clin Endocrinol Metab. 2011;96(7):1960–5.
Rubio-Cabezas O, Jensen JN, Hodgson MI, Codner E, Ellard S, Serup P, et al. Permanent neonatal diabetes and enteric anendocrinosis associated with biallelic mutations in NEUROG3. Diabetes. 2011;60(4):1349–53.
Solorzano-Vargas RS, Bjerknes M, Wang J, Wu SV, Garcia-Careaga MG, Pitukcheewanont P, et al. Null mutations of NEUROG3 are associated with delayed-onset diabetes mellitus. JCI Insight. 2020;5(1)
Auerbach A, Cohen A, Ofek Shlomai N, Weinberg-Shukron A, Gulsuner S, King MC, et al. NKX2-2 mutation causes congenital diabetes and infantile obesity with paradoxical glucose-induced ghrelin secretion. J Clin Endocrinol Metab. 2020;105(11):3486.
Article Google Scholar
Flanagan SE, De Franco E, Lango Allen H, Zerah M, Abdul-Rasoul MM, Edge JA, et al. Analysis of transcription factors key for mouse pancreatic development establishes NKX2-2 and MNX1 mutations as causes of neonatal diabetes in man. Cell Metab. 2014;19(1):146–54.
Ross AJ, Ruiz-Perez V, Wang Y, Hagan DM, Scherer S, Lynch SA, et al. A homeobox gene, HLXB9, is the major locus for dominantly inherited sacral agenesis. Nat Genet. 1998;20(4):358–61.
Bonnefond A, Vaillant E, Philippe J, Skrobek B, Lobbens S, Yengo L, et al. Transcription factor gene MNX1 is a novel cause of permanent neonatal diabetes in a consanguineous family. Diabetes Metab. 2013;39(3):276–80.
Stoy J, Edghill EL, Flanagan SE, Ye H, Paz VP, Pluzhnikov A, et al. Insulin gene mutations as a cause of permanent neonatal diabetes. Proc Natl Acad Sci U S A. 2007;104(38):15040–4.
Delepine M, Nicolino M, Barrett T, Golamaully M, Lathrop GM, Julier C. EIF2AK3, encoding translation initiation factor 2-alpha kinase 3, is mutated in patients with Wolcott-Rallison syndrome. Nat Genet. 2000;25(4):406–9.
Rubio-Cabezas O, Patch AM, Minton JA, Flanagan SE, Edghill EL, Hussain K, et al. Wolcott-Rallison syndrome is the most common genetic cause of permanent neonatal diabetes in consanguineous families. J Clin Endocrinol Metab. 2009;94(11):4162–70.
Julier C, Nicolino M. Wolcott-Rallison syndrome. Orphanet J Rare Dis. 2010;5:29.
Deeb A, Habeb A, Kaplan W, Attia S, Hadi S, Osman A, et al. Genetic characteristics, clinical spectrum, and incidence of neonatal diabetes in the emirate of Abu Dhabi, United Arab Emirates. Am J Med Genet A. 2016;170(3):602–9.
Nordström J, Lundgren M, Jorns C, Fischler B, Arnell H, Dlugosz R, et al. First European case of simultaneous liver and pancreas transplantation as treatment of Wolcott-Rallison syndrome in a small child. Transplantation. 2020;104(3):522–5.
Rivera E, Gupta S, Chavers B, Quinones L, Berger MR, Schwarzenberg SJ, et al. En bloc multiorgan transplant (liver, pancreas, and kidney) for acute liver and renal failure in a patient with Wolcott-Rallison syndrome. Liver Transpl. 2016;22(3):371–4.
Tzakis AG, Nunnelley MJ, Tekin A, Buccini LD, Garcia J, Uchida K, et al. Liver, pancreas and kidney transplantation for the treatment of Wolcott-Rallison syndrome. Am J Transplant. 2015;15(2):565–7.
Poulton CJ, Schot R, Kia SK, Jones M, Verheijen FW, Venselaar H, et al. Microcephaly with simplified gyration, epilepsy, and infantile diabetes linked to inappropriate apoptosis of neural progenitors. Am J Hum Genet. 2011;89(2):265–76.
Abdel-Salam GM, Schaffer AE, Zaki MS, Dixon-Salazar T, Mostafa IS, Afifi HH, et al. A homozygous IER3IP1 mutation causes microcephaly with simplified gyral pattern, epilepsy, and permanent neonatal diabetes syndrome (MEDS). Am J Med Genet A. 2012;158a(11):2788–96.
Shalev SA, Tenenbaum-Rakover Y, Horovitz Y, Paz VP, Ye H, Carmody D, et al. Microcephaly, epilepsy, and neonatal diabetes due to compound heterozygous mutations in IER3IP1: insights into the natural history of a rare disorder. Pediatr Diabetes. 2014;15(3):252–6.
Valenzuela I, Boronat S, Martínez-Sáez E, Clemente M, Sánchez-Montañez Á, Munell F, et al. Microcephaly with simplified gyral pattern, epilepsy and permanent neonatal diabetes syndrome (MEDS). A new patient and review of the literature. Eur J Med Genet. 2017;60(10):517–20.
Skopkova M, Hennig F, Shin BS, Turner CE, Stanikova D, Brennerova K, et al. EIF2S3 mutations associated with severe X-linked intellectual disability syndrome MEHMO. Hum Mutat. 2017;38(4):409–25.
de Heredia ML, Clèries R, Nunes V. Genotypic classification of patients with Wolfram syndrome: insights into the natural history of the disease and correlation with phenotype. Genet Med. 2013;15(7):497–506.
De Franco E, Flanagan SE, Yagi T, Abreu D, Mahadevan J, Johnson MB, et al. Dominant ER stress-inducing WFS1 mutations underlie a genetic syndrome of neonatal/infancy-onset diabetes, congenital sensorineural deafness, and congenital cataracts. Diabetes. 2017;66(7):2044–53.
van der Knaap MS, Leegwater PA, Konst AA, Visser A, Naidu S, Oudejans CB, et al. Mutations in each of the five subunits of translation initiation factor eIF2B can cause leukoencephalopathy with vanishing white matter. Ann Neurol. 2002;51(2):264–70.
De Franco E, Caswell R, Johnson MB, Wakeling MN, Zung A, Dung VC, et al. De novo mutations in EIF2B1 affecting eIF2 signaling cause neonatal/early onset diabetes and transient hepatic dysfunction. Diabetes. 2019.
De Franco E, Lytrivi M, Ibrahim H, Montaser H, Wakeling MN, Fantuzzi F, et al. YIPF5 mutations cause neonatal diabetes and microcephaly through endoplasmic reticulum stress. J Clin Invest. 2020;130(12):6338–53.
Johnson MB, Patel KA, De Franco E, Houghton JAL, McDonald TJ, Ellard S, et al. A type 1 diabetes genetic risk score can discriminate monogenic autoimmunity with diabetes from early-onset clustering of polygenic autoimmunity with diabetes. Diabetologia. 2018;61(4):862–9.
Park JH, Lee KH, Jeon B, Ochs HD, Lee JS, Gee HY, et al. Immune dysregulation, polyendocrinopathy, enteropathy, X-linked (IPEX) syndrome: a systematic review. Autoimmun Rev. 2020;19(6):102526.
Fontenot JD, Gavin MA, Rudensky AY. Foxp3 programs the development and function of CD4+CD25+ regulatory T cells. Nat Immunol. 2003;4(4):330–6.
Passerini L, Barzaghi F, Curto R, Sartirana C, Barera G, Tucci F, et al. Treatment with rapamycin can restore regulatory T-cell function in IPEX patients. J Allergy Clin Immunol. 2020;145(4):1262–71.e13.
Sharfe N, Dadi HK, Shahar M, Roifman CM. Human immune disorder arising from mutation of the alpha chain of the interleukin-2 receptor. Proc Natl Acad Sci U S A. 1997;94(7):3168–71.
Caudy AA, Reddy ST, Chatila T, Atkinson JP, Verbsky JW. CD25 deficiency causes an immune dysregulation, polyendocrinopathy, enteropathy, X-linked-like syndrome, and defective IL-10 expression from CD4 lymphocytes. J Allergy Clin Immunol. 2007;119(2):482–7.
Johnson MB, Hattersley AT, Flanagan SE. Monogenic autoimmune diseases of the endocrine system. Lancet Diabetes Endocrinol. 2016;4(10):862–72.
Zorn E, Nelson EA, Mohseni M, Porcheray F, Kim H, Litsa D, et al. IL-2 regulates FOXP3 expression in human CD4+CD25+ regulatory T cells through a STAT-dependent mechanism and induces the expansion of these cells in vivo. Blood. 2006;108(5):1571–9.
Lopez-Herrera G, Tampella G, Pan-Hammarstrom Q, Herholz P, Trujillo-Vargas CM, Phadwal K, et al. Deleterious mutations in LRBA are associated with a syndrome of immune deficiency and autoimmunity. Am J Hum Genet. 2012;90(6):986–1001.
Habibi S, Zaki-Dizaji M, Rafiemanesh H, Lo B, Jamee M, Gámez-Díaz L, et al. Clinical, immunologic, and molecular spectrum of patients with LPS-responsive beige-like anchor protein deficiency: a systematic review. J Allergy Clin Immunol Pract. 2019;7(7):2379–86.e5.
Johnson MB, De Franco E, Lango Allen H, Al Senani A, Elbarbary N, Siklar Z, et al. Recessively inherited LRBA mutations cause autoimmunity presenting as neonatal diabetes. Diabetes. 2017;66(8):2316–22.
Lo B, Zhang K, Lu W, Zheng L, Zhang Q, Kanellopoulou C, et al. AUTOIMMUNE DISEASE. Patients with LRBA deficiency show CTLA4 loss and immune dysregulation responsive to abatacept therapy. Science. 2015;349(6246):436–40.
Fabre A, Marchal S, Barlogis V, Mari B, Barbry P, Rohrlich PS, et al. Clinical aspects of STAT3 gain-of-function germline mutations: a systematic review. J Allergy Clin Immunol Pract. 2019;7(6):1958–69.e9.
Flanagan SE, Haapaniemi E, Russell MA, Caswell R, Allen HL, De Franco E, et al. Activating germline mutations in STAT3 cause early-onset multi-organ autoimmune disease. Nat Genet. 2014;46(8):812–4.
Haapaniemi EM, Kaustio M, Rajala HL, van Adrichem AJ, Kainulainen L, Glumoff V, et al. Autoimmunity, hypogammaglobulinemia, lymphoproliferation, and mycobacterial disease in patients with activating mutations in STAT3. Blood. 2015;125(4):639–48.
Milner JD, Vogel TP, Forbes L, Ma CA, Stray-Pedersen A, Niemela JE, et al. Early-onset lymphoproliferation and autoimmunity caused by germline STAT3 gain-of-function mutations. Blood. 2015;125(4):591–9.
Aitken RJ, Mehers KL, Williams AJ, Brown J, Bingley PJ, Holl RW, et al. Early-onset, coexisting autoimmunity and decreased HLA-mediated susceptibility are the characteristics of diabetes in down syndrome. Diabetes Care. 2013;36(5):1181–5.
Johnson MB, De Franco E, Greeley SAW, Letourneau LR, Gillespie KM, Wakeling MN, et al. Trisomy 21 is a cause of permanent neonatal diabetes that is autoimmune but not HLA associated. Diabetes. 2019;68(7):1528–35.
Johnson MB, Patel KA, De Franco E, Hagopian W, Killian M, McDonald TJ, et al. Type 1 diabetes can present before the age of 6 months and is characterised by autoimmunity and rapid loss of beta cells. Diabetologia. 2020;63(12):2605–15.
Patel KA, Oram RA, Flanagan SE, De Franco E, Colclough K, Shepherd M, et al. Type 1 diabetes genetic risk score: a novel tool to discriminate monogenic and type 1 diabetes. Diabetes. 2016;65(7):2094–9.
Mackay DJ, Temple IK. Transient neonatal diabetes mellitus type 1. Am J Med Genet C Semin Med Genet. 2010;154c(3):335–42.
Temple IK, Gardner RJ, Robinson DO, Kibirige MS, Ferguson AW, Baum JD, et al. Further evidence for an imprinted gene for neonatal diabetes localised to chromosome 6q22-q23. Hum Mol Genet. 1996;5(8):1117–21.
Temple IK, Shield JP. Transient neonatal diabetes, a disorder of imprinting. J Med Genet. 2002;39(12):872–5.
Mackay DJ, Callaway JL, Marks SM, White HE, Acerini CL, Boonen SE, et al. Hypomethylation of multiple imprinted loci in individuals with transient neonatal diabetes is associated with mutations in ZFP57. Nat Genet. 2008;40(8):949–51.
Download references
Author information
Authors and affiliations.
Institute of Biomedical and Clinical Sciences, University of Exeter College of Medicine and Health, Exeter, UK
Elisa De Franco & Matthew B. Johnson
You can also search for this author in PubMed Google Scholar
Corresponding author
Correspondence to Elisa De Franco .
Editor information
Editors and affiliations.
Division of Pediatrics, Department of Health Sciences, University of Piemonte Orientale, Novara, Italy
Ivana Rabbone
Department of Woman, Child, and General and Specialistic Surgery, University of Campania Luigi Vanvitelli, NAPOLI, Napoli, Italy
Dario Iafusco
4.1 Electronic Supplementary Material
Data (pptx 36 kb), rights and permissions.
Reprints and permissions
Copyright information
© 2023 The Author(s), under exclusive license to Springer Nature Switzerland AG
About this chapter
De Franco, E., Johnson, M.B. (2023). Classification of Neonatal Diabetes. In: Rabbone, I., Iafusco, D. (eds) Neonatal and Early Onset Diabetes Mellitus. Springer, Cham. https://doi.org/10.1007/978-3-031-07008-2_4
Download citation
DOI : https://doi.org/10.1007/978-3-031-07008-2_4
Published : 08 April 2023
Publisher Name : Springer, Cham
Print ISBN : 978-3-031-07007-5
Online ISBN : 978-3-031-07008-2
eBook Packages : Medicine Medicine (R0)
Share this chapter
Anyone you share the following link with will be able to read this content:
Sorry, a shareable link is not currently available for this article.
Provided by the Springer Nature SharedIt content-sharing initiative
- Publish with us
Policies and ethics
- Find a journal
- Track your research
- Alzheimer's disease & dementia
- Arthritis & Rheumatism
- Attention deficit disorders
- Autism spectrum disorders
- Biomedical technology
- Diseases, Conditions, Syndromes
- Endocrinology & Metabolism
- Gastroenterology
- Gerontology & Geriatrics
- Health informatics
- Inflammatory disorders
- Medical economics
- Medical research
- Medications
- Neuroscience
- Obstetrics & gynaecology
- Oncology & Cancer
- Ophthalmology
- Overweight & Obesity
- Parkinson's & Movement disorders
- Psychology & Psychiatry
- Radiology & Imaging
- Sleep disorders
- Sports medicine & Kinesiology
- Vaccination
- Breast cancer
- Cardiovascular disease
- Chronic obstructive pulmonary disease
- Colon cancer
- Coronary artery disease
- Heart attack
- Heart disease
- High blood pressure
- Kidney disease
- Lung cancer
- Multiple sclerosis
- Myocardial infarction
- Ovarian cancer
- Post traumatic stress disorder
- Rheumatoid arthritis
- Schizophrenia
- Skin cancer
- Type 2 diabetes
- Full List »
share this!
September 4, 2024
This article has been reviewed according to Science X's editorial process and policies . Editors have highlighted the following attributes while ensuring the content's credibility:
fact-checked
peer-reviewed publication
trusted source
Study: Black/African American individuals have an increased risk for severe insulin-deficient diabetes
by Hannah Echols, University of Alabama at Birmingham

While diabetes patients are categorized into two groups, either type 1 or type 2, no two patients are the same. The simple categorization often does not portray the disease and its many presentations, especially within different populations. For this reason, diabetes researchers and clinicians have emphasized the importance of increasing the understanding of diabetes subtypes.
Researchers at the University of Alabama at Birmingham have conducted a cluster analysis of diabetes in the Deep South to understand how the disease, and its subtypes, are clustered among the diverse population. Results published in the Journal of Clinical Endocrinology and Metabolism showed that Black/African American individuals have an increased risk for severe insulin-deficient diabetes, a subtype of type 2 diabetes.
Those with SIDD typically are diagnosed at a younger age, are leaner and have worse blood sugar control, leading to higher A1c. Their beta cell function is impaired, making it harder to produce insulin, and they have a higher risk for complications such as a heart attack.
"Understanding that Black/African American individuals have an increased risk for SIDD has practical implications as it can help guide clinicians to more appropriate treatments for these individuals and hopefully improve outcomes, reduce complications and cut health care expenditures ," said Anath Shalev, M.D., the Nancy R. and Eugene C. Gwaltney Family Endowed Chair in Juvenile Diabetes Research in the UAB Division of Endocrinology, Diabetes and Metabolism in the Department of Medicine and the director of the UAB Comprehensive Diabetes Center.
Recent studies have defined new subgroups of adult-onset diabetes and their associations with disease progression and complications. However, the studies were focused primarily on Northern European or North American white/Caucasian populations.
The multidisciplinary team from the UAB Comprehensive Diabetes Center, the Hugh Kaul Precision Medicine Institute, and Division of Endocrinology, Diabetes and Metabolism wanted to determine whether similar clustering as in previous studies could be applied to diverse cohorts. They studied data from 89,875 patients with diabetes in the Deep South over a 10-year period.
"UAB is uniquely located in a region where the prevalence of diabetes is much higher than other areas of the United States and consists of a more diverse population," said Brian Lu, Ph.D., first author and researcher in the UAB Comprehensive Diabetes Center. "Our study shows that racial background strongly influences diabetes subtype distribution."
Results add to the growing literature and push for more research to define and better understand diabetes subtypes. The findings are an example of precision diabetes that can be used in practical, clinical settings .
"The more that we know about an individual's risks and specific subtype of diabetes, the more personalized we can make their care," said Matt Might, Ph.D., director of the Hugh Kaul Precision Medicine Institute. "This is another exciting discovery in precision diabetes."
Explore further
Feedback to editors

Excessive light pollution may increase risk of Alzheimer's, especially in younger people
53 minutes ago

100-fold improvement in sight seen after gene therapy trial
6 hours ago

Autoimmune disease researchers find immune cells escape therapy due to 'exhausted' state
7 hours ago

Researchers develop rapid test to detect dopamine

Study: First sustained remission of HIV infection following a bone marrow transplant in absence of protective mutation

Brain scans reveal that mindfulness meditation for pain is not a placebo
8 hours ago

Innovative computational approach yields novel cancer targets

AI-driven tool could improve brain pressure monitoring in intensive care patients
9 hours ago

Risky combinations of psychiatric drugs prescribed for young patients

New study reveals the signals in your brain that initiate spontaneous actions
Related stories.

Researchers quantify the risk for diabetes after gestational diabetes
May 10, 2024

People with well-controlled, long-duration type 1 diabetes may still face high risk of heart disease
Jun 1, 2024

Researchers use algorithm to provide innovative insights into the heterogeneity of type 2 diabetes
Mar 18, 2024

Study shows diabetes tied to higher prevalence of overactive bladder
Jun 4, 2024

New insights into adult-onset type 1 diabetes
Apr 1, 2024

Childhood obesity linked to increased risk of 4 of 5 newly proposed subtypes of adult-onset diabetes
Feb 27, 2023
Recommended for you

Low-carb/high-fat diets for weight loss may actually boost risk of type 2 diabetes
14 hours ago

Study solves testosterone's paradoxical effects in prostate cancer
Sep 4, 2024

Sex hormones modulate the immune system to influence disease risk differently, research reveals

Insulin and metformin combo aids diabetic foot ulcer healing, new study finds

Beta cells alone can regulate blood sugar levels, study finds
Sep 3, 2024

No link found between popular diabetes medication and suicide
Let us know if there is a problem with our content.
Use this form if you have come across a typo, inaccuracy or would like to send an edit request for the content on this page. For general inquiries, please use our contact form . For general feedback, use the public comments section below (please adhere to guidelines ).
Please select the most appropriate category to facilitate processing of your request
Thank you for taking time to provide your feedback to the editors.
Your feedback is important to us. However, we do not guarantee individual replies due to the high volume of messages.
E-mail the story
Your email address is used only to let the recipient know who sent the email. Neither your address nor the recipient's address will be used for any other purpose. The information you enter will appear in your e-mail message and is not retained by Medical Xpress in any form.
Newsletter sign up
Get weekly and/or daily updates delivered to your inbox. You can unsubscribe at any time and we'll never share your details to third parties.
More information Privacy policy
Donate and enjoy an ad-free experience
We keep our content available to everyone. Consider supporting Science X's mission by getting a premium account.
E-mail newsletter

An official website of the United States government
The .gov means it’s official. Federal government websites often end in .gov or .mil. Before sharing sensitive information, make sure you’re on a federal government site.
The site is secure. The https:// ensures that you are connecting to the official website and that any information you provide is encrypted and transmitted securely.
- Publications
- Account settings
Preview improvements coming to the PMC website in October 2024. Learn More or Try it out now .
- Advanced Search
- Journal List
- Case Rep Endocrinol
- v.2023; 2023
- PMC10468271

Management of Neonatal Diabetes due to a KCNJ11 Mutation with Automated Insulin Delivery System and Remote Patient Monitoring
Ming yeh lee.
1 Division of Pediatric Endocrinology, Stanford University School of Medicine, Stanford, CA, USA
Anna L. Gloyn
2 Stanford Diabetes Research Center, Stanford University School of Medicine, Stanford, CA, USA
David M. Maahs
Priya prahalad, associated data.
The data used to support the findings of this study are available from the corresponding author upon request.
Neonatal diabetes mellitus (NDM) is a monogenic form of diabetes. Management of hyperglycemia in neonates with subcutaneous insulin is challenging because of frequent feeding, variable quantity of milk intake with each feed, low insulin dose requirements, and high risk for hypoglycemia and associated complications in this population. We present a case of NDM in a proband initially presenting with focal seizures and diabetic ketoacidosis due to a pathologic mutation in the beta cell potassium ATP channel gene KCNJ11 c.679G > A (p.E227K). We describe the use of continuous glucose monitoring (CGM), insulin pump, automated insulin delivery system, and remote patient monitoring technologies to facilitate rapid and safe outpatient cross-titration from insulin to oral sulfonylurea. Our case highlights the safety and efficacy of these technologies for infants with diabetes, including improvements in glycemia, quality of life, and cost-effectiveness by shortening hospital stay.
1. Introduction
Neonatal diabetes mellitus (NDM) occurs in approximately 1 in 90,000 to 160,000 live births [ 1 ]. Genetic testing provides the diagnosis and guides clinical therapy. Mutations affecting the pancreatic beta cell potassium ATP channel genes ( KCNJ11 and ABCC8 ) are common in neonatal diabetes, and these patients can often be transitioned from insulin to oral sulfonylurea therapy [ 2 ]. In a healthy pancreatic beta cell, glucose enters via a glucose transporter, where it is metabolized, resulting in a change in the ratio between ADP and ATP which results in closure of ATP-dependent potassium (K ATP ) channels leading to membrane depolarization and activation of voltage-dependent calcium channels. The subsequent influx of calcium is the trigger for insulin release. Heterozygous activating mutations in the genes encoding for the two subunits of the K ATP channel ( KCNJ11 or ABCC8 ) result in an inability of ATP to lead to channel closure which prevents insulin secretion [ 2 ]. Sulfonylureas close the K ATP channel by an ATP-independent mechanism, allowing for insulin to be released from the beta cell [ 1 ]. Patients with this genetic etiology for their diabetes can be treated with oral sulfonylureas rather than insulin with improvements in both glycemia and quality of life (QoL) [ 3 – 5 ].
Management of NDM with subcutaneous insulin is challenging because of frequent feeding, variable quantity of milk intake with each feed, low insulin dose requirements, and high risk for hypoglycemia and associated complications in infants. Various technologies including continuous glucose monitors (CGMs), insulin pumps, automated insulin delivery (AID) systems, and remote patient monitoring (RPM) have improved glycemia and QoL for youth with type 1 diabetes [ 6 ]. These technologies are likely to improve NDM management although they have not been well studied nor have FDA approval for use in this population. CGMs have been utilized for glucose monitoring in neonates with conditions such as NDM and congenital hyperinsulinism [ 7 ]. CGMs are beneficial for alerting impending hypoglycemia, allowing caregivers to recognize and treat hypoglycemia in neonates, who otherwise have difficult to recognize hypoglycemia symptoms. Insulin pumps may benefit NDM management by (1) allowing precise delivery of small insulin doses compared to subcutaneous injections, (2) allowing flexibility of frequent insulin boluses for hyperglycemia correction or carbohydrate coverage without additional injections, and (3) minimizing the potential for stacking of insulin boluses, leading to hypoglycemia with the insulin-on-board feature [ 1 ].
AID systems combine a CGM, an insulin pump, and a dosing algorithm for insulin delivery. Guidelines from the International Society for Pediatric and Adolescent Diabetes and American Diabetes Association strongly recommend the use of AID systems for youth with diabetes, as these systems improve CGM time in range (TIR, glucose 70–180 mg/dL) [ 8 , 9 ]. Previously, AID systems have been used for the management of hyperglycemia in extremely preterm infants in the neonatal intensive care unit, demonstrating improved TIR and optimized nutritional intake without increasing the risk of hypoglycemia [ 10 ]. However, outpatient use of AID systems for management of NDM has not been reported. RPM allows flexible and timely interventions for youth with diabetes without requiring additional clinic visits [ 11 ]. Historically, titration from insulin to sulfonylurea therapy has occurred rapidly in an inpatient setting or more slowly in an outpatient setting. Our case demonstrates that early diagnosis of sulfonylurea-responsive NDM and incorporation of AID and close guidance of experienced pediatric endocrinologists led to safe, fast, and effective transition to sulfonylurea therapy and glycemic control in the outpatient setting, while improving patient and family's QoL.
2. Case Presentation
A 2-month-old female presented to the emergency department with left-sided focal seizures. She had no infectious symptoms and had been feeding and acting normally. She was born full term with history of small for gestational age (birth weight of 2205 g, 0.6 percentile). At 6 weeks of age, she had good catch up growth to 3459 g (1.58 percentile) and normal development. Her physical exam was normal with no dysmorphic features and no focal neurological deficits. Initial laboratory evaluation was notable for glucose of 604 mg/dL and mild diabetic ketoacidosis (DKA) with venous pH 7.28, beta-hydroxybutyrate 4.2 mmol/L, elevated hemoglobin A1c 8.5%, and low c-peptide 0.45 ng/ml. Head CT showed no acute intracranial abnormalities. Brain MRI was notable for foci of restricted diffusion in the bilateral frontal cerebral white matter, bilateral cerebellar white matter, and right putamen with surrounding signal abnormality. She was admitted to the pediatric intensive care unit for intravenous insulin and fluids based on the institutional DKA protocol and for seizure monitoring and treatment. She remained on broad-spectrum antibiotics until infectious workup was negative. Continuous electroencephalogram (EEG) demonstrated focal right temporal seizures that were associated with left facial and eyelid twitching. EEG also demonstrated focal slowing in the right hemisphere, which is a nonspecific indicator of cerebral dysfunction from a wide variety of potential etiologies. Pediatric neurology and radiology considered these findings most likely related to severe infantile hyperglycemic injury.
3. Treatment, Outcome, and Follow-Up
On day 2 of admission, DKA resolved and she transitioned from intravenous to subcutaneous insulin ( Figure 1 ). Genetic panel for monogenic diabetes was sent. Since her clinical presentation was highly suspicious for NDM and genetic testing was still pending, we initiated discharge planning for potential long-term insulin requirement. She was initiated on the Dexcom G6 (Dexcom, San Diego, CA) CGM for glucose monitoring and family received teaching for diabetes management. While insulin pump and AID system were recognized as tools for aiding glycemia, barriers to inpatient initiation of technologies included lack of hospital policy and lack of familiarity and comfort from primary providers and nursing staff. Thus, initiation of insulin pump and AID system was deferred until an outpatient clinic visit.

Timeline of clinical events.
Subcutaneous insulin doses were initiated during admission using basal-bolus strategy with U100 Lantus once daily and diluted U10 Humalog for hyperglycemia correction every 4 hours. Doses were titrated daily during the first week of diagnosis, to total daily insulin dose of 5 units (1.4 units/kg/day). Blood glucose target was set at 200 mg/dL due to the risk for complications from hypoglycemia. Glycemia was poor during the initial week on subcutaneous insulin regimen, with mean glucose 300 mg/dL, standard deviation 59 mg/dL, CGM TIR (glucose 70-180 mg/dl) 4%, time in hyperglycemia (glucose above 180 mg/dL) 96%, and no hypoglycemia (glucose less than 70 mg/dL) ( Figure 2 ). Challenges to management include limitation of subcutaneous insulin dose increments, insulin dose injection volume, risk of hypoglycemia, frequency of feeding, and variable amount of feeding due to changing mental status while titrating antiepileptics. Seizure control required 4 antiepileptics.

CGM glucose pattern summary data during various periods of treatment.
By day 9 after diagnosis, patient returned to baseline mental status, was feeding well, and was seizure free on a stable regimen of antiepileptics. She was discharged directly to the diabetes clinic for initiation of the t:slim X2 insulin pump (Tandem, San Diego, CA) with Control-IQ technology for AID. The pump was loaded with dilute U10 Humalog, with basal rate set to 0.1 unit/hour, carbohydrate ratio of 1 unit per 60 g carbohydrates, and correction factor of 1 unit to glucose of 400 mg/dL. The pump was set to “exercise mode” of control-IQ to enable higher glucose target. CGM and insulin data are shared with the diabetes team via Dexcom Clarity (Dexcom, San Diego, CA) and t:connect (Tandem, San Diego, CA) web portals, respectively, for RPM in between clinic visits. Glycemia was improved on AID compared with multiple daily injection insulin. Her mean glucose was 211 ± 43 mg/dL, TIR 23%, hyperglycemia 77%, and no hypoglycemia ( Figure 2 ).
Week 2 after presentation, genetic testing identified a KCNJ11 c.679G > A (p.E227K) heterozygous mutation, a pathologic variant in the gene encoding for potassium ATP channel in pancreatic beta cells [ 1 ]. Reflex genetic testing on the patient's parents were negative for the same pathologic variant, indicating that this was a de novo mutation. Rare pathogenic mutations in KCNJ11 are a cause of multiple disorders of insulin secretion with the direction of effect depending on whether mutations result in a loss or gain of function. Heterozygous activating mutations can cause several varieties of NDM, including both permanent and transient forms, with the functional severity of the mutation influencing the clinical presentation [ 2 ]. Patients with KCNJ11 activating mutations are typically responsive to treatment with oral sulfonylureas with improvements in both glycemia and QoL due to the ease of administrating oral medication versus insulin injections multiple times daily [ 1 , 3 – 5 ]. Several studies indicate that commencing treatment at the earliest opportunity may also improve neurodevelopmental outcomes in sulfonylurea-responsive patients [ 1 ].
Day 18 after diagnosis, we initiated cross-titration from insulin to glyburide, an oral sulfonylurea, based on a published protocol [ 1 ]. Typically, outpatient cross-titration of oral sulfonylurea is titrated weekly due to the risk of hypoglycemia when insulin requirement decreases. However, we were able to safely and rapidly achieve outpatient cross-titration using the AID system coupled with RPM ( Figure 3 ). The AID system minimizes risk of hypoglycemia by suspending insulin delivery in response to hypoglycemia or anticipated hypoglycemia. Asynchronous RPM with pediatric endocrinologist reviewing CGM and AIDs data every 2-3 days facilitated frequent outpatient dose adjustments. By day 9 of cross-titration, glyburide was titrated to 0.45 mg/kg/day and insulin was weaned off. This was a similar timeline as inpatient cross-titration, and much more rapid compared to outpatient cross-titration [ 1 ]. During cross-titration, glycemia continued to improve (mean glucose 169 ± 47 mg/dL, TIR 58%, hyperglycemia 41%, and hypoglycemia <1%). Review of AID data showed that insulin delivery suspended appropriately in anticipation of hypoglycemia ( Figure 4 ). On glyburide monotherapy, glycemia was achieved (mean glucose 130 ± 38 mg/dL, TIR 86%, hyperglycemia 10%, and hypoglycemia <4%). Week 5 after diagnosis, she had intermittent preprandial hypoglycemia suggestive of clinical remission from diabetes. Glyburide was weaned off with subsequent normoglycemia (mean glucose 110 ± 26 mg/dL, CGM 95%, hyperglycemia 1%, and hypoglycemia <4%) ( Figure 2 ). Overall, this clinical course is consistent with an activating mutation in KCNJ11 leading to transient NDM (TNDM). A large proportion of TNDM cases subsequently relapse and will require insulin therapy, most often during adolescence [ 1 ]. Thus, it remains important to monitor for signs and symptoms of hyperglycemia and periodically monitor hemoglobin A1c even if she is off insulin and sulfonylurea.

Timeline of insulin to glyburide cross-titration. Glyburide dose was quickly up-titrated as an outpatient every 2-3 days to maximal effect over 1 week. Insulin was safely weaned off 9 days after initiation of glyburide.

Representative CGM and insulin pump data for 24 hours during glyburide cross-titration. Two dashed lines mark anticipated hypoglycemia and appropriate suspension of insulin delivery from the pump.
4. Discussion
Some activating mutations in KCNJ11 have been associated with developmental delay, epilepsy, and neonatal diabetes (DEND) and intermediate DEND (iDEND) syndrome. In addition to the pancreatic islet, KCNJ11 is also expressed in neurons and skeletal muscles; thus, altered activity of K ATP channels in these tissues may explain the neurodevelopmental features [ 2 ]. The KCNJ11 p.E227K mutation has been reported in patients with a range of diabetes presentation including neonatal and later-onset phenotypes [ 12 – 14 ]. It has been previously described in a patient with TNDM, axial hypotonia during infancy, and moderate neurodevelopmental delay in language and mathematical reasoning during childhood [ 15 ]. However, this mutation has not been associated with seizures. In children with type 1 diabetes, long-term changes in brain structure and cognitive function have been associated with hyperglycemia [ 16 ]. Thus, our patient's initial seizures may have been provoked by hyperglycemic brain injury. She has been followed by pediatric neurology and developmental-behavioral pediatrics with no concerns about development, no more clinical seizures, and a normal EEG while weaning antiepileptics. She will continue to follow with neurology for neurodevelopmental monitoring. Given her history of seizures, it is important to closely manage her blood glucoses to avoid further neurologic insult from hyperglycemia or hypoglycemia.
In summary, our experience demonstrates that management of infants with NDM using the AID system and RPM under close guidance of experienced pediatric endocrinologists can lead to safe and effective glycemic control and at home transition to sulfonylurea therapy. Benefits of these technologies include improved TIR by minimizing hyperglycemia and hypoglycemia and improved QoL for the family. The strategy of using AID systems coupled with RPM further allows frequent outpatient medication titration, thus reducing the length of hospital stay and cost. We advocate that all children should be allowed to use these technologies inpatient and outpatient when proper supervision by experienced medical providers is available. Our report has broad implications as hospital policies and infrastructure need to be reviewed and updated regularly to prevent hindering optimal patient care through application of novel technologies.
Acknowledgments
The authors would like to thank the patient and her family. They thank Jeannine Leverenz, the certified diabetes care and education specialists, and pediatric endocrinologists at the Division of Pediatric Endocrinology and Diabetes at Stanford for their expertise in diabetes technologies, patient education, and clinical care. They thank Dr. Siri Greeley and the Monogenic Diabetes Team at the University of Chicago Kovler Diabetes Center for discussions on sulfonylurea transfer. They thank Dr. Catarina Limbert for discussions on neurodevelopmental phenotype of KCNJ11 p.E227K mutation. MYL is supported by the Elizabeth and Russell Siegelman Postdoctoral Fellowship award by the Stanford Maternal & Child Health Research Institute (MCHRI) and the Ruth L. Kirschstein National Research Service Award (NRSA) T32 Training Program in Diabetes, Endocrinology, and Metabolism at Stanford (DK007217-46). ALG, DMM, and PP are supported by the National Institute of Diabetes and Digestive and Kidney Diseases under Grant no. P30DK113358.
Data Availability
Additional points.
(i) Availability of rapid genetic testing allows possibility of early transition from insulin to sulfonylurea therapy for patients with neonatal diabetes caused by KCNJ11 and ABCC8 mutations. (ii) Glycemic management of neonates with diabetes is challenging due to limitations in subcutaneous insulin dose increments, insulin dose injection volume, high risk of hypoglycemia, high frequency of feeding, and variable amount of feeding. (iii) Management of neonatal diabetes using AID systems and RPM under close guidance of experienced pediatric endocrinologists can lead to safe and effective glycemia by minimizing both hyperglycemia and hypoglycemia.
The authors have obtained written consent from the parent of the patient.
Conflicts of Interest
ALG's spouse works for Genentech and holds stock options in Roche. The authors declare that there are no conflicts of interest.
Authors' Contributions
MYL and PP conducted data analysis and wrote the initial manuscript. ALG and DMM provided critical revisions to the manuscript. All authors have approved the final manuscript prior to submission.
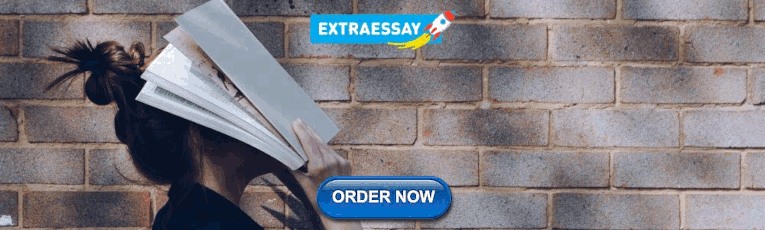
IMAGES
VIDEO
COMMENTS
Synopsis. Neonatal diabetes mellitus (also termed congenital diabetes, or diabetes of infancy) is highly likely to be due to an underlying monogenic defect when it occurs under 6 months of age. Early recognition and urgent genetic testing are important for predicting the clinical course and raising awareness of possible additional features, and ...
Learn about the causes, presentation, diagnosis, and management of neonatal diabetes mellitus, a rare form of diabetes that affects infants up to one year of age. Neonatal diabetes mellitus is usually caused by a single gene mutation affecting pancreatic beta cell function.
Monogenic diabetes is a rare form of diabetes caused by a single gene mutation that affects insulin production or action. Learn about neonatal diabetes mellitus (NDM) and maturity onset diabetes of the young (MODY), how they differ from type 1 and type 2 diabetes, and how they are diagnosed and treated.
Neonatal diabetes is a rare genetic disease with severe hyperglycaemia requiring insulin treatment before 6 months of age. It can be caused by abnormalities of the 6q24 locus, the ABCC8 or KCNJ11 genes, or pancreas malformation.
Neonatal diabetes mellitus (DM) is defined by the onset of persistent hyperglycemia within the first six months of life but may present up to 12 months of life. ... There was no difference in the age at presentation between preterm and term infants (1 week vs 0.7 weeks). 10 The diagnosis of neonatal DM, therefore, should be considered in the ...
Neonatal diabetes mellitus (NDM) is a rare insulin-requiring form of diabetes with an estimated prevalence of 1 in 100 000-300 000 live births ().Patients with NDM can be grouped into two well-defined subgroups, permanent and transient, each accounting for approximately 50% of patients ().Recent studies have indicated that diabetes in the majority of patients diagnosed with permanent NDM ...
Abstract. Neonatal diabetes mellitus is likely to be due to an underlying monogenic defect when it occurs at less than 6 months of age. Early recognition and urgent genetic testing are important for predicting the clinical course and raising awareness of possible additional features. Early treatment of sulfonylurea-responsive types of neonatal ...
Neonatal diabetes mellitus is likely to be due to an underlying monogenic defect when it occurs at less than 6 months of age. Early recognition and urgent genetic testing are important for predicting the clinical course and raising awareness of possible additional features. ... Clinical presentation of 6q24 transient neonatal diabetes mellitus ...
Neonatal diabetes is a rare form of diabetes that occurs in infants under 6 months old. Learn about the signs, causes and treatment options for this condition, which can be permanent or transient, and how to seek help from Nationwide Children's Hospital.
Transient neonatal diabetes mellitus. TNDM is caused by overexpression of an imprinted gene region in chromosome 6q24 in about 70% of cases. 4,5 Activating mutations of one of the two subunits of ...
Neonatal diabetes mellitus (NDM) is defined as insulin-requiring persistent hyperglycemia occurring in first 6 months of life. Although NDM is considered a rare disease and is usually estimated to affect one in 300,000 to 400,000 newborns, its incidence has been reported to be as high as 1:89,000 in some European countries. 1, 2 Based on the ...
Definition. Diabetes mellitus in very young children or neonatal diabetes is a rare genetic disease (minimal incidence: 1 in 90,000 live births) with variations within different ethnic groups (1 - 3). It is defined by the presence of severe hyperglycaemia requiring treatment and occurs between the neonatal period and infancy. It occurs mainly ...
The presentation of neonatal diabetes is rare and occurs in between 1 in 215,000 and 1 in 500,000 births. The diagnosis is made after symptoms develop within the first six weeks to three months of life (US National Library of Medicine, 2017. US National Library of Medicine ;
Introduction. Neonatal diabetes mellitus (NDM) is an extremely rare presentation of diabetes. Affected infants are often found to be hyperglycemic (but rarely ketotic). Once considered a single disease, neonatal DM is now known to be caused by mutation affecting insulin synthesis and release, and by several mutations causing severe insulin ...
Neonatal diabetes may not always present in the immediate neonatal period. More recent studies show that monogenic forms of NDM may still occur at up to 12 months ... Presentation Although KCNJ11/ABCC8 mutations typically lead to the onset of diabetes before 6 months of age, a diagnosis after 6 months is also possible. ...
Learn how to correct and prevent neonatal hypoglycemia, a condition that may cause neurologic and developmental problems. This article covers the causes, symptoms, diagnosis, and treatment of persistent or pathologic hypoglycemia in newborns.
Neonatal diabetes mellitus (DM) is defined by the onset of persistent hyperglycemia within the first six months of life. It is often caused by a mutation in a single gene affecting development and function of pancreatic beta cells leading to diminished insulin secretion/function. While most patients with neonatal DM present within the first 6 ...
Patients are now referred for genetic testing closer to their presentation with neonatal diabetes. Comprehensive testing of all causes identified causal mutations in more than 80% of cases. The genetic result predicts the best diabetes treatment and development of related features. This model represents a new framework for clinical care with genetic diagnosis preceding development of clinical ...
Abstract. Neonatal diabetes mellitus is likely to be due to an underlying monogenic defect when it occurs at less than 6 months of age. Early recognition and urgent genetic testing are important for predicting the clinical course and raising awareness of possible additional features. Early treatment of sulfonylurea-responsive types of neonatal ...
Neonatal diabetes mellitus (NDM) is a rare cause of hyperglycemia in the neonatal period, and its incidence is 1 in approximately 500.000 live births. [ 1] NDM is defined as a hyperglycemic condition requiring insulin therapy that emerges within the first months of life and persists for more than two weeks. NDM is caused by mutations encoding ...
Learn about the different types and causes of neonatal diabetes, a rare form of diabetes that affects infants in the first 6 months of life. Find out how genetic testing can help diagnose and manage the disease and its complications.
Results published in the Journal of Clinical Endocrinology and Metabolism showed that Black/African American individuals have an increased risk for severe insulin-deficient diabetes, a subtype of ...
The KCNJ11 p.E227K mutation has been reported in patients with a range of diabetes presentation including neonatal and later-onset phenotypes [12-14]. It has been previously described in a patient with TNDM, axial hypotonia during infancy, and moderate neurodevelopmental delay in language and mathematical reasoning during childhood . However ...