- Practice →
- Projects →
- Rail and Mass Transit
- Bridges and Infrastructure
- Culture and Exhibition
- Education and Science
- Residential
- Industry and Energy
- Master Planning
- Industrial Design
- News →
- Press Releases
- Careers →
- Journal →
- Building the Next Generation of Engineers & Architects
- Unlocking the Passenger Experience through Integrated Airport Design
- Learning Lessons for and from a World-Leading Transport Network
- Sustainable Skies
- Contact →
© 2024 GRIMSHAW
Project Gallery →
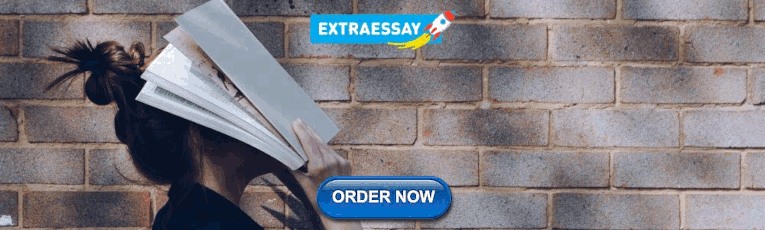
Civil Engineering Building: Case Study Cambridge, United Kingdom
The new civil engineering building is a world-class research space, and home to the Engineering Department's civil engineering division and the newly established National Research Facility for Infrastructure. Here researchers are exploring sustainable infrastructure and cities in collaboration with engineers from across the Department of Engineering. The building marks the completion of the first phase of the Department of Engineering's new campus at West Cambridge, also designed by Grimshaw. Due for completion in 2026, the new campus will reintegrate the entire Department of Engineering into a single site, providing 100,000 sq m of research, teaching and collaboration space.
Download PDF
Sustainability was a core driver, with the Department of Engineering setting ambitious targets for the design and construction of all building's at their new campus. Our brief required the design to minimise whole-life energy/carbon, while simultaneously providing a pleasant environment for occupants — promoting natural ventilation where possible, avoiding 'green bling', ensuring future flexibility and making sure the building's performance met predicted design performance targets.
The building's form, massing and location were informed by the local planning requirements, the wider engineering campus masterplan and the technical requirements of the end users.
The building's location was chosen to enhance the existing urban realm, achieved through alignment with the existing buildings and lawn to the west, creating a new courtyard at the approach to the building main entrance. The building's massing and height provide continuity with the existing building to its west, whilst minimise the visual impact to its residential neighbours to the east. Its form and internal layout were driven by the needs of the research to be undertaken in the laboratories, with heavy labs located on the ground floor, lighter labs located on the middle floor and offices arranged around a central courtyard on the upper floor to promote cross ventilation to the open plan offices and provide daylight to the labs below. The building also provides visual connectivity between the labs and public realm to give campus visitors an insight into the research being undertaken.
A modular approach to the building's planning grid and services distribution maximises the potential for future flexibility and adaptation, ensuring a long service life.

Service cores are located to the perimeter to minimise disruption during future phases and clad with demountable cassette panels that can be reused as the building grows.

The Grimshaw led design team were privileged to collaborate the late Sir David MacKay, a world-leading authority in sustainable energy at the University. MacKay wanted to address the proliferation of 'green bling' in architecture and the emerging gap between predicted and actual performance of modern buildings. Using the building as a test-bed, Grimshaw's team worked with MacKay, and other leading academics at the University, to develop the Energy Cost Metric (ECM). By combining whole-life energy and costing assessments into a single analytical tool, the ECM provided a new way to objectively evaluate and whole-life energy savings and associated cost for different design strategies in a single equation to establish which provided the best value to the University. Its use during the design phase directly influenced the decision to specify a ground source heat pump with heat recovery over gas boilers: The University's Estates Department has subsequently incorporated the ECM into their guidance for new buildings. The Department of Engineering has recently published a paper, outlining the ECM's development and application during the projects design phase, to support the construction industry's efforts to minimise whole-life energy and carbon within a cost-conscious framework.
The masterplan for the new engineering campus is conceived as 'loose-fit' framework to maximise the possible growth scenarios available. This approach was essential to accommodate the intermittent nature of funding streams available to finance the Department's move to West Cambridge and to maximise the potential to re-use as much of the existing building stock on site as possible. The building itself adopted a flexible spatial framework employing a regular planning module, zoning of heavy and light engineering activities to avoid operational disruption. The building adopted the principles of circularity by ensuring as many of the primary structural and facade components were designed for quality and longevity and with deconstruction and re-use in mind. An estimated 80% of the structural steel and façade components are recoverable. As a consequence of this approach the buildings whole-life embodied carbon profile was further reduced.
More numbers, less rhetoric
The project follows the UK Governments soft-landing’s framework to ensure commissioning and handover goes smoothly, with post-occupancy analysis undertaken over an extended two-year period. This will enable the project team to establish if the predicted performance targets are being met. This approach, combined with data captured from integrated environmental sensors, will enable the design team to draw lessons that can be applied to future phases of the engineering campus.

New Engineering Campus at West Cambridge and building site overview

This goal was central to the University’s brief with occupant comfort, access to daylight, natural ventilation, external spaces and dedicated cycling facilities (inc. showers) prioritised in the design.
The brief sought to minimise operational energy demands, maximise on-site renewables and banned the combustion of fossil fuels from the project.
The research undertaken in the building is part-funded by the UK Collaboratorium for Research on Infrastructure and Cities (UKCRIC) with a mission to underpin the renewal, sustainment and improvement of infrastructure and cities in the UK and elsewhere.
The design team’s focus on material and carbon reduction, from lightweight structures to the innovative ECM assessment tool, supports responsible consumption and production.
Minimising the building's whole-life energy, and carbon, was a central tenet of the Department of Engineering efforts to address climate change.
The project sort to create a net-positive contribution to biodiversity with the incorporation of a green roof and the restoration of the adjacent woodland belt to the buildings eastern perimeter.

1. Thermal mass – Exposed concrete floor slabs reduce internal annual temperature swings by storing heat in the summer months, preventing overheating, and releasing heat during the winter months to provide passive heating.
2. Natural ventilation & cooling – High level actuated louvres provide background ventilation year-round. In the summer they are supplemented by manual windows to maximise air flow and provide secure night-time cooling/purge ventilation.
3. Active ventilation & cooling – All laboratories are temperature controlled with fan coil units [FCU] with fresh air supplied and exhausted locally from the external façade with heat recovery.
4. Zoned underfloor heating – Office and light laboratory accommodation is heated via a low temperature underfloor heating system which can be zoned to maximise flexibility to subdivide and combine rooms at a later date.
5. Ground source heat pump [GSHP] – Fan coil units and underfloor heating systems are connected to a GSHP which stores excess heat from the laboratories to provide cooling and heating to the building throughout the year. Combined with the on-site PV array, the system provides an all electric, low energy/carbon solution.
6. Daylighting – A central courtyard, 40:60 window to wall ratio, external solar shading and glazing with a high visible light transmission above 55%, all help to maximise daylight within the buildings, while keeping excess glare and solar heat gains to a minimum.
7. Well-measured – Sensors integrated throughout the building measure environmental performance, including energy consumption.
8. Green roof – The entire roof is covered with sedum to provide amenity for wildlife. This provides a net gain in soft planting on site.
9. Woodland belt – The existing woodland belt to the east of the site has been cut back and replanted. These interventions, along with a commissioning of a detailed woodland management plan, will promote a net increase in quality vegetation, which over time should lead to a net gain in biodiversity.
10. Self-healing concrete – In collaboration with the Department of Engineering, self-healing concrete was installed in a section of the ground floor slab. This technology uses a graphene additive that acts as a binder when cracks form, to maintain the concrete's integrity.
11. Dynamic solar shading – A thermochromic film, incorporated into glass shading fins on the western facade, change opacity in response to increases in ambient temperature and direct solar heat gain. On hot sunny days the film becomes opaque helping to omit excess heat gains from the building, while maintaining unobstructed views out. On colder days the fins become fully transparent, maximising access to daylight and passive solar heat gains.
Key Sustainability Facts
Existing Site Expansion
Sub-Urban / Temperate Zone
EUI: 105 to 148 kWh/m2/yr [Predicted mid to high range] EUI: 85 kWh/m2/yr [In-use] * Energy/Fuel type: 100% electric building On-site renewables: 10.8kW photovoltaic [PV] array with a potential to generate 5,242kWh/yr
* Annual consumption reported for 2021 - Building occupied from Oct 2019
While the embodied energy for key building elements were measured as part of the ECM analysis, the building's total embodied carbon has not been measured during design or construction phases. As part of their campus wide carbon off-setting strategy, the University's Estates Department plans to measure the building's upfront embodied carbon from as-built drawings information and supply-chain documentation.
The design employs low-flow appliances to minimise on-site water use and incorporates a sustainable drainage strategy with on-site swales and a blue/green roof to attenuate water run-off from the site.
BREEAM Excellent [Design + Construction]
Location Cambridge, United Kingdom
Project Partner/Lead Neven Sidor, Partner Peter Swallow, Associate
Project Team Grimshaw, Max Fordham, Smith & Wallwork, Turkington Martin, Montressor [LLP]
Client University of Cambridge
Area 4380 sq m
Status Complete
© 2024 GRIMSHAW · Legal + Disclaimer · Subscribe

Whitepapers and Case Studies
Whitepapers and case studies for the civil and structural engineering industry.
The New Economy Versus the Old Economy: Winners and Losers in Tomorrow’s Built Environment
Which areas will fare better through a recession and which will contract? Download the paper to gain insights into areas of the new economy that will likely experience growth, such… Continuing Education Whitepapers and Case Studies Sep 15, 2022
Truck Scale Buying Guide
The purpose of this guide is to provide educational information to both new and sea-soned truck scale buyers. It is intended to provide more and different information than you find… Continuing Education Infrastructure Whitepapers and Case Studies Jun 28, 2022
New Vehicle Weighing Laws
The NIST Handbook 44 standard defines the specifications and tolerances for weighing and measuring devices used in the United States, including vehicle scales. In 2022, single draft weigh-in-motion (SD-WIM) vehicle… Continuing Education Infrastructure Whitepapers and Case Studies Jun 20, 2022
Chronic water waste results in alarming increase in carbon emissions, research shows
Water Whitepapers and Case Studies Mar 24, 2022
A Roadmap for Soil Organic Carbon Measurement and Verification
Continuing Education Whitepapers and Case Studies Dec 16, 2021
Salesforce Tower Chicago
Structures Whitepapers and Case Studies Nov 1, 2021
Infrastructure Digital Twin Maturity: A Model for Measuring Progress
Whitepapers and Case Studies Aug 3, 2021
Engineer Optimizes 25.5-Foot-Tall Gravity Retaining Wall
When excavation for a retaining wall at John C. Tune Airport in Nashville, Tennessee, opened up a geotechnical can of worms, engineer Clint Hines, P.E. found a way to keep… Geotechnical Whitepapers and Case Studies Sep 24, 2019
Plastic Pipes Inspection Methods Report Now Available
The Plastics Pipe Institute, Inc. (PPI) has published a new document that provides an overview of non-destructive testing and evaluation techniques that have been introduced into the plastic pipe industry. … Data + Publications Whitepapers and Case Studies Sep 23, 2019
BRAYN Tax Consulting
BRAYN Consulting is a niche consulting firm that guides businesses to greater value through tax credits and incentives, such as R&D Tax Credits, Cost Segregation, 179D, 45L, and Fuel Tax… Whitepapers and Case Studies Jun 18, 2019
A new paper reveals the hidden cost and impact of water waste in facilities NEW YORK — The water systems within the world’s building and facilities are a major source of… Water Whitepapers and Case Studies Mar 24, 2022
To Escape Paper, FHU Heads to the Cloud
This case study examines how an engineering consultant uses a cloud-based construction administration platform to automate manual tasks and cut down on wasted labor hours found in the daily reporting… Whitepapers and Case Studies Mar 17, 2020
NEW HDPE CONDUIT OVALITY TECHNICAL DOCUMENT AVAILABLE
Details Measures to Resolve Ovality During Power and Communications System Installation The Plastics Pipe Institute, Inc. (PPI), has published a new document about ovality in high-density polyethylene (HDPE) conduit, including… Whitepapers and Case Studies Mar 3, 2020
One of the world’s most effective climate solutions lays dormant under our feet. Regenerative agriculture restores the health of soils, improves ecosystem services, offers additional revenue for farmers, and provides… Continuing Education Whitepapers and Case Studies Dec 16, 2021
What is Ultrasound?
Sound waves, which are all around us, are simply organized mechanical vibrations traveling through a medium, which may be a solid, a liquid, or a gas. This applies to both… Whitepapers and Case Studies Dec 8, 2020
From Pitfall to Profit: The Hidden Potential of Business Licensing
Harbor Compliance has published a white paper that shows businesses how to turn a common source of frustration and expense into an asset that yields returns on investment (ROI) of 30X and more.… Whitepapers and Case Studies Dec 1, 2017
Photo: Nick Ulivieri Photography The Final Riverfront Development Site Salesforce Tower Chicago, a 58-story tower named after its primary tenant, provides 1.2 million square feet of office space and completes… Structures Whitepapers and Case Studies Nov 1, 2021
Structural and Civil Engineering Professors get Free Engineering Software for their Students
When engineering firms hire newly-graduated engineering students as EITs, their value to the firm and their early career progress is accelerated when they have experience with the professional engineering software… Whitepapers and Case Studies Nov 25, 2019
Cross-Laminated Timber – What is it and When Does it Make Sense?
As with many systems, the combination of parts is more valuable than the parts individually. If concrete and steel’s preeminence led to such astounding progress in the last two centuries,… Whitepapers and Case Studies Nov 21, 2019
If you are leading an infrastructure project you, are probably interested in investigating and applying digital twins. You may be asked two key questions: What is a digital twin and… Whitepapers and Case Studies Aug 3, 2021
Resolving Contractor Disputes in Minutes with Project Data Tracking Tools
RS&H Dissolves Disputes with Appia®’s Audit Trail CHALLENGE As the Construction Services Discipline Leader for RS&H, David Elliott and his team travel to job sites around the country to “kick… Latest Whitepapers and Case Studies Aug 28, 2020
Sustainability of Concrete Structures with Crystalline Technology
Engineers, architects, and contractors understand that in discussing sustainability in construction we are talking about employing an array of “best practices” in design, material selection, and processes to reduce the… Industry News Structures Whitepapers and Case Studies Aug 23, 2019
Assessment of Energy System Reliability Failures During the Extreme Cold Weather Event in the ERCOT Region
By John Dulude, PE, MBA & Paul Banks, PG Introduction According to Accuweather, the damage costs from the winter storm in mid-February could be as high as $130 billion in… Whitepapers and Case Studies Apr 14, 2021
Project Certification and Closeout in a Virtual COVID World and After
As we experience and emerge from “the great shutdown” of 2020, things have and will change in all aspects of life – including the design and construction process. Nowhere in… Continuing Education Whitepapers and Case Studies Apr 29, 2020
Dealing with “Time is of the Essence”
In this (or any other) time of social and/or business disruption, delays, or shutdowns, focus frequently goes to the scheduling clauses contained in design and construction professional service agreements. One… Continuing Education Whitepapers and Case Studies Apr 29, 2020
TowerPinkster Upgrades Spec Writing Solution Across Firm of Diverse Users – Case Study
Portland, ME – Chalkline, Inc. has published a Case Study on TowerPinkster’s evaluation, selection, and success of deploying and using VisiSpecs. TowerPinkster wanted to better utilize their BIM models to create and… Industry News Whitepapers and Case Studies Jan 18, 2021
Cross-Laminated Timber
What is it and When Does it Make Sense? By Augustus “Gus” Raymond Entering the 19th century, the 482’ Strasbourg Cathedral was the tallest structure in the world, horses, and… Building Materials Whitepapers and Case Studies Jan 1, 2020
Using Aerial Mapping to Grow Your Business
Business owners need to utilize technology effectively in order to compete. Whether you want a steady flow of work or to grow your business, adopting technology is the key. Download… Whitepapers and Case Studies Jan 28, 2019
Expense-2-Project’s File-Level Association
Cetrus’ Plug-in, Expense-2-Project (E2P), can uniquely identify application use, by a specific user for a specific project, down to the file level. This exact, file-level association allows companies to turn… Technology + Innovation Whitepapers and Case Studies Jul 26, 2019
Big-Box Retail Center Retention Pond Restoration
Swarms of mosquitoes near a retention pond adjacent to a big-box retail center was the first indication that the area was suffering from significant environmental damage. What started out as… Environmental Water Whitepapers and Case Studies Jul 25, 2019
Impervious Gravel vs. Porous Aggregate Paving Systems
The common belief shared by many stormwater engineers and local and state agencies is that gravel parking lots, roads, and driveways are not porous—and for purposes of stormwater runoff, gravel… Continuing Education Infrastructure Whitepapers and Case Studies May 31, 2019
[Ebook] Own Your Data: What Data Portability Means for the Construction Industry
Read our ebook to learn about the benefits of implementing productivity tools to eliminate information gaps that occur as data is generated over the course of a construction project. This ebook… Continuing Education Data + Publications Whitepapers and Case Studies May 24, 2019
3 Secrets to Delivering Successful Design Projects (Without Stress)
Your end goals are the same as they have been – achieving them has just been made simpler. In our e-book, we highlight how 3 organizations leveraged new solutions to: Better… Whitepapers and Case Studies May 2, 2019
Why Geocells Outperform Geogrids for Road Construction
Geocells (cellular confinement) offer a more effective and practical 3D design solution to load support challenges than multilayered 2D geogrid efforts. Geocells transfer applied loads instantaneously, delivering practical soil stabilization… Whitepapers and Case Studies Feb 1, 2019
A Ductile Iron Pile Deep Foundation Solution Provides More Than 2 Full Weeks of Schedule Savings
Helical Drilling installs Ductile Iron Piles, a new low-vibration deep foundation system, within an existing high-bay commercial building to support the new concrete structural slab. CLICK HERE TO VIEW THE… Whitepapers and Case Studies Oct 27, 2017
ORIGINAL RESEARCH article
A case study on design and optimization of adaptive civil structures.
- Institute for Structural Mechanics, University of Stuttgart, Stuttgart, Germany
Taking advantage of adaptivity in the field of civil engineering is a subject of ongoing research. Integration of adaptive elements in load-bearing structures is already well-established in many other engineering fields, albeit mostly for different purposes than withstanding predominantly static loads. Initial investigations have demonstrated potential for substantial material and energy savings also in the field of civil engineering, especially for high-rise buildings and wide-span structures, such as roofs or bridges. Adaptive civil structures show promise in tackling current challenges arising from emissions and shortages of materials. In this study, we compare the possible minimum-weight designs for different actuator placement approaches and for different structural topologies that satisfy various constraints for high-rise buildings. We use case studies as illustrative examples to show which advantages and disadvantages can be expected from a specific design. The overarching aim is to learn how truss and beam structures should be designed to perform well as adaptive structures.
1. Introduction
To tackle today's challenges arising from extensive material consumption, waste production, and emissions, innovative solutions are needed from the building industry. Nowadays, buildings are designed to withstand occurring loads and simultaneously satisfy defined conditions by using a large amount of material, leading to a large amount of emissions and waste. For most of a building's life span, some of this material is not needed, because extreme load conditions are not permanent. The incorporation of active elements in passive structures to make the structures adaptive offers a promising way of using material more efficiently. The resulting adaptive buildings therefore consist of passive elements, controllers, sensors, and actuators, which can influence the load-bearing and deformation behavior of the whole structure. The underlying idea is to significantly reduce embodied energy in built structures by avoiding the use of material that is necessary only in a small part of the building's life span to maintain, e.g., serviceability limits. The missing structural part is compensated for by using actuation energy, which affects the critical states that are assumed to overshoot defined limits. Preliminary investigations have demonstrated potential for significant material savings in the primary structure. In times of increasing demand for sand and other scarce raw materials, adaptive structures offer a very promising approach to more sustainable buildings.
However, compared to the design of passive structures, new challenges arise in the design of adaptive structures. Influences from architecture, structural engineering, mechanical engineering, and control engineering have to be integrated in order to design an optimal adaptive structure. To bring together specialists in all these different areas, the Collaborative Research Center SFB 1244, “Adaptive Skins and Structures for the Built Environment of Tomorrow,” was initiated in 2017 at the University of Stuttgart. Application of the design concepts in realistic scenarios and buildings is being researched and tested at the interdisciplinary Collaborative Research Center. A demonstrator high-rise will also be erected on the campus of the University of Stuttgart. Details are presented in Weidner et al. (2018) . Apart from the aforementioned challenges in the design of the load-bearing structure, the research center conducts work on adaptive facades and adaptivity in the field of building physics.
The first designs to include active elements in civil structures were developed in the 1960s. Zuk and Clark (1970) presented the idea of an active tendon system for controlling static deformations and manipulating internal forces, which was the origin of much work on this topic. The early approaches focused on the control of vibrations in order to maintain defined limits of serviceability and safety (e.g., Soong and Manolis, 1987 ). Another research focus was deployable large-span space structures (e.g., Kwan and Pellegrino, 1993 ). Pertinent overviews can be found in Soong and Spencer (1992) , Soong (1988) , Utku (1998) , and Korkmaz (2011) .
In the present work, design and optimization of adaptive structures are addressed. Initial approaches to optimized adaptive structures were due to Kirsch and Moses (1977) , who carried out an optimization for a given beam structure with fixed actuator position to determine the minimum cross-sectional dimensions. Optimization procedures involving simultaneous optimization of the controller and the structure were introduced by Hale et al. (1985) . An overview of further optimization approaches can be found in Frecker (2003) . Inspired by a separation of equilibrium and compatibility equations, introduced by Kirsch and Moses (1977) , Teuffel (2004) proposed a workflow for designing ultra-light adaptive structures, additionally incorporating the problem of actuator placement in adaptive truss structures. This approach was adopted by Senatore et al. (2019) and extended to an “all in one” formulation of the design of adaptive structures which included whole-life energy assessment. Additional computational improvements were also obtained, and the results have been experimentally validated by Senatore et al. (2017) . In contrast to the work of Senatore et al. (2019) , the present article proposes not a workflow for designing minimum-energy adaptive structures, but rather a method for learning about fundamental properties of adaptive structures. The main goal of the method is to learn which types of structures are suitable, and especially, why. Therefore, some simplifications are needed (e.g., neglecting additional masses of actuators), which are stated in our assumptions and models, to be introduced in the appropriate sections. The problem of suitable actuator placement to achieve certain goals was addressed by Kawaguchi et al. (1996) and, from a system dynamics point of view recently, by Wagner et al. (2018) , among other authors. The influence of the design process of adaptive structures on adaptability, performance, and actuation energy demand was investigated by Geiger et al. (2020b) in a simple case study. Fröhlich et al. (2019) proposed a method for optimizing structures toward their efficiency, using a measure of the total energy demand as the objective function.
This paper's focus is on incorporating adaptivity into the load-bearing structure, in order to manipulate structural behavior under different loading scenarios. Given a design task for a building, including, e.g., predefined overall dimensions and other structural constraints, finding the basic concept of the structure in terms of the topological layout of the structural members and the design of the load-bearing behavior is one of the most important tasks for the structural engineer. For conventional passive structures, layout and design strategies to produce structures that are efficient, reliable, redundant, cheap, etc., are well-known. With adaptive structures, however, building upon experience gained in the design of passive structures may lead to suboptimal results. Therefore, the aim of this research is to find design criteria and guidelines to determine whether the geometry and the topological layout of an optimal passive structure will lead to an optimal adaptive structure, and to identify how efficient adaptive structures can be characterized and designed. Preliminary studies can be found in Geiger et al. (2020a) , which describes an approach to actuator placement using the redundancy matrix in a forward calculation without employing an optimization algorithm. Additionally, a proof-of-concept for the idea of transforming stiffness-governed structures into strength-governed structures is presented. The term stiffness-governed means that the stiffness of the structure against displacements is design decisive for the cross-section of structural members. It is shown that when adaptivity comes into play, this is not the case. This leads to the conclusion that the normal force and hence the maximum allowable stress of the used material is decisive for the cross-section of a bar, which is a characteristic of strength-governed problems. This transformation from stiffness-governed to strength-governed makes possible a more efficient utilization of the material and thus significant savings of material. The present work extends these basic studies, first to a systematic comparison of different assemblies in terms of topology for truss structures and then to an investigation of beam structures, in order to deduce new design guidelines.
The paper is structured as follows: In section 2 the methodology for the case study, basic assumptions, and details of the modeling are presented. Section 3 presents two different adaptive structures in various configurations. The overall mass-saving potentials are computed for the different layouts, and the design ideas deduced from them are discussed.
2. Methodology
In this section, the methodology of the case study is motivated, and the necessary actuator placement, structural and actuation models, and efficient solution process are presented.
2.1. Case Study
The aim of this research is to learn how to design adaptive structures. To derive design guidelines, a case study on different adaptive structures is conducted. The results are compared and the observations discussed with regard to insights gained into the characteristics of adaptive structures. This process requires objective measures that can be used to compare different adaptive structures and to determine which design is preferable. Comparison of adaptive structures gives rise to a new class of problems in the field of structural design, and new measures have to be defined. In the present approach, different variants of adaptive structures are compared using two values, which have to be calculated for all variants. To obtain comparable results, the outer dimensions of the variants are kept constant, and identical loading scenarios and structural constraints are used. The first measure is the mass-saving potential of the adaptive structure relative to the passive structure with the same topology but without actuation; each active element is treated as the corresponding passive element. The second measure is the total mass of the adaptive structure. We do not compare the whole-life energy demand of the structures, because this would require an additional quantification of the material saving vs. the energy saving, which would entail numerous additional assumptions, such as assumptions concerning the energy mix in the following years for different countries, and is thus beyond the scope of this study, which is dedicated to structural behavior and the corresponding potential of adaptation.
Firstly, for a given topology, the minimum-weight design of the structure without actuation is compared to the minimum-weight design of the same structure using actuators. These designs for the passive and the active structures are the solutions of two non-linear optimization problems, the formulation of which may be found in section 2.4.
Secondly, for given outer dimensions (e.g., height and width) and a given application of the adaptive structure (e.g., high-rise building), different topologies are compared to generate guidelines. The comparison makes use of insights from a structural mechanics point of view in conjunction with observations, comparing the computed results. In this process, the influence of known structural properties of the investigated topologies is examined. Among other factors, the degree of static indeterminacy is investigated. Therefore, starting from a basic configuration of an exemplary structure, different variants are generated by inserting or removing elements and/or supports. This generation process is motivated by the aim to verify or falsify hypotheses, which are presented and discussed.
2.2. Structural Model
The basic assumptions and modeling aspects are presented in this subsection. In the present investigation only structures consisting of truss and beam elements are considered, and only centric linear actuators are used, e.g., hydraulic cylinders in the center of a truss or beam element. To keep the computations as simple and fast as possible, small deformations and linear elastic isotropic material behavior are assumed. For a single element e , a linear elastic material model is chosen with Young's modulus E e , tensile strength f y, e , mass density ρ e , and Poisson's ratio ν e . Dynamic effects are neglected in this case study. Under these assumptions, the discrete linear time-invariant equation of motion for the problem is given by
The system stiffness matrix K ∈ ℝ n × n describes the correlation of load vectors gathered column-wise in a matrix F ∈ ℝ n × l and the solution vector gathered column-wise in a matrix D ∈ ℝ n × l . The number of degrees of freedom in the model is represented by the variable n , and the number of load cases is represented by l . The load vector F is the sum of the vector of external forces F ext , which collects the external forces for each degree of freedom, and the vector F act = Bu , which contains the input matrix B ∈ ℝ n × m and the actuation input u ∈ ℝ m × l . The number of actuators is represented by m . The load vector can therefore be expressed as a function of the actuation input, F = F ( u ). The system stiffness matrix K is assembled from all the element stiffness matrices, which depend on the cross-sections of the elements. Therefore, the system stiffness matrix is a function of the vector a ∈ ℝ n ele , which collects the cross-sectional areas of all elements, and of the vector i , which collects the moments of inertia of all beam elements: K = K ( a, i ) = K ( s ). The vector of design variables relating to the cross-sections is defined as s : = [ a, i ] T . The solution D of Equation (1) gives the structural responses for all investigated load cases, which are used for further processing.
2.3. Actuator Model
For a proper and efficient simulation of the actuation, an active beam finite element is introduced, extending the active truss finite element presented in Geiger et al. (2020b) . The aim is to apply a prescribed stroke u of an actuator directly, without further pre- and post-processing steps, for beam finite elements as well. Figure 1 shows the element used. The derivation and particular modifications compared to the active truss are briefly discussed in the following. The starting point is the total potential energy functional Π tot of a plane Bernoulli beam element, given by
The total potential energy consists of both internal and external potential energy and depends on the displacement fields in the axial direction d ( x ) and the transverse direction w ( x ). The element is in equilibrium if and only if the first variation of the total potential energy, δΠ tot , vanishes. The total potential energy functional has a minimum for these particular displacement fields. For the derivation we assume geometrically and materially linear behavior and that the displacement fields in the axial and transverse directions are decoupled. Therefore, the effects can be separated in the total potential energy functional:
Quantities related to energy from axial forces in the beam, including all contributions from the actuation, are labeled with superscript N, and quantities related to bending energy are labeled with superscript M. Using this functional, the derivation simplifies to the separate derivation of the active truss element as shown in Geiger et al. (2020b) using the axial force part of the internal potential energy Π int,N [ d ( x )]. The actuation of the element is modeled as a discontinuity in the magnitude of the applied stroke u in the axial displacement field d ( x ). In order to describe this jump, the element is cut into two parts, each part is modeled separately, and the connection is introduced by the additional coupling equation for displacements in the axial direction between points a and b ,
as shown in Figure 1 . Including the constraint equation by means of the Lagrangian multiplier λ yields the enhanced internal energy from axial forces:
The Lagrangian functional yields
After applying the same procedure as in Geiger et al. (2020b) , consisting of variation and discretization using linear ansatz functions, to the axial force part, the bending part Π int,M [ w ( x )] can be treated separately, leading to the derivation of a passive beam element. No effects from the centric linear actuation have to be considered in this part. For further details on the derivation of the bending stiffness matrix, see e.g., Melosh (1963) . For a single plane and a straight active beam element in horizontal orientation as shown in Figure 1 , the derivation yields a linear system of equations at the element level:
Quantities for part 1 are labeled with subscript 1 and quantities for part 2 with subscript 2. The matrices k 1,N and k 2,N describe the particular elastic axial stiffness matrices, and the vectors g 1,N and g 2,N are the corresponding coupling vectors. The matrices k 1,M and k 2,M describe the elastic bending stiffness matrices for the bending action and k 12,M the corresponding coupling matrix. Load and displacement vectors are separated into displacements of the two parts and the additional variables f A and u . These parameters describe the discretized Lagrangian multiplier representing the actuator force f A , which is therefore directly computed when solving the linear system of equations, and the applied stroke in the actuator u , respectively. It can be seen that there is a coupling of the bending part between the two separated element parts 1 and 2 introduced by the matrix k 12,M . At the same position in the stiffness matrix for the axial part, there is no direct coupling. The coupling of the axial part is introduced by the additional condition and therefore by the vectors g 1,N and g 2,N . The decoupling of axial force and bending within one element may not be seen in this representation. The element stiffness matrix k ~ and the element load vector are used to assemble the global stiffness matrix K and the global load vector F . After assembly, the linear system of equations (1) can be solved for the global solution vector D .

Figure 1 . Active beam element (left) and degrees of freedom to connect the element to the rest of the structure (right) .
2.4. Structural Optimization
The minimum possible mass of the structure is computed by an optimization procedure using the total mass as the objective function and several non-linear constraints for displacements and stresses. Since we only look at plane examples, the feasibility of the stresses is evaluated at four particular positions of each element: at either end of the element at the upper and lower edges of the actual cross-section. To keep it simple, only nodal loads and no distributed loads are permitted, so that it is not necessary to check the stresses along the beam span. Additionally, each element in compression is checked for buckling. Therefore, Euler's critical buckling force N b, e is computed for the element's actual cross-section, and the absolute value of its normal force N e may not exceed this value. Assumptions on the cross-sections are necessary to keep calculations simple and the number of design variables as small as possible. In the present paper, a square hollow section (SHS) is chosen, which can be described by only two independent variables, the cross-sectional area A and the moment of inertia I . The feasibility with maximum allowable displacements is checked at predefined degrees of freedom. The maximum displacement at those chosen degrees of freedom D c must not exceed the predefined limit.
2.4.1. Formulation of the Optimization Problem for the Passive Structure
The formulation of the resulting mass minimization problem for the passive structure reads
2.4.2. Formulation of the Optimization Problem for the Active Structure
For the active structure, additionally the input strokes for the actuators is a design variable and part of the optimization. Therefore, the optimization problem reads
2.4.3. Solution Method for the Optimization Problems
To facilitate the simulation of several load cases in an efficient way, the solution procedure was implemented in MATLAB using vectorized solutions and post-processing. An SQP implementation available in MATLAB was chosen as the optimization algorithm, which requires the gradient and the Hessian of the objective function and of the constraint functions. The gradient of the objective function is calculated analytically and passed to the optimizer, and the gradient of the constraint functions is computed using a complex-step derivative approximation as in Squire and Trapp (1998) , also vectorized for all design variables.
The attractive features of this type of numerical differentiation are briefly outlined in the following. The classical forward-difference formula for computing the first derivative of a function f ( x ) reads
Two errors occur in the approximation of the first derivative, namely the subtraction cancellation error, from taking the difference of two similar-valued numbers f ( x + h ) and f ( x ), and the truncation error, from neglecting the O ( h ) part in the computation of the approximation. The step size has to be small enough to limit the truncation error but large enough to limit the error from subtraction cancellation, and it is not trivial to estimate the optimal compromise a priori. By using the complex-step derivative, the formula changes to
This method does not suffer from the subtraction cancellation error, because no subtraction is needed. The truncation error is reduced significantly, because the truncated parts are of order O ( h 2 ) . In the following optimizations a step size of h = 10 −10 is used, so the truncation error can be neglected. For further reading, a derivation of the formula, and numerical examples, see Martins et al. (2003) . The implementation in the MATLAB environment is not subject to major changes. Only computations of absolute values or transpositions that are suitable for complex-valued scalars, vectors, and matrices have to be implemented. The approximation of the Hessian is achieved by a Broyden-Fletcher-Goldfarb-Shanno (BFGS) algorithm; see Fletcher (2013) . For future work, the Hessian can also be computed using complex numbers to achieve higher accuracy than the approximation and hence faster convergence of the optimization.
3. Case Study and Discussion
The preliminary work of Geiger et al. (2020a) is used as a starting point for the formulation of hypotheses, which will be verified or falsified in two examples. Through this structured investigation, design guidelines are deduced. The preliminary work is extended to the comparison of different layouts for a truss structure in the first example and to the investigation of frame structures in the second example. In both examples, the mass savings achieved by adaptation and the minimized total masses are compared for several topological modifications of a basic structure exposed to the same load cases. Both constraints on maximum allowable stresses and constraints on maximum allowable displacements are met through active control. Failure of the actuators or of the control system is not considered in this case study.
The following assumptions hold for both examples. In all cases structural steel S235 with the following properties is chosen:
Square hollow sections with maximum outer dimensions of 0.50 × 0.50 m are used. The thickness of the walls is not limited until the box is fully filled with material, so the wall thickness is less than 0.25 m. The minimum outer edge length is fixed at 0.01 m, and the minimum wall thickness is defined as 1.0·10 −6 m. Penetration of material is prevented by a further constraint that requires twice the wall thickness to be less than or equal to the edge length. An element whose cross-section reaches the lower limit of the permissible range does not change the global results because of its low stiffness and low mass, but would regularize the stiffness matrix. Recall that the goal of the study is not to propose a directly buildable adaptive structure but to learn about adaptive structures. To keep things simple, the cross-section and the material model are assumed to be constant along the element axis in passive and in active elements. Additional masses of actuators are not considered here.
3.1. Example 1: Truss Design
As the first example, a structure is chosen that is inspired by high-rise buildings. The basic structure is shown in the left diagram of Figure 2 . A similar structure was investigated by Geiger et al. (2020a) . In a nutshell, their findings are that stiffness-governed design problems can be transformed into strength-governed design problems by manipulating the deformation state of the structure using active elements. This was also shown in Senatore et al. (2018) , for example. For statically indeterminate structures, it is shown that the additional internal forces due to internal constraints, which arise from introducing a length change of an element as actuation, can be manipulated by additional actuators. If enough additional actuators are chosen, the reduction of the additional internal forces to zero is included in the design space but is not necessarily the optimal solution. This is also known as the introduction of “impotent eigenstrain”; see Furuhashi and Mura (1979) . The results of the structural optimizations show that the necessary amount of material for the primary structure can be reduced significantly. Mass-saving potentials of 65–70% are achievable by structural adaptation in such cases. Results of this magnitude were also reported by Senatore et al. (2019) .
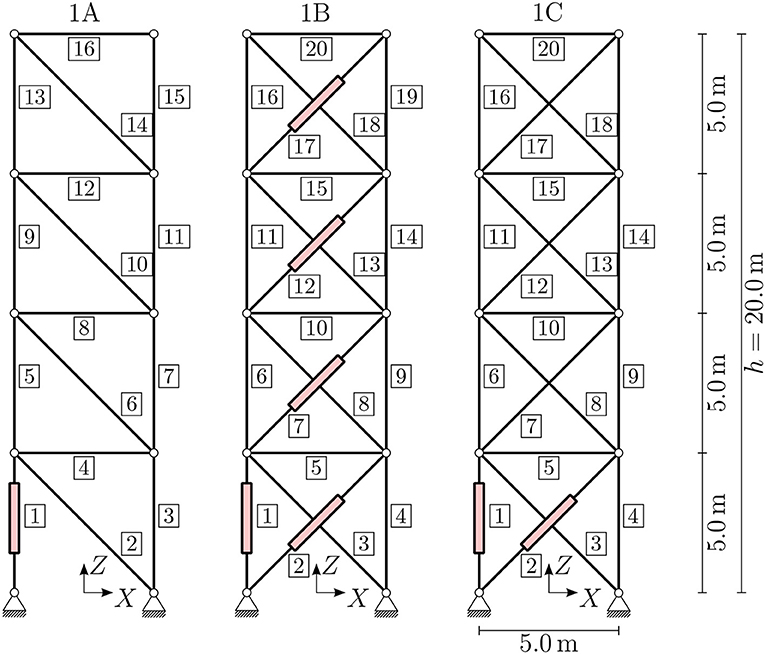
Figure 2 . Example 1, truss design: investigated variants with dimensions and actuator positions.
To obtain meaningful results for the mass-saving potential, three load cases are applied; these are shown in Figure 3 . Two of the load cases (red and green) can be interpreted as wind loads with constant values of ±15.0 kN/m in the X -direction along the height of the structure applied as nodal loads of ±75.0 and ±37.5 kN. The third load case (blue) is an additional load of −20.0 kN/m in the Z -direction on the horizontal floors, which results in nodal loads of 50 kN. All load cases are simulated separately. For simplicity, neither superposition nor safety factors are assumed. Additionally, the dead load of the members depending on the actual size of the cross-sectional area is considered in all load cases. For this example, horizontal deformations at all nodes, D c = D horiz , are constrained to a maximum absolute value of d max = h /500 = 20 m/500 = 0.04 m, which is a reasonable assumption in high-rise design. Additional assumptions on, for example, inter-story drift or maximum accelerations are not considered here.
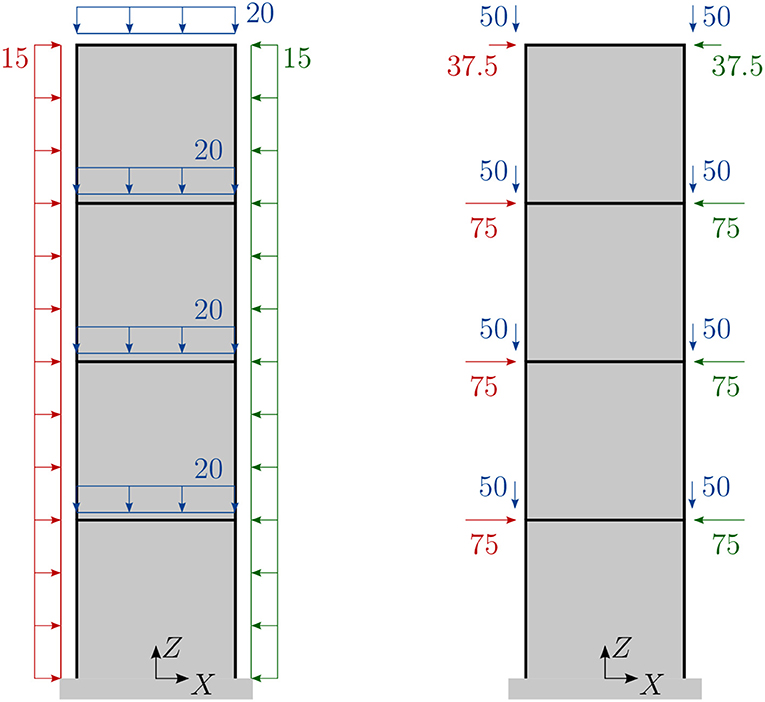
Figure 3 . Example 1, truss design: three investigated load cases (red, green, and blue), with line loads in kN/m and nodal forces in kN.
The first hypothesis arises from considering the essence of the design problem at hand. The resulting structure is subject to strict constraints on the allowable displacements, which are globally decisive for the dimensions of the cross-sections. Therefore, the aim of the actuation is efficient manipulation of the displacements. This implies the first hypothesis, H1, which is formulated as: “ Statically determinate structures are advantageous compared to statically indeterminate one if an adaptive structure is used to solve a stiffness-governed design problem.” The second hypothesis applies when statically indeterminate structures are investigated. The question is whether it is sufficient to enable constraint-free manipulation of the displacements, or if additional actuators are needed to enable actuation of the stress state in the structure. Therefore, the second hypothesis, H2, is formulated as: “ Implementing only as many actuators as necessary to enable constraint-free manipulation of the displacements performs worse than implementing n S additional actuators to manipulate all forces arising from internal constraints.”
To test these hypotheses, several structures are compared. The basic structure, variant 1A, is shown in the left diagram of Figure 2 . The figure also shows element numbers in rectangles. The truss structure is planar and consists of 16 single truss elements. Integrated actuators are shown in the figure. To determine actuator placement for the compensation of occurring deformations, methods from Wagner et al. (2018) are applied. A single actuator in element 1 is sufficient to restore about 95% of all deformations to the mean value for arbitrary load cases; therefore this actuator is chosen. As variant 1A is statically determinate, no additional actuators are necessary to control internal forces arising from internal constraints.
Variant 1B is shown in the middle diagram of Figure 2 . This variant incorporates additional diagonals into the basic structure to obtain a statically indeterminate structure. Following e.g., Senatore et al. (2019) , for variant 1B with static indeterminacy of degree 4, a total of four additional actuators have to be implemented to manipulate all internal forces arising from internal constraints (cf. Pellegrino and Calladine, 1986 ). Therefore, variant 1B has a prescribed number of five actuators, placed as shown in Figure 2 (middle). All additional elements are actuators. Variant 1C has the same topology as variant 1B but only one additional actuator to manipulate the internal forces arising from actuation. Additional information on actuator placement with the aim of compensating for introduced internal constraints can be found in Geiger et al. (2020a) .
Results for the necessary mass of variant 1A in the passive state, displayed in Figure 4 and Table 1 , show that in order to deal with the constraints on the horizontal displacements, the stiffness of the statically determinate passive truss structure has to be very high. If the truss were simplified to a vertical cantilever beam, the moment of inertia of the cross-section at the basement would have to be large enough to keep the deformations small. Therefore, the cross-sectional areas in the corresponding members are very large in the passive designs. Figure 5 (1A passive) shows that it is not possible to exploit the maximum possible strength in all members. The amount of mass required is determined by the required axial stiffness.
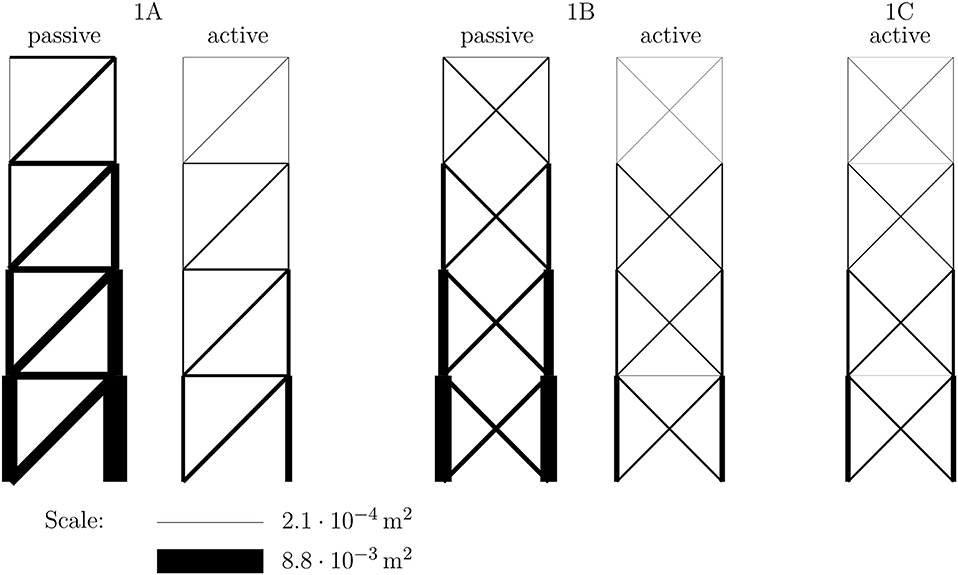
Figure 4 . Example 1, truss design: optimized cross-sectional areas.
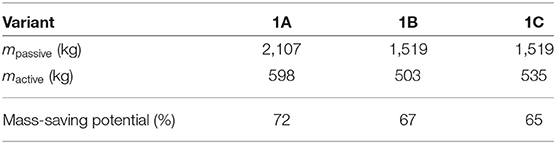
Table 1 . Example 1, truss design: total masses and mass-saving potentials (additional actuator mass not considered).
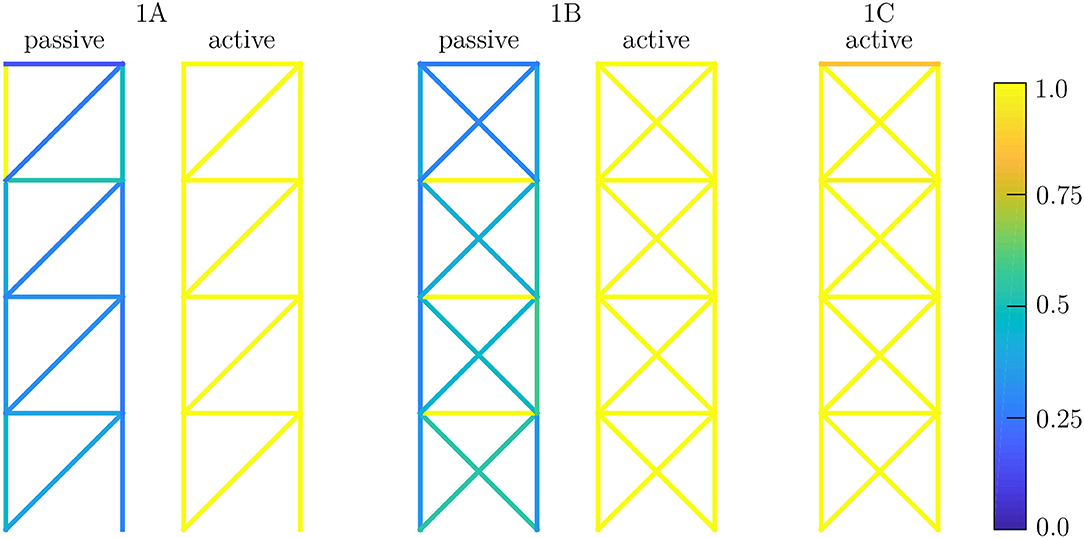
Figure 5 . Example 1, truss design: maximum utilization, envelope over all load cases.
The corresponding results for the adaptive structure 1A are shown in Figure 4 (1A active) and Table 1 . By incorporating the actuator in element 1, which can efficiently manipulate the horizontal displacements, it is now possible to satisfy the displacement constraints without large axial stiffness of the trusses. Therefore, the cross-sections can be utilized with their maximum strength capacity [see Figure 5 (1A active)], and this comes with a significant reduction in cross-sectional areas and mass.
Analogous observations are obtained for variant 1B. In order to check the first hypothesis, the results for the variants are compared with each other. The mass-saving potential reduces from 72% for variant 1A to 67% for variant 1B, but the total minimum mass also decreases from 598 to 503 kg, or around 15% relative to variant 1A. It can therefore be concluded that in this case hypothesis H1 does not hold. The general validity of this finding needs to be investigated in further work. Even though in both cases almost all elements are fully utilized in at least one load case, the statically indeterminate structure can carry the load to the supports using less material than the statically determinate variant. The intrinsically constraint-less actuation of the statically determinate case is not beneficial. Owing to actuation, the statically indeterminate structure also offers the possibility of constraint-free actuation. Additionally, several possible “load paths” are available to carry the load to the supports, and the manipulation of the internal constraint enables triggering of a normal force distribution that can be carried with less mass. This is not yet a universal design guideline, but it is a remarkable and somewhat counter-intuitive observation. Another notable observation is that for any actuator set, by using extra actuators starting from variant 1B no additional savings are possible. The complete actuation subspace is spanned by the chosen actuators, and any additional actuator is redundant.
Taking into account the results for variant 1C, it is found that the mass can be reduced by about 60 kg compared to variant 1A, but the minimum-weight design is not possible with only these two actuators. Figure 5 (1C active) shows that not all members are utilized 100%. The mass can be reduced further by introducing additional actuators. Although actuation of element 1 can be constraint-free by a suitable action of the second actuator, the absolute mass minimum cannot be attained. To obtain the structure with the least amount of material, the state of internal forces also has to be manipulated by using additional actuators in statically indeterminate structures. This is in line with the findings from comparing the statically determinate case 1A, where member forces cannot be manipulated, with case 1B, where all of the internal constraints can be manipulated by adaptation. It can therefore be deduced that hypothesis H2 holds in this case.
Already for passive structures, the mass needed to cope with all constraints in all load cases can be reduced by 28% if the structure's degree of static indeterminacy is 4 instead of 0. Considering only a single load case without constraints on the displacements, the design with the least amount of mass would be statically determinate (see Kirsch, 1991 ). However, considering multiple load cases with constrained deformations, the additional internal constraint provides different “load paths” for carrying the external forces to the supports, and the extra stiffness introduced by the additional members is beneficial to meeting the constraints on the displacements.
3.2. Example 2, Frame Design
The second example through which we aim to investigate and learn the optimal design of adaptive structures is a frame structure with the same outer dimensions as the truss in Example 1. The applied nodal loads are taken from the previous example and shown in the right diagram of Figure 3 . Bearing in mind that high-rise buildings are being considered, we adopt the commonplace assumption that distributed loads are transferred by a secondary structure, such as a facade, to the nodes, where they act as concentrated forces. The restrictions on horizontal deformations from the first example are also assumed to hold in the present example. Again a basic configuration, variant 2A, is defined, which is shown in the left diagram of Figure 6 . The basic configuration in this case is statically indeterminate to degree 12.
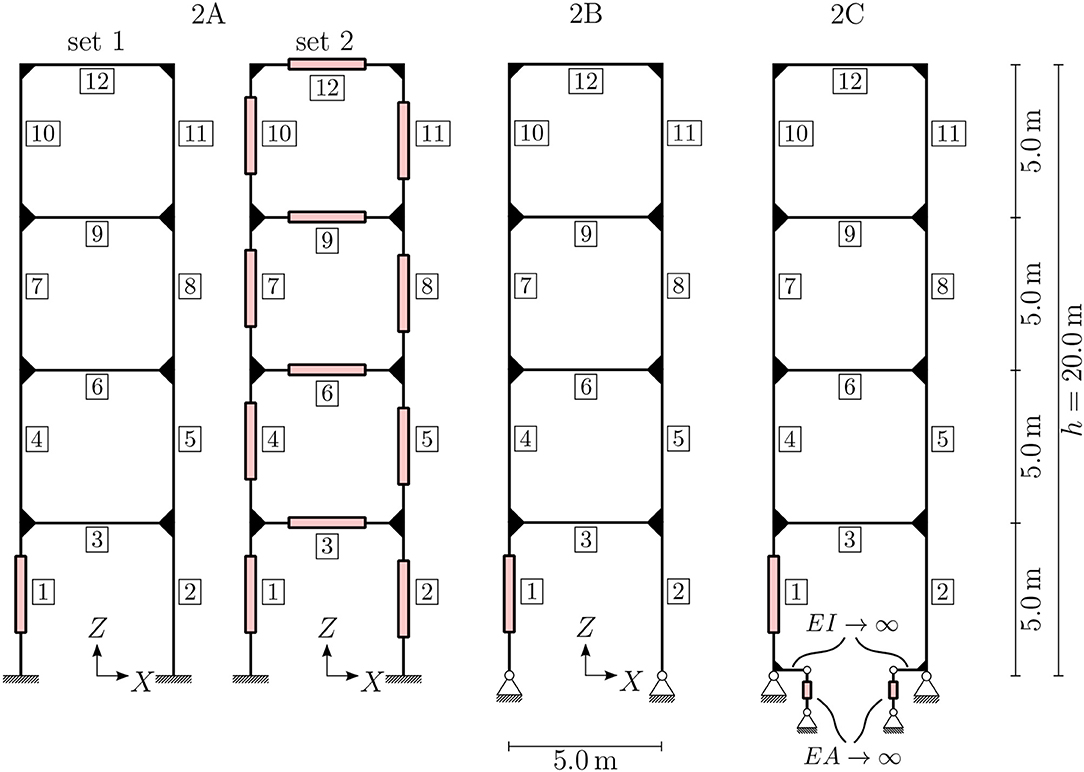
Figure 6 . Example 2, frame design: investigated variants with dimensions and actuator positions.
The proof of stability for all beams is simplified on the safe side. For the computation of Euler's critical buckling load it is assumed that all beams are hinged at both ends and that the beam element with the smallest bending stiffness is decisive for the computation of the critical buckling load. The first assumption neglects the bending stiffness of the rigid joints; if all stiffnesses were correctly taken into account, the resistance of the beams against buckling would be higher. Therefore, the assumptions are on the safe side in this case. Regardless of these assumptions, the computations show that member buckling is not critical for design in the investigated cases. As mentioned before, no distributed loads are applied, so linearly varying bending moments are expected in the structure. To approximate a linearly varying cross-section along one beam, the beam is discretized using 10 beam finite elements of constant cross-section. Further improvements could be achieved by the introduction of e.g., linearly varying cross-sections or a finer discretization of the beam. In the following, the terms beam element and element specify a single finite element, and a beam connecting two loaded or supported nodes consists of 10 beam elements. Figure 6 shows the positions of the actuators in varying beams of the frame structures. Since it suffices for only one element of a beam to be an active element, the exact position of the active element within one beam is not shown.
For frame structures, which are very typical for e.g., multi-story buildings, the degree of static indeterminacy is typically greater than for simple truss structures. The number of actuators needed to control all internal forces arising from constraints is significantly higher than for trusses. The assumption that only linear actuators in the central axis of an element are available is not suitable for frame structures, because, in contrast to truss structures, the degree of static indeterminacy in frame structures can be greater than the number of elements. This leads to the hypothesis H3 for beam structures: “ Actuation using only axial linear actuators is not sufficient to enable a well-performing adaptive frame structure. Direct manipulation of bending moments and transverse shear forces is necessary.”
In order to check this hypothesis, several variants of the structure are introduced. For the basic configuration, 2A, two actuator sets are compared. Set 1 has only one actuator, which can manipulate the displacements but will introduce additional internal forces due to internal constraints in the structure. Set 2 has a total of 12 actuators, one in each beam, to test whether it is possible to control the internal constraint completely. The setup of variant 2B is inspired by insights into load-bearing behavior from a structural mechanics point of view. The actuation of the structure can be constraint-free when using hinged supports, as shown in the middle diagram of Figure 6 . The axial forces in both columns are statically determinate now, so there is no additional force arising from actuating one of these elements.
The resulting cross-sections from the optimization processes are shown in Figure 7 (2A and 2B). Because of the missing clamping at the supports, and hence the missing stiffness for preventing large deformations, the variant 2B performs worse in terms of the amount of material used, although the adaptation is constraint-free. As shown in Table 2 , instead of 8,193 kg a total mass of 14,059 kg is needed to provide a functional passive building, and instead of 1,978 or 1,935 kg a new mass of 2,532 kg is needed for the adaptive structure. Relative to the passive structures, the mass-saving potentials of the adaptive structures are between 76 and 82%. This significant mass-saving potential can be traced back to the very inefficient load-bearing behavior of the passive construction. Horizontal deformation depends on the bending stiffness, which leads to an extensive need for material. Interestingly, the full actuation of the structure using set 2 does not yield significantly greater material savings.
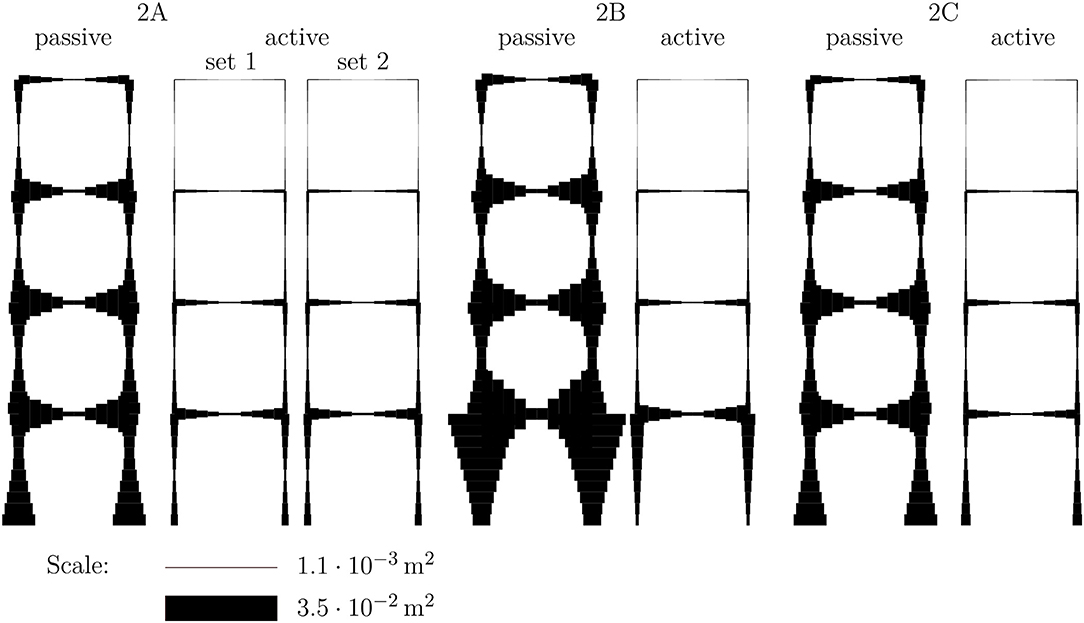
Figure 7 . Example 2, frame design: optimized cross-sectional areas.
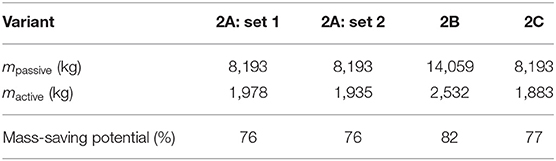
Table 2 . Example 2, frame design: total masses and mass-saving potentials (additional actuator mass not considered).
To overcome this issue, adaptive clamping of the support is investigated. This can be represented in the model by starting with variant 2 and introducing two additional actuators at the bottom of elements 1 and 2; see the right diagram of Figure 6 . These actuators can enable a constraint-free rotation of the beam at the support if they are controlled to introduce no actuation force. On the other hand, these actuators prevent (additional) rotation at the support if they are controlled to hold their actual stroke level. Therefore, variant 2C is an intermediate version between variants 2A and 2B. To preserve comparability of the results, the cross-section of these additional elements is taken to be very large compared to all the other elements and is assumed to be completely rigid. The mass of the additional elements is not considered, because the switchable clamping is part of the supporting structure, whose weight is also not considered in the other examples. Generally speaking, a clamping needs more mass than a hinged support.
The resulting structure after optimization of variant 2C is shown in the right diagram of Figure 7 . The switchability of the clamping in the support offers the possibility of constraint-free manipulation of the displacements with the actuator in element 1, combined with the additional stiffness of the clamping. Therefore, this results in the adaptive structure having a total mass of 1, 883 kg, which is 26% less than variant 2B and even 3% less than variant 2A. Even for a structure that is packed with active elements, such as variant 2A with actuator set 2, optimal performance in terms of mass-saving cannot be achieved. Actuators for direct manipulation of the bending moments or transverse shear forces have to be introduced. The workaround we have introduced provides an easy way to model an actuator that can apply a bending moment at the support. It can be concluded that hypothesis H3 holds in this case.
4. Conclusions and Further Work
New challenges arise in the design of adaptive structures compared to the design of passive structures. For classical passive structures, it is well-known how to lay out a structure so that it is efficient, reliable, redundant, cheap, etc. With adaptive elements coming into play, using intuition gained from the design of passive structures may lead to suboptimal results, because such intuition does not fully take into account the particular characteristics of adaptive structures. The two academic examples investigated in this study demonstrate the changes in the requirements, which are not necessarily obvious. The results represent an important step toward developing the needed design guidelines, although the process is still far from complete. The results presented in this paper can help to advance research into the optimal number and placement of actuators, while maintaining the reliability of the whole adaptive structure and, of course, controlling its cost. The results also highlight some opportunities that are opened up by the introduction of adaptive civil structures. Significant mass savings can be achieved, and serviceability can also be improved.
The results of this study provide new insights into the nascent field of designing adaptive structures. The first example investigates the incorporation of actuators into passive truss structures, and the second example examines a similar procedure for frame structures. For both of the investigated structures, significant mass-saving potentials of more than 65% in the primary structure can be achieved by using actuators, compared to the passive version of the structure, under the given assumptions. The potential savings are greater for the frame structure than for the truss structure. These results apply to the utilization of adaptive structures in stiffness-governed design tasks. The examples look at only several different variants and do not yet provide a genuine process for designing an adaptive structure. More investigations have to be carried out in order to formulate a suitable design process. The aim would not be to design passive structures and make them adaptive afterwards, but rather to design adaptive structures in an integrative design process; this would be an important continuation of the present study to a more applicable setting.
For future work, the investigation of different actuation concepts for frame structures would be the most important extension of the present study. Therefore, non-linear actuation and elements for bending and shear actuation also need to be taken into account. The inclusion of non-linear effects for beam actuators represents the next challenge in the optimization of adaptive structures, because optimized adaptive structures are getting more and more slender and hence non-linearities have to be considered in the simulation process. Additionally, it is planned to extend these investigations to plates and shells.
Data Availability Statement
All datasets generated for this study are included in the article/supplementary material.
Author Contributions
This research was initiated and globally supervised by MB. FG and JG developed the modeling, design strategies, and formulation, as well as executed the optimization processes together. FG contributed the selection and evaluation of the case study. The modeling and the case study were supervised by MB. MS supervised the optimization part. The first draft of the paper was written by FG. All authors actively revised, reviewed, and approved the final submission.
The work described in this paper was conducted in the framework of the Collaborative Research Center 1244 Adaptive Skins and Structures for the Built Environment of Tomorrow, within the projects A04 Form finding, structural optimization, and system optimization and B01 Characterization, modeling, and model order reduction, funded by the Deutsche Forschungsgemeinschaft (DFG, German Research Foundation) under project number 279064222. The authors were grateful for the generous support.
Conflict of Interest
The authors declare that the research was conducted in the absence of any commercial or financial relationships that could be construed as a potential conflict of interest.
Fletcher, R. (2013). Practical Methods of Optimization . Chichester: John Wiley & Sons, Ltd.
Google Scholar
Frecker, M. I. (2003). Recent advances in optimization of smart structures and actuators. J. Intell. Mater. Syst. Struct . 14, 207–216. doi: 10.1177/1045389X03031062
CrossRef Full Text | Google Scholar
Fröhlich, B., Gade, J., Geiger, F., Bischoff, M., and Eberhard, P. (2019). Geometric element parameterization and parametric model order reduction in finite element based shape optimization. Comput. Mech . 63, 853–868. doi: 10.1007/s00466-018-1626-1
Furuhashi, R., and Mura, T. (1979). On the equivalent inclusion method and impotent eigenstrains. J. Elast . 9, 263–270. doi: 10.1007/BF00041098
Geiger, F., Gade, J., von Scheven, M., and Bischoff, M. (2020a). “Anwendung der Redundanzmatrix bei der Bewertung adaptiver Strukturen [Application of the redundancy matrix in the assessment of adaptive stuctures],” in Baustatik-Baupraxis , eds B. Oesterle, M. von Scheven, and M. Bischoff, Vol. 14 (Stuttgart: Institute for Structural Mechanics, University of Stuttgart), 119–128.
Geiger, F., Gade, J., von Scheven, M., and Bischoff, M. (2020b). Optimal Design of Adaptive Structures vs. Optimal Adaption of Structural Design . Berlin: IFAC-PapersOnLine.
Hale, A. L., Lisowski, R. J., and Dahl, W. E. (1985). Optimal simultaneous structural and control design of maneuvering flexible spacecraft. J. Guid. Control Dyn . 8, 86–93. doi: 10.2514/3.19939
Kawaguchi, K.-I., Hangai, Y., Pellegrino, S., and Furuya, H. (1996). Shape and stress control analysis of prestressed truss structures. J. Reinf. Plast. Compos . 15, 1226–1236. doi: 10.1177/073168449601501204
Kirsch, U. (1991). Optimal design of structural control systems. Eng. Optim . 17, 141–155. doi: 10.1080/03052159108941066
Kirsch, U., and Moses, F. (1977). Optimization of structures with control forces and displacements. Eng. Optim . 3, 37–44. doi: 10.1080/03052157708902375
Korkmaz, S. (2011). A review of active structural control: challenges for engineering informatics. Comput. Struct . 89, 2113–2132. doi: 10.1016/j.compstruc.2011.07.010
Kwan, A., and Pellegrino, S. (1993). Prestressing a space structure. AIAA J . 31, 1961–1963. doi: 10.2514/3.11876
Martins, J. R. R. A., Sturdza, P., and Alonso, J. J. (2003). The complex-step derivative approximation. ACM Trans. Math. Softw . 29, 245–262. doi: 10.1145/838250.838251
Melosh, R. J. (1963). Basis for derivation of matrices for the direct stiffness method. AIAA J . 1, 1631–1637. doi: 10.2514/3.1869
Pellegrino, S., and Calladine, C. (1986). Matrix analysis of statically and kinematically indeterminate frameworks. Int. J. Solids Struct . 22, 409–428. doi: 10.1016/0020-7683(86)90014-4
Senatore, G., Duffour, P., and Winslow, P. (2018). Energy and cost assessment of adaptive structures: case studies. J. Struct. Eng . 144:04018107. doi: 10.1061/(ASCE)ST.1943-541X.0002075
Senatore, G., Duffour, P., and Winslow, P. (2019). Synthesis of minimum energy adaptive structures. Struct. Multidiscipl. Optim . 60, 849–877. doi: 10.1007/s00158-019-02224-8
Senatore, G., Duffour, P., Winslow, P., and Wise, C. (2017). Shape control and whole-life energy assessment of an ‘infinitely stiff’ prototype adaptive structure. Smart Mater. Struct . 27:015022. doi: 10.1088/1361-665X/aa8cb8
Soong, T. (1988). State-of-the-art review: active structural control in civil engineering. Eng. Struct . 10, 74–84. doi: 10.1016/0141-0296(88)90033-8
Soong, T. T., and Manolis, G. D. (1987). Active structures. J. Struct. Eng . 113, 2290–2302. doi: 10.1061/(ASCE)0733-9445(1987)113:11(2290)
Soong, T. T., and Spencer, B. F. (1992). Active structural control theory and practice. J. Eng. Mech . 118, 1282–1285. doi: 10.1061/(ASCE)0733-9399(1992)118:6(1282)
Squire, W., and Trapp, G. (1998). Using complex variables to estimate derivatives of real functions. SIAM Rev . 40, 110–112. doi: 10.1137/S003614459631241X
Teuffel, P. (2004). Entwerfen Adaptiver Strukturen (Ph.D. thesis). Stuttgart: Universität Stuttgart.
Utku, Ş. (1998). Theory of Adaptive Structures: Incorporating Intelligence Into Engineered Products. New Directions in Civil Engineering . Boca Raton, FL: CRC Press.
Wagner, J. L., Gade, J., Heidingsfeld, M., Geiger, F., von Scheven, M., Böhm, M., et al. (2018). On steady-state disturbance compensability for actuator placement in adaptive structures. At Automatisierungstech . 66, 591–603. doi: 10.1515/auto-2017-0099
Weidner, S., Kelleter, C., Sternberg, P., Haase, W., Geiger, F., Burghardt, T., et al. (2018). The implementation of adaptive elements into an experimental high-rise building. Steel Constr . 11, 109–117. doi: 10.1002/stco.201810019
Zuk, W., and Clark, R. H. (1970). Kinetic Architecture . New York, NY: Van Nostrand Reinhold.
Keywords: adaptive structures, structural optimization, integrative design approach, actuator modeling, adaptive truss, adaptive frame
Citation: Geiger F, Gade J, von Scheven M and Bischoff M (2020) A Case Study on Design and Optimization of Adaptive Civil Structures. Front. Built Environ. 6:94. doi: 10.3389/fbuil.2020.00094
Received: 01 April 2020; Accepted: 26 May 2020; Published: 30 July 2020.
Reviewed by:
Copyright © 2020 Geiger, Gade, von Scheven and Bischoff. This is an open-access article distributed under the terms of the Creative Commons Attribution License (CC BY) . The use, distribution or reproduction in other forums is permitted, provided the original author(s) and the copyright owner(s) are credited and that the original publication in this journal is cited, in accordance with accepted academic practice. No use, distribution or reproduction is permitted which does not comply with these terms.
*Correspondence: Florian Geiger, geiger@ibb.uni-stuttgart.de
Disclaimer: All claims expressed in this article are solely those of the authors and do not necessarily represent those of their affiliated organizations, or those of the publisher, the editors and the reviewers. Any product that may be evaluated in this article or claim that may be made by its manufacturer is not guaranteed or endorsed by the publisher.
Engineering Case Studies
- Getting Started
- Finding Case Studies in the U-M Library
- Case Studies That Are Freely Available
- Selected Case Studies Books
Open Access Case Studies
- Case Studies in Construction Materials Open access journal on case studies
- Case Studies in Engineering Failure Analysis Open access journal on case studies
- MIT Management Sloan School Case Studies Find case studies on Operations Management, Sustainability, Entrepreneurship, and others in the MIT Sloan School Teaching Resources Library.
- Open Case Studies from the University of British Columbia Case studies on a range of topics including Civil Engineering, Conservation, Forestry, and Soil Science
- Plant Engineering Case Studies Case studies about industrial plants and facilities
- Standardization Case Studies from ANSI This American National Standards Institute website shares case studies where standardization helped with real-world problems.
- Texas Tech University, Murdough Center for Engineering Professionalism Cases published by Dr. Ron Bucknam of the University of Washington that are based on real world situations and, for the most part, reflect his experiences during more than 40 years of consulting engineering experience. Ideas for other cases came from the program's Board of Review which consists of practicing engineers and faculty throughout the country.
- << Previous: Finding Case Studies in the U-M Library
- Next: Selected Case Studies Books >>
- Last Updated: Aug 28, 2024 12:02 PM
- URL: https://guides.lib.umich.edu/engrcasestudies
- Help & FAQ
Failure case studies in civil engineering: Structures, foundations, and the geoenvironment, second edition
- Architectural Engineering
Research output : Book/Report › Book
Failure Case Studies in Civil Engineering: Structures, Foundations, and the Geoenvironment, Second Edition, provides short descriptions of 50 real-world examples of constructed works that did not perform as intended. Designed for classroom use, each case study contains a brief summary, lessons learned, and references to key sources. This new edition, which replaces the 1995 classic edited by Robin Shepherd and J. David Frost, offers expanded descriptions, additional photographs and diagrams, and updated references. It also includes new case studies, such as the Alfred P. Murrah Federal Building, the Charles de Gaulle Airport Terminal, and the North Battleford, Saskatchewan, Water Treatment Plant.
Original language | English (US) |
---|---|
Publisher | |
Number of pages | 126 |
ISBN (Electronic) | 9780784477038 |
ISBN (Print) | 9780784412558 |
State | Published - Jan 1 2012 |
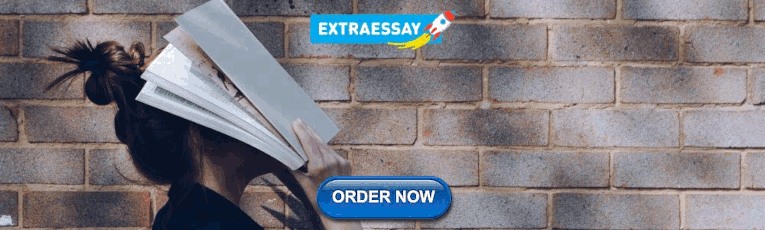
All Science Journal Classification (ASJC) codes
- General Engineering
Other files and links
- Link to publication in Scopus
- Link to the citations in Scopus
Fingerprint
- Water treatment plants Engineering & Materials Science 100%
- Civil engineering Engineering & Materials Science 71%
- Airports Engineering & Materials Science 67%
T1 - Failure case studies in civil engineering
T2 - Structures, foundations, and the geoenvironment, second edition
AU - Bosela, Paul A.
AU - Brady, Pamalee A.
AU - Delatte, Norbert J.
AU - Kevin Parfitt, M.
N1 - Publisher Copyright: © 2013 by the American Society of Civil Engineers. All Rights Reserved.
PY - 2012/1/1
Y1 - 2012/1/1
N2 - Failure Case Studies in Civil Engineering: Structures, Foundations, and the Geoenvironment, Second Edition, provides short descriptions of 50 real-world examples of constructed works that did not perform as intended. Designed for classroom use, each case study contains a brief summary, lessons learned, and references to key sources. This new edition, which replaces the 1995 classic edited by Robin Shepherd and J. David Frost, offers expanded descriptions, additional photographs and diagrams, and updated references. It also includes new case studies, such as the Alfred P. Murrah Federal Building, the Charles de Gaulle Airport Terminal, and the North Battleford, Saskatchewan, Water Treatment Plant.
AB - Failure Case Studies in Civil Engineering: Structures, Foundations, and the Geoenvironment, Second Edition, provides short descriptions of 50 real-world examples of constructed works that did not perform as intended. Designed for classroom use, each case study contains a brief summary, lessons learned, and references to key sources. This new edition, which replaces the 1995 classic edited by Robin Shepherd and J. David Frost, offers expanded descriptions, additional photographs and diagrams, and updated references. It also includes new case studies, such as the Alfred P. Murrah Federal Building, the Charles de Gaulle Airport Terminal, and the North Battleford, Saskatchewan, Water Treatment Plant.
UR - http://www.scopus.com/inward/record.url?scp=85018565774&partnerID=8YFLogxK
UR - http://www.scopus.com/inward/citedby.url?scp=85018565774&partnerID=8YFLogxK
AN - SCOPUS:85018565774
SN - 9780784412558
BT - Failure case studies in civil engineering
PB - American Society of Civil Engineers (ASCE)

Engineering Databases
- Academic Complete E-books
- Academic Search Complete
- Access Engineering
- ACM Digital Library
- American Chemical Society
- Applied Science and Technology Source
- ASM Handbooks
- ASME Digital Collection
- ASTM Compass Standards
- Auto Repair Source
- AVON - Academic Video Online
- Dictionary of Natural Products
- Digital Commons
- EBSCO eBooks Collection
Engineering Case Studies
- Google Scholar
- Grammarly Premium
- IEEE Xplore
- Journal Citation Reports
- Journal List A-Z
- JSTOR Collection
- Knovel Science & Engineering Datasets
- LinkedIn Learning
- MeLCat: Music, Books, Audio & More from all over Michigan
- SAE Mobilus
- Science Direct
- Small Engine Repair Reference Center
- Springer Journals and eBooks
- Web of Science
- WorldCat: Books, DVDs, CDs & Articles from around the world
- About the Database
- Creating Persistent Links

Creating a Persistent Link:
Engineering Case Studies has an option for persistent links inside each resource.
To locate the persistent link, select the resource you wish to share. Beneath the resource, you will see an option to "embed/link":

From here, you can choose to copy the persistent link from the pop-up window:

Login or register for a MyICE account
- Back What is civil engineering
- Civil engineering explained
- Infrastructure projects
- Meet the engineers
- Back Your career
- How to become a professionally qualified engineer
- Professional qualification support
- Continuing professional development
- Career FAQs
- Education resources
- Back Areas of interest
- Buildings and structures
- Coastal and offshore engineering
- Decarbonisation
- Development, planning and urban engineering
- Geology, geotechnical and ground engineering
- Infrastructure delivery
- Transportation
- Back Events
- Upcoming events
- Previous events
- Professional qualification training
- Recorded lectures
- Exhibitions
- Back News, views & insights
- Policy and advocacy
- Latest news
- Inside infrastucture
- Media centre
- Back About us
- Membership benefits
- Our organisation
- Our services
- Our members
- Back Get involved
- Volunteering
- Specialist knowledge societies
- Share your expertise
- For employers
- Awards and grants
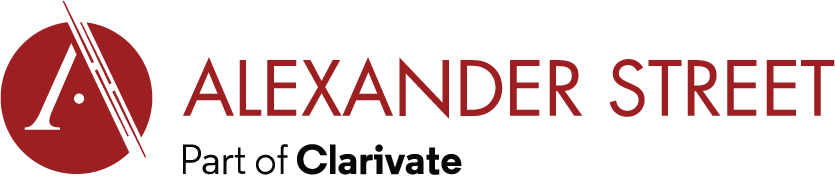
Engineering Case Studies Online
- Disciplines
Engineering Events
- Organizations
- Content Types
- Mechanical engineering (120)
- Aerospace engineering (86)
- Civil engineering (83)
- Structural engineering (63)
- Petroleum engineering (49)
- Aircraft accidents (48)
- Bridges (39)
- Nuclear engineering (37)
- Oil mines and mining (36)
- Airplanes (34)
- Chemical engineering (34)
- Deepwater Horizon Oil Spill (BP) (72)
- Fukushima Daiichi Nuclear Disaster (61)
- Chernobyl Disaster (26)
- Piper Alpha Accident (18)
- Titanic (18)
- Minneapolis Bridge Collapse (17)
- Space Shuttle Challenger Disaster (17)
- Air France Flight 4590 (15)
- Hyatt Regency Walkway Collapse (14)
- Tacoma Narrows Bridge Collapse (14)
- Bhopal Gas Disaster (13)
- TWA Flight 800 (12)
- Apollo 13 (10)
- Ford Pinto Controversy (10)
- Big Dig Ceiling Collapse (9)
- BOAC Flight 781 (8)
- September 11 Attacks (7)
- Texas City Refinery Explosion (BP) (5)
- Esso Longford Gas Explosion (4)
- Ashtabula River Railroad Disaster (3)
- Hindenburg (3)
- King's Cross Fire (3)
Featured Playlists
- Airplane Crashes: Causes and Effects (28)
- Apollo 13: Eyewitness Testimony and Primary Footage (20)
- Investigation into the Challenger Disaster (26)
- Nuclear Fallout from Chernobyl (7)
Engineering Case Studies Online will dramatically improve teaching and research by providing a single, comprehensive source for a wide range of video and text material focusing on engineering failures and successes. The collection covers over 300 videos and 70,000 pages of quality documentaries, accident reports, experiments, visualizations, case studies, lectures and interviews from leading engineering institutions around the world.
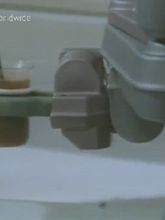
Robots: Taking the Biscuit
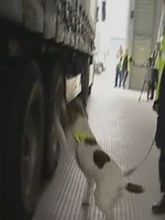
Tech Tools: Law and Order
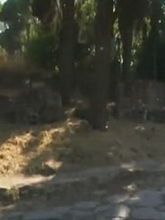
Ancient Rome: The Mobile Society
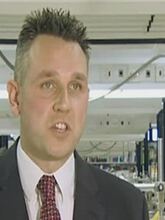
Footprint database
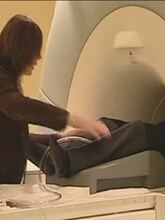
Magnetic resonance imaging (MRI)

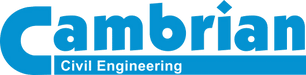
- Press Releases
- Case Studies
- Policy Documents
Case Studies of Civil Engineering Projects
Copyright © 2024 CAMBRIAN CIVIL ENGINEERING LTD - All Rights Reserved.
This website uses cookies.
We use cookies to analyze website traffic and optimize your website experience. By accepting our use of cookies, your data will be aggregated with all other user data.
Case topic suggestions
The Engineering Cases group believes that through cases, students will improve their ability to learn and retain concepts in their courses, on work terms and in their professional lives. One of the best means to create case studies is by converting them from student-generated work reports. As a result, it is in our best interest to ensure that work reports submitted to our group contain an adequate design process and topics that align with topics that professors have suggested would benefit from case studies. We also believe that students will benefit by having suggestions for work term report topics.
Engineering Cases welcomes any submitted work reports, regardless of topics; however, work reports that use one of the sample work report topics below would be very much appreciated!
Sample work report topics
Mechanical Engineering
- Stress analysis of prismatic members in axial, shearing, torsional and flexural deformations
- Force analysis of structures and structural components
- Hydrostatics
- Flow similarity and dimensional analysis as applied to engineering problems in fluid mechanics
- Thermodynamics, forensics based
- Machine design
- Linear vibrations
Civil and Environmental Engineering
- Simulation of Real Earthen Dams
- Slope stability, retaining walls or foundations
- Concrete flooring
- Waste to value-added product production using microorganisms
- Sustainability topics
- Geotechnical
- Structural Analysis 1
- Structure and properties of materials
Electrical and Computer Engineering
- Software development life cycle
- Project scheduling
- Maintenance considerations
- Assembly language programming
- Peripherals
- Digital Circuits and systems
- Electronic circuits and devices
- Control systems
- Circuit analysis and design
Systems Design Engineering
- Design for manufacturing and assembly
- Safety and responsibility in engineering design
- Automatic Process Control and Instrumentation
Chemical Engineering
- Bioseparations/Bioprocessing
- Fermentation
- Non-steady state operation, rate data analysis and collection
- Non-isothermal reactor design, energy balances
- Fuel Cells , battery testing and battery design
- Heat exchangers - double pipe, shell-and-tube and plate
- Electrowinning
- Batch Distillation
- Microbe growth and control
- Bomb Calorimetry
- Gas Absorption
- Statistical analysis, design of experiments, probability
- Engineering design process or synthesis
- Material sciences
- Manufacturing processes
- Food process engineering
- Process safety
- Life cycle analysis
Software Engineering
- Software Requisition: Specification and Analysis
- Software Test/Quality Assurance and maintenance
- Computer networks and security
Nanotechnology Engineering
- Microfluidics
- Nanotechnology ethics , law, and sustainability
- Polymer selection and testing
Management Engineering
- Bullwhip effect and supply chain management
- Double, triple integral and ordinary differential equation
- Finite element methods
- Numerical methods
- Heat transfer/Energy Conversion
- Thermodynamics
- Fluid Mechanics
Submit your ideas for useful work report topics
If you have any topics that can be added to the list above, please Contact Us ! Thank you!

Related links
- Submit work term report
- Case writing opportunity
- Work report support
- Engineering competitions
- Professional Master of Engineering Design Diploma

Contact Waterloo Cases in Design Engineering Steve Lambert Tel: (519) 888-4728 Email: [email protected]
Contact us Website feedback
- Contact Waterloo
- Maps & Directions
- Accessibility
The University of Waterloo acknowledges that much of our work takes place on the traditional territory of the Neutral, Anishinaabeg, and Haudenosaunee peoples. Our main campus is situated on the Haldimand Tract, the land granted to the Six Nations that includes six miles on each side of the Grand River. Our active work toward reconciliation takes place across our campuses through research, learning, teaching, and community building, and is co-ordinated within the Office of Indigenous Relations .
- Programmes and prizes Engineering programmes, grants, awards and prizes Engineering X Programmes Safer Complex Systems
- Programmes and prizes
- Engineering programmes, grants, awards and prizes
- Engineering X
- Safer Complex Systems
Case studies

Engineering X commissioned the development of 18 unique case studies by awardees from across academia and industry about Safer Complex Systems
The Safer Complex Systems community identified a lack of case studies, which are a useful tool for education and professional development, on complex systems. To address this gap, Engineering X commissioned the development of 18 unique case studies by awardees from across academia and industry. These were developed over a period of 18 months by the awardees in collaboration with their own networks and under the mentorship of our expert case study steering committee, chaired by Professor Brian Collins CB FREng.
The case studies cover a wide variety of complex system successes and failures, past and present, around the world. In examining these events, these case studies provide insights into how the design, construction, operation, management and governance of complex systems may result in safe or unsafe outcomes. Beyond informing future activities under our programme, we hope that the cross-cutting factors and lessons learned brought to light by these case studies will help to create safer complex systems globally.
The Safer Complex Systems Case Study Collection, downloadable below, contains all 18 case studies, along with commentary on the background motivation to and lessons learned from the case studies.
Download all case studies
A summary of each case study, also available for individual download, as well as extended versions of the research conducted for some of these case studies are provided further below.
Cyber-physical system shortfalls in the 2011 Brisbane flood
By Dr Giuliano Punzo
Australian climate extremes and building transport network resilience
By Dr Kristen MacAskill, Dr Marlies Barendrecht, Dr Catherine Tilley
Planned Adaptive Regulation: Learnings from the Delta Programme
By Dr Richard Judge, Prof Arthur Petersen
A comparative study of fire risk emergence in informal settlements in Dhaka and Cape Town
By Danielle Antonellis, Laura Hirst, John Twigg, Sandra Vaiciulyte, Reasat Faisal, Melissa Spiegel, George Faller, Richard Walls, Natalia Flores, Birgitte Messerschmidt
Community evacuation from wildfire events
By Dr Steve Gwynne, Dr Georgia Bateman, Dr Enrico Ronchi, Afroza Mallick, Prof Guillermo Rein, Hannah Neville, Dr Max Kinateder, Amanda Kimball, Dr Erica Kuligowski
Towards a simpler and safer nuclear sector: The 2005 THORP Internal Leak
By Prof Francis Livens, Dr William Bodel
Bexley train crash – a system failure
By Dr Chris Elliott MBE FREng
Revisiting the causes of the Hatfield Rail Crash
By Prof Roger Kemp MBE FREng
A systems approach to reducing train accident risk
By Brian Tomlinson
Understanding and utilising data for a seasonally agnostic railway
By Dr Brian Haddock, Dr John Beckford
Ro Ro passenger ferry safety: The capsizing of the Herald of Free Enterprise
By Prof Chengi Kuo, Prof Dracos Vassalos
Towards intelligent dynamics of an active transport system for biking
By Prof Andrés Medaglia, Maria Wilches-Mogollon, Prof Olga Sarmiento, Dr Felipe Montes, Dr Luis Guzman, Prof Mauricio Sanchez-Silva, Prof Ronaldo Menezes, Dr Darío Hidalgo, Karla Parra, Andrés Useche,
Complex systemic failures in the Edinburgh Schools case
By Prof Jonathan Gosling, Prof Mohamed Naim, Prof Bill Hewlett, Stewart Macartney
Systemic failures in nursing home care
By Prof Joachim Sturmberg, Dr Len Gainsford, Prof Nicholas Goodwin, Prof Dimity Pond
Social innovators as a human sensing network solving humanitarian challenges of the XXI century
By Matías René Rojas De Luca
Humanitarian supply chains during COVID-19: systems failures, recovery and emerging alternatives
By Claire Travers, Anna Lowe
Improving resilience to major safety events by analysing case studies
By Prof Richard Taylor MBE, Dr Neil Carhart, Dr Graeme Collinson, Richard Voke, Dr John May, Dr Andrew Weyman
Beyond the boundaries: characterising situational uncertainty in complex systems
By Dr Richard Judge, Shirin Elahi
Long form case studies
The papers below contain extended research into topics covered by some of the case studies above.
Assessment of the position and quantity of shear walls their correlation with building height on the seismic nonlinear performance
- Published: 07 September 2024
Cite this article
- Akram Khelaifia 1 ,
- Ali Zine 1 ,
- Salah Guettala 1 &
- Rachid Chebili 1
This study addresses a crucial research gap by investigating the optimal position of shear walls, the ideal shear wall-floor area ratio in building design, and their correlation with building height using non-linear analysis (Static and Dynamic). The results, including capacity curves, inter-story drift, and performance levels from both nonlinear static analysis and nonlinear dynamic analysis, are explored. Adopting principles of performance-based seismic design, the study reflects a comprehensive approach to seismic analysis and mitigation. The findings underscore that elevating the shear wall ratio not only enhances structural rigidity but also improves reliability in terms of inter-story drift, playing a crucial role in achieving the desired performance level during the design process. For a 7-story structure, a 1.00% shear wall–floor ratio is crucial, while a 1.5% ratio is essential for a 14-story structure to meet design conditions. The study highlights the intricate interplay among shear wall–floor ratios, optimal shear wall positions, and their correlation with building height as pivotal factors or main criteria influencing performance and structural integrity. Additionally, the presence of shear walls adopting compound forms (Box, U, and L) enhances reliability, while incomplete shear walls within the frame degrade half-filled frame stiffness, impacting short beam integrity. Furthermore, the study confirms the reliability of both nonlinear dynamic analysis and nonlinear static analysis, providing valuable insights into optimizing building designs for enhanced structural performance.
This is a preview of subscription content, log in via an institution to check access.
Access this article
Subscribe and save.
- Get 10 units per month
- Download Article/Chapter or eBook
- 1 Unit = 1 Article or 1 Chapter
- Cancel anytime
Price includes VAT (Russian Federation)
Instant access to the full article PDF.
Rent this article via DeepDyve
Institutional subscriptions
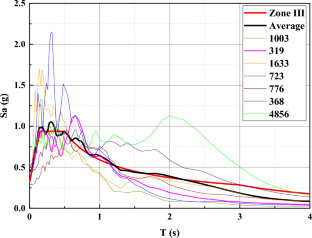
Data availability
No datasets were generated or analysed during the current study.
Abdesselam, I., Guettala, S., & Zine, A. (2024). Fiber-based modeling for investigating the existence of a soft storey for masonry infilled reinforced concrete structures. Asian Journal of Civil Engineering , 25 (2), 1949–1965. https://doi.org/10.1007/s42107-023-00887-9
Article Google Scholar
Ahamad, S., & Pratap, K. V. (2021). Dynamic analysis of G + 20 multi storied building by using shear walls in various locations for different seismic zones by using Etabs. Materials Today: Proceedings, 43 , 1043–1048. https://doi.org/10.1016/j.matpr.2020.08.014
Algerian Seismic Rules. (2003). Centre National De Recherche Appliquée en Génie Parasismique . Alger.
Almayah, A. A., & Taresh, R. G. (2019). Effect of shear wall location on the response of multistory buildings under seismic loads. International Journal of Scientific & Engineering Research , 10 (1), 1303–1311.
Google Scholar
Aminnia, M., & Hosseini, M. (2015). The effects of placement and cross-section shape of shear walls in multistory RC buildings with plan irregularity on their seismic behaviour by using nonlinear time history analyses. World Academy of Science Engineering and Technology Open Science Index 106 International Journal of Civil and Environmental Engineering , 9 (10), 1327–1334.
ATC-40 (1996). Seismic evaluation and retrofit of concrete buildings.
Burak, B., & Comlekoglu, H. G. (2013). Effect of Shear Wall Area to Floor Area ratio on the seismic behavior of Reinforced concrete buildings. Journal of Structural Engineering-ASCE , 139 (11), 1928–1937. https://doi.org/10.1061/(asce)st.1943-541x.0000785
Çavdar, Ö. (2019). Investigation of the earthquake performance of a Reinforced concrete Shear Wall Hotel using nonlinear methods. International Journal of Science and Engineering Applications . https://doi.org/10.7753/ijsea0812.1003
Djafar-Henni, N., & Chebili, R. (2023). Optimum shear walls distribution in Framed structures for buildings subjected to earthquake excitations. International Journal of Engineering Research in Africa , 65 , 55–72. https://doi.org/10.4028/p-ypjdg8
Fares, A. (2019). The effect of shear wall positions on the seismic response of frame-wall structures. World Academy of Science Engineering and Technology Open Science Index 147 International Journal of Civil and Environmental Engineering , 13 (3), 190–194.
FEMA 356. (2000). Prestandard and commentary for the seismic rehabilitation of buildings . Federal Emergency Management Agency.
FEMA 440. (2005). Improvement of nonlinear static seismic analysis procedures . Federal Emergency Management Agency.
Ghobarah, A. (2001). Performance-based design in earthquake engineering: State of development. Engineering Structures , 23 (8), 878–884.
Guettala, S., Abdesselam, I., Chebili, R., & Guettala, S. (2024 a). Assessment of the effects of infill walls’ layout in plan and/or elevation on the seismic performance of 3D reinforced concrete structures. Asian Journal of Civil Engineering , 25 (1), 657–673. https://doi.org/10.1007/s42107-023-00802-2
Guettala, S., Khelaifia, A., Chebili, R., & Guettala, S. (2024 b). Effect of infill walls on seismic performance of multi-story buildings with shear walls. Asian Journal of Civil Engineering , 25 (5), 3989–3999. https://doi.org/10.1007/s42107-024-01025-9
Guettala, S., Khelaifia, A., Abdesselam, I., Chebili, R., & Guettala, S. (2024 c). Assessment of seismic fragility of 3D reinforced concrete structures with masonry infill walls under different distribution arrangements. Asian Journal of Civil Engineering. https://doi.org/10.1007/s42107-024-01126-5
Günel, A. O. (2013). Influence of the shear wall area to floor area ratio on the seismic performance of existing reinforced concrete buildings (Master’s thesis, Middle East Technical University).
Jain, S., & Sathbhaiya, R. (2020). Analysis of Irregular shaped Tall structure considering Shear Wall at different positions using analysis Tool Staad. Pro International Journal of Scientific Research in Civil Engineering , 4 (4), 44–49.
Kaveh, A. (2014). P. Zakian (Ed.), Seismic design optimisation of RC moment frames and dual shear wall-frame structures via CSS algorithm. Asian Journal of Civil Engineering 15 2014 435–465.
Kaveh, A., & Zakian, P. (2012). Performance based optimal seismic design of RC shear walls incorporating soil-structure interaction using CSS algorithm. International Journal of Optimization in Civil Engineering , 2 (3), 383–405.
Kaveh, A., Kalateh-Ahani, M., & Fahimi-Farzam, M. (2013). Constructability optimal design of reinforced concrete retaining walls using a multi-objective genetic algorithm. Structural Engineering and Mechanics , 47 (2), 227–245. https://doi.org/10.12989/sem.2013.47.2.227
Kaveh, A., Izadifard, R. A., & Mottaghi, L. (2019). Optimal design of planar RC frames considering CO2 emissions using ECBO, EVPS and PSO metaheuristic algorithms. Journal of Building Engineering . https://doi.org/10.1016/j.jobe.2019.101014
Kaveh, A., Izadifard, R. A., & Mottaghi, L. (2020). Optimal design of planar RC frames considering CO2 emissions using ECBO, EVPS and PSO metaheuristic algorithms. Journal of Building Engineering , 28 , 101014. https://doi.org/10.1016/j.jobe.2019.101014
Kaveh, A., Mottaghi, L., & Izadifard, R. A. (2021). An integrated method for sustainable performance-based optimal seismic design of RC frames with non-prismatic beams. Scientia Iranica Transactions a: Civil Engineering , 28 (5), 2596–2612. http://scientiairanica.sharif.edu
Khelaifia, A., Chebili, R., & Zine, A. (2024). Impact of the position and quantity of shear walls in buildings on the seismic performance. Asian Journal of Civil Engineering , 25 (1), 953–964. https://doi.org/10.1007/s42107-023-00824-w
Mahmoud, S. (2021). In-plane shear-wall configuration effects on the seismic performance of symmetrical multistory reinforced-concrete buildings. International Journal of Civil Engineering , 19 (10), 1195–1208. https://doi.org/10.1007/s40999-021-00634-8 .
Mander, J. B., Priestley, M. J. N., & Park, R. (1988). Observed stress-strain behavior of confined concrete. Journal of Structural Engineering , 114 (8), 1827–1849.
Ozkul, T. A., Kurtbeyoglu, A., Borekci, M., Zengin, B., & Koçak, A. (2019). Effect of shear wall on seismic performance of RC frame buildings. Engineering Failure Analysis , 100 , 60–75. https://doi.org/10.1016/j.engfailanal.2019.02.032
Reshma, T. V., Sankalpasri, S. S., Tanu, H. M., & Nirmala, M. V. (2021). Multistorey Building Analysis and Its Behavior because of Shear Wall Location Underneath completely different Seismal Zones. IOP Conference Series: Earth and Environmental Science, 822(1), 012044. https://doi.org/10.1088/1755-1315/822/1/012044
Sumit, & Gupta, S. (2019). Performance-based seismic evaluation of multi-storey R.C.C. building with addition of Shear Wall. In lecture notes in civil engineering. Springer Nature . https://doi.org/10.1007/978-981-13-6717-5_6
Zakian, P., & Kaveh, A. (2020). Topology optimization of shear wall structures under seismic loading. Earthquake Engineering and Engineering Vibration , 19 (1), 105–116. https://doi.org/10.1007/s11803-020-0550-5
Zameeruddin, M., & Sangle, K. K. (2021). Performance-based Seismic Assessment of Reinforced concrete moment resisting Frame. Journal of King Saud University: Engineering Sciences , 33 (3), 153–165. https://doi.org/10.1016/j.jksues.2020.04.005
Download references
This research received no specific grant from funding agencies in any sector.
Author information
Authors and affiliations.
Civil Engineering Research Laboratory, Biskra University, 07000, Biskra, Algeria
Akram Khelaifia, Ali Zine, Salah Guettala & Rachid Chebili
You can also search for this author in PubMed Google Scholar
Contributions
CRediT authorship contribution statementAkram Khelaifia: Conceptualization, Data curation, Formal analysis, Investigation and validation, Methodology, Writing - original draft, Writing - review & editing. Ali Zine: Investigation and validation, Methodology.Salah Guettala: Investigation and validation, Supervision, Methodology, Writing - original draft, Writing - review & editing. Rachid Chebili: Project administration, Methodology, Supervision, Writing - review & editing, Validation.
Corresponding author
Correspondence to Salah Guettala .
Ethics declarations
Conflict of interest.
The authors have no competing interests to declare relevant to this article’s content.
Additional information
Publisher’s note.
Springer Nature remains neutral with regard to jurisdictional claims in published maps and institutional affiliations.
Rights and permissions
Springer Nature or its licensor (e.g. a society or other partner) holds exclusive rights to this article under a publishing agreement with the author(s) or other rightsholder(s); author self-archiving of the accepted manuscript version of this article is solely governed by the terms of such publishing agreement and applicable law.
Reprints and permissions
About this article
Khelaifia, A., Zine, A., Guettala, S. et al. Assessment of the position and quantity of shear walls their correlation with building height on the seismic nonlinear performance. Asian J Civ Eng (2024). https://doi.org/10.1007/s42107-024-01154-1
Download citation
Received : 27 July 2024
Accepted : 13 August 2024
Published : 07 September 2024
DOI : https://doi.org/10.1007/s42107-024-01154-1
Share this article
Anyone you share the following link with will be able to read this content:
Sorry, a shareable link is not currently available for this article.
Provided by the Springer Nature SharedIt content-sharing initiative
- Performance level
- Nonlinear dynamic analysis
- Nonlinear static analysis
- Performance-based seismic design
- Find a journal
- Publish with us
- Track your research
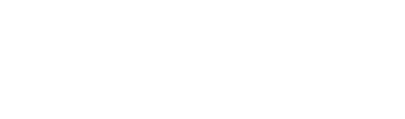
Engineering Reports and Case Studies – Civil Engineering
Engineering studies – h1 civil engineering – the engineering profession.
This topic outlines the purpose and contents of an engineering report. Case studies of Australian innovation and achievement in civil structures are key concepts for this topic.
Key Concepts
- The purposes of a civil engineering report
- Why a report is relevant
- Data collection and representation
- The key components of an engineering report
- The importance of test data and methods of testing
Case Studies
- Material selection based on material properties
- Manufacturing selection based on material properties
- Structural design based on mechanical analysis
- Testing and evaluation processes
- Australian achievement and innovation
Discussion Questions
- What are the purposes of engineering reports?
- Discuss the features of a civil structure report.
- Who writes an engineering report?
- Who is the audience for an engineering report?
- What data is collected for an engineering report?
- How is data collected and displayed?
- Why is test data and the method of testing important?
- Does the structure apply any engineering technologies you recognise?
- What typical engineering structures can be identified in the structure?
- What skills did the team members have who worked on the design of the structure?
- How might the design of the structure change during development?
- What can be stated about how engineering decisions were made, and how are engineering ‘criteria’ used to determine the ‘best’ engineering answer?
- What might have been the biggest engineering challenge in developing the structure?
- How did the Australian drawing standards help in the communication of the design?
- How do you think structures might be developed in the future?
- Engineering design process
- Safety guidelines and Australian Standards
- Materials selected
- Environmental considerations
- Manufacturing techniques
- Technical communication
- Engineering reports
- Discuss the process for design development (teamwork, project management). What role do engineers take at this stage?
- Describe examples of material choice and manufacturing options. Explain specific detail about polymer/metal/composite choices. What criteria are used to make engineering decisions with materials and processes? What are the technical specifications?
- What mechanical analysis was conducted? Was this done using particular testing equipment or modelling? How are the specifications for the product determined regarding strength and other properties?
- What role did engineers take in developing manufacturing specification and the technical drawings/graphics?
- What modelling was done? Was a prototype constructed and how was that made? How was the prototype tested?
- When was the engineer report written?
- What are the future plans?
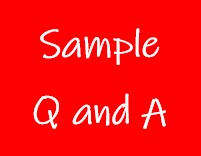
- Subject Engineering Studies
- Module H1 Civil Engineering
- Stage Stage 6
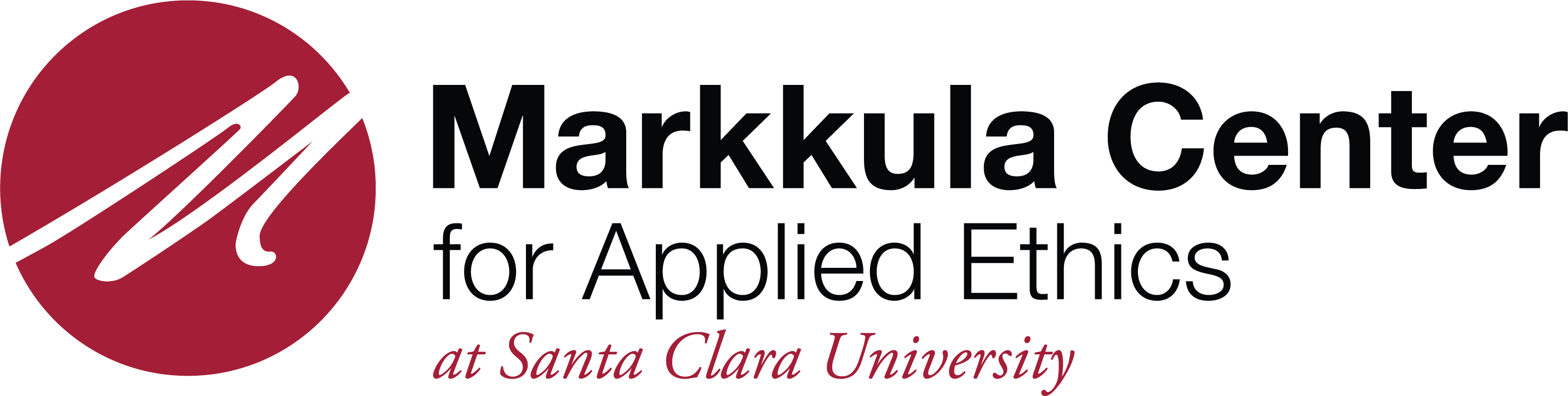
- Engineering Ethics Cases
- Markkula Center for Applied Ethics
- Focus Areas
- More Focus Areas
- Engineering Ethics
The following series of engineering ethics cases were created by interviewing numerous engineers from Silicon Valley and beyond.
The cases have been written, anonymized, and honed to highlight the ethical content from each interview. While these cases are meant for engineering students and professionals for their professional development, nearly all of the cases occur in the context of business, and therefore are also relevant for those seeking business ethics cases.
These cases are suitable as homework and/or for classroom discussion. The goal of this project is to acquaint engineering students and professionals with the variety of ethical experiences of engineering as practiced “in the field.” By becoming familiar with problems faced by other engineers we hope to thereby prepare those reading these cases if they too encounter difficult ethical dilemmas in their work.
Cases range from the mundane to the deadly. While we do not reveal how each particular case turned out, in general they turned out well – the people involved made the right decisions. But this is not to say that all of these right decisions came without personal cost. A few of the engineers did face negative repercussions and a very few even needed to find new employment. However, overall the interviewees were satisfied with how events turned out, even if they faced negative repercussions for their good decisions. They understood that doing the right thing is good in itself, regardless of the personal consequences they may have faced.
The engineering ethics cases can be sorted into the following categories:
- Academic Ethics
- Bioengineering
- Business Ethics
- Civil Engineering
- Computer/Software Engineering
- Electrical Engineering
- International
- Mechanical Engineering
- Science/Research Ethics
A quality assurance engineer must decide whether or not to ship products that might be defective.
An intern at a power electronics startup faces unkind comments from a fellow engineer. She suspects that her colleague is prejudice toward female engineers.
A chemical engineering professor discovers that a colleague has taken credit for his research.
A bioengineering researcher discovers an error in protocol and feels pressured not to report it to her supervisor.
A graduate student suspects her research adviser has earned tenure under false pretenses.
A computer startup company risks violating copyright laws if it reuses a code that is the intellectual property of another company.
A recently promoted manager at an industrial engineering company discovers that factory workers are asked to work more than eight hours a day without getting paid overtime.
Full transparency might prevent a project leader from closing a deal with a valuable client. Should he still clarify the situation to his client?
A manager at a consumer electronics company struggles over whether or not he should disclose confidential information to a valued customer.
A medical researcher is asked to trim data before presenting it to the scientific advisory board.
A technical sales engineer feels pressure to falsify a sales report in order to prevent the delay of her company's IPO.
When a computer filled with personal data gets stolen, a data company must decide how to manage the breach in security.
Employees of a computer hardware company are angered by a manager that demonstrates favoritism.
A project engineer believes his company is providing the wrong form of technology to an in-need community in East Africa.
A computer engineer is asked to divulge private medical data for marketing purposes.
Environmental engineers face pressure to come up with data that favors their employers.
In this ethics case, a woman is displeased with her work role at a computer hardware company.
A systems engineering company employee quits after getting pressured to falsify product testing paperwork.
A manager at a nonprofit mechanical engineering firm questions how responsible her company should be for ongoing maintenance on past projects.
An engineer for an environmental consulting firm must decide whether or not he should encourage his client to go with a more environmentally sustainable construction plan.
A genetic engineer feels a responsibility to educate colleagues on the truth behind stem cell research.
An engineering manager gets pressured to bribe a foreign official in order to secure a business venture in East Africa.
An African-American electronics design lead wonders whether his colleague's contentious behavior is motivated by racism.
A medical company asks blood sample suppliers to sign an ethically questionable consent form.
A quality assurance tester gets pressured to falsify data about a new product from a major cell phone company.
Should a production engineer prioritize a customer's desires over safety?
A female intern at a construction company faces disrespectful treatment because of her gender.
A new hire at an electronics startup struggles to decide between telling the truth and maximizing the company's profit.
A fellow for a global services program faces an ethical dilemma when a colleague asks him to falsify receipts.
A researcher of regenerative medicine meets a man who is eager sign up for potentially dangerous human testing.
A bioengineer's research leads to the discovery that a patient might have prostate cancer.
Two support engineers at a South Bay audio visual electronics startup question the fairness of a supervisor's decision.
An employee overseeing data analysis on a clinical drug trial has concerns about the safety of a client's drug.
The engineering ethics cases in this series were written by Santa Clara University School of Engineering students Clare Bartlett, Nabilah Deen, and Jocelyn Tan, who worked as Hackworth Engineering Ethics Fellows at the Markkula Center for Applied Ethics over the course of the 2014-2015 academic year. In order to write these cases, the fellows interviewed numerous engineers and collected nearly 40 engineering ethics cases from Silicon Valley and beyond. The Hackworth Fellowships are made possible by a generous gift from Joan and the late Michael Hackworth.
- Engineering Business
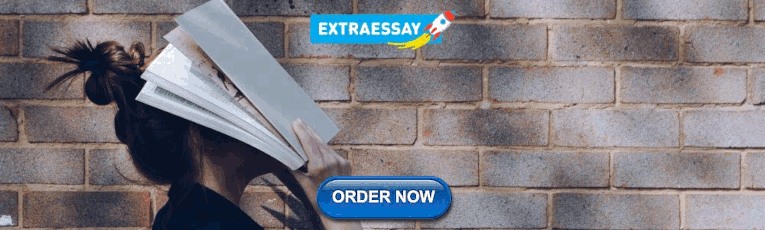
COMMENTS
This book contains essays and case studies that are based upon materials that I prepared for "Project Evaluation", which I designed and taught for more than ten years as one of the required subjects in MIT's Department of Civil & Environmental Engineering. The subject was designed to fill a void in the education of civil engineering students,
The building marks the completion of the first phase of the Department of Engineering's new campus at West Cambridge, also designed by Grimshaw. Due for completion in 2026, the new campus will reintegrate the entire Department of Engineering into a single site, providing 100,000 sq m of research, teaching and collaboration space. Download PDF.
Dealing with "Time is of the Essence". In this (or any other) time of social and/or business disruption, delays, or shutdowns, focus frequently goes to the scheduling clauses contained in design and construction professional service agreements. One…. Continuing Education Whitepapers and Case Studies. Apr 29, 2020.
Case studies, a qualitative research method, are an appealing choice that is suited to this unique reality. But the analytical training of engineers prefers quantitative approaches, even if statistical questions of sample size arise. If carefully designed and executed, case studies can achieve generalizability.
A Case Study on Design and Optimization of Adaptive Civil Structures. Florian Geiger * Jan Gade Malte von Scheven Manfred Bischoff. Institute for Structural Mechanics, University of Stuttgart, Stuttgart, Germany. Taking advantage of adaptivity in the field of civil engineering is a subject of ongoing research.
Find case studies on Operations Management, Sustainability, Entrepreneurship, and others in the MIT Sloan School Teaching Resources Library. Open Case Studies from the University of British Columbia Case studies on a range of topics including Civil Engineering, Conservation, Forestry, and Soil Science
N2 - Failure Case Studies in Civil Engineering: Structures, Foundations, and the Geoenvironment, Second Edition, provides short descriptions of 50 real-world examples of constructed works that did not perform as intended. Designed for classroom use, each case study contains a brief summary, lessons learned, and references to key sources.
The engineering field needs a comprehensive and authoritative resource to provide in-depth, impartial analysis of key engineering failures. Engineering Case Studies Online is a collection that will grow to include 250 hours of video and 50,000 pages of text resources to meet this growing need. Content is displayed alongside targeted learning ...
Compendium of Civil Engineering Education Strategies: Case Studies and Examples serves as a useful guide for engineering faculty, practitioners, and graduate students considering a career in academia. Academic faculty and working professionals will find the content helpful as instructional and reference material in developing and assessing ...
We award professional qualifications that are the civil engineering standard, lead the debates around infrastructure and the built environment and provide training, knowledge and insight.
AbstractThis article seeks to improve the understanding of applying case studies in qualitative research in construction culture. Being a soft and method-complex research strategy, the case study approach is challenging to implement in practice. This ...
Failure Case Studies in Civil Engineering - Structures, Foundations, and the Geoenvironment, 2 nd edition. American Society of Civil Engineers, Reston, VA. Crossref. Google Scholar. Cavalline, T. L. (2016). "Use of Failure Case Studies to Support UNC Charlotte's Prospect for Success Quality Enhancement Plan." Presentation at the 2016 ...
Engineering Case Studies Online will dramatically improve teaching and research by providing a single, comprehensive source for a wide range of video and text material focusing on engineering failures and successes.The collection covers over 300 videos and 70,000 pages of quality documentaries, accident reports, experiments, visualizations, case studies, lectures and interviews from leading ...
KSCE Journal of Civil Engineering - With tidal traffic becoming an important feature of urban transportation, the impact of tidal lanes on traffic flow is a research topic for many scholars. ... A Case Study of Xiongan New Area, China. Transportation Engineering; Published: ... We use a tidal coefficient of 0.7 as an example to analyze the ...
Each case study can be implemented with only an introductory level background in systems analysis. The project is designed to be completed near the end of a semester, taking a student about 2-3 weeks to complete and culminating in both a project report and an oral presentation. If an entire class in EWRS participates in a case study, with each ...
The objective of this paper is to estimate the equivalent permeability of the rock surrounding the tailrace tunnel of the Azad Dam pumped storage power plant, using geostatistical methods. The permeability of the rock mass is a critical factor that influences the estimation of water flow rates. Since the tunnel passes through various geological units with different permeabilities, it is ...
Celebrating 30 years in the civil engineering industry, these civil engineering projects were delivered within South Wales and South West England regions.
Sample work report topics. Mechanical Engineering. Stress analysis of prismatic members in axial, shearing, torsional and flexural deformations. Force analysis of structures and structural components. Hydrostatics. Flow similarity and dimensional analysis as applied to engineering problems in fluid mechanics. Thermodynamics, forensics based.
Civil Engineering Ethics Site. Menu. Home; 1992 NSF Case Report; 1995 NSF Workshop; Collected Case Studies
Abstract. The study of engineering failures can offer students valuable insights into associated technical, ethical, and professional issues. Lessons learned from failures have substantially affected civil engineering practice. For the student, study of these cases can help place design and analysis procedures into historical context and ...
The case studies cover a wide variety of complex system successes and failures, past and present, around the world. In examining these events, these case studies provide insights into how the design, construction, operation, management and governance of complex systems may result in safe or unsafe outcomes. Beyond informing future activities ...
This study addresses a crucial research gap by investigating the optimal position of shear walls, the ideal shear wall-floor area ratio in building design, and their correlation with building height using non-linear analysis (Static and Dynamic). The results, including capacity curves, inter-story drift, and performance levels from both nonlinear static analysis and nonlinear dynamic analysis ...
The key components of an engineering report. The importance of test data and methods of testing. Case Studies. The process of developing a product considering: Material selection based on material properties. Manufacturing selection based on material properties. Structural design based on mechanical analysis.
In this paper a master plan for the use of failure case studies in an undergraduate civil engineering curriculum is discussed. First, for a selected number of courses, several relevant principles are identified. Examples include drawing correct and complete free body diagrams (statics), compression member buckling (mechanics of materials ...
The engineering ethics cases in this series were written by Santa Clara University School of Engineering students Clare Bartlett, Nabilah Deen, and Jocelyn Tan, who worked as Hackworth Engineering Ethics Fellows at the Markkula Center for Applied Ethics over the course of the 2014-2015 academic year. In order to write these cases, the fellows ...