An official website of the United States government
Official websites use .gov A .gov website belongs to an official government organization in the United States.
Secure .gov websites use HTTPS A lock ( Lock Locked padlock icon ) or https:// means you've safely connected to the .gov website. Share sensitive information only on official, secure websites.
- Publications
- Account settings
- Advanced Search
- Journal List
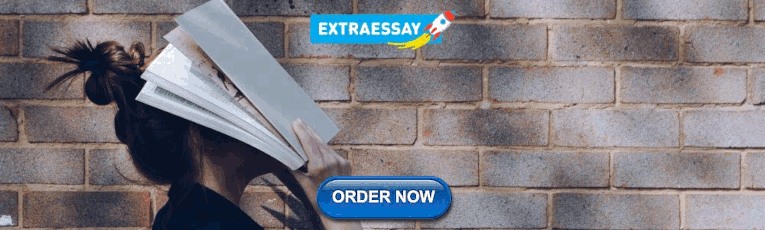
New approaches and procedures for cancer treatment: Current perspectives
Dejene tolossa debela, seke gy muzazu, kidist digamo heraro, maureen tayamika ndalama, betelhiem woldemedhin mesele, dagimawi chilot haile, sophia khalayi kitui, tsegahun manyazewal.
- Author information
- Article notes
- Copyright and License information
Dejene Tolossa Debela, Center for Innovative Drug Development and Therapeutic Trials for Africa (CDT-Africa), College of Health Sciences, Addis Ababa University, P.O. Box 9086, Addis Ababa, Ethiopia. Email: [email protected]
Received 2021 Mar 4; Accepted 2021 Jul 5; Collection date 2021.
This article is distributed under the terms of the Creative Commons Attribution-NonCommercial 4.0 License ( https://creativecommons.org/licenses/by-nc/4.0/ ) which permits non-commercial use, reproduction and distribution of the work without further permission provided the original work is attributed as specified on the SAGE and Open Access page ( https://us.sagepub.com/en-us/nam/open-access-at-sage ).
Cancer is a global health problem responsible for one in six deaths worldwide. Treating cancer has been a highly complex process. Conventional treatment approaches, such as surgery, chemotherapy, and radiotherapy, have been in use, while significant advances are being made in recent times, including stem cell therapy, targeted therapy, ablation therapy, nanoparticles, natural antioxidants, radionics, chemodynamic therapy, sonodynamic therapy, and ferroptosis-based therapy. Current methods in oncology focus on the development of safe and efficient cancer nanomedicines. Stem cell therapy has brought promising efficacy in regenerating and repairing diseased or damaged tissues by targeting both primary and metastatic cancer foci, and nanoparticles brought new diagnostic and therapeutic options. Targeted therapy possessed breakthrough potential inhibiting the growth and spread of specific cancer cells, causing less damage to healthy cells. Ablation therapy has emerged as a minimally invasive procedure that burns or freezes cancers without the need for open surgery. Natural antioxidants demonstrated potential tracking down free radicals and neutralizing their harmful effects thereby treating or preventing cancer. Several new technologies are currently under research in clinical trials, and some of them have already been approved. This review presented an update on recent advances and breakthroughs in cancer therapies.
Keywords: Cancer, treatment, stem cell, targeted drugs, ablation, natural antioxidants, gene therapy
Introduction
Cancer is a global health problem responsible for one in six deaths worldwide. In 2020, there were an estimated 19.3 million new cancer cases and about 10 million cancer deaths globally. Cancer is a very complicated sequence of disease conditions progressing gradually with a generalized loss of growth control. 1 – 3 There were only a few options of cancer treatment for patients for many decades which include surgery, radiation therapy, and chemotherapy as single treatments or in combination. 4 , 5 But recently, many pathways involved in cancer therapy progression and how they can be targeted has improved dramatically, with combinatorial strategies, involving multiple targeted therapies or “traditional” chemotherapeutics, such as the taxanes and platinum compounds, being found to have a synergistic effect. 6 New approaches, such as drugs, biological molecules, and immune-mediated therapies, are being used for treatment even if the excepted therapy level has not reached that resists the mortality rate and decreases the prolonged survival time for metastatic cancer.
The creation of a new revolution in neoplastic cancer or targeting drugs depends on the pathways and characteristics of different tumor entities. 7 Chemotherapy is considered the most effective and widely used modality in treating cancers as used alone or in combination with radiotherapy. Genotoxicity is how chemotherapy drugs target the tumor cells mainly producing reactive oxygen species that largely destroy tumor cells. 8 Hormonal treatments are also widely used for cancer malignancies and considered as cytostatic because it restricts tumor development by limiting the hormonal growth factors acting through the direction of hypothalamic–pituitary–gonadal axis (HPGA), hormone receptor blockage, and limiting of adrenal steroid synthesis. 9
In this narrative review, a general overview of the most advanced and novel cancer therapies was provided. In addition, also new strategies currently under investigation at the research stage that should overwhelm the drawbacks of standard therapies; different strategies to cancer diagnosis and therapy; and their current status in the clinical context, underlining their impact as innovative anti-cancer approaches.
Cancer treatment modalities
We can see cancer treatment modalities by dividing them into conventional (traditional) and advanced or novel or modern categories. In this era worldwide, over half of all ongoing medical treatment trials are focusing on cancer treatments. 7 Entities, such as the type of cancer, its site, and severity, guide to select treatment options and its progress. The most widely used traditional treatment methods are surgery, chemotherapy, and radiotherapy, while modern modalities include hormone therapy, anti-angiogenic, stem cell therapies, immunotherapy, and dendritic cell-based immunotherapy. 10
Conventional cancer therapies
The most recommended conventional cancer treatment strategies include surgical resection of the tumors followed by radiotherapy with x-rays and/or chemotherapy. 11 Of these modalities, surgery is most effective at an early stage of disease progression. Radiation therapy can damage healthy cells, organs, and tissues. Although chemotherapy has reduced morbidity and mortality, virtually all chemotherapeutic agents damage healthy cells, especially rapidly dividing and growing cells. 12 Drug resistance, a major problem with chemotherapy, is a phenomenon wherein cancer cells that initially were suppressed by an anti-cancer drug develop resistance to the drug. This is caused primarily by reduced drug uptake and increased drug efflux. 13 Limitations of conventional chemotherapeutic modality, such as dosage selection difficulty, lack of specificity, rapid drug metabolism, and mainly harmful side effects. 14
Advanced and innovative cancer therapies
Among the obstacles of cancer, drug resistance and its delivery systems are the most problem in cancer cure and decreasing signs and symptoms; but currently, there are many approved treatment approaches and drugs. The efficiency of conventional cancer is reduced due to tumor pathology and architectural abnormality of tumor tissue blood vessels. 15 The following are the advanced and innovative cancer therapy types with their benefits and challenges.
Stem cells therapy
Stem cells are undifferentiated cells present in the bone marrow (BM) with an ability to differentiate into any type of body cell. Stem cell therapeutic strategy is also one of the treatment options for cancer which are considered to be safe and effective. Application of stem cell is yet in the experimental clinical trial; for example, their use in the regeneration of other damaged tissue is being explored. Mesenchymal stem cells (MSCs) are currently being used in trials that are delivered from the BM, fat tissues, and connective tissues. 16
Pluripotent stem cells
Embryonic stem cells (ESCs) isolated from the uniform inner mass cells of the embryo possess the flexibility to administer rise to any or all kinds of cells except those within the placenta. In 2006, the invention of Yamanaka factors to induce pluripotent stem cells (iPSCs) from physical cells in a culture marked a breakthrough in cell biology. 17 Avoiding ethical issues from embryo destruction, iPSCs and ESCs have the same characteristics. Hematopoietic embryonic stem cells (hESCs) and iPSCs are currently used for the induction of effector T cells and natural killer (NK) cells, 18 and anti-tumor vaccine preparation. 19
Adult stem cells
Adult stem cells (ASCs) groups often used in tumor therapy include hematopoietic stem cells (HSCs), MSCs, and neural stem cells (NSCs). HSCs, located in BM, can form all mature blood cells in the body. Currently, only approved by the Food and Drug Administration (FDA) is the infusion of HSCs derived from cord blood to treat multiple myeloma and leukemia. 20 MSCs are found in many tissues and organs, playing important roles in tissue repair and regeneration into cells, such as osteocytes, adipocytes, and chondrocytes. MSCs have special biological characteristics and are used as complimentary with other approaches in treating tumors. 21 NSCs can self-renew and generate new neurons and glial cells and are used for treating both primary and metastatic breast and other tumors. 22
Cancer stem cells
Cancer stem cells (CSCs) are generated in normal stem cells or precursor/progenitor cells by the epigenetic mutations process. Their role in tumor treatment includes cancer growth, metastasis, and recurrence, so that it could give promise in the treatment of solid tumors. 23 Stem cells have several action mechanisms in treating the tumor. The homing process is one mechanism which is a rapid migration of HSCs into defined stem cell niches in BM after that the transplants undergo the engraftment process before giving rise to specialized blood cells. This mechanism is dependent on the active interaction between stem cell CXCR4 receptors and requires their interaction with endothelial cells through LFA-1, VLA-4/5, CD44, and the secretion of matrix degradable enzyme MMP-2/9. 22 The second mechanism is the tumor-tropic effect in which the migration of MSCs toward tumor microenvironment (TM) after attraction by CXCL16, SDF-1, CCL-25, and IL-6 secreted by tumor cells and differentiation of MSCs within the tumor cells which contributes to tumor stromal development. 24 Stem cells also act by paracrine factor secretion, including extracellular vesicles (EVs) and soluble materials, 25 and their differentiation capacity, such as transplanted HSCs, can give rise to all blood cell types. 26
Generally, cancer treatment using stem cell therapy by various strategies, including transplantation of HSC, 27 MSC infusion, 28 therapeutic carriers, 29 generation of immune effector cells, 30 and vaccine production. 31 The stem cell cancer therapy approach confronted the following side effects: (1) tumorigenesis, (2) adverse events in allogeneic HSC transplantation, (3) drug toxicity and drug resistance, (4) increased immune responses and autoimmunity, and (5) viral infection. 22 Despite several successes, there are challenges, such as therapeutic dose control, low cell targeting, and retention in tumor sites, that should be investigated and overcome in the future. In addition, existing results from stem cell technologies are highly encouraging for tumor treatment but it still needs further efforts to improve the safety and efficacy before they could enter clinical trials. Table 1 summarized the licensed list of stem cell therapies.
Licensed stem cell therapies.
AML: acute myelogenous leukemia; FDA: The US Food and Drug Administration; iPSC: induced pluripotent stem cell; MSC-INβ: mesenchymal stem cells with interferon beta.
Targeted drug therapy
Targeted cancer therapies are drugs or other substances which are sometimes interchangeably used as “molecularly targeted drugs,” “molecularly targeted therapies,” and “precision medicines.” Those drugs’ mechanism of action is by interfering with growth molecules which leads to blocking the growth and spreading of cancer. 34 Tumor initiation and progression are determined by the TM of an atypical tumor which comprises endothelial cells, pericytes, smooth muscle cells, fibroblasts, various inflammatory cells, dendritic cells, and CSCs. There are various signaling mechanisms and pathways that TM-forming cells dynamically interact with the cancerous cells which are suitable for sustaining a reasonably high cellular proliferation. So, it is the area of research interest using TM conditions to mediate effective targeting measures for cancer therapy. 35
Selectively treating cancer cells with conventional chemotherapy is difficult since it is similar to normal cells. So those problems are intervened by cellular mechanisms, such as cell cycle arrest, apoptosis induction, proliferation prevention, and interfering with metabolic reprogramming by targeted drug therapy agents. 36 Modifying TM and targeting TM for drug delivery for effective treatment are two strategies that can be used for the treatment of cancer. 37 Targeted therapy drugs do work in different ways from standard chemotherapy drugs treatment like attacking cancer cells while doing less damage to normal cells which is a programming that sets them apart from normal, healthy cells. 38
Using targeted therapy markedly increased the survival rate for some diseases, for example, from 17% to 24% in patients with advanced pancreatic cancer, the addition of erlotinib to standard chemotherapy. Imatinib has had a dramatic effect on chronic myeloid leukemia, and rituximab, sunitinib, and trastuzumab have revolutionized the treatment of renal cell carcinoma and breast cancer, respectively. 39
We can classify the targeted cell agents based on the mechanism of their work or their target site. Some enzymes serve as signals for cancer cells to grow. Some targeted therapies inhibit enzymes that are signals for cancer cells to grow. These drugs are called enzyme inhibitors. Blocking these cell signals can inhibit cancer from getting bigger and spreading. 40
Some targeted therapies are called apoptosis-inducing drugs because they are aimed right at the parts of the cell that control whether cells live or die. The examples are serine/threonine kinase, protein kinase B (PKB/Akt), which promotes cell survival, and inhibitors of this protein are in the preclinical phase. 41
These agents stop the tumors from making new blood vessels which helps cut off the tumors’ blood supply so that tumors cannot grow. In addition, they arrest tumor growth that involves by curtailing blood supply to the tumor by inhibiting angiogenic factors, such as vascular endothelial growth factor (VEGF) or its receptors. The study showed the survival of patients with advanced colorectal carcinoma extended by months after the use of Avastin (bevacizumab) in combination with 5-fluorouracil-based chemotherapy. 42
Types of target agents
Monoclonal antibodies.
Antibody drugs are man-made versions of immune system proteins administered intravenously to attack certain targets on cancer cells. They contain a more proportion of human components than murine components. 43 Their attack mechanisms of action are recruiting host immune functions to attack the target cell, binding to ligands or receptors thereby interrupting essential cancer cell processes, and carrying a lethal payload, such as radioisotope or toxin, to the target cell. 44 Gemtuzumab is an example of a CD-33-specific monoclonal antibody currently used for AML treatment by conjugating with calicheamicin. 45 In addition, ibritumomab tiuxetan is an anti-CD20, a 90Y metal isotope-based is developed in clinical therapy. 46 Delivery of active therapeutics, prodrug activation enzymes, and chemotherapy toxins are also another use of target agents of monoclonal antibodies. 47
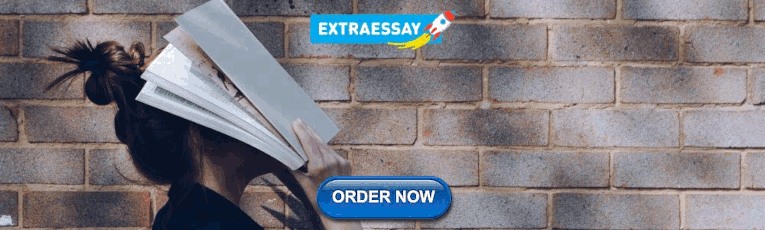
Small molecule inhibitors
These are smaller protein in size (⩽500 Da) than monoclonal antibodies, so that they can simply translocate through plasma membranes and can be taken orally. Their main function is interrupting cellular processes by interfering with the intracellular signaling of tyrosine kinases which leads to the inhibition of tyrosine kinase signaling and initiates a molecular cascade that can lead to the inhibition of cell growth, proliferation, migration, and angiogenesis in malignant tissues. 48 Examples of small molecule inhibitors are gefitinib and erlotinib which inhibit epidermal growth factor receptor (EGFR) kinase and EGFR in non-small cell lung cancer (NSCLC) patients, respectively. There are also lapatinib and sorafenib which act on the inhibition of EGFR/Erb-B2 Receptor Tyrosine Kinase 2 (ERBB2) for ERBB2-positive breast cancer and VEGFR kinase, in renal cancer. 49
Ablation cancer therapy
Ablation is a treatment technique that destroys tumors without removing them mostly indicated for small-size tumors of less than 3 cm and the surgical option is contraindicated. Ablation is also used with embolization for larger tumors. However, this technique might not be indicated for treating tumors near major blood vessels, the diaphragm, or major bile ducts due to destroying some of the normal tissue around the tumor. 50
Thermal ablation
This technique uses extreme hyperthermia or hypothermia to destruct tumor tissue concentrating on a focal zone in and around the tumor. Similar to surgery, thermal ablation removes the tumor and a 5–10 mm thick margin of seemingly normal tissue but the tissue is killed in situ and then absorbed by the body later. The procedure is similar to surgery using an open, laparoscopic, or endoscopic approach but is commonly applied using a percutaneous or non-invasive approach. The type of tumor, site, physician’s choice, and health status determine the approach. 51
Radiofrequency ablation (RFA), microwave ablation, high-intensity focused ultrasound, and cryoablation are currently being used in the clinical setting. Cryoablation uses a hypothermic modality to induce tissue damage by a freeze-thaw process against others. All these treatments operate on the principle of hyperthermia except cryoablation. Of all the ablation techniques, cryoablation demonstrated the highest potential to elicit a post-ablative immunogenic response. 52
Recent studies showed additional to tissue disruption RFA and cryoablation can modulate the immune system that they were applied as therapy on TM and in the systematic circulation. Evidence has shown that ablation procedures affect carcinogenesis due to its local inflammatory response leading to an immunogenic gene signature. 53
The advantage of this procedure over surgery is that it provides a minimal (e.g. percutaneously or laparoscopically) or non-invasive approach to cancer therapy and gets attention as an alternative to standard surgical therapies. 54
Cryoablation
Cryoablation is one of the ablation techniques which ablates the extensive tissue by freezing to lethal temperatures followed by liquid formation, causing extensive tissue. Benign and malignant primary tumors are mostly treated by this therapy. 55 James Arnott reported that the freezing temperatures can impair cancer cell viability after he attempted the usage of cold temperatures by salt and ice solutions for the generation of local numbness before surgical operations in the nineteenth century. He suggested cryoablation as an attractive therapeutic option and increased a patient’s survival. 56
Cryoablation techniques are based on the principle of the Joule–Thomson effect which was studied in the 1930s by many researchers and concluded using liquid CO 2 under high pressure, liquid air, and liquid oxygen to achieve the cooling effect and the subsequent formation of ice crystals so employed to treat lesions, warts, and keratosis. However, after 1950, Allington replaced liquid N 2 for the treatment of various skin lesion disorders. 47
RFA therapy
RFA is a minimally invasive procedure and an image-guided technique using hyperthermic (high-frequency electrical currents) conditions to destroy cancer cells. Imaging techniques, such as ultrasound, computed tomography (CT), or magnetic resonance imaging (MRI), guide needle electrodes into a tumor cell. Generally, RFA is the most effective approach for treating small-size tumors of less than 3 cm in diameter. RFA can be used in combination with other conventional cancer treatment options. 57 After starting the use of deployable devices or multiple-electrode systems, RFA can treat medium tumors (up to 5 cm diameter). 58
Gene therapy
Gene therapy is the insertion of a normal copy of a defective gene in the genome to cure a specific disorder. The first application dates back to 1990 when a retroviral vector was exploited to deliver the adenosine deaminase (ADA) gene to T cells in patients with severe combined immunodeficiency (SCID). Approximately, about 2900 gene therapy clinical trials are currently ongoing, two-third of which are related to cancer. Strategies, such as expression of proapoptotic and chemosensitizing genes, expression of wild-type tumor suppressor genes, expression of genes able to solicit specific anti-tumor immune responses, and targeted silencing of oncogenes, are under evaluation for cancer gene therapy. 47
Thymidine kinase (TK) gene delivery is effective for the administration of prodrug ganciclovir to activate its expression and induce specific cytotoxicity. 59 The p53 tumor suppressor gene which is vectors carrying has been assessed for the clinical purpose very recently. ONYX-015 has been tested in NSCLC patients and gave a high response rate when given alone or combined with chemotherapy. 60 Gendicine, a recombinant adenovirus carrying wild-type p53-induced complete disease regression in head and neck squamous cell cancer had similar success when combined with radiotherapy. 61
Some challenges that have been faced with gene therapy are the selection of the right conditions and the choice of the best delivery mechanism. Identified drawbacks of this therapy are genome integration, limited efficacy in specific subsets of patients, and high chances of being neutralized by the immune system. Basic research and medical translation used RNA interference (RNAi) as an efficient technology that able to produce targeted gene silencing. 62 RNA-induced silencing complex (RISC) mediates the targeted gene silencing process by cleaving the messenger RNA (mRNA) and interference with protein synthesis. 63 A siRNAs can be designed to block desired targets, involving cell proliferation and metastatic invasion; hence, precise molecular mechanisms are a triggering factor for tumor formation. This method relies on siRNA-mediated gene silencing of anti-apoptotic proteins, transcription factors (i.e. c-myc gene), 64 , 65 or cancer mutated genes (i.e. K-RAS). 66
Advantages of siRNA-based drugs are safety, high efficacy, specificity, few side effects, and low costs of production. 67 However, occasionally, they can induce off-target effects or elicit innate immune responses, followed by specific inflammation. 68 Delivery methods currently under study are chemical modification (insertion of a phosphorothioate at 3’ end, introduction of a 2’ O-methyl group, and modification by 2,4-dinitrophenol) and lipid encapsulation, or conjugation with organic molecules (polymers, peptides, lipids, antibodies, small molecules) efficiently target to spontaneously cross cell membranes of naked siRNAs. 69 Interaction of cationic liposomes with negatively charged nucleic acids facilitates easy transfection by simple electrostatic interactions. 70 They can be constituted by 1,2-dioleoyl-3-trimethylammonium propane (DOTAP) and N-[1-(2,3-dioleoyloxy) propyl]-N, Ntrimethylammonium methyl sulfate (DOTMA). 71 Currently, a Phase I clinical trial is recruiting patients for evaluating the safety of Eph receptor A2 (EphA2) targeting 1,2-dioleoyl-sn-glycero-3-phosphocholine (DOPC) encapsulated siRNA (siRNA-EphA2- DOPC) in patients with advanced and recurrent cancer. 72 siRNAs can be concentrated in cationic polymers, such as chitosan, cyclodextrin, and polyethyleneimine (PEI). 73 CALAA-01 is one of the cyclodextrin polymers conjugated with human transferrin is being entered a Phase I clinical trial. PEI has been used as an anti-cancer by forming small cationic nanoparticles and loading with human epidermal growth factor receptor 2 (HER-2 receptor)-specific siRNA. 74 Phase II clinical trial has been started to evaluate Local Drug EluteR (siG12D LODER) directed to mutated Kirsten rat sarcoma (K-RAS) oncogene for the treatment of advanced pancreatic cancer. Conjugating to peptides, antibodies, and aptamers improves stability during circulation and enhances cellular uptake of siRNAs. 75 The introduction of nanocarriers has largely improved siRNAs stability, pharmacokinetics and biodistribution properties, and targeting specificity. Polyallylamine phosphate nanocarriers have been developed to release siRNAs in the cytoplasm after disassembly at low endosomal pH. 76
Dose correction and variabilities between individuals and different stages of disease are challenging issues on clinical translation of the siRNA-based approach. In the future, the needed research is on setting up the best-personalized therapy and toward controlled release to reach only specific targets on treating the tumor. Table 2 summarizes the gene therapy drugs based on their mechanism of action and induction.
Summary of gene therapy approaches.
Natural antioxidants
Day to day, the anatomy undergoes many exogenous insults, such as ultraviolet (UV) rays, pollution, and tobacco smoke, that end in the assembly of reactive species, particularly oxidants and free radicals, liable for the onset of many diseases, together with cancer. These molecules can even be made as a consequence of clinical administration of medication; however, they are additionally naturally created within our cells and tissues by mitochondria and peroxisomes, and from macrophages metabolism, throughout traditional physiological aerobic processes. 47
Oxidative stress and radical oxygen species can significantly change the regulation of transcription factors by damaging the DNA and other bio-macromolecule. 77
Vitamins, polyphenols, and plant-derived bioactive compounds are natural antioxidants used as preventive and therapeutic drugs against these molecules that damage the body due to their anti-inflammatory and antioxidant properties. 78 Studies added to cancer therapy after appreciating their anti-proliferative and proapoptotic properties. Compounds, such as vitamins, alkaloids, flavonoids, carotenoids, curcumin, berberine, quercetin, and others, are examples of natural antioxidants screened in vitro and in vivo. 79
Limited bioavailability and/ or toxicity is one of the challenges of natural drugs while their translation into clinical practice. 47 Curcumin has cytotoxic effects in different kinds of tumors, such as the brain, lung, leukemia, pancreatic, and hepatocellular carcinoma, 80 while sparing normal cells at effective therapeutic doses. The curcumin’s biological properties, treatment duration and efficient therapeutic doses are under study. 80 This day, about 27 clinical trials are done, while 40 are under study on curcumin.
Berberine is an alkaloid compound that has been studied to be effective against different cancers as a chemopreventive agent, modulating many signaling pathways. Different nanotechnological strategies have been developed to facilitate its delivery across cell membranes due to their poorly soluble in water. 81 Six clinical trials are under study and two have been completed.
Quercetin is another natural plant origin agent that is effective alone and also in combination with chemotherapeutic agents in treating many cancers, such as lung, prostate, liver, colon, and breast cancers. 82 Quercetin’s mechanism of action is by binding to cellular receptors and the interference of several signaling pathways. 83 Currently, six clinical trials are under study and seven studies have been completed.
Current clinical trials
In recent years, analysis of cancer medication has taken outstanding steps toward more practical, precise, and fewer invasive cancer treatments in the research of clinical trials ( Figure 1 ).
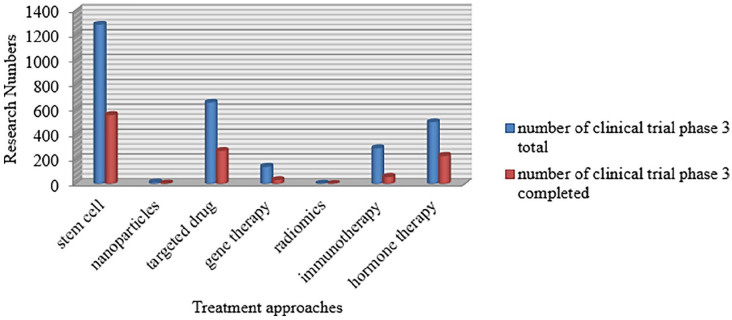
Current status of clinical trials of innovative and novel strategies of cancer therapy.
Currently, the most frequent entries focusing on cancer therapies in the database of clinical trials ( www.clinicalTrials.gov ) include the terminologies stem cell, targeted therapy, immunotherapy, and gene therapy because they are very promising and effective. Table 3 summarizes the potential advantages and disadvantages of the new treatment approaches.
Comparison of advantageous and disadvantageous of new cancer therapies.
MRI: magnetic resonance imaging.
Table 4 summarizes the approaches to advanced cancer therapies and their respective delivery systems with examples.
Advanced therapy approaches and delivery systems.
Current methods in oncology focus on the development of safe and efficient cancer nanomedicines. Targeted medical care helped rising the biodistribution of recent or already tested chemotherapeutical agents around the specific tissue to be treated; different methods, such as sequence medical care, siRNAs delivery, therapy, and inhibitor molecules, supply new potentialities to cancer patients. Gene therapy acts by direct in situ insertion of exogenous genes into benign tumors. Noticeably, stem cells can be used as regenerative medicine, therapeutic carriers, drug targeting, and generation of immune cells because of having unique biological actions on other cells. 22 On the opposite hand, thermal ablation and magnetic hyperthermia are promising alternatives to the growth surgical process. Finally, radionics and pathomics approaches facilitate the management of huge knowledge sets from cancer patients to enhance prognosis and outcomes. Much progress has been made, but many others are likely to come soon, producing more and more ad hoc personalized therapies. Further development and refinement of drug delivery systems are essential for improving therapeutic outcomes.
Acknowledgments
The authors thank the Center for Innovative Drug Development and Therapeutic Trials for Africa (CDT-Africa), Addis Ababa University, for the support rendered.
Author contributions: D.T.D. is a major contributor in writing the manuscript. All others reviewed and approved the manuscript.
Declaration of conflicting interests: The author(s) declared no potential conflicts of interest with respect to the research, authorship, and/or publication of this article.
Funding: The author(s) received no financial support for the research, authorship, and/or publication of this article.

- 1. Sung H, Ferlay J, Siegel RL, et al. Global cancer statistics 2020: GLOBOCAN estimates of incidence and mortality worldwide for 36 cancers in 185 countries. CA Cancer J Clin 2021; 71: 209–249. [ DOI ] [ PubMed ] [ Google Scholar ]
- 2. Ganesh K, Massagué J. Targeting metastatic cancer. Nat Med 2021; 27: 34–44. [ DOI ] [ PMC free article ] [ PubMed ] [ Google Scholar ]
- 3. Merriel SWD, Ingle SM, May MT, et al. Retrospective cohort study evaluating clinical, biochemical and pharmacological prognostic factors for prostate cancer progression using primary care data. BMJ Open 2021; 11: e044420. [ DOI ] [ PMC free article ] [ PubMed ] [ Google Scholar ]
- 4. GlobalSurg Collaborative and National Institute for Health Research Global Health Research Unit on Global Surgery. Global variation in postoperative mortality and complications after cancer surgery: a multicentre, prospective cohort study in 82 countries. Lancet 2021; 397: 387–397. [ DOI ] [ PMC free article ] [ PubMed ] [ Google Scholar ]
- 5. Roy A, Li SD. Modifying the tumor microenvironment using nanoparticle therapeutics. Wiley Interdiscip Rev Nanomed Nanobiotechnol 2016; 8(6): 891–908. [ DOI ] [ PMC free article ] [ PubMed ] [ Google Scholar ]
- 6. Bayat Mokhtari R, Homayouni TS, Baluch N, et al. Combination therapy in combating cancer. Oncotarget 2017; 8: 38022–38043. [ DOI ] [ PMC free article ] [ PubMed ] [ Google Scholar ]
- 7. Abbas Z, Rehman S. An overview of cancer treatment modalities. Neoplasm 2018; 1: 139–157. [ Google Scholar ]
- 8. El-Hussein A, Manoto SL, Ombinda-Lemboumba S, et al. A review of chemotherapy and photodynamic therapy for lung cancer treatment. Anticancer Agents Med Chem 2021; 21(2): 149–161. [ DOI ] [ PubMed ] [ Google Scholar ]
- 9. Ghanghoria R, Kesharwani P, Tekade RK, et al. Targeting luteinizing hormone-releasing hormone: a potential therapeutics to treat gynecological and other cancers. J Control Release 2018; 269: 277–301. [ DOI ] [ PubMed ] [ Google Scholar ]
- 10. Charmsaz S, Collins DM, Perry AS, et al. Novel strategies for cancer treatment: highlights from the 55th IACR annual conference. Cancers 2019; 11: 1125. [ DOI ] [ PMC free article ] [ PubMed ] [ Google Scholar ]
- 11. Arruebo M, Vilaboa N, Sáez-Gutierrez B, et al. Assessment of the evolution of cancer treatment therapies. Cancers 2011; 3: 3279–3330. [ DOI ] [ PMC free article ] [ PubMed ] [ Google Scholar ]
- 12. Moses MA, Brem H, Langer R. Advancing the field of drug delivery: taking aim at cancer. Cancer Cell 2003; 4(5): 337–341. [ DOI ] [ PubMed ] [ Google Scholar ]
- 13. Shapira A, Livney YD, Broxterman HJ, et al. Nanomedicine for targeted cancer therapy: towards the overcoming of drug resistance. Drug Resist Updat 2011; 14(3): 150–163. [ DOI ] [ PubMed ] [ Google Scholar ]
- 14. Mondal J, Panigrahi AK, Khuda-Bukhsh AR. Conventional chemotherapy: problems and scope for combined therapies with certain herbal products and dietary supplements. Austin J Mol Cell Biol 2014; 1: 10. [ Google Scholar ]
- 15. El-Readi MZ, Althubiti MA. Cancer nanomedicine: a new era of successful targeted therapy. J Nanomater 2019; 2019: 4927312. [ Google Scholar ]
- 16. Naji A, Eitoku M, Favier B, et al. Biological functions of mesenchymal stem cells and clinical implications. Cell Mol Life Sci 2019; 76(17): 3323–3348. [ DOI ] [ PMC free article ] [ PubMed ] [ Google Scholar ]
- 17. Takahashi K, Yamanaka S. Induction of pluripotent stem cells from mouse embryonic and adult fibroblast cultures by defined factors. Cell 2006; 126: 663–676. [ DOI ] [ PubMed ] [ Google Scholar ]
- 18. Li Y, Hermanson DL, Moriarity BS, et al. Human iPSC-derived natural killer cells engineered with chimeric antigen receptors enhance anti-tumor activity. Cell Stem Cell 2018; 23: 181.e5–192.e5. [ DOI ] [ PMC free article ] [ PubMed ] [ Google Scholar ]
- 19. Ouyang X, Telli ML, Wu JC. Induced pluripotent stem cell-based cancer vaccines. Front Immunol 2019; 10: 1510. [ DOI ] [ PMC free article ] [ PubMed ] [ Google Scholar ]
- 20. Trujillo-Alonso V, Pratt EC, Zong H, et al. FDA-approved ferumoxytol displays anti-leukaemia efficacy against cells with low ferroportin levels. Nat Nanotechnol 2019; 14(6): 616–622. [ DOI ] [ PMC free article ] [ PubMed ] [ Google Scholar ]
- 21. Lin W, Huang L, Li Y, et al. Mesenchymal stem cells and cancer: clinical challenges and opportunities. Biomed Res Int 2019; 2019: 2820853. [ DOI ] [ PMC free article ] [ PubMed ] [ Google Scholar ]
- 22. Chu DT, Nguyen TT, Tien NLB, et al. Recent progress of stem cell therapy in cancer treatment: molecular mechanisms and potential applications. Cells 2020; 9: 563. [ DOI ] [ PMC free article ] [ PubMed ] [ Google Scholar ]
- 23. Chang JC. Cancer stem cells: role in tumor growth, recurrence, metastasis, and treatment resistance. Medicine 2016; 95(1 Suppl. 1): S20–S25. [ DOI ] [ PMC free article ] [ PubMed ] [ Google Scholar ]
- 24. Sullivan CB, Porter RM, Evans CH, et al. TNFα and IL-1β influence the differentiation and migration of murine MSCs independently of the NF-κB pathway. Stem Cell Res Ther 2014; 5: 104. [ DOI ] [ PMC free article ] [ PubMed ] [ Google Scholar ]
- 25. Vakhshiteh F, Atyabi F, Ostad SN. Mesenchymal stem cell exosomes: a two-edged sword in cancer therapy. Int J Nanomedicine 2019; 14: 2847–2859. [ DOI ] [ PMC free article ] [ PubMed ] [ Google Scholar ]
- 26. Reddy RL. Mobilization and collection of peripheral blood progenitor cells for transplantation. Transfus Apher Sci 2005; 32: 63–72. [ DOI ] [ PubMed ] [ Google Scholar ]
- 27. Copelan EA. Hematopoietic stem-cell transplantation. N Engl J Med 2006; 354: 1813–1826. [ DOI ] [ PubMed ] [ Google Scholar ]
- 28. Casper J, Wolff D, Knauf W, et al. Allogeneic hematopoietic stem-cell transplantation in patients with hematologic malignancies after dose-escalated treosulfan/fludarabine conditioning. J Clin Oncol 2010; 28: 3344–3351. [ DOI ] [ PubMed ] [ Google Scholar ]
- 29. Sage EK, Thakrar RM, Janes SM. Genetically modified mesenchymal stromal cells in cancer therapy. Cytotherapy 2016; 18(11): 1435–1445. [ DOI ] [ PMC free article ] [ PubMed ] [ Google Scholar ]
- 30. Dai H, Wang Y, Lu X, et al. Chimeric antigen receptors modified T-cells for cancer therapy. J Natl Cancer Inst 2016; 108(7): djv439. [ DOI ] [ PMC free article ] [ PubMed ] [ Google Scholar ]
- 31. Batlle E, Clevers H. Cancer stem cells revisited. Nat Med 2017; 23: 1124–1134. [ DOI ] [ PubMed ] [ Google Scholar ]
- 32. Khalil C, Moussa M, Azar A, et al. Anti-proliferative effects of mesenchymal stem cells (MSCs) derived from multiple sources on ovarian cancer cell lines: an in-vitro experimental study. J Ovarian Res 2019; 12: 70. [ DOI ] [ PMC free article ] [ PubMed ] [ Google Scholar ]
- 33. Konopleva M, Pollyea DA, Potluri J, et al. Efficacy and biological correlates of response in a phase II study of Venetoclax monotherapy in patients with acute myelogenous leukemia. Cancer Discov 2016; 6(10): 1106–1117. [ DOI ] [ PMC free article ] [ PubMed ] [ Google Scholar ]
- 34. National Cancer Institute. Targeted cancer therapies. Bethesda, MD: National Cancer Institute, 2020. [ Google Scholar ]
- 35. Adjei IM, Blanka S. Modulation of the tumor microenvironment for cancer treatment: a biomaterials approach. J Funct Biomater 2015; 6: 81–103. [ DOI ] [ PMC free article ] [ PubMed ] [ Google Scholar ]
- 36. Valter K, Chen L, Kruspig B, et al. Contrasting effects of glutamine deprivation on apoptosis induced by conventionally used anticancer drugs. Biochim Biophys Acta Mol Cell Res 2017; 1864(3): 498–506. [ DOI ] [ PubMed ] [ Google Scholar ]
- 37. Bailey KM, Wojtkowiak JW, Hashim AI, et al. Chapter four: targeting the metabolic microenvironment of tumors. In: Smalley KSM. (ed.) Advances in pharmacology. Cambridge, MA: Academic Press, 2012, pp. 63–107. [ DOI ] [ PMC free article ] [ PubMed ] [ Google Scholar ]
- 38. Yadav P. Targeted cancer therapy; researchers reflection. Int Peer-Rev J 2017; 1: 11–17. [ Google Scholar ]
- 39. Weber WA. Positron emission tomography as an imaging biomarker. J Clin Oncol 2006; 24: 3282–3292. [ DOI ] [ PubMed ] [ Google Scholar ]
- 40. Citri A, Yarden Y. EGF-ERBB signalling: towards the systems level. Nat Rev Mol Cell Biol 2006; 7(7): 505–516. [ DOI ] [ PubMed ] [ Google Scholar ]
- 41. Luo J, Manning BD, Cantley LC. Targeting the PI3K-Akt pathway in human cancer: rationale and promise. Cancer Cell 2003; 4(4): 257–262. [ DOI ] [ PubMed ] [ Google Scholar ]
- 42. Hurwitz H, Fehrenbacher L, Novotny W, et al. Bevacizumab plus irinotecan, fluorouracil, and leucovorin for metastatic colorectal cancer. N Engl J Med 2004; 350: 2335–2342. [ DOI ] [ PubMed ] [ Google Scholar ]
- 43. Carter P. Improving the efficacy of antibody-based cancer therapies. Nat Rev Cancer 2001; 1(2): 118–129. [ DOI ] [ PubMed ] [ Google Scholar ]
- 44. Adams GP, Weiner LM. Monoclonal antibody therapy of cancer. Nat Biotechnol 2005; 23: 1147–1157. [ DOI ] [ PubMed ] [ Google Scholar ]
- 45. Sorokin P. Mylotarg approved for patients with CD33+ acute myeloid leukemia. Clin J Oncol Nurs 2000; 4(6): 279–280. [ PubMed ] [ Google Scholar ]
- 46. Jacobs SA. Yttrium ibritumomab tiuxetan in the treatment of non-Hodgkin’s lymphoma: current status and future prospects. Biologics 2007; 1(3): 215–227. [ PMC free article ] [ PubMed ] [ Google Scholar ]
- 47. Pucci C, Martinelli C, Ciofani G. Innovative approaches for cancer treatment: current perspectives and new challenges. ecancer 2019; 13: 961. [ DOI ] [ PMC free article ] [ PubMed ] [ Google Scholar ]
- 48. Tanner JE. Designing antibodies for oncology. Cancer Metastasis Rev 2005; 24(4): 585–598. [ DOI ] [ PubMed ] [ Google Scholar ]
- 49. Yap TA, Workman P. Exploiting the cancer genome: strategies for the discovery and clinical development of targeted molecular therapeutics. Annu Rev Pharmacol Toxicol 2012; 52: 549–573. [ DOI ] [ PubMed ] [ Google Scholar ]
- 50. American Cancer Society. Ablation for liver cancer. Atlanta, GA: American Cancer Society, 2019. [ Google Scholar ]
- 51. Singh S, Melnik R. Thermal ablation of biological tissues in disease treatment: a review of computational models and future directions. Electromagn Biol Med 2020; 39: 49–88. [ DOI ] [ PubMed ] [ Google Scholar ]
- 52. Yakkala C, Chiang CL, Kandalaft L, et al. Cryoablation and immunotherapy: an enthralling synergy to confront the tumors. Front Immunol 2019; 10: 2283. [ DOI ] [ PMC free article ] [ PubMed ] [ Google Scholar ]
- 53. Liu Y, Cao CS, Yu Y, et al. Thermal ablation in cancer (review). Oncol Lett 2016; 12: 2293–2295. [ DOI ] [ PMC free article ] [ PubMed ] [ Google Scholar ]
- 54. Cabuy E. Thermal ablation in cancer treatment. Reliab Cancer Ther 2012; 2: 1–68. [ Google Scholar ]
- 55. Gage AA, Baust J. Mechanisms of tissue injury in cryosurgery. Cryobiology 1998; 37(3): 171–186. [ DOI ] [ PubMed ] [ Google Scholar ]
- 56. Korpan NN. A history of cryosurgery: its development and future. J Am Coll Surg 2007; 204(2): 314–324. [ DOI ] [ PubMed ] [ Google Scholar ]
- 57. Radiological Society of North America (RSNA) and American College of Radiology (ACR). Radiofrequency ablation (RFA) / microwave ablation (MWA) of liver tumors (internet). Oak Brook, IL: Radiological Society of North America; Reston, VA: American College of Radiology, 2020. [ Google Scholar ]
- 58. Brace C. Thermal tumor ablation in clinical use. IEEE Pulse 2011; 2(5): 28–38. [ DOI ] [ PMC free article ] [ PubMed ] [ Google Scholar ]
- 59. Freeman SM, Abboud CN, Whartenby KA, et al. The “bystander effect”: tumor regression when a fraction of the tumor mass is genetically modified. Cancer Res 1993; 53: 5274–5283. [ PubMed ] [ Google Scholar ]
- 60. Ahrendt SA, Hu Y, Buta M, et al. p53 mutations and survival in stage I non-small-cell lung cancer: results of a prospective study. J Natl Cancer Inst 2003; 95: 961–970. [ DOI ] [ PubMed ] [ Google Scholar ]
- 61. Räty JK, Pikkarainen JT, Wirth T, et al. Gene therapy: the first approved gene-based medicines, molecular mechanisms and clinical indications. Curr Mol Pharmacol 2008; 1(1): 13–23. [ DOI ] [ PubMed ] [ Google Scholar ]
- 62. Elbashir SM, Harborth J, Lendeckel W, et al. Duplexes of 21-nucleotide RNAs mediate RNA interference in cultured mammalian cells. Nature 2001; 411: 494–498. [ DOI ] [ PubMed ] [ Google Scholar ]
- 63. Weiss B, Davidkova G, Zhou LW. Antisense RNA gene therapy for studying and modulating biological processes. Cell Mol Life Sci 1999; 55(3): 334–358. [ DOI ] [ PMC free article ] [ PubMed ] [ Google Scholar ]
- 64. Putney SD, Brown J, Cucco C, et al. Enhanced anti-tumor effects with microencapsulated c-myc antisense oligonucleotide. Antisense Nucleic Acid Drug Dev 1999; 9(5): 451–458. [ DOI ] [ PubMed ] [ Google Scholar ]
- 65. Vita M, Henriksson M. The Myc oncoprotein as a therapeutic target for human cancer. Semin Cancer Biol 2006; 16(4): 318–330. [ DOI ] [ PubMed ] [ Google Scholar ]
- 66. Fleming JB, Shen GL, Holloway SE, et al. Molecular consequences of silencing mutant K-ras in pancreatic cancer cells: justification for K-ras-directed therapy. Mol Cancer Res 2005; 3(7): 413–423. [ DOI ] [ PubMed ] [ Google Scholar ]
- 67. Subhan MA, Torchilin VP. siRNA based drug design, quality, delivery and clinical translation. Nanomedicine 2020; 29: 102239. [ DOI ] [ PubMed ] [ Google Scholar ]
- 68. Meng Z, Lu M. RNA interference-induced innate immunity, off-target effect, or immune adjuvant? Front Immunol 2017; 8: 331. [ DOI ] [ PMC free article ] [ PubMed ] [ Google Scholar ]
- 69. Xu CF, Wang J. Delivery systems for siRNA drug development in cancer therapy. Asian J Pharm Sci 2015; 10: 1–12. [ Google Scholar ]
- 70. Sarisozen C, Salzano G, Torchilin VP. Recent advances in siRNA delivery. Biomol Concepts 2015; 6: 321–341. [ DOI ] [ PubMed ] [ Google Scholar ]
- 71. Kim HS, Song IH, Kim JC, et al. In vitro and in vivo gene-transferring characteristics of novel cationic lipids, DMKD (O, O’-dimyristyl-N-lysyl aspartate) and DMKE (O,O’-dimyristyl-N-lysyl glutamate). J Control Release 2006; 115: 234–241. [ DOI ] [ PubMed ] [ Google Scholar ]
- 72. Wagner MJ, Mitra R, McArthur MJ, et al. Preclinical mammalian safety studies of EPHARNA (DOPC nanoliposomal EphA2-targeted siRNA). Mol Cancer Ther 2017; 16: 1114–1123. [ DOI ] [ PMC free article ] [ PubMed ] [ Google Scholar ]
- 73. Mao S, Sun W, Kissel T. Chitosan-based formulations for delivery of DNA and siRNA. Adv Drug Deliv Rev 2010; 62: 12–27. [ DOI ] [ PubMed ] [ Google Scholar ]
- 74. Urban-Klein B, Werth S, Abuharbeid S, et al. RNAi-mediated gene-targeting through systemic application of polyethylenimine (PEI)-complexed siRNA in vivo. Gene Ther 2005; 12(5): 461–466. [ DOI ] [ PubMed ] [ Google Scholar ]
- 75. Jeong JH, Mok H, Oh YK, et al. siRNA conjugate delivery systems. Bioconjug Chem 2009; 20(1): 5–14. [ DOI ] [ PubMed ] [ Google Scholar ]
- 76. Andreozzi P, Diamanti E, Py-Daniel KR, et al. Exploring the pH sensitivity of poly(allylamine) phosphate supramolecular nanocarriers for intracellular siRNA delivery. ACS Appl Mater Interfaces 2017; 9: 38242–38254. [ DOI ] [ PubMed ] [ Google Scholar ]
- 77. Halliwell B. Oxidative stress and cancer: have we moved forward? Biochem J 2007; 401: 1–11. [ DOI ] [ PubMed ] [ Google Scholar ]
- 78. Bernardini S, Tiezzi A, Laghezza Masci V, et al. Natural products for human health: an historical overview of the drug discovery approaches. Nat Prod Res 2018; 32(16): 1926–1950. [ DOI ] [ PubMed ] [ Google Scholar ]
- 79. Chikara S, Nagaprashantha LD, Singhal J, et al. Oxidative stress and dietary phytochemicals: role in cancer chemoprevention and treatment. Cancer Lett 2018; 413: 122–134. [ DOI ] [ PubMed ] [ Google Scholar ]
- 80. Imran M, Ullah A, Saeed F, et al. Cucurmin, anticancer, & antitumor perspectives: a comprehensive review. Crit Rev Food Sci Nutr 2018; 58: 1271–1293. [ DOI ] [ PubMed ] [ Google Scholar ]
- 81. Farooqi AA, Qureshi MZ, Khalid S, et al. Regulation of cell signaling pathways by berberine in different cancers: searching for missing pieces of an incomplete jig-saw puzzle for an effective cancer therapy. Cancers 2019; 11: 478. [ DOI ] [ PMC free article ] [ PubMed ] [ Google Scholar ]
- 82. Liu Y, Tang ZG, Lin Y, et al. Effects of quercetin on proliferation and migration of human glioblastoma U251 cells. Biomed Pharmacother 2017; 92: 33–38. [ DOI ] [ PubMed ] [ Google Scholar ]
- 83. Yang F, Song L, Wang H, et al. Quercetin in prostate cancer: chemotherapeutic and chemopreventive effects, mechanisms and clinical application potential (review). Oncol Rep 2015; 33(6): 2659–2668. [ DOI ] [ PubMed ] [ Google Scholar ]
- 84. Wang H, He X. Nanoparticles for targeted drug delivery to cancer stem cells and tumor. Methods Mol Biol 2018; 1831: 59–67. [ DOI ] [ PMC free article ] [ PubMed ] [ Google Scholar ]
- 85. Zhao Z, Zheng L, Chen W, et al. Delivery strategies of cancer immunotherapy: recent advances and future perspectives. J Hematol Oncol 2019; 12: 126. [ DOI ] [ PMC free article ] [ PubMed ] [ Google Scholar ]
- 86. Sung YK, Kim SW. Recent advances in the development of gene delivery systems. Biomater Res 2019; 23: 8. [ DOI ] [ PMC free article ] [ PubMed ] [ Google Scholar ]
- 87. Vaiserman A, Koliada A, Zayachkivska A, et al. Nanodelivery of natural antioxidants: an anti-aging perspective. Front Bioeng Biotechnol 2020; 7: 447. [ DOI ] [ PMC free article ] [ PubMed ] [ Google Scholar ]
- View on publisher site
- PDF (588.0 KB)
- Collections
Similar articles
Cited by other articles, links to ncbi databases.
- Download .nbib .nbib
- Format: AMA APA MLA NLM
Add to Collections
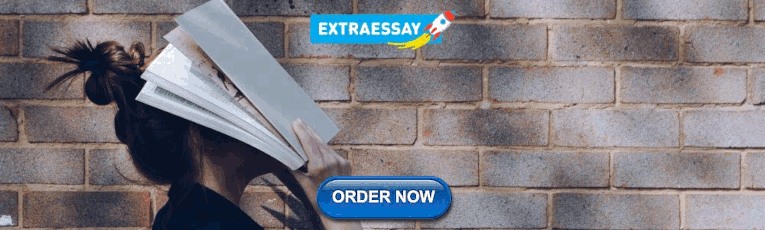
IMAGES