Thank you for visiting nature.com. You are using a browser version with limited support for CSS. To obtain the best experience, we recommend you use a more up to date browser (or turn off compatibility mode in Internet Explorer). In the meantime, to ensure continued support, we are displaying the site without styles and JavaScript.
- View all journals
- Explore content
- About the journal
- Publish with us
- Sign up for alerts
- Published: 04 September 2019
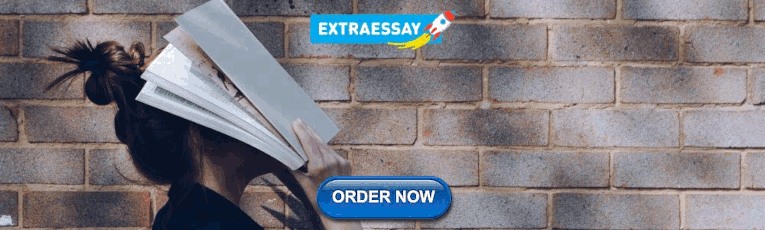
Magnitude of urban heat islands largely explained by climate and population
- Gabriele Manoli 1 nAff6 ,
- Simone Fatichi 1 ,
- Markus Schläpfer 2 ,
- Kailiang Yu 3 ,
- Thomas W. Crowther 3 ,
- Naika Meili 1 , 2 ,
- Paolo Burlando 1 ,
- Gabriel G. Katul 4 &
- Elie Bou-Zeid 5
Nature volume 573 , pages 55–60 ( 2019 ) Cite this article
31k Accesses
574 Citations
506 Altmetric
Metrics details
- Atmospheric science
- Attribution
- Climate-change mitigation
Urban heat islands (UHIs) exacerbate the risk of heat-related mortality associated with global climate change. The intensity of UHIs varies with population size and mean annual precipitation, but a unifying explanation for this variation is lacking, and there are no geographically targeted guidelines for heat mitigation. Here we analyse summertime differences between urban and rural surface temperatures (Δ T s ) worldwide and find a nonlinear increase in Δ T s with precipitation that is controlled by water or energy limitations on evapotranspiration and that modulates the scaling of Δ T s with city size. We introduce a coarse-grained model that links population, background climate, and UHI intensity, and show that urban–rural differences in evapotranspiration and convection efficiency are the main determinants of warming. The direct implication of these nonlinearities is that mitigation strategies aimed at increasing green cover and albedo are more efficient in dry regions, whereas the challenge of cooling tropical cities will require innovative solutions.
This is a preview of subscription content, access via your institution
Access options
Access Nature and 54 other Nature Portfolio journals
Get Nature+, our best-value online-access subscription
24,99 € / 30 days
cancel any time
Subscribe to this journal
Receive 51 print issues and online access
185,98 € per year
only 3,65 € per issue
Buy this article
- Purchase on SpringerLink
- Instant access to full article PDF
Prices may be subject to local taxes which are calculated during checkout
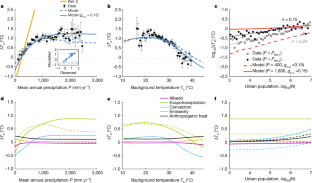
Similar content being viewed by others

Urbanization-induced warming amplifies population exposure to compound heatwaves but narrows exposure inequality between global North and South cities
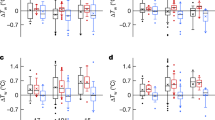
Increased heat risk in wet climate induced by urban humid heat
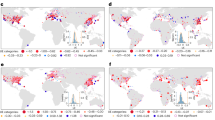
Widespread global exacerbation of extreme drought induced by urbanization
Data availability.
The Global Urban Heat Island Data Set 2013 is available at https://doi.org/10.7927/H4H70CRF (accessed on 7 December 2017). MERRA data were retrieved from https://disc.gsfc.nasa.gov/daac-bin/FTPSubset2.pl (downloaded on 4 March 2018) while GPCC data are available at https://www.esrl.noaa.gov/psd/data/gridded/data.gpcc.html (accessed on 13 September 2016). MODIS albedo data are available at https://gcmd.nasa.gov/records/GCMD_MCD43B3.html (accessed on 15 July 2018). Urban green cover data for EU and SEA cities are available, respectively, at https://ec.europa.eu/eurostat/statistics-explained/index.php/Urban_Europe_-_statistics_on_cities,_towns_and_suburbs_-_green_cities#Further_Eurostat_information (accessed on 14 June 2017) and https://doi.org/10.1016/j.landurbplan.2016.09.005 (accessed on 29 September 2017). A summary table containing the urban and climate characteristics of the cities analysed is also available on Code Ocean ( https://doi.org/10.24433/CO.9808462.v1 ).
Code availability
The MATLAB code ( https://www.mathworks.com/products/matlab.html ) of the coarse-grained UHI model is available on Code Ocean ( https://doi.org/10.24433/CO.9808462.v1 ).
Oke, T. R. City size and the urban heat island. Atmos. Environ . 7 , 769–779 (1973).
Article ADS Google Scholar
Zhao, L., Lee, X., Smith, R. B. & Oleson, K. Strong contributions of local background climate to urban heat islands. Nature 511 , 216–219 (2014).
Article ADS CAS Google Scholar
Oke, T. R., Mills, G., Christen, A. & Voogt, J. A. Urban Climates (Cambridge Univ. Press, 2017).
Grimm, N. B. et al. Global change and the ecology of cities. Science 319 , 756–760 (2008).
Rydin, Y. et al. Shaping cities for health: complexity and the planning of urban environments in the 21st century. Lancet 379 , 2079–2108 (2012).
Article Google Scholar
Mora, C. et al. Global risk of deadly heat. Nat. Clim. Chang . 7 , 501–506 (2017).
Zhao, L. et al. Interactions between urban heat islands and heat waves. Environ. Res. Lett . 13 , 034003 (2018).
Imhoff, M. L., Zhang, P., Wolfe, R. E. & Bounoua, L. Remote sensing of the urban heat island effect across biomes in the continental USA. Remote Sens. Environ . 114 , 504–513 (2010).
Zhou, B., Rybski, D. & Kropp, J. P. On the statistics of urban heat island intensity. Geophys. Res. Lett . 40 , 5486–5491 (2013).
Zhou, D., Zhang, L., Li, D., Huang, D. & Zhu, C. Climate–vegetation control on the diurnal and seasonal variations of surface urban heat islands in China. Environ. Res. Lett . 11 , 074009 (2016).
Liao, W. et al. Stronger contributions of urbanization to heat wave trends in wet climates. Geophys. Res. Lett . 45 , 11310–11317 (2018).
ADS Google Scholar
Peng, S. et al. Surface urban heat island across 419 global big cities. Environ. Sci. Technol . 46 , 696–703 (2012).
Clinton, N. & Gong, P. MODIS detected surface urban heat islands and sinks: global locations and controls. Remote Sens. Environ . 134 , 294–304 (2013).
Li, D. et al. Urban heat island: aerodynamics or imperviousness? Sci. Adv . 5 , eaau4299 (2019).
Bai, X. et al. Six research priorities for cities and climate change. Nature 555 , 23–25 (2018).
Gu, Y. & Li, D. A modeling study of the sensitivity of urban heat islands to precipitation at climate scales. Urban Clim . 24 , 982–993 (2018).
Schläpfer, M., Lee, J. & Bettencourt, L. Urban skylines: building heights and shapes as measures of city size. Preprint at https://arxiv.org/abs/1512.00946 (2015).
Grimmond, S. & Oke, T. R. Aerodynamic properties of urban areas derived from analysis of surface form. J. Appl. Meteorol . 38 , 1262–1292 (1999).
Gunawardena, K. R., Wells, M. J. & Kershaw, T. Utilising green and bluespace to mitigate urban heat island intensity. Sci. Total Environ . 584-585 , 1040–1055 (2017).
Eurostat. Urban Europe—Statistics on Cities, Towns and Suburbs https://doi.org/10.2785/91120 (Publications Office of the European Union, Luxembourg, 2016).
CIESIN. Global Urban Heat Island (UHI) Data Set, 2013 https://doi.org/10.7927/H4H70CRF (Center for International Earth Science Information Network, 2016).
Richards, D. R., Passy, P. & Oh, R. Impacts of population density and wealth on the quantity and structure of urban green space in tropical Southeast Asia. Landsc. Urban Plan . 157 , 553–560 (2017).
Bettencourt, L. M., Lobo, J., Helbing, D., Kühnert, C. & West, G. B. Growth, innovation, scaling, and the pace of life in cities. Proc. Natl Acad. Sci. USA 104 , 7301–7306 (2007).
Chrysoulakis, N. et al. Urban energy exchanges monitoring from space. Sci. Rep . 8 , 11498 (2018).
Sobstyl, J. M., Emig, T., Qomi, M. J. A., Ulm, F. J. & Pellenq, R. J. Role of city texture in urban heat islands at nighttime. Phys. Rev. Lett . 120 , 108701 (2018).
Gill, S. E., Handley, J. F., Ennos, A. R. & Pauleit, S. Adapting cities for climate change: the role of the green infrastructure. Built Environ . 33 , 115–133 (2007).
Scott, A. A., Waugh, D. W. & Zaitchik, B. F. Reduced urban heat island intensity under warmer conditions. Environ. Res. Lett . 13 , 064003 (2018).
Imamura, I. R. Role of soil moisture in the determination of urban heat island intensity in different climate regimes. WIT Trans. Ecol. Envir . 1 , 395–402 (1970).
Google Scholar
Lee, X. et al. Observed increase in local cooling effect of deforestation at higher latitudes. Nature 479 , 384–387 (2011).
Oke, T. R. The energetic basis of the urban heat island. Q. J. R. Meteorol. Soc . 108 , 1–24 (1982).
Shashua-Bar, L., Pearlmutter, D. & Erell, E. The cooling efficiency of urban landscape strategies in a hot dry climate. Landsc. Urban Plan . 92 , 179–186 (2009).
Kumar, R. et al. Dominant control of agriculture and irrigation on urban heat island in India. Sci. Rep . 7 , 14054 (2017).
Madani, N. et al. Future global productivity will be affected by plant trait response to climate. Sci. Rep . 8 , 2870 (2018).
Lim, Y. K., Cai, M., Kalnay, E. & Zhou, L. Observational evidence of sensitivity of surface climate changes to land types and urbanization. Geophys. Res. Lett . 32 , L22712 (2005).
Shastri, H., Barik, B., Ghosh, S., Venkataraman, C. & Sadavarte, P. Flip flop of day-night and summer-winter surface urban heat island intensity in India. Sci. Rep . 7 , 40178 (2017).
Juang, J. Y., Katul, G., Siqueira, M., Stoy, P. & Novick, K. Separating the effects of albedo from eco-physiological changes on surface temperature along a successional chronosequence in the southeastern United States. Geophys. Res. Lett . 34 , L21408 (2007).
Willis, K. J. & Petrokofsky, G. The natural capital of city trees. Science 356 , 374–376 (2017).
Manickathan, L., Defraeye, T., Allegrini, J., Derome, D. & Carmeliet, J. Parametric study of the influence of environmental factors and tree properties on the transpirative cooling effect of trees. Agric. For. Meteorol . 248 , 259–274 (2018).
Jendritzky, G., de Dear, R. & Havenith, G. UTCI—why another thermal index? Int. J. Biometeorol . 56 , 421–428 (2012).
Llaguno-Munitxa, M. & Bou-Zeid, E. Shaping buildings to promote street ventilation: a large-eddy simulation study. Urban Clim . 26 , 76–94 (2018).
Yang, J. & Bou-Zeid, E. Should cities embrace their heat islands as shields from extreme cold? J. Appl. Meteorol. Climatol . 57 , 1309–1320 (2018).
Seino, N., Aoyagi, T. & Tsuguti, H. Numerical simulation of urban impact on precipitation in Tokyo: how does urban temperature rise affect precipitation? Urban Clim . 23 , 8–35 (2018).
Endreny, T. A. Strategically growing the urban forest will improve our world. Nat. Commun . 9 , 1160 (2018).
Emmanuel, R., Rosenlund, H. & Johansson, E. Urban shading—a design option for the tropics? A study in Colombo, Sri Lanka. Int. J. Climatol . 27 , 1995–2004 (2007).
Wong, M. S., Nichol, J. E., To, P. H. & Wang, J. A simple method for designation of urban ventilation corridors and its application to urban heat island analysis. Build. Environ . 45 , 1880–1889 (2010).
Akbari, H., Menon, S. & Rosenfeld, A. Global cooling: increasing world-wide urban albedos to offset CO 2 . Clim. Change 94 , 275–286 (2009).
Georgescu, M., Morefield, P. E., Bierwagen, B. G. & Weaver, C. P. Urban adaptation can roll back warming of emerging megapolitan regions. Proc. Natl Acad. Sci. USA 111 , 2909–2914 (2014).
Estrada, F., Botzen, W. W. J. & Tol, R. S. J. A global economic assessment of city policies to reduce climate change impacts. Nat. Clim. Chang . 7 , 403–406 (2017).
Mayor of London. London Environment Strategy https://www.london.gov.uk/what-we-do/environment/london-environment-strategy (Mayor of London, 2018).
Bastin, J.-F. et al. Understanding climate change from a global analysis of city analogues. PloS One 14 , e0217592 (2019).
Bettencourt, L. M. & Lobo, J. Urban scaling in Europe. J. R. Soc. Interface 13 , 20160005 (2016).
Fuller, R. A. & Gaston, K. J. The scaling of green space coverage in European cities. Biol. Lett . 5 , 352–355 (2009).
Fang, Y. & Jawitz, J. W. High-resolution reconstruction of the United States human population distribution, 1790 to 2010. Sci. Data 5 , 180067 (2018).
Gasparrini, A. et al. Mortality risk attributable to high and low ambient temperature: a multicountry observational study. Lancet 386 , 369–375 (2015).
Clarke, J. F. Some effects of the urban structure on heat mortality. Environ. Res . 5 , 93–104 (1972).
Article CAS Google Scholar
Li, Y. et al. Evaluating biases in simulated land surface albedo from CMIP5 global climate models. J. Geophys. Res. Atmos . 121 , 6178–6190 (2016).
Chen, D., Loboda, T. V., He, T., Zhang, Y. & Liang, S. Strong cooling induced by stand-replacing fires through albedo in Siberian larch forests. Sci. Rep . 8 , 4821 (2018).
Oke, T. R. The urban energy balance. Prog. Phys. Geogr . 12 , 471–508 (1988).
Taha, H., Akbari, H., Rosenfeld, A. & Huang, J. Residential cooling loads and the urban heat island—the effects of albedo. Build. Environ . 23 , 271–283 (1988).
Akbari, H., Rosenfeld, A. & Taha, H. in Proc. American Society of Heating, Refrigeration, and Air-Conditioning Engineers, Lawrence Berkeley National Laboratory Report LBNL-28308 (Atlanta, Georgia, 1990)
Yang, X. & Li, Y. The impact of building density and building height heterogeneity on average urban albedo and street surface temperature. Build. Environ . 90 , 146–156 (2015).
Gelaro, R. et al. The modern-era retrospective analysis for research and applications, version 2 (MERRA–2). J. Clim . 30 , 5419–5454 (2017).
Schneider, U. et al. GPCC full data reanalysis version 7.0 at 0.5: monthly land-surface precipitation from rain-gauges built on GTS-based and historic data. https://doi.org/10.5676/DWD_GPCC/FD_M_V7_050 (Global Precipitation Climatology Centre, 2015).
Miguez-Macho, G. & Fan, Y. The role of groundwater in the Amazon water cycle: 2. Influence on seasonal soil moisture and evapotranspiration. J. Geophys. Res. Atmos . 117 , D15114 (2012).
Maxwell, R. M. & Condon, L. E. Connections between groundwater flow and transpiration partitioning. Science 353 , 377–380 (2016).
Huxman, T. E. et al. Convergence across biomes to a common rain-use efficiency. Nature 429 , 651–654 (2004).
Bettencourt, L. M. The origins of scaling in cities. Science 340 , 1438–1441 (2013).
Article ADS MathSciNet CAS Google Scholar
Shepherd, J. M. A review of current investigations of urban-induced rainfall and recommendations for the future. Earth Interact . 9 , 1–27 (2005).
Taleghani, M., Tenpierik, M., van den Dobbelsteen, A. & Sailor, D. J. Heat mitigation strategies in winter and summer: field measurements in temperate climates. Build. Environ . 81 , 309–319 (2014).
Download references
Acknowledgements
G.M. was supported by the The Branco Weiss Fellowship—Society in Science administered by ETH Zurich. E.B.-Z. acknowledges support by the US National Science Foundation under grant no. ICER 1664091, the SRN under cooperative agreement no. 1444758, and the Army Research Office under contract W911NF-15-1-0003 (program manager J. Barzyk). M.S. was supported by the Future Cities Laboratory at the Singapore-ETH Centre, which was established collaboratively between ETH Zurich and Singapore’s National Research Foundation (FI 370074016), under its Campus for Research Excellence and Technological Enterprise programme. We thank P. Edwards, J. Carmeliet, C. Küffer, and D. Richards for help and discussions at the beginning of this research.
Author information
Gabriele Manoli
Present address: Department of Civil, Environmental and Geomatic Engineering, University College London, London, UK
Authors and Affiliations
Institute of Environmental Engineering, ETH Zurich, Zurich, Switzerland
Gabriele Manoli, Simone Fatichi, Naika Meili & Paolo Burlando
Future Cities Laboratory, Singapore-ETH Centre, ETH Zurich, Singapore, Singapore
Markus Schläpfer & Naika Meili
Department of Environmental Systems Science, ETH Zurich, Zurich, Switzerland
Kailiang Yu & Thomas W. Crowther
Nicholas School of the Environment, Duke University, Durham, NC, USA
Gabriel G. Katul
Department of Civil and Environmental Engineering, Princeton University, Princeton, NJ, USA
Elie Bou-Zeid
You can also search for this author in PubMed Google Scholar
Contributions
G.M. designed the study, developed the model and conducted the analysis with contributions from S.F., G.G.K. and E.B.-Z. K.Y. and T.W.C. analysed albedo remote sensing observations. G.M. wrote the original draft of the manuscript with input from S.F., G.G.K. and E.B.-Z. M.S., K.Y., T.W.C., N.M. and P.B. reviewed and edited the manuscript. All authors discussed the results and contributed to the final version of the manuscript.
Corresponding author
Correspondence to Gabriele Manoli .
Ethics declarations
Competing interests.
The authors declare no competing interests.
Additional information
Publisher’s note: Springer Nature remains neutral with regard to jurisdictional claims in published maps and institutional affiliations.
Peer review information Nature thanks Lahouari Bounoua, Ben Crawford and Qihao Weng for their contribution to the peer review of this work.
Supplementary information
Supplementary information.
Supplementary Methods, Supplementary Tables 1–6, Supplementary Figs 1–25 and Supplementary References.
Source data
Source data fig. 1, source data fig. 2, source data fig. 3, rights and permissions.
Reprints and permissions
About this article
Cite this article.
Manoli, G., Fatichi, S., Schläpfer, M. et al. Magnitude of urban heat islands largely explained by climate and population. Nature 573 , 55–60 (2019). https://doi.org/10.1038/s41586-019-1512-9
Download citation
Received : 12 February 2019
Accepted : 24 June 2019
Published : 04 September 2019
Issue Date : 05 September 2019
DOI : https://doi.org/10.1038/s41586-019-1512-9
Share this article
Anyone you share the following link with will be able to read this content:
Sorry, a shareable link is not currently available for this article.
Provided by the Springer Nature SharedIt content-sharing initiative
This article is cited by
The evolution of social-ecological system interactions and their impact on the urban thermal environment.
- Fanhua Kong
npj Urban Sustainability (2024)
Street trees provide an opportunity to mitigate urban heat and reduce risk of high heat exposure
- Ailene K. Ettinger
- Gregory N. Bratman
- Lowell Wyse
Scientific Reports (2024)
- Shengjun Gao
- Yunhao Chen
npj Climate and Atmospheric Science (2024)
Unprivileged groups are less served by green cooling services in major European urban areas
- Alby Duarte Rocha
- Stenka Vulova
- Birgit Kleinschmit
Nature Cities (2024)
Exploring the spatial and seasonal heterogeneity of cooling effect of an urban river on a landscape scale
By submitting a comment you agree to abide by our Terms and Community Guidelines . If you find something abusive or that does not comply with our terms or guidelines please flag it as inappropriate.
Quick links
- Explore articles by subject
- Guide to authors
- Editorial policies
Sign up for the Nature Briefing newsletter — what matters in science, free to your inbox daily.

Information
- Author Services
Initiatives
You are accessing a machine-readable page. In order to be human-readable, please install an RSS reader.
All articles published by MDPI are made immediately available worldwide under an open access license. No special permission is required to reuse all or part of the article published by MDPI, including figures and tables. For articles published under an open access Creative Common CC BY license, any part of the article may be reused without permission provided that the original article is clearly cited. For more information, please refer to https://www.mdpi.com/openaccess .
Feature papers represent the most advanced research with significant potential for high impact in the field. A Feature Paper should be a substantial original Article that involves several techniques or approaches, provides an outlook for future research directions and describes possible research applications.
Feature papers are submitted upon individual invitation or recommendation by the scientific editors and must receive positive feedback from the reviewers.
Editor’s Choice articles are based on recommendations by the scientific editors of MDPI journals from around the world. Editors select a small number of articles recently published in the journal that they believe will be particularly interesting to readers, or important in the respective research area. The aim is to provide a snapshot of some of the most exciting work published in the various research areas of the journal.
Original Submission Date Received: .
- Active Journals
- Find a Journal
- Proceedings Series
- For Authors
- For Reviewers
- For Editors
- For Librarians
- For Publishers
- For Societies
- For Conference Organizers
- Open Access Policy
- Institutional Open Access Program
- Special Issues Guidelines
- Editorial Process
- Research and Publication Ethics
- Article Processing Charges
- Testimonials
- Preprints.org
- SciProfiles
- Encyclopedia
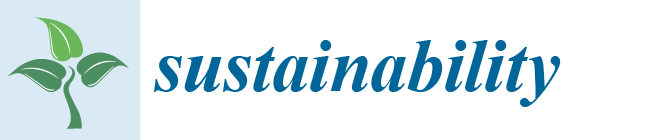
Article Menu
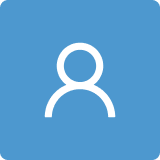
- Subscribe SciFeed
- Recommended Articles
- Google Scholar
- on Google Scholar
- Table of Contents
Find support for a specific problem in the support section of our website.
Please let us know what you think of our products and services.
Visit our dedicated information section to learn more about MDPI.
JSmol Viewer
Urban heat island and its interaction with heatwaves: a review of studies on mesoscale.
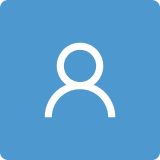
1. Introduction
- How does HW affect UHI?
- What are the main drivers controlling the synergies between UHI and HW?
- What can be done to mitigate UHI under HW conditions?
2. Current Status of Research
2.1. methods for retrieving uhi, 2.1.1. in situ measurement, 2.1.2. remote sensing, 2.1.3. numerical modeling, 2.2. classifications of urban heat island, 2.3. features, causes and definitions of heatwaves, 2.4. interactions between urban heat island and heatwaves, 2.4.1. urban heat island intensity, 2.4.2. energy balance models, 2.4.3. variation in energy budgets and corresponding drivers.
- UHII increases during HWs
- UHII decreases during HWs
- UHII remains unchanged during HWs
2.5. Mitigation of Urban Heat Island during Heatwaves
2.5.1. increasing albedo, 2.5.2. increasing vegetation coverage, 2.5.3. irrigation, 2.5.4. combined strategies, 3. discussion, 3.1. addressing the important questions, 3.2. research gaps and suggestions for future research.
- Time duration: Some studies, such as [ 25 , 108 ], only consider a short time period or even a single heatwave event, which may not be sufficiently representative of long-term behavior. However, the evolution of climate change is a long-term and complex process, and HWs are reported to become more intense and last for longer durations in the future [ 57 ]. Therefore, future studies exploring the synergies between UHI and HW and mitigation strategies should consider multi-year datasets or even future weather datasets.
- Study area: The synergistic effect between UHI and HW has been quantified mostly for metropolitan cities, but few studies have considered the different responses of urban and non-urban sites to HWs. Future studies should focus more on developing countries and the comparison between urban and rural sites. More detailed classifications such as low-intensity urban areas, high-intensity urban areas, forest, grassland and croplands, may be considered and compared. Moreover, although individual cities in different regions are worth investigating due to their unique geographies and climates [ 163 ], further studies may pay more attention to the comparisons among cities of different sizes or with different climates to reveal the general trend.
- Research method: In terms of numerical simulation, more complex UCMs such as MLUCMs may be preferred over the SLUCM for better prediction [ 25 , 84 , 164 , 165 ], but the latter is computationally inexpensive. In addition, high-resolution downscaling methods [ 166 ] may be considered in further studies. In terms of onsite observations, unified and dense weather stations can be established to improve spatial resolution. The data obtained through onsite measurements can be used to validate the numerical simulations. It is also advisable that a hybrid method incorporating both numerical simulations and onsite observations may be adopted alongside machine learning methods to construct accurate prediction models [ 167 ].
- Data collection: Some studies, such as [ 25 , 89 , 105 ], do not consider the anthropogenic heat flux or its variations during HWs. Future studies should include this as it plays an important role in the interactions between UHI and HW.
- UHI types: Despite a great deal of studies on UHIs during HWs, few studies have considered the differences in the thermal conditions, such as the temperature between urban and rural areas in the entire urban boundary layer. In addition, the relationships between the different UHI types also need to be investigated as studying the connections between air temperature and surface temperature may enable us to better understand the heat transfer in the atmospheric boundary layer and propose effective mitigation methods.
- Efficacy of mitigation strategies: When considering vegetation as a strategy, factors such as the efficiency of water use for different irrigation methods must be considered. Moreover, the choice of optimal vegetation type for different urban morphologies should be studied for cities in different climates. The sensitivity to soil moisture is worth investigating to provide more information about variations in latent heat flux. Mitigation by highly reflective surfaces may be effective in summer but may be undesirable in winter. The efficacy of these strategies for the mitigation of the HW effect and their impact in various seasons and in future climates should be investigated. More studies on the efficiency of combined mitigation strategies involving various physical mechanisms and processes should be conducted. In addition, studies on the efficiency of lightweight building materials may be conducted as they have a smaller heat capacity, which may reduce heat release at night. It is also worth noting that the efficacy of mitigation strategies may need to be assessed with reference to the ‘absolute’ urban thermal climate in addition to UHII due to the large diurnal variation in UHII and other factors influencing thermal sensation, as suggested by Martilli et al. [ 168 ].
- Health, economy and environmental considerations: Future work may consider other environmental and socio-economic impacts of the mitigation strategies, such as their impact on air quality, storm water, energy use, health, thermal comfort, visual comfort (albedo change), social aspects as wellbeing, costs and so on.
- Other considerations: A holistic approach considering changes in both climate and urbanization (horizontal and vertical spatial extent, building size, building material) may be adopted in future studies. Ramamurthy and Bou-Zeid [ 89 ] pointed out that the soil state and properties in urban areas are different from natural soils, which leads to variability in their thermal and hydrological characteristics. Accordingly, more research effort may be devoted to this topic.
4. Concluding Remark
Supplementary materials, author contributions, institutional review board statement, informed consent statement, data availability statement, acknowledgments, conflicts of interest.
- Yun, G.Y.; Ngarambe, J.; Duhirwe, P.N.; Ulpiani, G.; Paolini, R.; Haddad, S.; Vasilakopoulou, K.; Santamouris, M. Predicting the magnitude and the characteristics of the urban heat island in coastal cities in the proximity of desert landforms. The case of Sydney. Sci. Total. Environ. 2020 , 709 , 136068. [ Google Scholar ] [ CrossRef ]
- Kim, S.W.; Brown, R.D. Urban heat island (UHI) intensity and magnitude estimations: A systematic literature review. Sci. Total. Environ. 2021 , 779 , 146389. [ Google Scholar ] [ CrossRef ]
- He, B.-J.; Ding, L.; Prasad, D. Relationships among local-scale urban morphology, urban ventilation, urban heat island and outdoor thermal comfort under sea breeze influence. Sustain. Cities Soc. 2020 , 60 , 102289. [ Google Scholar ] [ CrossRef ]
- Santamouris, M. Recent progress on urban overheating and heat island research. Integrated assessment of the energy, environmental, vulnerability and health impact. Synergies with the global climate change. Energy Build. 2020 , 207 , 109482. [ Google Scholar ] [ CrossRef ]
- Steeneveld, G.J.; Koopmans, S.; Heusinkveld, B.G.; Theeuwes, N.E. Refreshing the role of open water surfaces on mitigating the maximum urban heat island effect. Landsc. Urban Plan. 2014 , 121 , 92–96. [ Google Scholar ] [ CrossRef ]
- Imran, H.M.; Kala, J.; Ng, A.W.M.; Muthukumaran, S. Effectiveness of green and cool roofs in mitigating urban heat island effects during a heatwave event in the city of Melbourne in southeast Australia. J. Clean. Prod. 2018 , 197 , 393–405. [ Google Scholar ] [ CrossRef ]
- Li, Y.; Schubert, S.; Kropp, J.P.; Rybski, D. On the influence of density and morphology on the Urban Heat Island intensity. Nat. Commun. 2020 , 11 , 1–9. [ Google Scholar ] [ CrossRef ] [ PubMed ]
- Jamei, Y.; Rajagopalan, P.; Sun, Q.C. Spatial structure of surface urban heat island and its relationship with vegetation and built-up areas in Melbourne, Australia. Sci. Total. Environ. 2019 , 659 , 1335–1351. [ Google Scholar ] [ CrossRef ] [ PubMed ]
- Gao, K.; Santamouris, M.; Feng, J. On the cooling potential of irrigation to mitigate urban heat island. Sci. Total. Environ. 2020 , 740 , 139754. [ Google Scholar ] [ CrossRef ]
- Zhao, L.; Lee, X.; Smith, R.B.; Oleson, K. Strong contributions of local background climate to urban heat islands. Nature 2014 , 511 , 216–219. [ Google Scholar ] [ CrossRef ]
- Manoli, G.; Fatichi, S.; Schläpfer, M.; Yu, K.; Crowther, T.W.; Meili, N.; Burlando, P.; Katul, G.G.; Bou-Zeid, E. Magnitude of urban heat islands largely explained by climate and population. Nature 2019 , 573 , 55–60. [ Google Scholar ] [ CrossRef ]
- Li, D.; Liao, W.; Rigden, A.J.; Liu, X.; Wang, D.; Malyshev, S.; Shevliakova, E. Urban heat island: Aerodynamics or imperviousness? Sci. Adv. 2019 , 5 , eaau4299. [ Google Scholar ] [ CrossRef ] [ Green Version ]
- Zinzi, M.; Agnoli, S.; Burattini, C.; Mattoni, B. On the thermal response of buildings under the synergic effect of heat waves and urban heat island. Sol. Energy 2020 , 211 , 1270–1282. [ Google Scholar ] [ CrossRef ]
- Santamouris, M.; Haddad, S.; Saliari, M.; Vasilakopoulou, K.; Synnefa, A.; Paolini, R.; Ulpiani, G.; Garshasbi, S.; Fiorito, F. On the energy impact of urban heat island in Sydney: Climate and energy potential of mitigation technologies. Energy Build. 2018 , 166 , 154–164. [ Google Scholar ] [ CrossRef ]
- Santamouris, M. On the energy impact of urban heat island and global warming on buildings. Energy Build. 2014 , 82 , 100–113. [ Google Scholar ] [ CrossRef ]
- Santamouris, M.; Papanikolaou, N.; Livada, I.; Koronakis, I.; Georgakis, C.; Argiriou, A.; Assimakopoulos, D. On the impact of urban climate on the energy consumption of buildings. Sol. Energy 2001 , 70 , 201–216. [ Google Scholar ] [ CrossRef ]
- Baccini, M.; Biggeri, A.; Accetta, G.; Kosatsky, T.; Katsouyanni, K.; Analitis, A.; Anderson, H.R.; Bisanti, L.; D’Ippoliti, D.; Danova, J. Heat effects on mortality in 15 European cities. Epidemiology 2008 , 19 , 711–719. [ Google Scholar ] [ CrossRef ]
- Sarkar, C.; Webster, C. Urban environments and human health: Current trends and future directions. Curr. Opin. Environ. Sustain. 2017 , 25 , 33–44. [ Google Scholar ] [ CrossRef ]
- Katavoutas, G.; Founda, D. Response of Urban Heat Stress to Heat Waves in Athens (1960–2017). Atmosphere 2019 , 10 , 483. [ Google Scholar ] [ CrossRef ] [ Green Version ]
- Vicedo-Cabrera, A.M.; Scovronick, N.; Sera, F.; Royé, D.; Schneider, R.; Tobias, A.; Astrom, C.; Guo, Y.; Honda, Y.; Hondula, D. The burden of heat-related mortality attributable to recent human-induced climate change. Nat. Clim. Chang. 2021 , 11 , 492–500. [ Google Scholar ] [ CrossRef ]
- O’Neill, M.S.; Ebi, K.L. Temperature extremes and health: Impacts of climate variability and change in the United States. J. Occup. Environ. Med. 2009 , 51 , 13–25. [ Google Scholar ] [ CrossRef ]
- Victorian Auditor-General’s Report: Heatwave management: Reducing the Risk to Public Health. Available online: https://www.audit.vic.gov.au/sites/default/files/2017-07/20141014-Heatwave-Management.pdf (accessed on 22 September 2021).
- Whitman, S.; Good, G.; Donoghue, E.R.; Benbow, N.; Shou, W.; Mou, S. Mortality in Chicago attributed to the July 1995 heat wave. Am. J. Public Health 1997 , 87 , 1515–1518. [ Google Scholar ] [ CrossRef ] [ Green Version ]
- Zhong, S.; Qian, Y.; Zhao, C.; Leung, R.; Wang, H.; Yang, B.; Fan, J.; Yan, H.; Yang, X.-Q.; Liu, D. Urbanization-induced urban heat island and aerosol effects on climate extremes in the Yangtze River Delta region of China. Atmos. Chem. Phys. 2017 , 17 , 5439–5457. [ Google Scholar ] [ CrossRef ] [ Green Version ]
- Chew, L.W.; Liu, X.; Li, X.-X.; Norford, L.K. Interaction between heat wave and urban heat island: A case study in a tropical coastal city, Singapore. Atmos. Res. 2021 , 247 , 105134. [ Google Scholar ] [ CrossRef ]
- Lhotka, O.; Kyselý, J.; Farda, A. Climate change scenarios of heat waves in Central Europe and their uncertainties. Theor. Appl. Climatol. 2018 , 131 , 1043–1054. [ Google Scholar ] [ CrossRef ]
- Li, D.; Sun, T.; Liu, M.; Wang, L.; Gao, Z. Changes in Wind Speed under Heat Waves Enhance Urban Heat Islands in the Beijing Metropolitan Area. J. Appl. Meteorol. Climatol. 2016 , 55 , 2369–2375. [ Google Scholar ] [ CrossRef ]
- Li, D.; Sun, T.; Liu, M.; Yang, L.; Wang, L.; Gao, Z. Contrasting responses of urban and rural surface energy budgets to heat waves explain synergies between urban heat islands and heat waves. Environ. Res. Lett. 2015 , 10 , 054009. [ Google Scholar ] [ CrossRef ]
- An, N.; Dou, J.; González-Cruz, J.E.; Bornstein, R.D.; Miao, S.; Li, L. An Observational Case Study of Synergies between an Intense Heat Wave and the Urban Heat Island in Beijing. J. Appl. Meteorol. Climatol. 2020 , 59 , 605–620. [ Google Scholar ] [ CrossRef ]
- Oliveira, A.; Lopes, A.; Correia, E.; Niza, S.; Soares, A. Heatwaves and Summer Urban Heat Islands: A Daily Cycle Approach to Unveil the Urban Thermal Signal Changes in Lisbon, Portugal. Atmosphere 2021 , 12 , 292. [ Google Scholar ] [ CrossRef ]
- Ferrari, A.; Kubilay, A.; Derome, D.; Carmeliet, J. The use of permeable and reflective pavements as a potential strategy for urban heat island mitigation. Urban Clim. 2020 , 31 , 100534. [ Google Scholar ] [ CrossRef ]
- Kubilay, A.; Allegrini, J.; Strebel, D.; Zhao, Y.; Derome, D.; Carmeliet, J. Advancement in Urban Climate Modelling at Local Scale: Urban Heat Island Mitigation and Building Cooling Demand. Atmosphere 2020 , 11 , 1313. [ Google Scholar ] [ CrossRef ]
- Kubilay, A.; Ferrari, A.; Derome, D.; Carmeliet, J. Smart wetting of permeable pavements as an evaporative-cooling measure for improving the urban climate during heat waves. J. Build. Phys. 2020 , 45 , 36–66. [ Google Scholar ] [ CrossRef ]
- Sun, T.; Kotthaus, S.; Li, D.; Ward, H.C.; Gao, Z.; Ni, G.-H.; Grimmond, C.S.B. Attribution and mitigation of heat wave-induced urban heat storage change. Environ. Res. Lett. 2017 , 12 , 114007. [ Google Scholar ] [ CrossRef ] [ Green Version ]
- Rossi, F.; Castellani, B.; Presciutti, A.; Morini, E.; Filipponi, M.; Nicolini, A.; Santamouris, M. Retroreflective façades for urban heat island mitigation: Experimental investigation and energy evaluations. Appl. Energy 2015 , 145 , 8–20. [ Google Scholar ] [ CrossRef ]
- Onishi, A.; Cao, X.; Ito, T.; Shi, F.; Imura, H. Evaluating the potential for urban heat-island mitigation by greening parking lots. Urban For. Urban Green. 2010 , 9 , 323–332. [ Google Scholar ] [ CrossRef ]
- Mussetti, G.; Brunner, D.; Henne, S.; Allegrini, J.; Krayenhoff, E.S.; Schubert, S.; Feigenwinter, C.; Vogt, R.; Wicki, A.; Carmeliet, J. COSMO-BEP-Tree v1. 0: A coupled urban climate model with explicit representation of street trees. Geosci. Model Dev. 2020 , 13 , 1685–1710. [ Google Scholar ] [ CrossRef ] [ Green Version ]
- Chen, T.; Yang, H.; Chen, G.; Lam, C.K.C.; Hantg, J.; Wang, X.; Liu, Y.; Ling, H. Integrated impacts of tree planting and aspect ratios on thermal environment in street canyons by scaled outdoor experiments. Sci. Total. Environ. 2021 , 764 , 142920. [ Google Scholar ] [ CrossRef ]
- Ng, E.; Chen, L.; Wang, Y.; Yuan, C. A study on the cooling effects of greening in a high-density city: An experience from Hong Kong. Build. Environ. 2012 , 47 , 256–271. [ Google Scholar ] [ CrossRef ]
- Wang, Y.; Akbari, H. Analysis of urban heat island phenomenon and mitigation solutions evaluation for Montreal. Sustain. Cities Soc. 2016 , 26 , 438–446. [ Google Scholar ] [ CrossRef ]
- Wang, Y.; Akbari, H. The effects of street tree planting on Urban Heat Island mitigation in Montreal. Sustain. Cities Soc. 2016 , 27 , 122–128. [ Google Scholar ] [ CrossRef ]
- Solecki, W.D.; Rosenzweig, C.; Parshall, L.; Pope, G.; Clark, M.; Cox, J.; Wiencke, M. Mitigation of the heat island effect in urban New Jersey. Glob. Environ. Chang. Part B Environ. Hazards 2005 , 6 , 39–49. [ Google Scholar ] [ CrossRef ]
- Susca, T.; Gaffin, S.R.; Dell’Osso, G. Positive effects of vegetation: Urban heat island and green roofs. Environ. Pollut. 2011 , 159 , 2119–2126. [ Google Scholar ] [ CrossRef ]
- Bahi, H.; Mastouri, H.; Radoine, H. Review of methods for retrieving urban heat islands. Mater. Today Proc. 2020 , 27 , 3004–3009. [ Google Scholar ] [ CrossRef ]
- Ngarambe, J.; Nganyiyimana, J.; Kim, I.; Santamouris, M.; Yun, G.Y. Synergies between urban heat island and heat waves in Seoul: The role of wind speed and land use characteristics. PLoS ONE 2020 , 15 , e0243571. [ Google Scholar ] [ CrossRef ]
- Jiang, S.; Lee, X.; Wang, J.; Wang, K. Amplified Urban Heat Islands during Heat Wave Periods. J. Geophys. Res. Atmos. 2019 , 124 , 7797–7812. [ Google Scholar ] [ CrossRef ] [ Green Version ]
- De Ridder, K.; Maiheu, B.; Lauwaet, D.; Daglis, I.; Keramitsoglou, I.; Kourtidis, K.; Manunta, P.; Paganini, M. Urban Heat Island Intensification during Hot Spells—The Case of Paris during the Summer of 2003. Urban Sci. 2017 , 1 , 3. [ Google Scholar ] [ CrossRef ] [ Green Version ]
- Richard, Y.; Pohl, B.; Rega, M.; Pergaud, J.; Thevenin, T.; Emery, J.; Dudek, J.; Vairet, T.; Zito, S.; Chateau-Smith, C. Is Urban Heat Island intensity higher during hot spells and heat waves (Dijon, France, 2014–2019)? Urban Clim. 2021 , 35 , 100747. [ Google Scholar ] [ CrossRef ]
- Scott, A.A.; Waugh, D.W.; Zaitchik, B.F. Reduced Urban Heat Island intensity under warmer conditions. Environ. Res. Lett. 2018 , 13 , 064003. [ Google Scholar ] [ CrossRef ]
- Stewart, I.D. A systematic review and scientific critique of methodology in modern urban heat island literature. Int. J. Climatol. 2011 , 31 , 200–217. [ Google Scholar ] [ CrossRef ]
- Keramitsoglou, I.; Daglis, I.A.; Amiridis, V.; Chrysoulakis, N.; Ceriola, G.; Manunta, P.; Maiheu, B.; De Ridder, K.; Lauwaet, D.; Paganini, M. Evaluation of satellite-derived products for the characterization of the urban thermal environment. J. Appl. Remote Sens. 2012 , 6 , 061704. [ Google Scholar ] [ CrossRef ]
- Holderness, T.; Barr, S.; Dawson, R.; Hall, J. An evaluation of thermal Earth observation for characterizing urban heatwave event dynamics using the urban heat island intensity metric. Int. J. Remote Sens. 2013 , 34 , 864–884. [ Google Scholar ] [ CrossRef ] [ Green Version ]
- Ramamurthy, P.; Gonzalez, J.; Ortiz, L.; Arend, M.; Moshary, F. Impact of heatwave on a megacity: An observational analysis of New York City during July 2016. Environ. Res. Lett. 2017 , 12 , 054011. [ Google Scholar ] [ CrossRef ]
- Tomlinson, C.J.; Chapman, L.; Thornes, J.E.; Baker, C.J. Derivation of Birmingham’s summer surface urban heat island from MODIS satellite images. Int. J. Climatol. 2012 , 32 , 214–224. [ Google Scholar ] [ CrossRef ] [ Green Version ]
- Wu, C.-D.; Lung, S.-C.C.; Jan, J.-F. Development of a 3-D urbanization index using digital terrain models for surface urban heat island effects. ISPRS J. Photogramm. Remote Sens. 2013 , 81 , 1–11. [ Google Scholar ] [ CrossRef ]
- Kumar, R.; Mishra, V. Decline in surface urban heat island intensity in India during heatwaves. Environ. Res. Commun. 2019 , 1 , 031001. [ Google Scholar ] [ CrossRef ] [ Green Version ]
- Ward, K.; Lauf, S.; Kleinschmit, B.; Endlicher, W. Heat waves and urban heat islands in Europe: A review of relevant drivers. Sci. Total. Environ. 2016 , 569 , 527–539. [ Google Scholar ] [ CrossRef ]
- Herbel, I.; Croitoru, A.-E.; Rus, A.V.; Roşca, C.F.; Harpa, G.V.; Ciupertea, A.-F.; Rus, I. The impact of heat waves on surface urban heat island and local economy in Cluj-Napoca city, Romania. Theor. Appl. Climatol. 2018 , 133 , 681–695. [ Google Scholar ] [ CrossRef ]
- Rasilla, D.; Allende, F.; Martilli, A.; Fernández, F. Heat Waves and Human Well-Being in Madrid (Spain). Atmosphere 2019 , 10 , 288. [ Google Scholar ] [ CrossRef ] [ Green Version ]
- Skamarock, W.C.; Klemp, J.B.; Dudhia, J.; Gill, D.O.; Liu, Z.; Berner, J.; Wang, W.; Powers, J.G.; Duda, M.G.; Barker, D.M. A Description of the Advanced Research WRF Model Version 4 ; National Center for Atmospheric Research: Boulder, CO, USA, 2019; p. 145. [ Google Scholar ]
- Grell, G.A.; Peckham, S.E.; Schmitz, R.; McKeen, S.A.; Frost, G.; Skamarock, W.C.; Eder, B. Fully coupled “online” chemistry within the WRF model. Atmos. Environ. 2005 , 39 , 6957–6975. [ Google Scholar ] [ CrossRef ]
- Schättler, G.; Doms, G.; Schraff, C. A Description of the Nonhydrostatic Regional COSMO-Model Part VII: User’s Guide ; Deutscher Wetterdienst: Offenbach, Germany, 2016. [ Google Scholar ]
- Gerard, L.; Piriou, J.-M.; Brožková, R.; Geleyn, J.-F.; Banciu, D. Cloud and Precipitation Parameterization in a Meso-Gamma-Scale Operational Weather Prediction Model. Mon. Weather Rev. 2009 , 137 , 3960–3977. [ Google Scholar ] [ CrossRef ]
- De Ridder, K.; Lauwaet, D.; Maiheu, B. UrbClim—A fast urban boundary layer climate model. Urban Clim. 2015 , 12 , 21–48. [ Google Scholar ] [ CrossRef ] [ Green Version ]
- Lafore, J.P.; Stein, J.; Asencio, N.; Bougeault, P.; Ducrocq, V.; Duron, J.; Fischer, C.; Héreil, P.; Mascart, P.; Masson, V. The Meso-NH atmospheric simulation system. Part I: Adiabatic formulation and control simulations. Ann. Geophys. 1997 , 16 , 90–109. [ Google Scholar ] [ CrossRef ]
- Lac, C.; Chaboureau, J.-P.; Masson, V.; Pinty, J.-P.; Tulet, P.; Escobar, J.; Leriche, M.; Barthe, C.; Aouizerats, B.; Augros, C.; et al. Overview of the Meso-NH model version 5.4 and its applications. Geosci. Model Dev. 2018 , 11 , 1929–1969. [ Google Scholar ] [ CrossRef ] [ Green Version ]
- Sievers, U. Das kleinskalige Strömungsmodell MUKLIMO_3. Teil 1: Theoretische Grundlagen, PC-Basisversion und Validierung ; Deutschen Wetterdienstes: Offenbach am Maine, Germany, 2012. [ Google Scholar ]
- Sievers, U. Das kleinskalige Strömungsmodell MUKLIMO_3. Teil 2: Thermodynamische Erweiterungen ; Deutschen Wetterdienstes: Offenbach am Maine, Germany, 2016. [ Google Scholar ]
- Hurrell, J.W.; Holland, M.M.; Gent, P.R.; Ghan, S.; Kay, J.E.; Kushner, P.J.; Lamarque, J.-F.; Large, W.G.; Lawrence, D.; Lindsay, K. The community earth system model: A framework for collaborative research. Bull. Am. Meteorol. Soc. 2013 , 94 , 1339–1360. [ Google Scholar ] [ CrossRef ]
- Geletič, J.; Lehnert, M.; Jurek, M. Spatiotemporal variability of air temperature during a heat wave in real and modified landcover conditions: Prague and Brno (Czech Republic). Urban Clim. 2020 , 31 , 100588. [ Google Scholar ] [ CrossRef ]
- Kwok, Y.T.; Ng, E.Y.Y. Trends, topics, and lessons learnt from real case studies using mesoscale atmospheric models for urban climate applications in 2000–2019. Urban Clim. 2021 , 36 , 100785. [ Google Scholar ] [ CrossRef ]
- Luo, X.; Vahmani, P.; Hong, T.; Jones, A. City-Scale Building Anthropogenic Heating during Heat Waves. Atmosphere 2020 , 11 , 1206. [ Google Scholar ] [ CrossRef ]
- He, X.; Wang, J.; Feng, J.; Yan, Z.; Miao, S.; Zhang, Y.; Xia, J. Observational and modeling study of interactions between urban heat island and heatwave in Beijing. J. Clean. Prod. 2020 , 247 , 119169. [ Google Scholar ] [ CrossRef ]
- Gao, Z.; Hou, Y.; Chen, W. Enhanced sensitivity of the urban heat island effect to summer temperatures induced by urban expansion. Environ. Res. Lett. 2019 , 14 , 094005. [ Google Scholar ] [ CrossRef ] [ Green Version ]
- Pokhrel, R.; Ramírez-Beltran, N.D.; González, J.E. On the assessment of alternatives for building cooling load reductions for a tropical coastal city. Energy Build. 2019 , 182 , 131–143. [ Google Scholar ] [ CrossRef ]
- Chen, F.; Dudhia, J. Coupling an advanced land surface–hydrology model with the Penn State–NCAR MM5 modeling system. Part I: Model implementation and sensitivity. Mon. Weather Rev. 2001 , 129 , 569–585. [ Google Scholar ] [ CrossRef ] [ Green Version ]
- Giannaros, C.; Nenes, A.; Giannaros, T.M.; Kourtidis, K.; Melas, D. A comprehensive approach for the simulation of the Urban Heat Island effect with the WRF/SLUCM modeling system: The case of Athens (Greece). Atmos. Res. 2018 , 201 , 86–101. [ Google Scholar ] [ CrossRef ]
- Cady, T.J.; Rahn, D.A.; Brunsell, N.A.; Lyles, W. Conversion of Abandoned Property to Green Space as a Strategy to Mitigate the Urban Heat Island Investigated with Numerical Simulations. J. Appl. Meteorol. Climatol. 2020 , 59 , 1827–1843. [ Google Scholar ] [ CrossRef ]
- Kusaka, H.; Kondo, H.; Kikegawa, Y.; Kimura, F. A simple single-layer urban canopy model for atmospheric models: Comparison with multi-layer and slab models. Bound.-Layer Meteorol. 2001 , 101 , 329–358. [ Google Scholar ] [ CrossRef ]
- Martilli, A.; Clappier, A.; Rotach, M.W. An urban surface exchange parameterisation for mesoscale models. Bound.-Layer Meteorol. 2002 , 104 , 261–304. [ Google Scholar ] [ CrossRef ]
- Heaviside, C.; Cai, X.M.; Vardoulakis, S. The effects of horizontal advection on the urban heat island in Birmingham and the West Midlands, United Kingdom during a heatwave. Q. J. R. Meteorol. Soc. 2015 , 141 , 1429–1441. [ Google Scholar ] [ CrossRef ] [ Green Version ]
- Fallmann, J.; Forkel, R.; Emeis, S. Secondary effects of urban heat island mitigation measures on air quality. Atmos. Environ. 2016 , 125 , 199–211. [ Google Scholar ] [ CrossRef ] [ Green Version ]
- Salamanca, F.; Krpo, A.; Martilli, A.; Clappier, A. A new building energy model coupled with an urban canopy parameterization for urban climate simulations—part I. formulation, verification, and sensitivity analysis of the model. Theor. Appl. Climatol. 2010 , 99 , 331–344. [ Google Scholar ] [ CrossRef ]
- Jandaghian, Z.; Berardi, U. Analysis of the cooling effects of higher albedo surfaces during heat waves coupling the Weather Research and Forecasting model with building energy models. Energy Build. 2020 , 207 , 109627. [ Google Scholar ] [ CrossRef ]
- Morini, E.; Touchaei, A.; Castellani, B.; Rossi, F.; Cotana, F. The Impact of Albedo Increase to Mitigate the Urban Heat Island in Terni (Italy) Using the WRF Model. Sustainability 2016 , 8 , 999. [ Google Scholar ] [ CrossRef ] [ Green Version ]
- Gutiérrez, E.; González, J.E.; Bornstein, R.; Arend, M.; Martilli, A. A New Modeling Approach to Forecast Building Energy Demands During Extreme Heat Events in Complex Cities. J. Sol. Energy Eng. 2013 , 040906. [ Google Scholar ] [ CrossRef ]
- Salamanca, F.; Martilli, A. A new Building Energy Model coupled with an Urban Canopy Parameterization for urban climate simulations—part II. Validation with one dimension off-line simulations. Theor. Appl. Climatol. 2010 , 99 , 345–356. [ Google Scholar ] [ CrossRef ]
- Wang, Z.-H.; Bou-Zeid, E.; Smith, J.A. A coupled energy transport and hydrological model for urban canopies evaluated using a wireless sensor network. Q. J. R. Meteorol. Soc. 2013 , 139 , 1643–1657. [ Google Scholar ] [ CrossRef ]
- Ramamurthy, P.; Bou-Zeid, E. Heatwaves and urban heat islands: A comparative analysis of multiple cities. J. Geophys. Res. Atmos. 2017 , 122 , 168–178. [ Google Scholar ] [ CrossRef ]
- Ramamurthy, P.; Li, D.; Bou-Zeid, E. High-resolution simulation of heatwave events in New York City. Theor. Appl. Climatol. 2017 , 128 , 89–102. [ Google Scholar ] [ CrossRef ]
- Li, D.; Bou-Zeid, E.; Oppenheimer, M. The effectiveness of cool and green roofs as urban heat island mitigation strategies. Environ. Res. Lett. 2014 , 9 , 055002. [ Google Scholar ] [ CrossRef ]
- Masson, V. A physically-based scheme for the urban energy budget in atmospheric models. Bound.-Layer Meteorol. 2000 , 94 , 357–397. [ Google Scholar ] [ CrossRef ]
- Daniel, M.; Lemonsu, A.; Viguié, V. Role of watering practices in large-scale urban planning strategies to face the heat-wave risk in future climate. Urban Clim. 2018 , 23 , 287–308. [ Google Scholar ] [ CrossRef ]
- Masson, V.; Lion, Y.; Peter, A.; Pigeon, G.; Buyck, J.; Brun, E. “Grand Paris”: Regional landscape change to adapt city to climate warming. Clim. Chang. 2013 , 117 , 769–782. [ Google Scholar ] [ CrossRef ] [ Green Version ]
- Noilhan, J.; Mahfouf, J.-F. The ISBA land surface parameterisation scheme. Glob. Planet. Chang. 1996 , 13 , 145–159. [ Google Scholar ] [ CrossRef ]
- Broadbent, A.M.; Krayenhoff, E.S.; Georgescu, M. Efficacy of cool roofs at reducing pedestrian-level air temperature during projected 21st century heatwaves in Atlanta, Detroit, and Phoenix (USA). Environ. Res. Lett. 2020 , 15 , 084007. [ Google Scholar ] [ CrossRef ]
- Wang, X.; Liu, H.; Miao, S.; Wu, Q.; Zhang, N.; Qiao, F. Effectiveness of urban hydrological processes in mitigating urban heat island and human thermal stress during a heat wave event in Nanjing, China. J. Geophys. Res. Atmos. 2020 , 125 , e2020JD033275. [ Google Scholar ] [ CrossRef ]
- Macintyre, H.L.; Heaviside, C. Potential benefits of cool roofs in reducing heat-related mortality during heatwaves in a European city. Environ. Int. 2019 , 127 , 430–441. [ Google Scholar ] [ CrossRef ]
- Tewari, M.; Yang, J.; Kusaka, H.; Salamanca, F.; Watson, C.; Treinish, L. Interaction of urban heat islands and heat waves under current and future climate conditions and their mitigation using green and cool roofs in New York City and Phoenix, Arizona. Environ. Res. Lett. 2019 , 14 , 034002. [ Google Scholar ] [ CrossRef ]
- Falasca, S.; Ciancio, V.; Salata, F.; Golasi, I.; Rosso, F.; Curci, G. High albedo materials to counteract heat waves in cities: An assessment of meteorology, buildings energy needs and pedestrian thermal comfort. Build. Environ. 2019 , 163 , 106242. [ Google Scholar ] [ CrossRef ]
- Imran, H.M.; Kala, J.; Ng, A.W.M.; Muthukumaran, S. Effectiveness of vegetated patches as Green Infrastructure in mitigating Urban Heat Island effects during a heatwave event in the city of Melbourne. Weather Clim. Extrem. 2019 , 25 , 100217. [ Google Scholar ] [ CrossRef ]
- Chen, Y.; Zhang, N. Urban heat island mitigation effectiveness under extreme heat conditions in the Suzhou–Wuxi–Changzhou metropolitan area, China. J. Appl. Meteorol. Climatol. 2018 , 57 , 235–253. [ Google Scholar ] [ CrossRef ]
- Ortiz, L.E.; Gonzalez, J.E.; Wu, W.; Schoonen, M.; Tongue, J.; Bornstein, R. New York City Impacts on a Regional Heat Wave. J. Appl. Meteorol. Climatol. 2018 , 57 , 837–851. [ Google Scholar ] [ CrossRef ]
- Sun, T.; Grimmond, C.S.B.; Ni, G.-H. How do green roofs mitigate urban thermal stress under heat waves? J. Geophys. Res. Atmos. 2016 , 121 , 5320–5335. [ Google Scholar ] [ CrossRef ]
- Zhou, Y.; Shepherd, J.M. Atlanta’s urban heat island under extreme heat conditions and potential mitigation strategies. Nat. Hazards 2010 , 52 , 639–668. [ Google Scholar ] [ CrossRef ]
- Ma, H.; Shao, H.; Song, J. Modeling the relative roles of the foehn wind and urban expansion in the 2002 Beijing heat wave and possible mitigation by high reflective roofs. Meteorol. Atmos. Phys. 2014 , 123 , 105–114. [ Google Scholar ] [ CrossRef ]
- Wang, M.; Yan, X.; Liu, J.; Zhang, X. The contribution of urbanization to recent extreme heat events and a potential mitigation strategy in the Beijing–Tianjin–Hebei metropolitan area. Theor. Appl. Climatol. 2013 , 114 , 407–416. [ Google Scholar ] [ CrossRef ]
- Li, D.; Bou-Zeid, E. Synergistic Interactions between Urban Heat Islands and Heat Waves: The Impact in Cities Is Larger than the Sum of Its Parts. J. Appl. Meteorol. Climatol. 2013 , 52 , 2051–2064. [ Google Scholar ] [ CrossRef ] [ Green Version ]
- Jandaghian, Z.; Akbari, H. The Effect of Increasing Surface Albedo on Urban Climate and Air Quality: A Detailed Study for Sacramento, Houston, and Chicago. Climate 2018 , 6 , 19. [ Google Scholar ] [ CrossRef ] [ Green Version ]
- Chen, L.; Zhang, M.; Zhu, J.; Wang, Y.; Skorokhod, A. Modeling Impacts of Urbanization and Urban Heat Island Mitigation on Boundary Layer Meteorology and Air Quality in Beijing Under Different Weather Conditions. J. Geophys. Res. Atmos. 2018 , 123 , 4323–4344. [ Google Scholar ] [ CrossRef ]
- Touchaei, A.G.; Akbari, H.; Tessum, C.W. Effect of increasing urban albedo on meteorology and air quality of Montreal (Canada)—Episodic simulation of heat wave in 2005. Atmos. Environ. 2016 , 132 , 188–206. [ Google Scholar ] [ CrossRef ]
- Hamdi, R.; Duchêne, F.; Berckmans, J.; Delcloo, A.; Vanpoucke, C.; Termonia, P. Evolution of urban heat wave intensity for the Brussels Capital Region in the ARPEGE-Climat A1B scenario. Urban Clim. 2016 , 17 , 176–195. [ Google Scholar ] [ CrossRef ]
- Zhao, L.; Oppenheimer, M.; Zhu, Q.; Baldwin, J.W.; Ebi, K.L.; Bou-Zeid, E.; Guan, K.; Liu, X. Interactions between urban heat islands and heat waves. Environ. Res. Lett. 2018 , 13 , 034003. [ Google Scholar ] [ CrossRef ]
- Oke, T.R. Urban Climates ; Cambridge University Press: Cambridge, UK, 2017. [ Google Scholar ]
- Ao, X.; Wang, L.; Zhi, X.; Gu, W.; Yang, H.; Li, D. Observed Synergies between Urban Heat Islands and Heat Waves and Their Controlling Factors in Shanghai, China. J. Appl. Meteorol. Climatol. 2019 , 58 , 1955–1972. [ Google Scholar ] [ CrossRef ] [ Green Version ]
- Perkins, S.E. A review on the scientific understanding of heatwaves—Their measurement, driving mechanisms, and changes at the global scale. Atmos. Res. 2015 , 164–165 , 242–267. [ Google Scholar ] [ CrossRef ]
- Unger, J.; Skarbit, N.; Kovács, A.; Gál, T. Comparison of regional and urban outdoor thermal stress conditions in heatwave and normal summer periods: A case study. Urban Clim. 2020 , 32 , 100619. [ Google Scholar ] [ CrossRef ]
- Hong, J.W.; Hong, J.; Kwon, E.E.; Yoon, D.K. Temporal dynamics of urban heat island correlated with the socio-economic development over the past half-century in Seoul, Korea. Environ. Pollut. 2019 , 254 , 112934. [ Google Scholar ] [ CrossRef ] [ PubMed ]
- Pyrgou, A.; Hadjinicolaou, P.; Santamouris, M. Urban-rural moisture contrast: Regulator of the urban heat island and heatwaves’ synergy over a mediterranean city. Environ. Res. 2020 , 182 , 109102. [ Google Scholar ] [ CrossRef ] [ PubMed ]
- Xu, Z.; FitzGerald, G.; Guo, Y.; Jalaludin, B.; Tong, S. Impact of heatwave on mortality under different heatwave definitions: A systematic review and meta-analysis. Environ. Int. 2016 , 89–90 , 193–203. [ Google Scholar ] [ CrossRef ]
- Steadman, R.G. A universal scale of apparent temperature. J. Appl. Meteorol. Climatol. 1984 , 23 , 1674–1687. [ Google Scholar ] [ CrossRef ]
- Founda, D.; Pierros, F.; Petrakis, M.; Zerefos, C. Interdecadal variations and trends of the Urban Heat Island in Athens (Greece) and its response to heat waves. Atmos. Res. 2015 , 161–162 , 1–13. [ Google Scholar ] [ CrossRef ]
- Founda, D.; Santamouris, M. Synergies between Urban Heat Island and Heat Waves in Athens (Greece), during an extremely hot summer (2012). Sci. Rep. 2017 , 7 , 10973. [ Google Scholar ] [ CrossRef ] [ Green Version ]
- Rogers, C.D.W.; Gallant, A.J.E.; Tapper, N.J. Is the urban heat island exacerbated during heatwaves in southern Australian cities? Theor. Appl. Climatol. 2019 , 137 , 441–457. [ Google Scholar ] [ CrossRef ]
- Davis, R.E.; Hondula, D.M.; Patel, A.P. Temperature Observation Time and Type Influence Estimates of Heat-Related Mortality in Seven U.S. Cities. Environ. Health Perspect. 2016 , 124 , 795–804. [ Google Scholar ] [ CrossRef ]
- Russo, S.; Sillmann, J.; Sterl, A. Humid heat waves at different warming levels. Sci. Rep. 2017 , 7 , 7477. [ Google Scholar ] [ CrossRef ]
- Fernando, H.J. Handbook of Environmental Fluid Dynamics, Volume Two: Systems, Pollution, Modeling, and Measurements ; CRC press: Boca Raton, FL, USA, 2012. [ Google Scholar ]
- Khan, H.S.; Paolini, R.; Santamouris, M.; Caccetta, P. Exploring the Synergies between Urban Overheating and Heatwaves (HWs) in Western Sydney. Energies 2020 , 13 , 470. [ Google Scholar ] [ CrossRef ] [ Green Version ]
- Nicholson, A. Analysis of the diurnal cycle of air temperature between rural Berkshire and the University of Reading: Possible role of the urban heat island. Weather 2020 , 75 , 235–241. [ Google Scholar ] [ CrossRef ]
- Bohnenstengel, S.I.; Evans, S.; Clark, P.A.; Belcher, S.E. Simulations of the London urban heat island. Q. J. R. Meteorol. Soc. 2011 , 137 , 1625–1640. [ Google Scholar ] [ CrossRef ]
- Li, X.-X.; Koh, T.-Y.; Entekhabi, D.; Roth, M.; Panda, J.; Norford, L.K. A multi-resolution ensemble study of a tropical urban environment and its interactions with the background regional atmosphere. J. Geophys. Res. Atmos. 2013 , 118 , 9804–9818. [ Google Scholar ] [ CrossRef ]
- Kottek, M.; Grieser, J.; Beck, C.; Rudolf, B.; Rubel, F. World map of the Köppen-Geiger climate classification updated. Meteorol. Z. 2006 , 15 , 259–263. [ Google Scholar ] [ CrossRef ]
- Kunkel, K.E.; Changnon, S.A.; Reinke, B.C.; Arritt, R.W. The July 1995 heat wave in the Midwest: A climatic perspective and critical weather factors. Bull. Am. Meteorol. Soc. 1996 , 77 , 1507–1518. [ Google Scholar ] [ CrossRef ] [ Green Version ]
- Oke, T.; Cleugh, H. Urban heat storage derived as energy balance residuals. Bound.-Layer Meteorol. 1987 , 39 , 233–245. [ Google Scholar ] [ CrossRef ]
- Arnfield, A.J. Two decades of urban climate research: A review of turbulence, exchanges of energy and water, and the urban heat island. Int. J. Climatol. J. R. Meteorol. Soc. 2003 , 23 , 1–26. [ Google Scholar ] [ CrossRef ]
- Barlow, J.F. Progress in observing and modelling the urban boundary layer. Urban Clim. 2014 , 10 , 216–240. [ Google Scholar ] [ CrossRef ] [ Green Version ]
- Oke, T.R. Boundary Layer Climates , 2nd ed.; Routledge: London, UK, 2002; p. 26. [ Google Scholar ]
- Ward, H.C.; Evans, J.G.; Grimmond, C.S.B. Multi-season eddy covariance observations of energy, water and carbon fluxes over a suburban area in Swindon, UK. Atmos. Chem. Phys. 2013 , 13 , 4645–4666. [ Google Scholar ] [ CrossRef ] [ Green Version ]
- Wang, L.; Li, D.; Gao, Z.; Sun, T.; Guo, X.; Bou-Zeid, E. Turbulent Transport of Momentum and Scalars Above an Urban Canopy. Bound.-Layer Meteorol. 2014 , 150 , 485–511. [ Google Scholar ] [ CrossRef ]
- Offerle, B.; Grimmond, C.S.B.; Fortuniak, K.; Kłysik, K.; Oke, T.R. Temporal variations in heat fluxes over a central European city centre. Theor. Appl. Climatol. 2006 , 84 , 103–115. [ Google Scholar ] [ CrossRef ]
- Kotthaus, S.; Grimmond, C.S.B. Energy exchange in a dense urban environment—Part I: Temporal variability of long-term observations in central London. Urban Clim. 2014 , 10 , 261–280. [ Google Scholar ] [ CrossRef ] [ Green Version ]
- Ching, J.; Clarke, J.; Godowitch, J. Modulation of heat flux by different scales of advection in an urban environment. Bound.-Layer Meteorol. 1983 , 25 , 171–191. [ Google Scholar ] [ CrossRef ]
- Schatz, J.; Kucharik, C.J. Urban climate effects on extreme temperatures in Madison, Wisconsin, USA. Environ. Res. Lett. 2015 , 10 , 094024. [ Google Scholar ] [ CrossRef ] [ Green Version ]
- Zeng, Z.; Piao, S.; Li, L.Z.X.; Zhou, L.; Ciais, P.; Wang, T.; Li, Y.; Lian, X.; Wood, E.F.; Friedlingstein, P.; et al. Climate mitigation from vegetation biophysical feedbacks during the past three decades. Nat. Clim. Chang. 2017 , 7 , 432–436. [ Google Scholar ] [ CrossRef ]
- Monin, A.S.; Obukhov, A.M. Basic laws of turbulent mixing in the surface layer of the atmosphere. Contrib. Geophys. Inst. Acad. Sci. USSR 1954 , 151 , e187. [ Google Scholar ]
- Itenfisu, D.; Elliott, R.L.; Allen, R.G.; Walter, I.A. Comparison of reference evapotranspiration calculations as part of the ASCE standardization effort. J. Irrig. Drain. Eng. 2003 , 129 , 440–448. [ Google Scholar ] [ CrossRef ]
- Zangvil, A. Six years of dew observations in the Negev Desert, Israel. J. Arid. Environ. 1996 , 32 , 361–371. [ Google Scholar ] [ CrossRef ]
- Black, E.; Blackburn, M.; Harrison, G.; Hoskins, B.; Methven, J. Factors contributing to the summer 2003 European heatwave. Weather 2004 , 59 , 217–223. [ Google Scholar ] [ CrossRef ]
- Oláh, A. The possibilities of decreasing the urban heat island. Appl. Ecol. Environ. Res. 2012 , 10 , 173–183. [ Google Scholar ] [ CrossRef ]
- Masson, V.; Marchadier, C.; Adolphe, L.; Aguejdad, R.; Avner, P.; Bonhomme, M.; Bretagne, G.; Briottet, X.; Bueno, B.; de Munck, C.; et al. Adapting cities to climate change: A systemic modelling approach. Urban Clim. 2014 , 10 , 407–429. [ Google Scholar ] [ CrossRef ]
- Allegrini, J.; Dorer, V.; Carmeliet, J. Influence of the urban microclimate in street canyons on the energy demand for space cooling and heating of buildings. Energy Build. 2012 , 55 , 823–832. [ Google Scholar ] [ CrossRef ]
- Peng, S.; Piao, S.; Ciais, P.; Friedlingstein, P.; Ottle, C.; Breon, F.M.; Nan, H.; Zhou, L.; Myneni, R.B. Surface urban heat island across 419 global big cities. Environ. Sci. Technol. 2012 , 46 , 696–703. [ Google Scholar ] [ CrossRef ]
- Rahman, M.A.; Hartmann, C.; Moser-Reischl, A.; von Strachwitz, M.F.; Paeth, H.; Pretzsch, H.; Pauleit, S.; Rötzer, T. Tree cooling effects and human thermal comfort under contrasting species and sites. Agric. For. Meteorol. 2020 , 287 , 107947. [ Google Scholar ] [ CrossRef ]
- Rahman, M.A.; Moser, A.; Rötzer, T.; Pauleit, S. Comparing the transpirational and shading effects of two contrasting urban tree species. Urban Ecosyst. 2019 , 22 , 683–697. [ Google Scholar ] [ CrossRef ]
- Santamouris, M.; Ban-Weiss, G.; Osmond, P.; Paolini, R.; Synnefa, A.; Cartalis, C.; Muscio, A.; Zinzi, M.; Morakinyo, T.E.; Ng, E.; et al. Progress in Urban Greenery Mitigation Science—Assessment Methodologies Advanced Technologies and Impact on Cities. J. Civ. Eng. Manag. 2018 , 24 , 638–671. [ Google Scholar ] [ CrossRef ] [ Green Version ]
- Manickathan, L.; Defraeye, T.; Allegrini, J.; Derome, D.; Carmeliet, J. Parametric study of the influence of environmental factors and tree properties on the transpirative cooling effect of trees. Agric. For. Meteorol. 2018 , 248 , 259–274. [ Google Scholar ] [ CrossRef ] [ Green Version ]
- Alavipanah, S.; Wegmann, M.; Qureshi, S.; Weng, Q.; Koellner, T. The Role of Vegetation in Mitigating Urban Land Surface Temperatures: A Case Study of Munich, Germany during the Warm Season. Sustainability 2015 , 7 , 4689–4706. [ Google Scholar ] [ CrossRef ] [ Green Version ]
- Gill, S.E.; Handley, J.F.; Ennos, A.R.; Pauleit, S. Adapting cities for climate change: The role of the green infrastructure. Built Environ. 2007 , 33 , 115–133. [ Google Scholar ] [ CrossRef ] [ Green Version ]
- Ballinas, M.; Barradas, V.L. The Urban Tree as a Tool to Mitigate the Urban Heat Island in Mexico City: A Simple Phenomenological Model. J. Environ. Qual. 2016 , 45 , 157–166. [ Google Scholar ] [ CrossRef ] [ PubMed ]
- Rahman, M.A.; Moser, A.; Gold, A.; Rotzer, T.; Pauleit, S. Vertical air temperature gradients under the shade of two contrasting urban tree species during different types of summer days. Sci. Total Environ. 2018 , 633 , 100–111. [ Google Scholar ] [ CrossRef ] [ PubMed ]
- Gao, K.; Santamouris, M. The use of water irrigation to mitigate ambient overheating in the built environment: Recent progress. Build. Environ. 2019 , 164 , 106346. [ Google Scholar ] [ CrossRef ]
- Broadbent, A.M.; Coutts, A.M.; Tapper, N.J.; Demuzere, M. The cooling effect of irrigation on urban microclimate during heatwave conditions. Urban Clim. 2018 , 23 , 309–329. [ Google Scholar ] [ CrossRef ]
- Im, E.-S.; Pal, J.S.; Eltahir, E.A. Deadly heat waves projected in the densely populated agricultural regions of South Asia. Sci. Adv. 2017 , 3 , e1603322. [ Google Scholar ] [ CrossRef ] [ Green Version ]
- Mughal, M.O.; Li, X.X.; Yin, T.; Martilli, A.; Brousse, O.; Dissegna, M.A.; Norford, L.K. High-resolution, multilayer modeling of Singapore’s urban climate incorporating local climate zones. J. Geophys. Res. Atmos. 2019 , 124 , 7764–7785. [ Google Scholar ] [ CrossRef ]
- Liu, X.; Li, X.-X.; Harshan, S.; Roth, M.; Velasco, E. Evaluation of an urban canopy model in a tropical city: The role of tree evapotranspiration. Environ. Res. Lett. 2017 , 12 , 094008. [ Google Scholar ] [ CrossRef ]
- Rai, R.K.; Berg, L.K.; Kosović, B.; Mirocha, J.D.; Pekour, M.S.; Shaw, W.J. Comparison of measured and numerically simulated turbulence statistics in a convective boundary layer over complex terrain. Bound.-Layer Meteorol. 2017 , 163 , 69–89. [ Google Scholar ] [ CrossRef ]
- Fan, Y.; Ding, X.; Wu, J.; Ge, J.; Li, Y. High spatial-resolution classification of urban surfaces using a deep learning method. Build. Environ. 2021 , 200 , 107949. [ Google Scholar ] [ CrossRef ]
- Martilli, A.; Krayenhoff, E.S.; Nazarian, N. Is the urban heat island intensity relevant for heat mitigation studies? Urban Clim. 2020 , 31 , 100541. [ Google Scholar ] [ CrossRef ]
Click here to enlarge figure
Platforms | Record Period (Year) | Spectral Resolution (Bands) | Temporal Resolution (Days) | Spatial Resolution (m) |
---|---|---|---|---|
MODIS | 2000+ | 36 | 1–2 | 250, 500, 1000 |
Landsat | 1972+ | 7, 8, 11 | 16 | 15, 30, 60 /100 /120 |
Ref. | Atmospheric Models | Urban Parameterizations | Resolution (m) | Validation |
---|---|---|---|---|
[ ] | WRF | SLUCM, Noah LSM | 300 | In situ measure |
[ ] | WRF | SLUCM, Noah LSM | 500 | In situ measure |
[ ] | WRF | BEP, BEM, Noah LSM | 1000 | In situ measure |
[ ] | WRF | BEP, Noah LSM | 1000 | In situ measure |
[ ] | WRF | SLUCM | 1000 | In situ measure |
[ ] | WRF | SLUCM, Noah LSM | 300 | In situ measure |
[ ] | WRF | BEP, BEM, Noah LSM | 333 | In situ measure |
[ ] | WRF | BEP, Noah LSM | 1000 | In situ measure |
[ ] | WRF | SLUCM, Noah LSM | 3000 | In situ measure |
[ ] | WRF | BEP | 1333 | In situ measure |
[ ] | WRF | SLUCM | 1000 | In situ measure, Remote sensing |
[ ] | WRF | SLUCM, Noah LSM | 2000 | In situ measure |
[ ] | WRF | SLUCM, Noah LSM | 2000 | In situ measure |
[ ] | WRF | BEP, BEM, Noah LSM | 1000 | In situ measure |
[ ] | WRF | SLUCM, Noah LSM | 2000 | In situ measure |
[ ] | WRF | SLUCM, Noah LSM | 2000 | In situ measure |
[ ] | WRF | PUCM, Noah LSM | 1000 | In situ measure, Remote sensing |
[ ] | WRF | PUCM, Noah LSM | 3000 | In situ measure, Remote sensing |
[ ] | WRF | MLUCM, BEM, Noah LSM | 260 | In situ measure |
[ ] | WRF | PUCM, Noah LSM | 1000 | In situ measure |
[ ] | WRF | Noah LSM | 2000 | In situ measure |
[ ] | WRF | PUCM, Noah LSM | 1000 | N/A |
[ ] | WRF | SLUCM, Noah LSM | 1000 | In situ measure |
[ ] | WRF | SLUCM, Noah LSM | 4000 | In situ measure |
[ ] | WRF | UCM, Noah LSM | 1000 | In situ measure |
[ ] | WRF-Chem | MLUCM, Noah LSM | 2400 | In situ measure |
[ ] | WRF-Chem | SLUCM, Noah LSM | 3000 | In situ measure |
[ ] | WRF-Chem | BEP, Noah LSM | 3000 | In situ measure |
[ ] | WRF-Chem | MLUCM, BEM, Noah LSM | 1000 | In situ measure |
[ ] | ALARO-0 | TEB | 1000 | In situ measure |
[ ] | UrbClim | N/A | 1000 | In situ measure |
[ ] | Meso-NH | TEB, ISBA | 1000 | In situ measure |
[ ] | Meso-NH | TEB, ISBA | 500 | N/A |
[ ] | MUKLIMO_3 | N/A | 100 | In situ measure |
[ ] | CESM | N/A | 99,000 × 138,750 | Remote sensing |
Type | Processes | Impacts |
---|---|---|
UHI | Subsurface energy balance | Carbon exchange between soil and atmosphere |
UHI | Surface energy balance | Thermal comfort |
UHI | Surface energy balance, energy balance of UCL volume | Thermal comfort, building energy demand, thermal circulation, air quality |
UHI | Boundary layer energy balance, energy balance at top of roughness sublayer | Air quality, precipitation, local circulation |
Metric | Intensity | Duration (Days) | Location | Ref. |
---|---|---|---|---|
T | 32 °C | 10 | Taiwan Region | [ ] |
T | 32.2 °C | 3 | USA | [ , , , , ] |
T | 33 °C | 2 | South Korea | [ ] |
T | 33 °C | 3 | South Korea | [ ] |
T | 35 °C | 3 | China Mainland | [ , , ] |
T | 35 °C | 10 | Taiwan Region | [ ] |
T | 36.5 °C | 3 | Spain | [ ] |
T | 37 °C | 3 | Greece | [ , ] |
T | 90th | 3 | Romania | [ ] |
T | 98th | 3 | Romania | [ ] |
T | 95th | 3 | Romania, Australia | [ , ] |
T | 95th | 4 | Cyprus | [ ] |
T | 97.5th | 5 | USA | [ ] |
T | 97.5th and 81st | 3 | USA, China Mainland, Poland, UK | [ , , , ] |
T | T + 5 °C | 3 | UK | [ ] |
T | T + 5 °C | 5 | Hungary | [ ] |
T | 25 °C | 3 | Hungary | [ ] |
T | 27 °C | 3 | Hungary | [ ] |
T | 90th | 3 | Portugal | [ ] |
T and T | 32 °C and 16 °C | 2 | France | [ ] |
T and T | 30 °C and 18 °C | 3 | Belgium | [ ] |
T and T | 30 °C and 20 °C | 5 | Hungary | [ ] |
T and T | 90th | 3 | Australia | [ ] |
T and T | 95th | 3 | India | [ ] |
T and T | 35 °C and 29 °C | 3 | Singapore | [ ] |
HI | 65 °C | 4 | Cyprus | [ ] |
MDPI stays neutral with regard to jurisdictional claims in published maps and institutional affiliations. |
Share and Cite
Kong, J.; Zhao, Y.; Carmeliet, J.; Lei, C. Urban Heat Island and Its Interaction with Heatwaves: A Review of Studies on Mesoscale. Sustainability 2021 , 13 , 10923. https://doi.org/10.3390/su131910923
Kong J, Zhao Y, Carmeliet J, Lei C. Urban Heat Island and Its Interaction with Heatwaves: A Review of Studies on Mesoscale. Sustainability . 2021; 13(19):10923. https://doi.org/10.3390/su131910923
Kong, Jing, Yongling Zhao, Jan Carmeliet, and Chengwang Lei. 2021. "Urban Heat Island and Its Interaction with Heatwaves: A Review of Studies on Mesoscale" Sustainability 13, no. 19: 10923. https://doi.org/10.3390/su131910923
Article Metrics
Article access statistics, supplementary material.
ZIP-Document (ZIP, 582 KiB)
Further Information
Mdpi initiatives, follow mdpi.
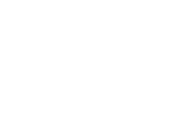
Subscribe to receive issue release notifications and newsletters from MDPI journals

An official website of the United States government
The .gov means it’s official. Federal government websites often end in .gov or .mil. Before sharing sensitive information, make sure you’re on a federal government site.
The site is secure. The https:// ensures that you are connecting to the official website and that any information you provide is encrypted and transmitted securely.
- Publications
- Account settings
Preview improvements coming to the PMC website in October 2024. Learn More or Try it out now .
- Advanced Search
- Journal List
- Int J Environ Res Public Health

The Impact of Urbanization on Urban Heat Island: Predictive Approach Using Google Earth Engine and CA-Markov Modelling (2005–2050) of Tianjin City, China
Nadeem ullah.
1 School of Architecture, Tianjin University, Tianjin 300272, China
Muhammad Amir Siddique
Mengyue ding, sara grigoryan, irshad ahmad khan.
2 Centre for Research in Agricultural Genomics (CRAG) CSIC-IRTA-UAB-UB, Campus UAB, Bellaterra, 08193 Barcelona, Spain
Zhihao Kang
Shangen tsou, tianlin zhang, yazhuo zhang.
3 School of Civil Engineering, Tianjin University, Tianjin 300272, China
Associated Data
On request, the authors will provide the data from this study.
Urbanization has adverse environmental effects, such as rising surface temperatures. This study analyzes the relationship between the urban heat island (UHI) intensity and Tianjin city’s land cover characteristics. The land use cover change (LUCC) effects on the green areas and the land surface temperature (LST) were also studied. The land cover characteristics were divided into five categories: a built-up area, an agricultural area, a bare area, a forest, and water. The LST was calculated using the thermal bands of spatial images taken from 2005 to 2020. The increase in the built-up area was mainly caused by the agricultural area decreasing by 11.90%. The average land surface temperature of the study area increased from 23.50 to 36.51 °C, and the region moved to a high temperature that the built-up area’s temperature increased by 1.5%. Still, the increase in vegetation cover was negative. From 2020 to 2050, the land surface temperature is expected to increase by 9.5 °C. The high-temperature areas moved into an aerial distribution, and the direction of urbanization determined their path. Urban heat island mitigation is best achieved through forests and water, and managers of urban areas should avoid developing bare land since they may suffer from degradation. The increase in the land surface temperature caused by the land cover change proves that the site is becoming more urbanized. The findings of this study provide valuable information on the various aspects of urbanization in Tianjin and other regions. In addition, future research should look into the public health issues associated with rapid urbanization.
1. Introduction
The rapid growth of urban areas worldwide has been observed over the past few decades [ 1 ]. The main factors contributing to urbanization are the lack of economic development and the increasing population [ 2 ]. Despite the slow growth of the global population, it is still expected that the number of people will continue to increase by around 2030 [ 1 ]. According to estimates, the world’s urban area is expected to grow by over a million kilometers by 2030 [ 1 , 3 , 4 , 5 , 6 ]. Urbanization is most prevalent in developing countries due to rapid economic development. China is one of the most prominent in the world regarding urbanization. It has been estimated that the country’s urban land area expanded at an annual rate of 13.3% [ 7 ].
Urbanization positively impacts people’s lives, as it allows them to improve their living standards and reduce their energy consumption. It can also help mitigate climate change by reducing vehicle miles travelled and greenhouse gas emissions [ 8 ]. Unfortunately, there are still negative impacts of urbanization. Due to the human activities that have occurred in the past few decades, the city has expanded. This process has caused both positive and negative effects [ 9 ].
Urbanization is a complex process involving multiple modelling variables and mechanisms involved in its development. The various aspects of this process must be thoroughly studied to understand its effects. One of the most effective ways to predict an urban area’s characteristics is through Land Use Cover change (LUCC) analysis [ 8 , 10 , 11 ]. A comprehensive simulation of the urban development process is necessary in today’s world [ 12 , 13 ]. With the help of spatial data, such as land area and development characteristics, urban models can be used to study the patterns of urbanization. These models can also simulate the conditions affecting the city’s development. Urban models use mathematical equations to describe the urban system [ 14 , 15 ]. They can also deal with the various factors that affect the development of a city. The study results are based on the interactions between different strategies and aspects [ 9 , 16 ]. Urban models are becoming more effective at predicting future changes in the LUCC due to the complexity of the process. They can use the available data and conditions to model the different factors affecting the city’s development. Numerous studies have been conducted on the use of LUCC in policy formulation and decision making.
However, the application of cellular automata and the Markov process is relatively rare. Tianjin is considered one of the most prominent cities in China that has experienced sustained urbanization, industrialization, and urbanization in China [ 17 , 18 ]. As a result of its ongoing development, many cities are expected to continue to grow [ 19 ]. It is essential that the cities’ LUCC change be studied and analyzed to determine its future trend [ 20 , 21 ]. This study was conducted to comprehensively analyze the various factors that have affected the city’s development. The study examined the LUCC change in Tianjin from 1995 to 2015. It first created five maps with different classifications at different points in time. The analysis revealed that many areas were converted into built-up areas. The model was then analyzed to create a set of dynamic variables for the Cellular Automata Model (CA) [ 11 , 22 , 23 ]. These variables were then used to project the LUCC change in the city from 2025 to 2050.
2. Materials and Methods
2.1. study area.
The city of Tianjin, the largest city on China’s northern coast, is straddled at 38°34′ N to 40°15′ N and 116°43′ E to 118°04′ E ( Figure 1 ), having a thousand square kilometers. It is regarded as the fifth-largest city in the country after Shanghai, Beijing, Guangzhou, and Shenzhen [ 24 ]. With a warm, temperate, semi-humid monsoonal climate, it is characterized by four distinct seasons during the year [ 25 , 26 ]. Over the past few years, Tianjin has experienced massive urbanization, with its population increasing from 12.99 million in 2010 to 13.86 million in 2021 [ 1 ].The city of Tianjin has a gross domestic product of about 240 billion yuan, making it one of the most prominent economic centers in China’s northern region [ 25 , 27 , 28 ]. It is an international port city and has experienced rapid urbanization over the past few decades. Due to rapid urbanization, large areas of land, such as forests, farmland, and meadows, have been converted into built-up areas [ 29 , 30 ].

Geographic location and characterization of the study area: ( A ) People’s Republic of China; ( B ) Beijing–Tianjin–Hebei (TBH); and ( C ) multispectral satellite image of Tianjin city.
2.2. Acquisition of Spatial Dataset
The United States Geological Survey (USGS) provided cloud-free images of the study area, which were taken from path 170 and series 053, through its website ( http://earthexplorer.com ) [ 6 , 9 , 10 , 13 , 17 , 25 , 31 ]. Due to the varying time of day and night in the study area, the data collected by the Landsat 5 Thematic Mapper (TM) and the Landsat 7 Enhanced Thematic Mapper (ETM) were used to create the LUCC map [ 13 , 18 , 32 , 33 , 34 ]. The data collected by the two satellites ( Table 1 ) were also used to calculate the Normalized Difference of Vegetation Index (NDVI) and Land Surface Temperature (LST).
The Landsat data used in this study are outlined in detail.
Period-Images | Satellite Sensor ID | Spatial Resolution |
---|---|---|
2005 May–September | Landsat-5 TM | 30 m|100 m |
2010 May–September | Landsat-5 TM | 30 m|100 m |
2015 May–September | Landsat-8 OLI_TIRS | 30 m|100 m |
2020 May–September | Landsat-8 OLI_TIRS | 30 m|100 m |
2.3. Methodology
An integrated workflow template ( Figure 2 ) was used to perform a series of steps. We began by processing the information sets in GEE to create a false colour positive (FCC) [ 10 , 11 , 25 , 35 , 36 ]. A georeferenced map of the outer boundaries of Tianjin was used to extract and mask the study area from all spatial ideas. The support Vector Machine (SVM) classification method was applied to improve the supervised classification results obtained from Landsat imagery [ 13 , 30 , 37 , 38 ]. Then, the LST was calculated to determine the time zones in the city [ 17 ]. A Pearson correlation analysis was performed based on the land cover, average LST, and percentage of greened and non-greened areas from 2005, 2010, 2015, and 2020 [ 8 , 31 , 39 , 40 ]. The CA-Markov model was used to forecast future trends for LUCC and LST in 2035 and 2050 [ 41 ]. All spatial statistical analyses and maps were created using ArcGIS 10.7, and ggplot2, corrplot and psych packages used in RStudio [ 42 ].

Flowchart of the methodology for the present study.
2.4. Land Use Cover Change (LUCC) Calculation
Landsat imagery (Landsat-5 TM & Landsat-8 OLI) was used to map the LUCC of Tianjin city for a four-time frame (2005, 2010, 2015, and 2020). The Support Vector Machine (SVM) classification algorithm in GEE was used to classify land use and areas [ 43 , 44 ]. Five types of LUCC were identified: built-up land, cropland, lowland, forest, and water body ( Figure 3 A). Built-up land included artificial structures such as buildings, roads, and other impervious surfaces. Water included rice fields, reservoirs, and rivers [ 28 , 35 , 45 ].

( A ) Shows the land use land cover area, and ( B ) proportional changes of LUCC during 2005-2020.
At specified intervals, GEE was used to assess the accuracy of the classification results. Field reference points were collected using a Google Earth explorer, which collected field reference average of 250 points for 2005, 2010, 2015, and 2020.
The classification accuracy of the signatures and images was evaluated by creating a confusion matrix consisting of rows and columns that refer to the categories derived from the image. The matrix rows are labelled with the reference values, while the columns represent the categories identified using the same criteria. The total number of entries that formed the main diagonal was then divided by the number of pixels. The Kappa coefficient was calculated using Equations (1)–(3) [ 1 , 2 , 46 , 47 ]:
where r = the number of rows in the error matrix; P ij = The proportion of pixels in a row “ i ” and column “ j ”; and P i = the fraction of the marginal sum of row “ i ”.
2.5. Calculation of Land Surface Temperature (LST)
The Landsat-8 thermal infrared sensor (TIRS) of bands 10 and 11 and the OLI sensor of bands 2–5 were used individually to convert the raw image into a radiance spectral image (SR) by following the equations ( Table 2 ) step by step.
Stepwise process for Land surface temperature (LST) determination.
Steps | Process Name | Equations | References |
---|---|---|---|
1 | Spectral Radiance (SR) | [ , , ] | |
2 | Brightness Temperature (T ) | [ , , ] | |
3 | NDVI | [ , , ] | |
4 | Fractional Vegetation (F ) | [ , , , ] | |
5 | Surface Emissivity (S ) | [ , , , ] | |
6 | Land Surface Temperature (LST) | [ , , , ] |
where λ is the effective wavelength (10.9 mm for a thermal band in Landsat 8 data), σ is the Boltz–Mann constant (1.38 × 10 −23 J/K), h is the Plank constant (6.626 × 10 −34 Js), and c is the speed of light in vacuum (2.998 × 10 −8 m/sec).
2.6. CA-Markov Prediction Model Analysis
This model uses a stochastic Markov probability matrix to predict the transition from one state to another [ 14 , 43 , 50 ]. The study aims to analyze the various effects of urbanization on the land use and development of the city of Tianjin using a computer model known as a Markov chain model. This model was used to predict land use and development trends [ 13 , 36 , 51 ]. A conditional probability formula was used to estimate trend lines from Equations (4)–(6).
Because of Markov chain and cellular automata modelling, LUCC and LST’s future scenarios are calculated by projecting 2035 and 2050 using Terrset’s land use change modeler (LCM) (Clark Labs TerrSet 18.31).
3.1. Changes in LUCC between 2005 and 2020
According to the LUCC distribution values for 2005, 2010, 2015, and 2020, the built-up area in cities has increased ( Figure 3 ). Built-up area increased from 15.46% in 2005 to 17.80% in 2010, 19.56% in 2020, and 22.72% in 2050. In 2005, the study area included 18.43% of the lowlands; this number decreased to 12.52% by 2010, 11.89% by 2015, and 10.21% by 2020. Arable land increased rapidly from 26.10% in 2005 to 28.95% in 2020, while other land decreased from 26.47% to 26.10%. Increasing migration from villages to cities has led to an expansion of cultivated land outside prime locations. The cultivated area decreased from 10.72% in 2005 to 7.98% in 2020. Water covered 1.21% of the site in 2005, 0.92% in 2010, 0.87% in 2015, and 0.68% in 2020. An assessment of land use changes during 2005–2020 showed that farmland in the northeastern study area was converted to urban areas (mainly industrial areas). Between 2005 and 2020, built-up urban land and cropland increased by 15.45% and 1.64%, respectively, while lowland land decreased by 13.73%. These results show that about 11.45% of the lowlands have been converted into built-up areas. The LUCC changes were classified into five categories LUC with corresponding definitions ( Table 3 ).
Land use cover (LUC) statistics in 2035 and 2050.
Category | LUC_2035 | LUC_2050 | ||
---|---|---|---|---|
Area | %Age | Area | %Age | |
urban | 3001.75 | 18% | 3204.70 | 19% |
cropland | 401.69 | 2% | 272.27 | 2% |
water | 1716.04 | 10% | 1678.71 | 10% |
forest | 1982.96 | 12% | 1946.75 | 11% |
lowland | 9914.57 | 58% | 9914.57 | 58% |
The results of all studies show that urban built-up has changed significantly over two decades. In recent decades, Tianjin has gone from a village to a city residential settlement. This transition happens between agricultural land to residential areas. Urban growth and LST are sensitive to accuracy assessment [ 29 ]. According to [ 52 , 53 ], a method was defined for assessing the accuracy of the classification of maps. According to the LUCC maps, the overall accuracy was 84.39% in 2005, 90.43% in 2010, and 94.11% in 2020. Kappa coefficients for the LUCC maps were 0.79, 0.87, and 0.92. The kappa coefficient should be greater than 0.75 or 0.80 to show compatibility between the classification and the reference data [ 54 ]. The United States Geological Survey (USGS) recommends using Landsat satellite images for LUCC mapping if the accuracy level is 85% [ 55 ]. Our accuracy evaluation results are consistent with those recommended in the literature.
3.2. Relationship between LUCC and LST
LST is significantly affected by land use changes (LUCC). The number and distribution of hotspots increase with LUCC types (especially urban expansion) [ 56 ]. A map of LST distribution was created using Landsat TM/ETM+/OLI imagery for the study area ( Figure 4 ). There were temperature variations from 21 °C to 43 °C in 2005, 21.8 °C to 44.3 °C in 2010, 22.1 °C to 44.9 °C in 2010, and 22.5 °C to 45.9 °C in 2020. During 2005–2020, built-up urban areas had the highest average temperatures, followed by lowland, cropland, vegetation, and water. In 2005, all LUCC categories had the most elevated average temperatures ( Figure 5 A). In 2005, urban built-up areas had an average LST of 38.43 °C, 38.99 °C in 2010, 41.86 °C in 2015, and 44.80 °C in 2020. During 2005–2020, the temperature in built-up urban areas LST decreased by 4.12 °C but increased by a maximum of 6.82 °C from 2010 to 2020. Lowlands had the second-highest LST for all LUCC categories during the study period. The LST for wasteland decreased by 3.38 °C from 2005 to 2010 but did not change significantly between 2010 and 2020. The LST for cropland was 31.04 °C in 2015 and increased to 31.98 °C, 32.63 °C, and 33.75 °C in 2010, 2015, and 2020, respectively.

( A ) Maps for land use land cover (LUCC) classes: (i) water, (ii) vegetation, (iii) forest, (iv) urban, (v) barren land, (vi) cropland; ( B ) land surface temperature (LST) was divided into five thermal categories: (i) <20 °C, (ii) 20–25 °C, (iii) 25–30 °C, (iv) 30–35 °C, and (v) >35 °C of Tianjin between the study period of 2005 to 2020, at 5-year intervals.

( A ) Distribution of land surface temperature, and ( B ) proportional changes of LST during 2004–2019.
From 2005 to 2020, the temperature of cropland LST decreased by 3.85 °C, while it increased by 15.35% in developed areas. Vegetated areas recorded a decrease of 5.32 °C between 2005 and 2010 LST but an increase of 6.83 °C between 1999 and 2015. All LUCC categories recorded the lowest LST in 2010, and the average temperature in water bodies and vegetated areas was the lowest overall. According to statistics from LST for 2005–2020, the maximum difference between urban areas and water bodies is 14.35 °C.
LST has remained relatively stable between 2005 and 2020. These areas are also referred to as lowlands. Compared to urban areas, lowlands have a higher LST value. Our study came to similar conclusions. High LST values may be found in these areas due to the soil composition (sand, clay, etc.). The average daily air temperature may influence the LST values at the satellite imagery data on the day the satellite imagery was taken rather than the spatial values of the land use classes. To verify that LST results calculated from the Landsat TM/ETM heat band are comparable to actual field temperatures, temperatures of the various LUCC properties must be measured from field observations [ 57 ]. Considering the values reported at LST, the daily mean air temperatures of the reported data (the daily mean air temperature on 19 June 2005 is 27.5 °C; on 10 July 2010, it is 23.03 °C; on 23 July 2015, it is 26.86 °C; and the daily mean air temperature on 11 August 2020 is 29.53 °C) are all parallel to each other (See Figure 6 ).

Spatial distribution map for change detection of ( A ) LUCC (km 2 ) and ( B ) LST (°C) during 2005–2020.
Pearson’s correlation analysis shows LST is statistically associated with populated/developed areas. Even though LST is bad for water and plants and does not have much to do with them, it is strongly linked to forested areas. In the same way, LSTs in cities have a negative and insignificant effect on water and plants. LST and urban/built-up areas have a significant and favourable relationship, as shown by the simple correlation coefficient [ 51 ]. In urban areas, a temperature rise may also be caused by the construction of new buildings, highways, businesses, and industrial regions. Negative and insignificant correlations are observed with barren land, while optimistic and negligible correlations are marked with arable and cropland. Pearson correlation analysis results are reported for all LUC variables and LST indices ( Figure 7 ).

Pearson correlation analysis of land use change and land surface temperature during 2005-2020.
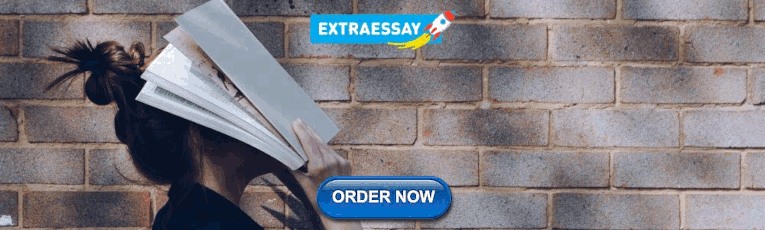
3.3. Variations of LST Changes over Different LUCC
We estimated the mean LST distributions for LUCC classes over 2005–2020. During the study period, mean values of LST increased significantly in all LUCC classes, but matters of LST were substantially higher in built-up areas and bare ground. The importance of LST in the built-up area increased from 28.86 °C to 37.23 °C between 2005 and 2020, while in the empty ground area, they increased from 21.56 °C to 25.01 °C. Over the past two decades, the average LST distribution in built-up and bare-ground regions has risen by about 9 °C and 4 °C, respectively. The LST distribution in water bodies and vegetated areas have also changed. In 2000, the mean LST for vegetated areas was 21.31 °C, but it is expected to reach 25.98 °C by 2020. The LST of water bodies increased from 20 to 24.45 °C. The following figure ( Figure 8 ) briefly describes the changes in LUCC types and their relative impacts on land surface temperature.

Shows classified maps of LUC and LST for the year 2020, and predicted maps of 2020, 2035, and 2050.
3.4. Validation of Predicted LUCC and LST Scenarios
To validate the accuracy of the predicted values, we first used the CA-Markov model to estimate the LUCC and LST for 2020 ( Figure 8 ). Based on various kappa parameters, the predicted and estimated maps were compared using the land use Change Modeler in Clark Lab’s Terrset software. The average error value for all parameters during the comparison was about 12.86%, and all kappa parameters, percentage of accuracy, and total kappa values were above 0.80.
3.5. Predicted LUCC for 2035 and 2050
We could predict the scenario for 2035 and 2050 based on the classified maps for the study period. According to the predicted LUCC map, the growth of urban areas will be concentrated by 37% in the northwestern and central regions if the trend of the building continues without planned actions. Urban areas will replace the lowlands and vegetation cover. Vegetation cover has decreased by 9.62% from 12.82% in 2020. Based on the study scenario, LUCC would face a 20.51% increase in developed land, followed by a significant decrease in lowlands, vegetation cover, and water bodies of 10.87%, 9.62%, 8.32%, and 2.45%, respectively ( Figure 8 ). The category-wise land use statistics for the forecast years are shown in the following table: Ecosystem services, urban health, and thermal characteristics may be affected by decreased vegetation cover and increased urbanization. If unplanned urban expansion continues, the environmental, economic, and medical problems will increase significantly. A proper land use plan, the protection of water bodies, and the reforestation of forests are needed to make Tianjin city more environmentally sustainable.
By forecasting LST for 2035 and 2050, the simulation showed that higher temperatures will occur in the built-up areas in the northwest and central parts of the country ( Figure 8 ), ranging from 41.56 °C to 44.34 °C in 2035 and 2050, respectively. We divide the temperature zone into five classes to estimate how much area is covered by each temperature range ( Table 4 ). Based on the projections, LST has increased over the past two decades (2005–2020), with urban areas influencing the prevalence of LST. UHI effects will increase as urban areas and vegetation cover decrease. It would be possible to explain the temperature increase without urbanization by climate change, greenhouse effects, and surface features. The LST prediction highlights the real risks of the temperature rise in the trend, including higher UHI effects. A combination of energy use, greenhouse gas emissions, and air pollution contribute to the UHI effect. It threatens aquatic systems (rivers, lakes, ponds, streams, and oceans) and human health. Human health is primarily harmed by increased greenhouse gas emissions, which affect urban health and reduce the urban environment’s sustainability [ 58 ].
Land surface temperature (LST) statistics in 2035 and 2050.
Category | LST-2035 | LST-2050 | ||
---|---|---|---|---|
Area | %Age | Area | %Age | |
<20 °C | 1839.763 | 16.85% | 1851.812 | 16.96% |
20–25 °C | 4933.693 | 45.18% | 5056.619 | 46.31% |
25–30 °C | 4118.498 | 37.72% | 3859.214 | 35.34% |
30–35 °C | 26.86548 | 0.25% | 150.4464 | 1.38% |
>35 °C | 0.113955 | 0.00% | 0.842528 | 0.01% |
3.6. Limitations of the CA-Markov Model
The prediction of LUCC and LST can be improved using the CA-Markov model if the previous LUCC and LST patterns are consistent. As a result, CA-Markov models do not provide accurate spatial predictions for raster datasets [ 59 ]. Since influential factors can be directly determined between CA-Markov and other factors, CA-Markov is based on a probability matrix [ 60 ]. Given the relative importance of the different variables in identifying the most important variables, it is essential to note that the CA-Markov model generates training patterns and automatically begins training after receiving inputs from the strata. The input parameters are not individually weighted according to established standards [ 61 ]. Since urbanization, the loss of green space and increase in surface temperatures are primarily influenced by human activities and conscious decisions at regional to metropolitan scales; it is impossible to predict them accurately. It is essential to recognize that dynamic models have some limitations. Still, they help develop hypotheses and make decisions about changes in land cover or surface temperatures in any given area, regardless of their rules. In recent years, LUCC and LST variability and predictive maps have emerged as one of the best tools for managing and mitigating vital natural resources.
4. Discussion
Tianjin’s rapid urbanization and development between the 1990s and 2020s significantly altered the LUCC landscapes caused by farmland separation and reduced total vegetation cover [ 25 , 33 ]. The city’s urban development also resulted in the establishment of new industries and residential areas. Rapid vegetation cover loss affects an area’s natural cooling effect [ 29 , 62 ]. Some factors contributing to this phenomenon are vegetation shading and transpiration. To amplify this, LST and NDVI have shown that VC, due to its cooling effect, serves as a sink in an urban heat island [ 11 , 63 ]. Rapid vegetation cover loss has several consequences for an area’s natural cooling effect. It has the potential to eventually eliminate the processes that regulate surface transpiration and evaporation [ 11 , 17 , 56 , 64 ]. Urbanization leads to distorted construction, reducing soil infiltration and increasing surface runoff. As a result, the water table and groundwater table decrease. Evapotranspiration is not adequately realized due to these two factors. Climate change leads to a deterioration of the water balance [ 49 ]. Climate variables such as daily maximum and minimum temperatures are affected by changes in land use. Surface albedo changes due to changes in land use. Therefore, land use changes disturb the balance of Earth’s radiation [ 65 ]. An important factor in reducing air temperatures is the conversion of wetlands to agricultural land with high albedo [ 66 ].
Although the impact of this phenomenon on the LST of various types of plants is less than that of urban tree cover and gardens, studies have shown that it still contributes to the overall reduction of the area’s natural cooling effect [ 32 , 67 ]. The impact of various types of urban vegetation, water bodies, and forests on the LST varies according to their proportional area [ 23 , 37 ]. In urban areas, vegetation plays a vital role in controlling or mitigating temperature. Evaporation from urban water bodies contributes to moisture accumulation in the surrounding air. According to studies, these bodies regulate the LST in residential areas. It is also known that urban areas contribute to the development of intricate heat flows within these regions [ 46 , 68 ]. Various private and public entities have worked together to revitalize large tracts of land for industrial, commercial, and residential development. Traditional wooden structures have been demolished and replaced with tall structures made of non-evaporative materials such as glass, concrete, and aluminium. These materials can directly impact heat flows in urban areas [ 8 , 10 ]. According to studies, urban areas in China are more vulnerable to severe LST than rural areas. LST has risen due to the government’s decision to convert agricultural and forest land into urban areas [ 38 , 69 ]. The government has relocated factories and businesses to the outskirts of cities to improve their efficiency. These facilities are typically found in developed areas. Before the development of urban areas, forests and vegetation were regarded as buffer zones between rural and urban areas, absorbing excess heat generated by factories and automobiles [ 3 , 29 , 40 ]. According to the scientific literature, the cooling effect of LUCC is well-matched to the expected warming effect caused by the physical interaction of the Indian region and its surroundings [ 32 ]. For example, the maximum cooling contribution from forested areas is 0.27%, while the minimum cooling effect is 0.06%. The most negligible difference between the surface temperature and the impervious surface is the primary reason why vegetation contributes the least to the cooling effect. The greatest cooling effect, on the other hand, is observed when forested areas are converted into water bodies. This is due to the fact that the contribution of land cover to cooling is negligible in various areas, such as urban areas, water bodies, and vegetation. The results of the study revealed that the built-up area in the southeastern and central port areas will continue growing. The paper discussed the various effects of the LUCC on the Tianjin city’s development. The study used the CA model and Geographic Information Systems to analyze the data. The results of the analysis helped improve the Tianjin city’s planning process. In addition, the paper discussed the use of remote sensing tools for improving the urban planning process.
5. Conclusions
The objective of this study was to analyze the influence of LUCC on land surface temperature (LST) in a large urban area of Tianjin. Data from RS were used to observe the area’s various socioeconomic and development parameters. The study also used the CA-Markov model and Pearson correlation coefficient to evaluate the contribution of landscape dynamics to temperature. A 5.94% increase in built-up area was found to increase the temperature by 1.5%. However, the increase in vegetation cover by 10% showed a negative correlation. In addition, the study concluded that LUCC has a cooling effect of about 1.40 °C in the city. The average warming effect of LUCC on the UHI is about 0.5%.
On the other hand, the cooling effect of LUCC compared to the shifts in the reverse direction is 0.11%. The positive contribution of LUCC to the UHI was higher than the negative one. Urban development and infrastructure planning should be further targeted to minimize the impacts of climate change. In addition to improving water bodies and parks, other measures, such as the establishment of green spaces and linear planting of woody plants, should also be implemented. The study found that further research is needed to analyze the impact of land use change on the climate of regions and cities. As more areas are affected by climate change, the government and private sector must work together to develop effective cooling strategies. Environmental education should be made accessible to promote the development of ecological resources. This needs effective urban planning and green policies to address the increasing thermal stress. In addition, a quantitative analysis of these parameters needs to be conducted. Although the study found that urbanization directly impacts land surface temperature, it is not yet clear how the effects of this process are related to the other factors. The practical application of the study provides essential guidance for urban landscape planning. It shows how landscape connectivity between impervious and green areas can affect LST. Future research should also address infrastructure stress and public health issues associated with rapid urbanization.
Acknowledgments
The authors wish to express his appreciation and gratitude to the anonymous reviewers and editors for their insightful comments and suggestions to improve the paper’s quality.
Funding Statement
Reconstructing the Architecture System based on the coherence mechanism of “Architecture-human-environment” in the Chinese context, Key project of National Natural Science Foundation of China, grant number 52038007, 2021-01-2025-12.
Author Contributions
Conceptualization, N.U., M.A.S., Y.Z. and Y.H.; data curation, N.U., M.A.S., M.D., S.G., S.T. and T.Z.; formal analysis, N.U., M.A.S., S.T., Z.K. and M.D.; funding acquisition, Y.H. and Y.Z.; methodology, N.U., M.A.S., S.T., I.A.K. and S.G.; project administration, Y.H. and Y.Z.; software, N.U. and M.A.S.; supervision, Y.H. and Y.Z.; visualization, N.U., M.A.S., T.Z., Z.K. and Y.H.; writing—original draft, N.U. and M.A.S.; writing—review and editing, N.U., M.A.S., I.A.K. and Y.H. All authors have read and agreed to the published version of the manuscript.
Institutional Review Board Statement
Not applicable.
Informed Consent Statement
Data availability statement, conflicts of interest.
The authors declare no conflict of interest.
Disclaimer/Publisher’s Note: The statements, opinions and data contained in all publications are solely those of the individual author(s) and contributor(s) and not of MDPI and/or the editor(s). MDPI and/or the editor(s) disclaim responsibility for any injury to people or property resulting from any ideas, methods, instructions or products referred to in the content.
Landscapes of Urban Thermal and Green Inequities: Insights into Australian Capital Cities’ Urban Heat Island and Green Infrastructure Accessibility
33 Pages Posted: 22 Aug 2024
Queensland University of Technology
Ruihong Jiao
Tan yigitcanlar, mirko guaralda.
Climate change has intensified urban heat islands (UHIs) in recent decades. While green infrastructure (GI) can mitigate UHIs, access to GI varies due to socioeconomic factors and location, leading to green inequity. This study addresses this gap by analysing UHI and green infrastructure accessibility (GIA) in these cities. It uses urban heat island intensity (UHII) to capture thermal inequity and network analysis to identify green inequity. A green infrastructure accessibility index (GIAI) is proposed alongside UHII to analyse their spatial relationship. Key findings reveal that: (a) UHII varies across capitals, with high UHII in urban and coastal zones of Sydney, sparse distribution in Darwin’s eastern rural areas, and extensive coverage in Melbourne, Perth, and Canberra. It is broadly distributed in Brisbane, Adelaide, and Hobart; (b) Urban areas have less GI but greater accessibility due to advanced road networks, while most cities offer 85% GI access within 20 minutes by car and over 60% within a 15-minute walk in urban areas; (c) Melbourne, Adelaide, and Perth have high GIAI despite sparse GI, while Sydney, Darwin, and Canberra show a clear urban-rural divide; (d) Sydney, Hobart, and Canberra exhibit balanced UHII and GIAI in both urban and rural areas.
Keywords: thermal inequity, green inequity, urban heat island, green infrastructure accessibility, climate change, Australia
Suggested Citation: Suggested Citation
Queensland University of Technology ( email )
2 George Street Brisbane, 4000 Australia
Tan Yigitcanlar (Contact Author)
2 George Street Brisbane, Queensland 4000 Australia
Do you have a job opening that you would like to promote on SSRN?
Paper statistics, related ejournals, urban economics & regional studies ejournal.
Subscribe to this fee journal for more curated articles on this topic
Urban & Regional Resilience eJournal
Emerging markets economics: environmental & social aspects ejournal, geography of human settlements, populations & movements ejournal.
Advertisement
Urban Heat Island studies: Current status in India and a comparison with the International studies
- Published: 04 March 2020
- Volume 129 , article number 85 , ( 2020 )
Cite this article
- K Veena 1 , 2 ,
- K M Parammasivam 1 &
- T N Venkatesh 2
2362 Accesses
43 Citations
8 Altmetric
Explore all metrics
Urbanization has resulted in many critical issues like increase in pollution levels, sudden climatic changes and the rise of temperature in the urban area, that is the formation of Urban Heat Islands (UHI). As the density of population rises, most of the land areas are being converted into cities and cities grows very rapidly. Due to the UHI effect, the cities are becoming hotter day by day. In India, all the metropolitan cities are victims of UHI effect and the severity of heat formation, necessitates research in this area. The present paper evaluates the trends of UHI studies in Indian cities and its out reach till 2018. Heat Island classification, methods of studying UHI in India and their limitation are discussed. Eventually a comparison of new trends of UHI studies in the world and where India lacks its growth in UHI research are included in this paper. One of the findings is that numerical modelling studies are very limited in India in this field and more focus in this area is required.
This is a preview of subscription content, log in via an institution to check access.
Access this article
Subscribe and save.
- Get 10 units per month
- Download Article/Chapter or eBook
- 1 Unit = 1 Article or 1 Chapter
- Cancel anytime
Price includes VAT (Russian Federation)
Instant access to the full article PDF.
Rent this article via DeepDyve
Institutional subscriptions
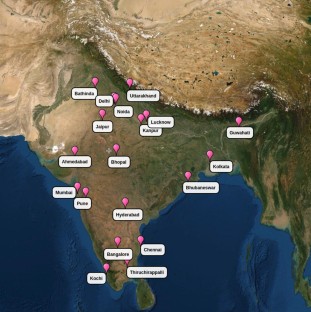
Similar content being viewed by others
Urban Heat Island in a Small Size Town in Northwestern Greece—A Comparison to Other Greek Areas
Urban Heat Island Simulation and Monitoring in the Hot and Humid Climate Cities of Guayaquil and Durán, Ecuador
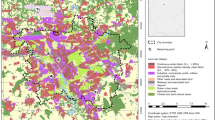
The urban heat island under extreme heat conditions: a case study of Hannover, Germany
Ahmad S, Matloob H, Warsi T R and Khan M D A 2011 The evaluation of heat island effect in various cities in Ganga–Yamuna Doab region using MODIS land surface temperature product; Asian J. Environ. Sci. 6(2) 185–190.
Google Scholar
Ali Gazi M A and Mondal I 2018 Urban Heat Island and its effect on Dweller of Kolkata Metropolitan area using geospatial techniques; Int. J. Comput. Sci. Eng. 6 741–753.
Ambinakudige S 2011 Remote sensing of land cover’s effect on surface temperatures: A case study of the urban heat Island in Bangalore, India; Applied GIS 7(1) 1–12.
Amirtham L R 2016 Urbanization and its impact on Urban Heat Island intensity in Chennai Metropolitan area India; Indian J. Sci. Technol. 9(5) .
Aslam M Y, Krishna K R, Beig G, Tinmaker M I R and Chate D M 2017 Seasonal variation of Urban Heat Island and its impact on air-quality using SAFAR observations at Delhi, India; Am. J. Clim. Change 6(02) 294–305.
Article Google Scholar
Babazadeh M and Kumar P 2015 Estimation of the Urban Heat Island in local climate change and vulnerability assessment for air quality in Delhi; Eur. Sci. J. , 55–65.
Baby M M and Arya G 2016 A Study of Urban Heat Island and its mapping; Int. J. Sci. Eng. Res. 4(3) 45–47.
Benali A, Carvalho A, Nunes J, Carvalhais N and Santos A 2012 Estimating air surface temperature in Portugal using MODIS LST data; Remote Sens. Environ., 108–121.
Blocken B 2015 Computational Fluid Dynamics for urban physics: Importance scales possibilities limitations and ten tips and tricks towards accurate and reliable simulations; Build. Environ., 1–27.
Borbora J and Das A K 2014 Summertime Urban Heat Island study for Guwahati City, India; Sustain. Cities Soc. 11 61–66.
Chakraborty T, Sarangi C and Tripathi S N 2016 Understanding diurnality and inter-seasonality of a sub-tropical Urban Heat Island; Bound.-Layer Meteorol. , https://doi.org/10.1007/s10546-016-0223-0 .
Deosthali V 2000 Impact of rapid urban growth on heat and moisture Islands in Pune City, India; Atmos. Environ. 34 2745–2754.
Devi S S 2006 Urban Heat Islands and environmental impact; 6th Symposium on the Urban Environment and Forum on Managing our Physical and Natural Resources.
Gopalakrishnan S G, Rama Krishna T V B P S and Sharan M 2003 Some signatures of Urban Heat Patches in southern India; Proc. Indian Natl. Sci. Acad. 5 603–614.
Goswami A, Mohammad P and Sattar A 2016 A temporal study of Urban Heat Island (UHI) – A evaluation of Ahmedabad city, Gujarat; International Conference on Climate Change Mitigation and Technologies for Adaptation, pp. 1–5.
Grover A and Singh R B 2015 Analysis of Urban Heat Island (UHI) in relation to Normalized Difference Vegetation Index (NDVI): A comparative study of Delhi and Mumbai; Environments 2 125–138.
Gupta A, Dey V and Goel D A 2009 Mitigation of thermal pollution to enhance urban air quality through Remote Sensing and GIS; 10th ESRI India User Conference.
Gupta R 2012 Temporal and spatial variations of Urban Heat Island effect in Jaipur City using satellite data; Environ. Urban. Asia 3(2) 359–374.
Jain G and Sarkar S 2017 Urban Heat Island: Causes effects and mitigating strategies; Imp. J. Interdiscip. Res. 3(5) 2098–2103.
Joshi R, Raval H, Pathak M, Prajapati S, Patel A, Singh V and Kalubarme M H 2015 Urban Heat Island characterization and isotherm mapping using geo-informatics technology in Ahmedabad City, Gujarat State, India; Int. J. Geosci. 6 274–285.
Kannamma D and Sundaram D A M 2015 Significance of microclimatic study in Urban Canyons towards ambient urban space design; J. Today’s Ideas – Tomorrow’s Technol. 3(1) 95–109.
Kikon N, Singh P, Singh S K and Vyas A 2016 Assessment of urban heat islands (UHI) of Noida City, India using multi-temporal satellite data; Sustain. Cities Soc. 22 19–28.
Kratzer A 1937 Das Stadtklima.
Kratzer A 1956 The Climate of Cities (Das Stadtklima).
Kumar R and Singh S 2016 Case study for change detection analysis and land surface temperature retrieval in Uttrakhand Region and their correlation; Imp. J. Interdiscip. Res. 2(9) 1821–1825.
Kumar K S, Udaya Bhaskar P and Kumari K P 2017a Assessment and mapping of urban heat island using field data in the new capital region of Andhra Pradesh, India; Indian J. Sci. Technol. 10(11) 1–8.
Kumar R, Mishra V, Buzan J, Kumar R, Shindell D and Huber M 2017b Dominant control of agriculture and irrigation on Urban Heat Island in India; Sci. Rep. , 1–10.
Landsberg H E 1981 The Urban Climate; Int. Geophys. , 28 .
Luke H 1818 The climate of London deduced from meteorological observations made at different places in the Neighbourhood of the Metropolis; 1p.
Marques Filho E P, Cassol M A, Karam H and Rizza U 2013 The vertical structure of Tropical Urban Heat Island with LES; Am. J. Environ. Eng. 3 24–31.
Mehrotra S 2018 Urban informal housing and surface Urban Heat Island intensity: Exploring spatial association in the city of Mumbai; Environ. Urban. Asia 9(2 ) 1–20.
Mills G 2008 Luke Howard and The Climate of London; Weather 63(6) 153–157.
Mills G 2009 Luke Howard, Tim Oke and the study of urban climates; Eighth Symposium on the Urban Environment.
Mills G 2014 Urban climatology: History, status and prospects; Urban Clim. 10 479–489.
Mirzaei P A and Haghighat F 2010 Approaches to study Urban Heat Island – Abilities and limitations; Build. Environ. 45 2192–2201.
Mohan M, Kandya A and Battiprolu A 2011 Urban Heat Island Effect over national capital region of India: A study using the temperature trends; J. Environ. Prot. 2 465–472.
Mohan M, Kikegawa Y, Gurjar B R, Bhati S and Reddy Kolli N 2013 Assessment of Urban Heat Island effect for different land use–land cover from micrometeorological measurements and remote sensing data for megacity Delhi; Theor. Appl. Climatol . 112 647–658.
Mohan M, Kikegawa Y, Gurjar B R, Bhati S, Kandya A and Ogawa K 2012 Urban Heat Island assessment for a Tropical Urban Airshed in India; Atmos. Clim. Sci. 2 127–138.
Nakata-osaki C M, Lucas de Souza L C and Rodrigues D S 2015 A GIS extension model to calculate Urban Heat Island intensity based on urban geometry; Comput. Urban. Plan. Urban. Manag ., 1–16.
Nesarikar-Patki P and Raykar-Alange P 2012 Study of inuence of land cover on Urban Heat Islands in Pune using remote sensing; IOSR J. Mech. Civil Eng. 3 39–43.
Oke T R 1968 Sustainable urban development: Minimising Urban Heat Island effect and imperviousness factor; pp. 1–20.
Oke T R 1973 City size and the Urban Heat Island; Atmos. Environ. 7 769–779.
Oke T R 2006 Towards better scientific communication in urban climate; Theor. Appl. Climatol. 84 179–190.
Oke T R, Mills G, Christen A and Voogt J A 2017 Urban Climates.
Pandey P, Kumar D, Prakash A, Masih J, Singh M, Kumar S, Jain V K and Kumar K 2012 A study of Urban Heat Island and its association with particulate matter during winter months over Delhi; Sci. Total Environ. 414 494–507.
Ramachandra T V and Kumar U 2010 Greater Bangalore: Emerging Urban Heat Island; GIS Develop. 14(1) 1–16.
Sahu S, Pawar A and Mukherjee M 2014 Urban Heat Island investigation: Techniques and methods so far; Architecture – Time Space & People , pp. 16–22.
Sastry M, Majumder M, Kumar P and Kiran Kumar D E V S 2013 Sustainable urban development: Minimising Urban Heat Island effect and imperviousness factor; Sanei Working Paper Series, 13–03.
Sharma D and Bharat A 2009 Urban Heat Island effect – causes impacts methods of measurement and mitigation options; Institute of Town Planners, India 6(2) 69–77.
Sharma N and Pandey P 2015 Study of Urban Heat Island in Bathinda City, Punjab; 16th ESRI India User Conference, pp. 1–14.
Shastri H, Barik B, Ghosh S, Venkataraman C and Sadavarte P 2017 Flip flop of Day–night and Summer–winter Surface Urban Heat Island intensity in India; Sci. Rep. – Nature 1–8.
Singh P and Kikon Noyingbeni Verma P 2017 Impact of land use change and urbanization on Urban Heat Island in Lucknow city, Central India: A remote sensing based estimate; Sustain. Cities Soc. 32 100–114.
Swain D, Roberts G J, Dash J, Vinoj V, Lekshmi K and Tripathy S 2016 Impact of rapid urbanization on the microclimate of Indian cities: A case study for the city of Bhubaneswar; Proc. SPIE 9877 1–7.
Thomas G, Sherin A P, Ansar S and Zachariah E J 2014 Analysis of Urban Heat Island in Kochi, India, using a modified local climate zone classification; Procedia Environ. Sci. 21 3–13.
Toparlar Y, Blocken B, Maiheu B and van Heijst G 2017 A review on the CFD analysis of urban microclimate; Renew. Sust. Energ. Rev., 1–28.
Vogt J V, Viau A A and Paquet P F 1997 Mapping regional air temperature fields using satellite-derived surface skin temperature; Int. J. Climatol. , 1559–1579.
Zhang N, Wang X and Peng Z 2013 Large-Eddy simulation of mesoscale circulations forced by inhomogeneous Urban Heat Island; Bound.-Layer Meteorol. , 179–194.
Download references
Acknowledgement
The first author is financially supported by CSIR (Grant No. Ack.No.141410/2K15/1) through Direct SRF Scheme and it is gratefully acknowledged.
Author information
Authors and affiliations.
Madras Institute of Technology, Chennai, 600 044, India
K Veena & K M Parammasivam
CTFD Division, CSIR-National Aerospace Laboratories, Bengaluru, 560 017, India
K Veena & T N Venkatesh
You can also search for this author in PubMed Google Scholar
Corresponding author
Correspondence to K Veena .
Additional information
Communicated by Amit Kumar Patra
Rights and permissions
Reprints and permissions
About this article
Veena, K., Parammasivam, K.M. & Venkatesh, T.N. Urban Heat Island studies: Current status in India and a comparison with the International studies. J Earth Syst Sci 129 , 85 (2020). https://doi.org/10.1007/s12040-020-1351-y
Download citation
Received : 20 May 2019
Revised : 17 October 2019
Accepted : 14 December 2019
Published : 04 March 2020
DOI : https://doi.org/10.1007/s12040-020-1351-y
Share this article
Anyone you share the following link with will be able to read this content:
Sorry, a shareable link is not currently available for this article.
Provided by the Springer Nature SharedIt content-sharing initiative
- Urbanization
- Urban Heat Islands
- numerical modelling
- Find a journal
- Publish with us
- Track your research
- A-Z Publications
Annual Review of Environment and Resources
Volume 40, 2015, review article, urban heat island: mechanisms, implications, and possible remedies.
- Patrick E. Phelan 1 , Kamil Kaloush 2 , Mark Miner 1 , Jay Golden 3 , Bernadette Phelan 4 , Humberto Silva III 5 , and Robert A. Taylor 6
- View Affiliations Hide Affiliations Affiliations: 1 School for Engineering of Matter, Transport & Energy, Arizona State University, Tempe, Arizona 85287; email: [email protected] , [email protected] 2 School of Sustainable Engineering and the Built Environment, Arizona State University, Tempe, Arizona 85281; email: [email protected] 3 Division of Earth and Ocean Sciences, Nicholas School of the Environment and Pratt School of Engineering, Duke University, Durham, North Carolina 27708; email: [email protected] 4 Synectics for Management Decisions, Inc., Arlington, Virginia 22209; email: [email protected] 5 Mechanical Engineering Department, University of New Mexico, Albuquerque, New Mexico 87131; email: [email protected] 6 School of Mechanical and Manufacturing Engineering and the School of Photovoltaic and Renewable Energy Engineering, University of New South Wales, NSW 2052, Australia; email: [email protected]
- Vol. 40:285-307 (Volume publication date November 2015) https://doi.org/10.1146/annurev-environ-102014-021155
- First published as a Review in Advance on September 02, 2015
- © Annual Reviews
Urban heat island (UHI) manifests as the temperature rise in built-up urban areas relative to the surrounding rural countryside, largely because of the relatively greater proportion of incident solar energy that is absorbed and stored by man-made materials. The direct impact of UHI can be significant on both daytime and night-time temperatures, and the indirect impacts include increased air conditioning loads, deteriorated air and water quality, reduced pavement lifetimes, and exacerbated heat waves. Modifying the thermal properties and emissivity of roofs and paved surfaces and increasing the vegetated area within the city are potential mitigation strategies. A quantitative comparison of their efficacies and costs suggests that so-called cool roofs are likely the most cost-effective UHI mitigation strategy. However, additional research is needed on how to modify surface emissivities and dynamically control surface and material properties, as well as on the health and socioeconomic impacts of UHI.
Article metrics loading...
Full text loading...
Literature Cited
- Fragkias M , Güneralp B , Seto K , Goodness J . 1. 2013 . Urbanization, biodiversity and ecosystem services: challenges and opportunities. Urbanization, Biodiversity and Ecosystem Services: Challenges and Opportunities: A Global Assessment T Elmqvist, M Fragkias, J Goodness, B Güneralp, P Marcotullio, R McDonald, C Wilkinson 409– 35 Dordrecht, Netherlands: Springer [Google Scholar]
- Clinton N , Gong P . 2. 2013 . MODIS detected surface urban heat islands and sinks: global locations and controls. Remote Sensing Environ. 134 : 294– 304 [Google Scholar]
- Tran H , Uchihama D , Ochi S , Yasuoka Y . 3. 2006 . Assessment with satellite data of the urban heat island effects in Asian mega cities. Int. J. Appl. Earth Observation Geoinf. 8 : 1 34– 48 [Google Scholar]
- Mohan M , Kikegawa Y , Gurjar B , Bhati S , Kolli N . 4. 2012 . Assessment of urban heat island effect for different land use–land cover from micrometeorological measurements and remote sensing data for megacity Delhi. Theor. Appl. Climatol. 112 : 3–4 647– 58 [Google Scholar]
- Gaffin S , Rosenzweig C , Khanbilvardi R , Parshall L , Mahani S . 5. et al. 2008 . Variations in New York City's urban heat island strength over time and space. Theor. Appl. Climatol. 94 : 1–2 1– 11 [Google Scholar]
- Kolokotroni M , Giridharan R . 6. 2008 . Urban heat island intensity in London: an investigation of the impact of physical characteristics on changes in outdoor air temperature during summer. Solar Energy 82 : 11 986– 98 [Google Scholar]
- Kolokotsa D , Santamouris M , Zerefos S . 7. 2013 . Green and cool roofs' urban heat island mitigation potential in European climates for office buildings under free floating conditions. Solar Energy 95 : 118– 30 [Google Scholar]
- Gallo K , Xian G . 8. 2014 . Application of spatially gridded temperature and land cover data sets for urban heat island analysis. Urban Climate 8 : 1– 10 [Google Scholar]
- Kardinal Jusuf S , Wong N , Hagen E , Anggoro R , Hong Y . 9. 2007 . The influence of land use on the urban heat island in Singapore. Habitat Int. 31 : 2 232– 42 [Google Scholar]
- Sharifi E , Lehmann S . 10. 2014 . Comparative analysis of surface urban heat island effect in central Sydney. J. Sustainable Dev. 7 : 3 23– 34 [Google Scholar]
- Weng Q , Fu P . 11. 2014 . Modeling diurnal land temperature cycles over Los Angeles using downscaled GOES imagery. ISPRS J. Photogrammetry Remote Sensing 97 : 78– 88 [Google Scholar]
- Sarkar A , De Ridder K . 12. 2010 . The urban heat island intensity of Paris: a case study based on a simple urban surface parametrization. Boundary-Layer Meteorol. 138 : 3 511– 20 [Google Scholar]
- Otanicar T , Carlson J , Golden J , Kaloush K , Phelan P . 13. 2010 . Impact of the urban heat island on light duty vehicle emissions for the Phoenix, AZ area. Int. J. Sustain. Transport. 4 : 1 1– 13 [Google Scholar]
- Giannaros T , Melas D , Daglis I , Keramitsoglou I , Kourtidis K . 14. 2013 . Numerical study of the urban heat island over Athens (Greece) with the WRF model. Atmos. Environ. 73 : 103– 11 [Google Scholar]
- Scott C , Halper E , Yool S , Comrie A . 15. 2009 . The evolution of urban heat island and water demand. Proc. Am. Meteorol. Soc. Symp. Urban Environ., 8th Phoenix, AZ [Google Scholar]
- Memon R , Leung D , Chunho L . 16. 2008 . A review on the generation, determination and mitigation of urban heat island. J. Environ. Sci. 20 : 1 120– 28 [Google Scholar]
- Schwarz N , Schlink U , Franck U , Großmann K . 17. 2012 . Relationship of land surface and air temperatures and its implications for quantifying urban heat island indicators—an application for the city of Leipzig (Germany). Ecol. Indicators 18 : 693– 704 [Google Scholar]
- Zhang H , Qi Z , Ye X , Cai Y , Ma W , Chen M . 18. 2013 . Analysis of land use/land cover change, population shift, and their effects on spatiotemporal patterns of urban heat islands in metropolitan Shanghai, China. Appl. Geogr. 44 : 121– 33 [Google Scholar]
- Tan J , Zheng Y , Tang X , Guo C , Li L . 19. et al. 2010 . The urban heat island and its impact on heat waves and human health in Shanghai. Int. J. Biometeorol. 54 : 1 75– 84 [Google Scholar]
- Adamowski J , Prokoph A . 20. 2013 . Assessing the impacts of the urban heat island effect on streamflow patterns in Ottawa, Canada. J. Hydrol. 496 : 225– 37 [Google Scholar]
- Radhi H , Fikry F , Sharples S . 21. 2013 . Impacts of urbanisation on the thermal behaviour of new built up environments: a scoping study of the urban heat island in Bahrain. Landscape Urban Plan. 113 : 47– 61 [Google Scholar]
- Kang H-Q , Zhu B , Zhu T , Sun J-L , Ou J-J . 22. 2014 . Impact of megacity Shanghai on the urban heat-island effects over the downstream city Kunshan. Boundary-Layer Meteorol. 152 : 3 411– 26 [Google Scholar]
- Santamouris M , Cartalis C , Synnefa A , Kolokotsa D . 23. 2015 . On the impact of urban heat island and global warming on the power demand and electricity consumption of buildings—a review. Energy Build. 98 : 119– 24 [Google Scholar]
- Solecki W , Rosenzweig C , Parshall L , Pope G , Clark M . 24. et al. 2005 . Mitigation of the heat island effect in urban New Jersey. Environ. Hazards 6 : 1 39– 49 [Google Scholar]
- Silva H , Phelan P , Golden J . 25. 2010 . Modeling effects of urban heat island mitigation strategies on heat-related morbidity: a case study for Phoenix, Arizona, USA. Int. J. Biometeorol. 54 : 1 13– 22 [Google Scholar]
- Gago E , Roldan J , Pacheco-Torres R , Ordóñez J . 26. 2013 . The city and urban heat islands: a review of strategies to mitigate adverse effects. Renewable Sustainable Energy Rev. 25 : 749– 58 [Google Scholar]
- Santamouris M . 27. 2013 . Using cool pavements as a mitigation strategy to fight urban heat island—a review of the actual developments. Renewable Sustain. Energy Rev. 26 : 224– 40 [Google Scholar]
- Alchapar N , Correa E , Cantón M . 28. 2014 . Classification of building materials used in the urban envelopes according to their capacity for mitigation of the urban heat island in semiarid zones. Energy Build. 69 : 22– 32 [Google Scholar]
- Yang J , Wang Z , Kaloush K . 29. 2014 . Unintended consequences: a research synthesis examining the use of reflective pavements to mitigate the urban heat island effect White Pap., Natl. Cent. Excell. Smart Innov., Arizona State Univ. https://ncesmart.asu.edu/docs/smart/unintended-consequences-1013.pdf [Google Scholar]
- Oke T . 30. 1988 . The urban energy balance. Prog. Phys. Geogr. 12 : 4 471– 508 [Google Scholar]
- Mirzaei P , Haghighat F . 31. 2010 . Approaches to study urban heat island—abilities and limitations. Build. Environ. 45 : 2192– 201 [Google Scholar]
- Silva H , Bhardwaj R , Phelan P , Golden J , Grossman-Clarke S . 32. 2009 . Development of a zero-dimensional mesoscale thermal model for urban climate. J. Appl. Meteorol. Climatol. 48 : 657– 68 [Google Scholar]
- Sailor D , Lu L . 33. 2004 . A top-down methodology for developing diurnal and seasonal anthropogenic heating profiles for urban areas. Atmos. Environ. 38 : 2737– 48 [Google Scholar]
- Hinkel K , Nelson F , Klene A , Bell J . 34. 2003 . The urban heat island in winter at Barrow, Alaska. Int. J. Climatol. 23 : 1889– 905 [Google Scholar]
- Silva H , Golden J . 35. 2012 . Spatial superposition method via model coupling for urban heat island albedo mitigation strategies. J. Appl. Meteorol. Climatol. 51 : 1971– 79 [Google Scholar]
- Lee T-W , Choi H , Lee J . 36. 2014 . Generalized scaling of urban heat island effect and its applications for energy consumption and renewable energy. Adv. Meteorol. 2014 : 948306 [Google Scholar]
- Hu Z , Yu B , Chen Z , Li T , Liu M . 37. 2012 . Numerical investigation on the urban heat island in an entire city with an urban porous media model. Atmos. Environ. 47 : 509– 18 [Google Scholar]
- Hoffmann P , Krueger O , Heinke Schlunzen K . 38. 2012 . A statistical model for the urban heat island and its application to a climate change scenario. Int. J. Climatol. 32 : 1238– 48 [Google Scholar]
- Sailor D , Dietsch N . 39. 2007 . The urban heat island Mitigation Impact Screening Tool (MIST). Environ. Model. Softw. 22 : 1529– 41 [Google Scholar]
- Chen F , Yang X , Zhu W . 40. 2014 . WRF simulations of urban heat island under hot-weather synoptic conditions: the case study of Hangzhou City, China. Atmospheric Res. 138 : 364– 377 [Google Scholar]
- Kaloush KE , Wang Z , Yang J . 41. 2015 . Examining the use of reflective pavements to mitigate the urban heat island effect. Asphalt Pavement Magazine http://www.nxtbook.com/naylor/NAPS/NAPS0115/index.php?startid=44 [Google Scholar]
- 42. Energy Information Administration 2014 . Annual Energy Outlook 2014 with Projections to 2040 Washington, DC: US Dep. Energy http://www.eia.gov/forecasts/aeo/er/index.cfm [Google Scholar]
- Werner S . 43. 2006 . Ecoheatcool work package 1: the European heat market Final Rep., Eur. Heat Water, Eur. Union, Bruss., Belg. [Google Scholar]
- Howard L . 44. 1833 . Climate of London, Deduced from Meteorological Observations, Made in the Metropolis and at Various Places Around It. London: Int. Assoc. Urban Climate [Google Scholar]
- Wouters H , De Ridder K , Demuzere M , Lauwaet D , van Lipzig N . 45. 2006 . The diurnal evolution of the urban heat island of Paris: a model-based case study during summer 2006. Atmospheric Chem. Phys. 13 : 17 8525– 41 [Google Scholar]
- Stathopoulou M , Caralis C , Andritsos A . 46. 2005 . Assessing the thermal environment of major cities in Greece Presented at Int. Conf. Passive Low Energy Cooling Built Environ., 1st, Santorini, Greece [Google Scholar]
- Yang P , Ren G , Liu W . 47. 2013 . Spatial and temporal characteristics of Beijing urban heat island intensity. J. Appl. Meteorol. Climatol. 52 : 8 1803– 16 [Google Scholar]
- Brazel A , Selover N , Vose R , Heiser G . 48. 2000 . The tale of two climates—Baltimore and Phoenix urban LTER sites. Climate Res. 15 : 123– 35 [Google Scholar]
- Gedzelman S , Austin S , Cermak R , Stefano N , Partridge S , Quesenberry S . 49. 2003 . Mesoscale aspects of the urban heat island around New York City. Theor. Appl. Climatol. 75 : 1–2 29– 42 [Google Scholar]
- Rosenthal J , Sastre Pena M , Rosenzweig C , Knowlton K , Goldberg R , Kinney P . 50. 2003 . One hundred years of New York City's “urban heat island”: temperature trends and public health impacts Abstract #U32A-0030, Am Geophys. Union [Google Scholar]
- Rong F . 51. 2006 . Impact of urban sprawl on U.S. residential energy use. PhD Thesis, Univ. Md. http://drum.lib.umd.edu/bitstream/1903/3848/1/umi-umd-3694.pdf [Google Scholar]
- Giridharan R , Kolokotroni M . 52. 2009 . Urban heat island characteristics in London during winter. Solar Energy 83 : 1668– 82 [Google Scholar]
- Kolokotroni M , Ren X , Davies M , Mavrogianni A . 53. 2012 . London's urban heat island: impact on current and future energy consumption in office buildings. Energy Build. 47 : 302– 11 [Google Scholar]
- Golden J , Brazel A , Salmond J , Laws D . 54. 2006 . Energy and water sustainability: the role of urban climate change from metropolitan infrastructure. J. Green Build. 1 : 3 124– 38 [Google Scholar]
- Yaghoobian N , Kleissl J , Krayenhoff E . 55. 2010 . Modeling the thermal effects of artificial turf on the urban environment. J. Appl. Meteor. Climatol. 49 : 3 332– 45 [Google Scholar]
- Yaghoobian N , Kleissl J . 56. 2012 . Effect of reflective pavements on building energy use. Urban Climate 2 : 25– 42 [Google Scholar]
- 57. United Nations 2014 . Coping with Water Scarcity: Challenge of the Twenty-First Century: 22 March 2007 New York: United Nations http://www.unwater.org/downloads/escarcity.pdf [Google Scholar]
- Erickson A , Weiss P , Gulliver J . 58. 2013 . Optimizing Stormwater Treatment Practices: A Handbook of Assessment and Maintenance New York: Springer [Google Scholar]
- Solimini AG , Cardoso AC , Heiskanen A-S . 59. 2007 . Indicators and Methods for the Ecological Status Assessment Under the Water Framework Directive: Linkages Between Chemical and Biological Quality of Surface Waters Bruss., Belg.: Eur. Comm. Eur. Union [Google Scholar]
- Butterwick C , Heaney S , Talling J . 60. 2005 . Diversity in the influence of temperature on the growth rates of freshwater algae, and its ecological relevance. Freshwater Biol. 50 : 291– 300 [Google Scholar]
- Van Buren M , Watt W , Marsalek J , Anderson B . 61. 2000 . Thermal enrichment stormwater runoff by paved surfaces. Water Res. 34 : 4 1359– 137 [Google Scholar]
- Winston R , Hunt W , Lord W . 62. 2011 . Thermal mitigation of urban storm water by level spreader-vegetative filter strips. J. Environ. Eng. 137 : 707– 16 [Google Scholar]
- Muller N , Kuttler W , Barlag A . 63. 2014 . Analysis of the subsurface urban heat islands in Oberhausen, Germany. Climate Res. 58 : 247– 56 [Google Scholar]
- Santamouris M . 64. 2014 . On the energy impact of urban heat island and global warming on buildings. Energy Build. 82 : 100– 13 [Google Scholar]
- Torcellini P , Long N , Judkoff R . 65. 2003 . Consumptive water use for US power production. Tech. Rep. NREL/TP-550-33905, Nat. Renew. Energy Lab., Golden, Colo. [Google Scholar]
- 66. US Geological Survey (USGS) 2014 . Thermoelectric Power Water Use: Water Use in the United States. Washington, DC: USGS http://water.usgs.gov/watuse/wupt.html [Google Scholar]
- Spellman F . 67. 2014 . Handbook of Water and Wastewater Treatment Plant Operations Boca Raton, FL: CRC Press [Google Scholar]
- Guhathakurta S , Gober P . 68. 2007 . The impact of the Phoenix urban heat island on residential water use. J. Am. Plann. Assoc. 73 : 3 317– 29 [Google Scholar]
- Aggarwal R , Guhathakurta S , Grossman-Clarke S , Lathey V . 69. 2012 . How do variations in the urban heat islands in space and time influence household water use? The case of Phoenix, Arizona. Water Resour. Res. 48 : W06518 [Google Scholar]
- Kambezidis H , Peppes A , Melas D . 70. 1995 . An environmental experiment over Athens urban area under sea breeze conditions. Atmos. Res. 36 : 139– 56 [Google Scholar]
- Menut L , Vautard R , Flamant C . 71. 2000 . Measurements and modeling of atmospheric pollution over the Paris area: an overview of the ESQUIF project. Ann. Geophys. 18 : 11 1467– 81 [Google Scholar]
- Cros B , Durand P , Cachier H . 72. 2004 . An overview of the ESCOMPTE campaign. Atmos. Res. 69 : 3–4 241– 79 [Google Scholar]
- Lai L , Cheng W . 73. 2009 . Air quality influenced by urban heat island coupled with synoptic weather patterns. Sci. Total Environ. 407 : 8 2724– 33 [Google Scholar]
- Akbari H . 74. 2005 . Energy saving potentials and air quality benefits of urban heat island mitigation Rep. LBNL–58285, US. Dep. Energy. http://www.osti.gov/scitech/servlets/purl/860475 [Google Scholar]
- Wilby R . 75. 2008 . Constructing climate change scenarios of urban heat island intensity and air quality. Environ. Plan. B 35 : 902– 19 [Google Scholar]
- Bornstein R , Lin Q . 76. 2000 . Urban heat islands and summertime convective thunderstorms in Atlanta: three case studies. Atmos. Environ. 34 : 507– 16 [Google Scholar]
- Bornstein R , LeRoy M . 77. 1990 . Urban barrier effects on convective and frontal thunderstorms. Fourth AMS Conference on Mesoscale Processes AMS 25– 29 Boulder, CO: AMS [Google Scholar]
- Parker D . 78. 2010 . Urban heat island effects on estimates of observed climate change. WIREs Clim. Change 1 : 123– 33 [Google Scholar]
- Trenberth K , Jones P , Ambenje P , Bojariu R , Easterling D . 79. 2007 . Observations: surface and atmospheric climate change. Climate Change 2007: The Physical Science Basis S Solomon, D Qin, M Manning, Z Chen, M Marquis, KB Averyt 235– 36 Cambridge, MA: Cambridge Univ. Press [Google Scholar]
- Bowler D , Buyung-Ali L , Knight T , Pullin A . 80. 2010 . Urban greening to cool towns and cities: a systematic review of the empirical evidence. Landscape Urban Plan. 97 : 147– 55 [Google Scholar]
- Shashua-Bar L , Pearlmutter D , Erell E . 81. 2009 . The cooling efficiency of urban landscape strategies in a hot dry climate. Landscape Urban Plan. 92 : 179– 86 [Google Scholar]
- Cooney CM . 82. 2011 . Preparing a people: climate change and public health. Environ. Health Perspect. 119 : 166– 71 [Google Scholar]
- Jessup C , Balbus J , Christian C , Haque E , Howe S . 83. et al. 2013 . Climate change, human health, and biomedical research: analysis of the national institutes of health research portfolio: commentary. Environ. Health Perspect. 399– 404 [Google Scholar]
- Chuang W-C , Gober P , Chow W , Golden J . 84. 2013 . Sensitivity to heat: a comparative study of Phoenix, Arizona and Chicago, Illinois: 2003–2006. Urban Climate 5 : 1– 18 [Google Scholar]
- Changnon S , Kunkel K , Reinke B . 85. 1996 . Impacts and responses to the 1995 heat wave: a call to action. Bull. Am. Meteorol. Soc. 77 : 1497– 506 [Google Scholar]
- D'Amato G , Baena-Cagnani C , Cecchi L , Annesi-Maesano I , Nunes C . 86. et al. 2013 . Climate change, air pollution and extreme events leading to increasing prevalence of allergic respiratory diseases. Multidiscip. Respir. Med. 8 : 12 [Google Scholar]
- Nigel Bark M . 87. 1998 . Deaths of psychiatric patients during heat waves. Psychiatr. Serv. 1088– 90 [Google Scholar]
- Chand P , Murthy P . 88. 2008 . Climate change and mental health. Reg. Health Forum 12 : 43– 48 [Google Scholar]
- Lin S , Hsu W-H , Van Zutphen A , Saha S , Luber G , Hwang S-A . 89. 2012 . Excessive heat and respiratory hospitalizations in New York State: estimating current and future public health burden related to climate change. Environ. Health Perspect. 120 : 1571– 77 [Google Scholar]
- Uejio C , Wilhelmi O , Golden J , Mills D , Gulino S . 90. 2011 . Intra-urban societal vulnerability to extreme heat: the role of heat exposure and the built environment, socioeconomics, and neighborhood stability. Health Place. 17 : 498– 507 [Google Scholar]
- Golden J , Hartz D , Brazel A , Luber G , Phelan P . 91. 2008 . A biometeorology study of climate and heat-related morbidity in Phoenix from 2001 to 2006. Int. J. Biometeorol. 52 : 471– 80 [Google Scholar]
- Silva H , Phelan P , Golden J . 92. 2010 . Modeling effects of urban heat island mitigation strategies: a case study for Phoenix, Arizona, USA. Int. J. Biometeorol. 54 : 13– 22 [Google Scholar]
- Anenberg S , Schwartz J , Shindell D , Amann M , Faluvegi G . 93. et al. 2012 . Global air quality and health co-benefits of mitigating near-term climate change through methane and black carbon emission controls. Environ. Health Perspect. 120 : 831– 39 [Google Scholar]
- Lwasa S , Mugagga F , Wahab B , Simon D . 94. 2013 . Urban and peri-urban agriculture and forestry: transcending poverty alleviation to climate change mitigation and adaptation. Urban Climate 7 : 92– 106 [Google Scholar]
- McMichael C , Barnett J , McMichael A . 95. 2012 . An Ill wind? Climate change, migration, and health. Environ. Health Perspect. 120 : 646– 54 [Google Scholar]
- Juhola S , Driscoll P , de Suarez J , Suarez P . 96. 2013 . Social strategy games in communicating trade-offs. Urban Climate 4 : 102– 16 [Google Scholar]
- Boumans R , Phillips D , Victery W , Fontaine T . 97. 2014 . Developing a model for effects of climate change on human health and health–environment interactions: heat stress in Austin, Texas. Urban Climate 8 : 78– 99 [Google Scholar]
- Akbari H , Konopacki S . 98. 2005 . Calculating energy-saving potentials of heat-island reduction strategies. Energy Policy 33 : 721– 56 [Google Scholar]
- 99. US Energy Information Administration 2015 . Annual Energy Outlook 2015 Washington, DC: US Dep. Energy http://www.eia.gov/forecasts/aeo/ [Google Scholar]
- Rosenfeld A , Akbari H , Bretz S , Fishman B , Kurn D . 100. et al. 1995 . Mitigation of urban heat islands: materials, utility programs, updates. Energy Build. 22 : 255– 65 [Google Scholar]
- Rosenzweig C , Solecki W , Parshall L , Gaffin S , Lynn B . 101. et al. 2006 . Mitigating New York City's heat island with urban forestry, living roofs, and light surfaces Rep. 06-06, N.Y. State Energy Res. Dev. Authority. http://www.giss.nasa.gov/research/news/20060130/103341.pdf [Google Scholar]
- Taha H . 102. 1997 . Urban climates and heat islands: albedo, evapotranspiration, and anthropogenic heat. Energy Build. 25 : 99– 103 [Google Scholar]
- Akbari H , Matthews H . 103. 2012 . Global cooling updates: reflective roofs and pavements. Energy Build. 55 : 2– 6 [Google Scholar]
- Pompeii II W , Hawkins T . 104. 2011 . Assessing the impact of green roofs on urban heat island mitigation: a hardware scale modeling approach. Geogr. Bull. 52 : 52– 61 [Google Scholar]
- Lee J , Kim J , Lee M . 105. 2014 . Mitigation of urban heat island effect and green roofs. Indoor Built. Environ. 23 : 1 62– 69 [Google Scholar]
- Takebayashi H , Moriyama M . 106. 2007 . Surface heat budget on green roof and high reflection roof for mitigation of urban heat island. Build. Environ. 42 : 2971– 79 [Google Scholar]
- Karlessi T , Santamouris M , Apostolakis K , Synnefa A , Livada I . 107. 2009 . Development and testing of thermochromic coatings for buildings and urban structures. Solar Energy 83 : 538– 51 [Google Scholar]
- Balling RJ , Brazel S . 108. 1987 . Time and space characteristics of the Phoenix urban heat island. J. Ariz. Nev. Acad. Sci. 21 : 2 75– 81 [Google Scholar]
- Gui J , Phelan P , Kaloush K , Golden J . 109. 2007 . Impact of pavement thermophysical properties on surface temperatures. J. Mater. Civ. Eng. 19 : 8 683– 90 [Google Scholar]
- Rephaeli E , Raman A , Fan S . 110. 2013 . Ultrabroadband photonic structures to achieve high-performance daytime radiative cooling. Nano Lett. 13 : 1457– 61 [Google Scholar]
- Golden J , Carlson J , Kaloush K , Phelan P . 111. 2007 . A comparative study of the thermal and radiative impacts of photovoltaic canopies on pavement surface temperatures. Solar Energy 81 : 872– 83 [Google Scholar]
- Steeneveld G , Koopmans S , Heusinkveld B , Theeuwes N . 112. 2014 . Refreshing the role of open water surfaces on mitigating the maximum urban heat island effect. Landscape Urban Plan. 121 : 92– 96 [Google Scholar]
- Rossi F , Pisello A , Nicolini A , Filipponi M , Palombo M . 113. 2014 . Analysis of retro-reflective surfaces for urban heat island mitigation: a new analytical model. Appl. Energy 114 : 621– 31 [Google Scholar]
- Golden JS . 114. 2014 . The urban heat island—a sustainable systems complexity. White Pap., Center Sustain. Comm., Duke Univ. http://center.sustainability.duke.edu/reports [Google Scholar]
Data & Media loading...
- Article Type: Review Article
Most Read This Month
Most cited most cited rss feed, adaptive governance of social-ecological systems, dynamics of land-use and land-cover change in tropical regions, climate change and food systems, wetland resources: status, trends, ecosystem services, and restorability, global water pollution and human health, adaptation to environmental change: contributions of a resilience framework, i ndustrial s ymbiosis : literature and taxonomy, environmental governance, c arbon d ioxide e missions from the g lobal c ement i ndustry 1, global biogeochemical cycling of mercury: a review.
Academia.edu no longer supports Internet Explorer.
To browse Academia.edu and the wider internet faster and more securely, please take a few seconds to upgrade your browser .
- We're Hiring!
- Help Center
Urban heat island
- Most Cited Papers
- Most Downloaded Papers
- Newest Papers
- Last »
- Cool Roofs Follow Following
- Waste Generation Calculation Follow Following
- Thermal comfort Follow Following
- Climatology Follow Following
- Urban Heat Island Effect Follow Following
- Satellite Data Follow Following
- Urban Climate Follow Following
- Landsat TM Follow Following
- Atmospheric sciences Follow Following
- Identification of Urban Heat Islands Follow Following
Enter the email address you signed up with and we'll email you a reset link.
- Academia.edu Journals
- We're Hiring!
- Help Center
- Find new research papers in:
- Health Sciences
- Earth Sciences
- Cognitive Science
- Mathematics
- Computer Science
- Academia ©2024
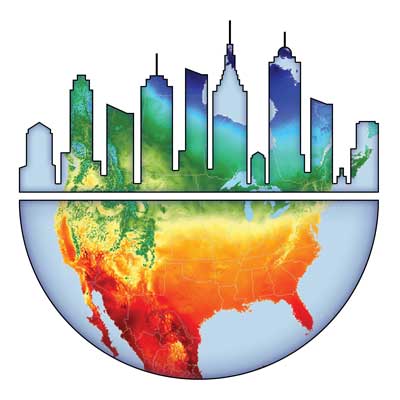
< Return to Highlights
April 01, 2024
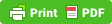
Exploring Urban Heat Islands and Temperature Inequality in U.S. Cities
Using satellite data to understand how city design impacts temperature variations.
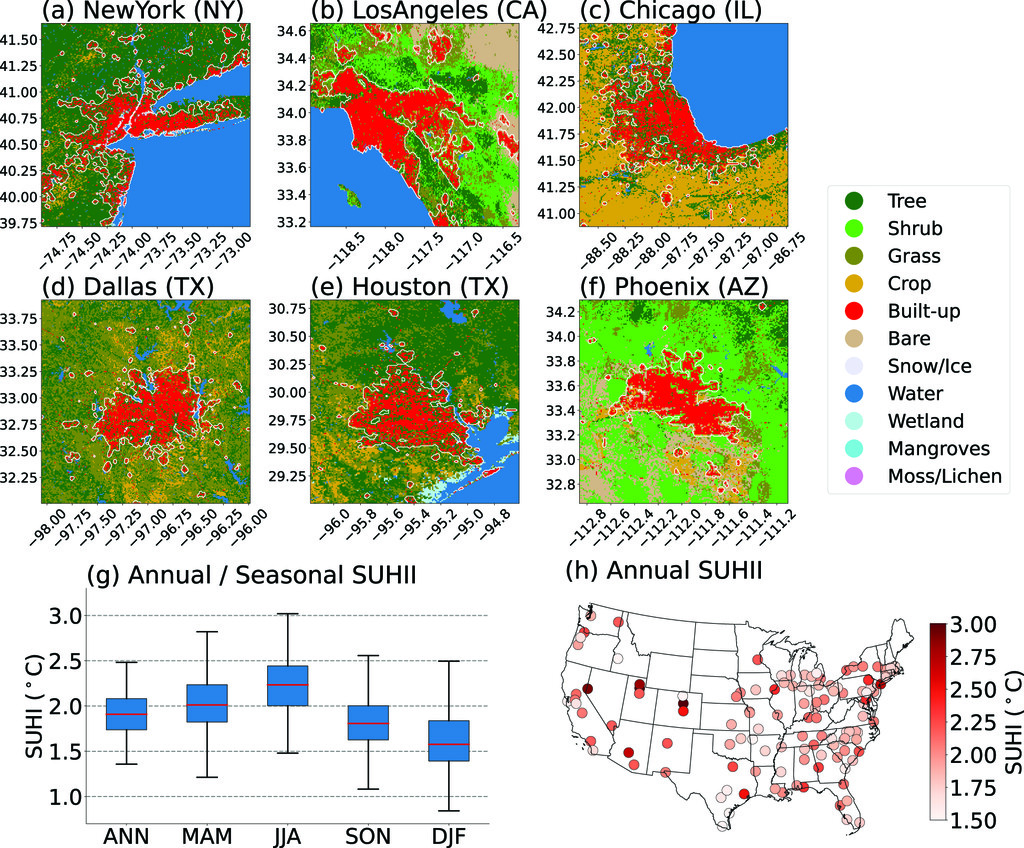
Land cover of the six largest cities analyzed. The white line delineates the boundary between urban and nonurban areas, determined by applying Gaussian smoothing (a-f). Distribution of annual and seasonal surface urban heat island intensity (SUHII) across all 120 cities (g). A spatial depiction of the cities with their corresponding annual mean SUHII values (h).
[Reprinted under a Creative Commons Attribution 4.0 International License ( CC BY 4.0 ) from Lee, J. "Assessment of U.S. Urban Surface Temperature Using GOES-16 and GOES-17 Data: Urban Heat Island and Temperature Inequality." Weather, Climate, and Society 16 (2), 315–29 (2024). DOI: 10.1175/WCAS-D-23-0129.1 .]
The Science
A team of researchers used satellite data to study how temperatures vary in 120 large U.S. cities. By examining how heat changes throughout the day and year, the study found green spaces and reflective surfaces help cool cities and urban areas get hotter as they become more developed. Vulnerable communities often live in these hotter areas, facing more extreme heat. This research provides insight into how city planning can reduce these temperature differences.
Certain areas in cities are much hotter than others, especially where vulnerable communities live. By understanding these temperature patterns, city planners can design better strategies to cool hot spots. This can lead to fairer living conditions and reduce health risks associated with extreme heat.
The study used hourly land surface temperature (LST) data from the Geostationary Operational Environmental Satellite–16 (GOES-16) and GOES-17 to analyze the surface urban heat island intensity (SUHII) in the 120 largest U.S. cities and their surrounding areas. The analysis reveals distinct patterns in seasonal and diurnal SUHII, with the enhanced vegetation index and albedo significantly influencing temperature variations. SUHII’s diurnal cycle shows climate conditions, urban and nonurban land covers, and nighttime human activities affect SUHII peaks differently.
Examining intracity LST dynamics revealed a strong correlation between urban intensity (UI) and LST, indicating LST rises with increasing UI. Vulnerable populations, identified using the social vulnerability index, are often located in high UI regions, resulting in notable LST inequality. These communities face higher LST conditions, potentially leading to greater heat exposure risks. This study highlights the need for city-specific climate change mitigation strategies addressing LST variations and their societal implications, aiming to create cooler and more equitable urban environments.
Principal Investigator
Max Berkelhammer University of Illinois Chicago [email protected]
Program Manager
Sally McFarlane U.S. Department of Energy, Biological and Environmental Research (SC-33) Urban Integrated Field Laboratories [email protected]
This material is based upon work supported by the U.S. Department of Energy, Office of Science, Biological and Environmental Research program’s Urban Integrated Field Laboratories Community Research on Climate and Urban Science research activity under Award Number DE-SC0023226.
Lee, J. "Assessment of U.S. Urban Surface Temperature Using GOES-16 and GOES-17 Data: Urban Heat Island and Temperature Inequality." Weather, Climate, and Society 16 (2), 315–29 (2024). https://doi.org/10.1175/WCAS-D-23-0129.1 .
Impact of urbanization on urban heat island intensity-a case study of Larkana City, Sindh, Pakistan
- Lanjwani, Muhammad Umar
- Lanjwani, Muhammad Farooque
- Hussain, Muhammad
- Sodhar, Khalida
The climate change is one of the important problem of the current situation in the world. The urban heat island intensity is a major problem of increasing the climate condition in the developed and developing countries. In current situation, the growth of population in the Pakistan causes over population in the cities. The population of Larkana is increasing rapidly day by day. The purpose of this research was to investigate the impact of Urbanization on the Climate. In this proposed research study, two types of data were collected (i) satellite data of Thematic Mapper (TM) Landsat 5 which was downloaded from the United States Geological Survey (USGS) of 1990, 2000, and 2010, furthermore satellite data of 2023 downloaded the Landsat 8 from USGS. (ii) Second data used from secondary sources of population census report of Pakistan 1981, 1998, 2017 and 2023 was collected from the Pakistan Statistics Bureau. The land surface temperature was found from satellite data of 1990, 2000, 2010 and 2023. The average temperature in 1990 was 4.25 0 C greater than 2000 in summer season and average temperature of 2010 was 4.73 0 C less than from 2023 in summer season. The average temperature in 1990 was 3.15 0 C less than from 2000 in winter season and in 2022 was 1 0 C higher than 2010 in winter season average temperature. Recently census reported above 579,000 populations lived in the urban city of Larkana. The shape file of the Larkana classification total area showed 41 Square kilometers. The supervised classification showed that settlement increased from 8.16 Square kilometers in 1990 to 23.98 Square kilometers in 2023. The correlation was shown between urban expansion and the growth of population strongly positive to each other. Another finding relationship between urban heat islands with urban expansion that correlation showed a positive relationship between each other.
- Environmental Health
- Why This Matters
Everything you need to know about the urban heat island effect

Photo by Pixabay via Pexels
With 80% of Americans living in urban areas , understanding the heat island effect is crucial when reporting on environmental health as our climate gets hotter .
The heat island effect occurs in urban areas where buildings, roads and other infrastructure absorb and re-emit heat from the sun. City temperatures can be 1–7°F higher than in greener outlying areas ; the effect is especially extreme in heavily urbanized or industrial areas with sparse greenery.
The relentlessness of heat islands from day to night can be dangerous. Even after sunset, the heat can cause dehydration and heat exhaustion. Since man-made structures hold onto heat, especially if they have dark surfaces, nighttime temperatures in cities remain higher by about 2-5°F . These temperature averages can seem relatively small, but there can be enormous differences felt in cities like Las Cruces, NM, where there was a 44.5 °F difference between shaded grass and exposed pavement on a hot day.
The heat experienced in a heat island is often described as atmospheric or surface urban heat islands . The former varies less and describes the warmer air felt in cities compared to its surroundings. The latter changes more significantly when the sun is shining and describes the heat felt from roads, buildings, and non-green surfaces absorbing heat and re-emitting it over time.
Research is limited on chronic exposure to high temperatures and humidity . But, the acute effects of a heat wave exacerbated by the heat island effect can be the difference between life and death. Portland, Oregon, experienced a record high temperature of 116°F during a heat dome in the summer of 2021, a 42°F spike above average. As a result of the event, 69 people died. According to the county’s official report, the heat dome accounted for 61% of all heat-related visits to emergency departments and urgent care centers.
Research has shown that low-income communities of color often experience significantly higher heat conditions than surrounding areas. Low-income communities are also at greater risk of heat-related illness and death , which can be due to inadequate housing conditions without working air conditioning. Also, low-income communities don’t have proper resources to find alternative shelter during heat waves .
In formerly redlined neighborhoods, a discriminatory practice used to deny housing loans to minorities, research has shown that these neighborhoods were 4.6°F warmer than non-redlined areas . Running air conditioning during high temperatures can be a financial burden, and between a quarter and one-third of US households experience some form of energy insecurity .
Here are some health equity-related resources to inform your reporting on urban heat islands:
- Mapping Inequality is an online resource for understanding the history of racial and ethnic discrimination in housing policy.
- The National League of Cities created a tool to mitigate heat wave risk by visualizing tree canopy data and bus stops.
- The EPA created a webpage that helps communities identify and assess heat islands .
Satellite imaging and redline maps
Extreme heat is a silent killer and can be challenging to visualize compared to other disasters like flooding or wildfires. However, satellite imaging of heat maps can help readers visualize and understand heat islands when layered with vegetation and redlined maps .
A city’s redlining history can illuminate health disparities related to extreme heat. Journalists can help readers understand the causes of the heat island effect in redlined areas by covering the inequality of urban tree cover , the percentage of cityscape covered by tree canopy, and how vegetation can be used to reduce heat-related health impacts .
While the urban heat island effect doesn’t contribute significantly to climate change by increasing global temperatures, the phenomenon is affected by it.
The summer of 2023 was 0.41°F warmer than any other summer in NASA records, and NOAA’s latest projections gave 2024 a 61% chance of beating 2023 . The earth is warming quickly, and people in low-income urban neighborhoods are disproportionately affected.
Additional resources
- Dangerous urban heat exposure has tripled since the 1980s, with the poor most at risk — The Conversation.
- As Rising Heat Bakes U.S. Cities, The Poor Often Feel It Most — NPR.
- NOAA is crowdsourcing a national urban heat map — Grist.
- The danger of urban ‘heat islands’ — High Country News.
- The surprisingly simple way cities could save people from extreme heat — Grist.

Recent Posts

Paul Gordon
- climate change
- heat island
- urban heat island
Related Posts
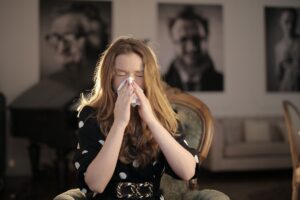
Why climate change matters for seasonal allergies and asthma
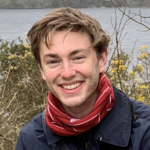
Lyme disease on the rise as climate change expands tick range

Why you should pay attention to climate lawsuits
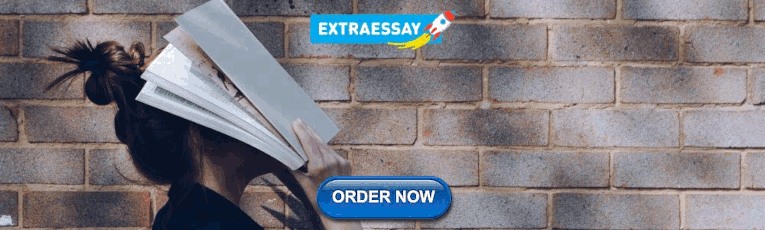
IMAGES
COMMENTS
rural areas. Experts frequently split heat isl ands into two categories [16, 20]: The canopy layer of the urban heat island (CUHI), extends upwards from the ground level to. the ground mean height ...
This paper reports a systematic and overarching review of the literature on urban heat island (UHI) effect. The main objective of this research is to review two broad categories of factors underpinning the UHI intensities: a) spatial factors - the impacts of changes in the spatial aspects of urban environment (e.g. changes in urban form and land cover patterns) on UHI intensity; and b ...
Urban heat stress poses a major risk to public health. Case studies of individual cities suggest that heat exposure, like other environmental stressors, may be unequally distributed across income ...
Abstract. Urban heat island (UHI) effect is a kind of heat accumulation phenomenon within urban area due to urban construction and human. activities. It is recognized as the most evident ...
Abstract. Urban Heat Island (UHI) phenomenon concerns the development of higher ambient temperatures in urban districts compared to the surrounding rural areas. Several studies investigated the ...
Urban heat islands (UHIs) exacerbate the risk of heat-related mortality associated with global climate change. ... the parallel research track of detailed urban energy balance studies 24,41,42 can ...
Abstract. Urban heat island (UHI) effect is a kind of heat accumulation phenomenon within urban area due to urban construction and human activities. It is recognized as the most evident characteristic of urban climate. The increase of land surface temperature caused by UHI effect will definitely influence material flow and energy flow in urban ...
Urban Heat Islands (UHI) consist of the occurrence of higher temperatures in urbanized areas when compared to rural areas. During the warmer seasons, this effect can lead to thermal discomfort, higher energy consumption, and aggravated pollution effects. The application of Remote Sensing (RS) data/techniques using thermal sensors onboard satellites, drones, or aircraft, allow for the ...
Abstract. Urbanization leads to an increase in urban heat islands (UHIs), which is a cause for concern because they endanger the ecological environment and human health. Scenario experiments using the Weather Research and Forecasting Model (WRF) can quantify the independent impact of each urbanization variable on a UHI and predict future urban ...
With rapid urbanization, population growth and anthropogenic activities, an increasing number of major cities across the globe are facing severe urban heat islands (UHI). UHI can cause complex impacts on the urban environment and human health, and it may bring more severe effects under heatwave (HW) conditions. In this paper, a holistic review is conducted to articulate the findings of the ...
A bibliometric analysis based on the Science Citation Index-Expanded (SCI-Expanded) database from the Web of Science was performed to review urban heat island (UHI) research from 1991 to 2015 and statistically assess its developments, trends, and directions. In total, 1822 papers published in 352 journals over the past 25 years were analyzed for scientific output; citations; subject categories ...
Urban heat island mitigation is best achieved through forests and water, and managers of urban areas should avoid developing bare land since they may suffer from degradation. ... The paper discussed the various effects of the LUCC on the Tianjin city's development. The study used the CA model and Geographic Information Systems to analyze the ...
more generation of pow er is n eeded, which results increased. amount of greenhouse gases emission and decline of climate. One of the vital reasons for the formation of UHI is the large. amount of ...
A large number of studies and experiments have shown that urban green space can effectively alleviate the heat island effect. In this paper, we searched the relevant literature through China National Knowledge Infrastructure (CNKI) and WOS Core Collection databases, reviewed the impact of urban green space pattern on urban thermal environment, and summarized the research status of the impact ...
The UHI intensity analysis demonstrates an escalation in surface temperature variation from 15.96% in 2003 to 19.08% in 2023, indicating an intensification of the heat island effect. Spatial analysis reveals that construction sites, transition zones, and urban areas exhibit markedly worse ecological and thermal conditions compared to rural regions.
Abstract. Climate change has intensified urban heat islands (UHIs) in recent decades. While green infrastructure (GI) can mitigate UHIs, access to GI varies due to socioeconomic factors and location, leading to green inequity.
In this paper, a systematic review is conducted on the existing knowledge, collected since 1990, on the link between urban heat island (UHI) and urban pollution island (UPI). Results from 16 countries and 11 Köppen-Geiger climatic zones are perused and compared to delineate methodological and experimental trends, geographical dependencies and ...
This research article is based on 37 papers collected on the UHI studies conducted in different parts of Indian cities. From the papers, it is found that majority of the studies are concentrated towards the capital city. About 30% of the col-lected papers include the heat island formation in Delhi area, and the causes and eAects of it. After
Urbanization has resulted in many critical issues like increase in pollution levels, sudden climatic changes and the rise of temperature in the urban area, that is the formation of Urban Heat Islands (UHI). As the density of population rises, most of the land areas are being converted into cities and cities grows very rapidly. Due to the UHI effect, the cities are becoming hotter day by day ...
The causes of the Urban Heat Island effect [50]. The relation between L* value and reduction of pavement surface temperatures (Black circles indicate high-quality coatings in terms of brightness ...
Urban heat island (UHI) manifests as the temperature rise in built-up urban areas relative to the surrounding rural countryside, largely because of the relatively greater proportion of incident solar energy that is absorbed and stored by man-made materials. The direct impact of UHI can be significant on both daytime and night-time temperatures, and the indirect impacts include increased air ...
This study aims to determine the impact of skyscrapers on the formation of surface urban heat islands (SUHIs). Accordingly, an area within a radius of one kilometer, centered on the skyscraper region in Levent, Istanbul, was selected as the study area. This area was chosen because it shows three different urban textures.
3.1. Consequences of the urban heat island. The UHI effect has significant consequences for the liveability in our cities, and is the source of a significant number of environmental problems in urban areas (Yang et al., 2015).The warming effect of urbanisation has critical impacts on health and wellbeing, as well as human comfort and the local atmosphere (Grimmond, 2007).
A Review on Remote Sensing of Urban Heat and Cool Islands. The variation between land surface temperature (LST) within a city and its surrounding area is a result of variations in surface cover, thermal capacity and three-dimensional geometry. The objective of this research is to review the state... more. Download.
The Science . A team of researchers used satellite data to study how temperatures vary in 120 large U.S. cities. By examining how heat changes throughout the day and year, the study found green spaces and reflective surfaces help cool cities and urban areas get hotter as they become more developed.
Urban Heat Island (UHI) is a phenomenon where higher temperatures are observed in the city centers as compared to its surrounding areas. ... This review paper presents global literature on ...
The climate change is one of the important problem of the current situation in the world. The urban heat island intensity is a major problem of increasing the climate condition in the developed and developing countries. In current situation, the growth of population in the Pakistan causes over population in the cities. The population of Larkana is increasing rapidly day by day. The purpose of ...
Abstract. Motivated by the international tendency to improve the urban microclimate, minimize building energy consumption and improve air quality, this paper carries out a literature review of the ...
The urban heat island (UHI) effect in arid cities can be small or even negative, the latter known as the urban cool island (UCI) effect. ... competing financial interests or personal relationships that could have appeared to influence the work reported in this paper. Acknowledgments. This research was supported by the Postdoctoral Science ...
With 80% of Americans living in urban areas, understanding the heat island effect is crucial when reporting on environmental health as our climate gets hotter.. The heat island effect occurs in urban areas where buildings, roads and other infrastructure absorb and re-emit heat from the sun. City temperatures can be 1-7°F higher than in greener outlying areas; the effect is especially ...